-
PDF
- Split View
-
Views
-
Cite
Cite
Christopher Green, Antoaneta Bilyanska, Mags Bradley, Jason Dinsdale, Lorraine Hutt, Thomas Backhaus, Frank Boons, David Bott, Chris Collins, Sarah E. Cornell, Mark Craig, Michael Depledge, Bob Diderich, Richard Fuller, Tamara S. Galloway, Gary R. Hutchison, Nicola Ingrey, Andrew C. Johnson, Rachael Kupka, Peter Matthiessen, Robin Oliver, Stewart Owen, Susan Owens, John Pickett, Sam Robinson, Kerry Sims, Pete Smith, John P. Sumpter, Svetlana Tretsiakova‐McNally, Mengjiao Wang, Tom Welton, Katherine J. Willis, Iseult Lynch, A Horizon Scan to Support Chemical Pollution–Related Policymaking for Sustainable and Climate‐Resilient Economies, Environmental Toxicology and Chemistry, Volume 42, Issue 6, 1 June 2023, Pages 1212–1228, https://doi.org/10.1002/etc.5620
- Share Icon Share
Abstract
While chemicals are vital to modern society through materials, agriculture, textiles, new technology, medicines, and consumer goods, their use is not without risks. Unfortunately, our resources seem inadequate to address the breadth of chemical challenges to the environment and human health. Therefore, it is important we use our intelligence and knowledge wisely to prepare for what lies ahead. The present study used a Delphi‐style approach to horizon‐scan future chemical threats that need to be considered in the setting of chemicals and environmental policy, which involved a multidisciplinary, multisectoral, and multinational panel of 25 scientists and practitioners (mainly from the United Kingdom, Europe, and other industrialized nations) in a three‐stage process. Fifteen issues were shortlisted (from a nominated list of 48), considered by the panel to hold global relevance. The issues span from the need for new chemical manufacturing (including transitioning to non‐fossil‐fuel feedstocks); challenges from novel materials, food imports, landfills, and tire wear; and opportunities from artificial intelligence, greater data transparency, and the weight‐of‐evidence approach. The 15 issues can be divided into three classes: new perspectives on historic but insufficiently appreciated chemicals/issues, new or relatively new products and their associated industries, and thinking through approaches we can use to meet these challenges. Chemicals are one threat among many that influence the environment and human health, and interlinkages with wider issues such as climate change and how we mitigate these were clear in this exercise. The horizon scan highlights the value of thinking broadly and consulting widely, considering systems approaches to ensure that interventions appreciate synergies and avoid harmful trade‐offs in other areas. We recommend further collaboration between researchers, industry, regulators, and policymakers to perform horizon scanning to inform policymaking, to develop our ability to meet these challenges, and especially to extend the approach to consider also concerns from countries with developing economies. Environ Toxicol Chem 2023;42:1212–1228. © 2023 Crown copyright and The Authors. Environmental Toxicology and Chemistry published by Wiley Periodicals LLC on behalf of SETAC. This article is published with the permission of the Controller of HMSO and the King's Printer for Scotland.
Abstract
HORIZON SCANNING FOR CHEMICAL ISSUES TO INFORM POLICY
Pollution, climate change, and biodiversity loss represent three planetary crises faced by society that require urgent, international action (United Nations Environment Programme [UNEP], 2021c). The interconnections among these environmental threats and the need to tackle them holistically are being increasingly recognized (UNEP, 2021c). From the chemicals perspective, it is crucial that efforts to tackle climate change and biodiversity loss and to drive forward sustainable development and circular economy do not result in harmful trade‐offs in pollution that could undermine recent progress toward breaking the trend of environmental decline. There is overwhelming evidence from environmental degradation studies that burgeoning consumption globally, associated with increasing affluence, has diminished or canceled out any gains from technological change aimed at reducing environmental impact (Wiedmann et al., 2020). To ensure that the accelerated transition to a benign circular economy is achieved in a manner that does not further degrade the environment, move the pollution impacts to elsewhere in the product or process life cycle (e.g., embedding nanomaterials into composites reduces exposure risk during use phase but makes recycling much more challenging), or shift the problems to another geographical location (e.g., export of plastic waste or products that are at end of life) requires foresight and a holistic understanding of synergies.
Chemicals are an intrinsic part of our everyday lives, with a wide range of applications from consumer products and food to healthcare, construction, transport, crop protection, and green energy (UNEP, 2019). The use of chemicals in society is anticipated to evolve, with some substances also providing the solutions needed in climate change mitigation and our ability to address the UN Sustainable Development Goals (SDGs). The chemical and related sciences are central to (1) the development of clean and sustainable forms of energy, for example, through an efficient capture of solar energy and clean fuel cells; (2) the application of green chemistry principles and processes to manufacturing and for materials substitution; (3) the efficient and affordable recycling of resources in short supply, including “endangered elements” and natural products, in a safe and environmentally sound way; and (4) the development of new analytical techniques needed for more effective monitoring of the environment (Matlin et al., 2015).
Policies are being set internationally and in individual countries to reduce pollution, such as UNEP's Towards a Pollution Free Planet (UNEP, 2017), the European Union's “Zero Pollution” Action Plan (European Commission, 2021), and actions of the United Kingdom's 25‐year environment plan (HM Government, 2018a), each of which recognizes the importance of effective management of chemicals in a safe and environmentally sound way. This is increasingly challenging because it is estimated that there are already over 350,000 chemicals and mixtures of chemicals available for production and use globally (Wang et al., 2020). Innovation exceeds the pace of regulation, and because chemicals are released into ecosystems throughout their life cycle, some cause harmful effects to wildlife and humans who have little opportunity to adapt to them or avoid them (Collins et al., 2020). The Lancet Commission on Pollution and Health estimated that pollution is responsible for 9 million premature deaths each year globally and up to 1 in 4 deaths in the most polluted countries (Landrigan et al., 2018). In 2019, it was estimated that 2 million lives and 53 million disability‐adjusted life‐years were lost as a result of contact with chemicals for which exposures could be reduced or eliminated through environmental management (World Health Organization [WHO], 2021).
Chemical production and trade have become increasingly globalized, and supply chains for chemicals and chemicals in products have become increasingly complex; but international progress on chemicals management has been slow. The SDG Target 12.4, to achieve environmentally sound management of chemicals and wastes worldwide throughout their life cycle by 2020, was not achieved. Achieving SDG Target 3.9 (to substantially reduce the number of deaths and illnesses from hazardous chemicals and air, water, and soil pollution and contamination) by 2030 is also not on track, with few countries even able to report on official indicators. Official development assistance from donor countries to respond to pollution‐related disease and death through better pollution control and management is inadequate to meet this global challenge (Fuller et al., 2022). Despite these targets, sales from the global chemicals industry are expected to double by 2030, with the strongest growth rates predicted outside the Organisation for Economic Co‐operation and Development (OECD) countries, mainly in Asia, Africa, and the Middle East—shifting production from developed to low‐ and middle‐income countries (UNEP, 2019). Some have argued that “novel entities”—including new substances and new forms of existing substances—push Earth's systems beyond a “safe operating space” because annual production and releases are increasing at a pace that outstrips the global capacity for assessment and monitoring, risking the erosion of vital ecosystems and ecosystem services and further threatening human health and well‐being (Diamond et al., 2015; Persson et al., 2022).
History provides numerous examples of the need to identify and heed early warnings regarding chemical impacts. Environmental legislation tends to be retrospective because the pollution problem usually needs to become highly visible before policy is created and attempts to reverse the damage are implemented. Initial reactions to the suspicion, or manifestation, of harm have typically included denial that a problem exists, exploitation of uncertainty (Oreskes & Conway, 2010; Wynne, 2010), and claims that the costs of preventative action would be prohibitive. The protracted nature of these conflicts is well documented in the European Environment Agency (EEA) reports Late Lessons from Early Warnings (2001, 2013), which include case studies on hazardous substances (dichlorodiphenyltrichloroethane [DDT, insecticide], polychlorinated biphenyls, chlorofluorocarbons, tributyltin (biocide), lead in petrol, mercury and Minamata disease, the pesticide dibromochloropropane, antimicrobials used as growth promoters, and many others). There is a critical need to move toward an approach where there is greater foresight of potential problems before they manifest themselves aligned with the precautionary principle. One way to achieve this is through horizon scanning, a systematic examination of potential future problems and threats, potential opportunities, and future developments (van Rij, 2010). Horizon scanning is often employed to explore novel and unexpected issues, including weak signals at the fringes of current thinking and policy agendas. Through horizon scanning, it is possible to identify knowledge gaps, which provide insights to shape research agendas, which in turn feed into more resilient policy decisions and facilitate a remedial action based on early warnings of harm.
Horizon scanning encourages a consideration of a wide variety of issues that can sit outside the immediate focus of a policy area, the future relevance and impact of which may not be immediately apparent from within that policy area but which can bring significant disruption or unintended impacts if not considered in the context of a longer‐term view of future changes. It is an extension of the meta‐analytic approaches used more widely to identify research gaps or historical trends and emphasis changes over time. For example, a data‐driven analysis of 20 years of publications in the journal Environmental Science & Technology identified plastics and per‐ and polyfluoroalkyl substance (PFAS) as clear emerging topics in the past 5 years (Zhu et al., 2021). However, a recent analysis of the scientific literature shows that only a minute fraction of relevant chemicals are well researched, while the vast majority receive almost no attention (Kristiansson et al., 2021). Horizon scanning, therefore, has an important role to play in systematically identifying and planning our responses to potential future pollution problems and to direct research and policy development toward realistic approaches to solving them. However, there is currently a lack of regular horizon scanning and early warning mechanisms internationally for chemicals and pollution (Wang et al., 2021).
To explore ways of developing greater foresight in chemicals management, England's Environment Agency has designed the Prioritisation and Early Warning System, which aimed to identify and prioritize emerging substances of concern to inform policy actions (Sims et al., 2021). The concept was reviewed by the United Kingdom's Hazardous Substances Advisory Committee (HSAC), an independent expert group that advises the Department for the Environment, Food and Rural Affairs (Defra), which highlighted its utility for identifying specific substances of concern. It also recommended exploring wider horizon scanning to identify novel and emerging issues of concern as well as new technologies and approaches that would be beneficial in supporting more forward‐looking chemicals policy, including a chemicals strategy at Defra (Sumpter et al., 2021). The HSAC specifically recommended adoption of a horizon scanning approach that had previously shown some utility in identifying threats to biodiversity over successive years (Sutherland et al., 2010). These scans have identified 15 topics annually since 2009 that could have major positive or negative effects on the future of conservation of global biodiversity but that have a low awareness within the scientific community. They aim to increase the likelihood of identifying issues as they materialize but which are still sufficiently distant for action to avert crises or to seize the opportunities to be taken. The utility of the approach has been noted in the authors' retrospective analysis of their previous horizon scans (Sutherland et al., 2019). Because pollution is one of the top five drivers of biodiversity loss, some topics relevant to a chemicals horizon scan have already been identified, including per‐fluorinated compounds (Sutherland et al., 2011), RNA‐based gene‐silencing pesticides (Sutherland et al., 2018), the increased use of pharmaceuticals as populations age (Sutherland et al., 2014), multigenerational effects of endocrine disruptors (Sutherland et al., 2021), and, most recently, new refrigerants and neonicotinoid replacements (Sutherland et al., 2022). Supporting Information, Table S1, shows the chemical‐relevant topics identified in 12 years of horizon scans by the biodiversity conservation community.
Drawing on the HSAC's recommendations and the work of the biodiversity conservation community, we present the first horizon scan specifically for emerging chemical pollution issues. We utilized expert insights from a multidisciplinary, multisectoral, and multinational panel to identify 15 issues that could have a positive or negative impact on chemical pollution in the environment in the United Kingdom and globally in the future. While driven by a desire for greater foresight on emerging issues related to chemical pollution in the environment for United Kingdom policymaking, it appreciated that many issues may have wider international relevance and that pollutants simply do not respect borders. The horizon scan aimed to increase recognition and encourage a wider dialogue about future risks and opportunities from innovations, investments, new policies, and emerging issues that society may wish to address to effectively manage chemicals, reduce their environmental or health impacts, and achieve wider environmental goals while better understanding synergies and potentially harmful trade‐offs. This comes at a pivotal moment as the international community aims to accelerate action toward goals set by the Paris Agreement and the United Nations Framework Convention on Climate Change, and new frameworks with goals for 2030 are being set under the Convention on Biological Diversity, the UN's International Conference for Chemicals Management, and the UN's Environment Assembly (UNEA). Concurrently, the COVID‐19 pandemic posed significant challenges, while discussions on the concept of “green recovery” continue to consider how to build back economies recognizing environmental crises. Finally, the establishment of the Intergovernmental Science‐Policy Body for Chemicals, Waste and Pollution (Wang et al., 2021), to operate along similar lines as the well‐respected UN Intergovernmental Panel on Climate Change (IPCC) is underway. Horizon scanning of emerging environmental threats from chemicals could thus become a central tool, feeding into national, regional, and intergovernmental decision‐making, policy‐setting, and long‐term monitoring, enabling systematic gathering of information on relevant trends and developments (monitoring) and exploration of their possible implications.
METHODOLOGY
The process took inspiration from the established methodology of Sutherland et al. (2010) using an expert panel to identify and shortlist a series of key issues, as shown schematically in Figure 1. Policy professionals from Defra were engaged from the study design phase to ensure that its outputs would be relevant to the development of chemicals and wider policy, including the chemical strategy which is under development. A core panel of 26 participants was recruited with the intention of being multidisciplinary, multisectoral, and multinational, although we note that the panelists are from industrialized nations, and thus this represents the views and concerns of developed economies and lacks a developing economies perspective at present. The panel consisted of experts from academia, a government agency, industry (consumer products, pharmaceuticals, agrochemicals, and water), nongovernmental organizations, and intergovernmental organizations (short biographies for panelists are included in Supporting Information, Table S2). Eight panelists were members of the United Kingdom government's independent scientific advisory group—the HSAC. The panel's expertise covered ecotoxicology and environmental fate, nanomaterials, endocrine disruption, pharmaceuticals, agrochemicals, landfill leachate, wastewater treatment, environmental governance, history of science, green chemistry, materials science, innovation and sustainability, sustainable consumption, polymers and plastics, climate change adaptation and mitigation, biodiversity and environmental change, planetary boundaries, global pollution, and remediation and global chemicals management (see Supporting Information, Table S2, for brief biographies of the Delphi panelists). All panelists were encouraged to reach out to their networks, including via social media, when identifying issues to raise. Members of the panel were kept anonymous from each other throughout the process, to obtain independent opinions from the contributors because independence is key to the Delphi process. Twenty‐three out of the initial 26 panelists completed the whole desk‐based process and are coauthors of the present study.
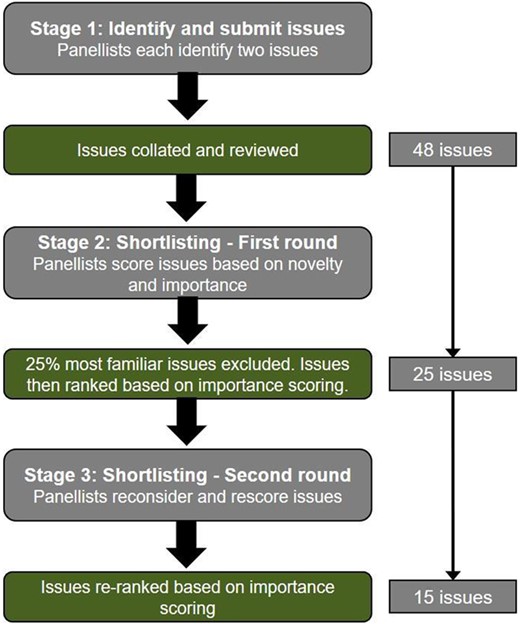
Methodology used for identifying and evaluating issues for the chemical priorities horizon scan.
In Stage 1, panelists were invited to submit two short abstracts (250 words each) describing two future issues that could have a positive or negative impact on chemical pollution in the environment in the United Kingdom or globally, which policymakers or other stakeholders may wish or need to respond to in the future. They were instructed to identify issues of greatest concern, where the concern may be due to changes in how chemicals are designed, used, released, or disposed of, including in products and processes, or how pollution can be understood and mitigated. Some issues could be expected to present new opportunities to reduce environmental pollution; others may present new threats, contributing to pollution in the future. Some issues may pose both a threat and an opportunity. The abstracts could also capture an emerging trend, a weak signal, or even a wild card (something where there is currently only one example). The threats/opportunities could even be as a result of an unexpected “trade‐off” from actions taken to benefit the environment. A template for collection of the issues was shared with the panelists, as shown in Supporting Information, Figure S1.
For inclusion in the present study, an issue was required to meet the following criteria, modified from the approach adopted by Sutherland et al. (2010). It must (1) be novel or represent novelty through a step‐change in impact, (2) have potentially substantive positive or negative impacts on chemical pollution in the environment at a United Kingdom or global level, and (3) appear likely to have greater impact in the future. In total, 48 unique issues were identified by the panel for shortlisting. Submitted issues were collated and checked in terms of their main topic focus to remove duplicate issues.
In Stage 2, the panelists were given the opportunity to comment on and score the issues based on two criteria: novelty and importance. For novelty, panelists were first asked to indicate how familiar they were with an issue on a four‐point scale, ranging from “Not at all familiar with the issue” to “The issue is well known to me.” The 25% most familiar issues submitted were excluded from subsequent stages of the process, to drive the shortlisting of novel issues. For importance, panelists were required to provide a single score, taking into account its potential impact (positive or negative) and the magnitude of this, its novelty, and its significance to chemical and related environmental policy on pollution. Consistent with Sutherland et al.'s (2010) approach, an issue could be awarded up to 1000 points, with higher values signifying greater importance. Each issue was required to have a score (so no scores of zero), and no two issues were allowed to have the same score. A four‐point indicative scale was provided to assist panelists with scoring, ranging from “Not important” (1–250) to “Critically important” (751–1000). Individual panelist scores were then ranked in order of relative importance from 1 to 48, with the issue they scored the highest receiving a score of 1 and the lowest receiving a score of 48. The panelists' rankings were then collated, and the median ranking for each issue was used to determine the final ranking of issues. The highest‐scoring 25 issues were then taken forward to the third stage.
In Stage 3, the scores and comments on the 25 shortlisted issues were distributed to the panelists. The panelists were then asked to reconsider their own importance scores for the 25 shortlisted issues in light of the collective scores and to alter their individual scores if they felt that it was appropriate to do so. As with the first round of scoring, the issues were then reranked based on their median importance scores to identify the panel's top 15 issues for future chemical pollution. The top 15 issues are presented below (see The Shortlisted Chemical Pollution Issues of Greatest Concern), and summaries of the additional issues that were not shortlisted are provided in Supporting Information, Table S3, to facilitate further discussion and retrospective analysis in the future.
Keywords related to the identified chemicals or materials (e.g., metals, PFAS, plastics, biochar, pesticides), environmental compartment (e.g., ecosystem, natural capital, landfill, wastewater treatment plants [WWTPs], freshwater, soil), and environmental policy topics (e.g., circular economy, precautionary principle, climate change) were identified manually for each of the 48 unique issues nominated from Stage 1 (Supporting Information, Table S4), which were fed into an association rule mining via the Apriori algorithm in python. The resulting “Support” values (shown in Supporting Information, Table S5) provide an indication of the popularity of these environmental topics as well as their co‐occurrence across the 48 topic submissions. These results were further visualized with Gephi, an open‐source network analysis and visualization software package. Specifically, the nodes of the input for Gephi were the keywords, and the edges between nodes were the corresponding “Support” values. The Force Atlas 2 Algorithm was chosen for the layout, with the visualization further optimized by moving a few nodes around manually. This is presented in Figure 2, where keywords that appeared in the top 15 issues (described in the following section) are highlighted in red.
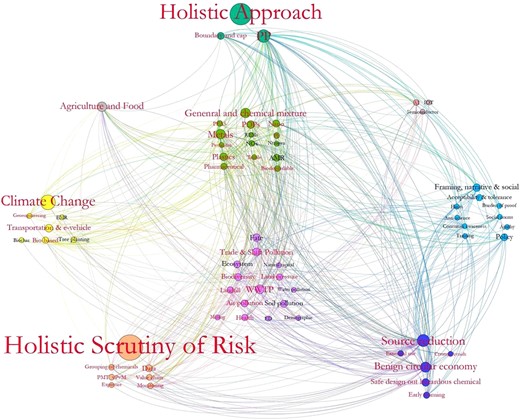
Analysis of the keywords included in 48 submitted issues showing the complicated interrelationships among the elements, with those that appeared in all 15 prioritized issued highlighted in red. The size of the bubbles and letters are proportionate to the frequency of occurrence of the elements in all topics. This was visualized using Gephi. PP = precautionary principle; PFAS = polyfluoroalkyl substances; POPs = persistant organic pollutants; REM = rare earth metals; AMR = antimicrobial resistance; AI = artificial intelligence; IOT = internet of things; EMR = enhanced mineral weathering; WWTP = wastewater treatment plant; ED = endocrine disrupting; PMT = persistent, mobile, and toxic; vPvM = very persistent, very mobile.
THE SHORTLISTED CHEMICAL POLLUTION ISSUES OF GREATEST CONCERN
Transitioning to nonvirgin fossil feedstocks in the chemicals industry
The modern chemical industry is highly dependent on feedstocks sourced from petroleum. Currently, demand for such feedstocks is forecast to grow significantly, accounting for a third of growth in oil demand by 2030 and almost half by 2050 (International Energy Agency [IEA], 2018), which provides a challenge for meeting the 1.5 °C target set out in the 2015 Paris Agreement. Business as usual is not an option (UNEP, 2019), but for organic chemistry neither is total decarbonization. Thus, an alternative carbon feedstock strategy is needed; but this is complex, and transitioning at scale toward biobased feedstocks could add substantial pressures to natural capital, food, biodiversity, and climate change, especially given projected future increases in production of plastics and chemicals. Projections indicate that 10%–20% of global chemicals production could utilize biobased feedstocks by 2025 (OECD, 2020a), which could have significant implications for land use and biodiversity if not addressed. There is an opportunity to bring together multiple stakeholders, including feedstock suppliers and end users, to create frameworks for faster adoption of alternative feedstocks, including biobased, carbon‐captured, and upcycled waste that reduces risks of undesirable trade‐offs. Further gains in resource efficiency may be made by considering more efficient use of chemicals and materials; designing out hazardous chemicals to facilitate a benign circular economy; innovative business models, such as chemicals as a service; and research and development into low‐carbon chemical and material production processes (European Commission, 2020).
“Green”‐bioderived chemicals, solvents, and materials
To enable the transition to nonvirgin fossil feedstocks in the chemicals industry, new biomass‐derived chemicals, solvents, and materials are being developed (Ragauskas et al., 2006). These are often perceived to be “green,” but little is known as yet about how they behave in the environment and how they might be recycled. Examples include new greener solvents (Clarke et al., 2018), which are already being found in waste streams (e.g., from pharmaceutical manufacturers). Lack of knowledge about the thermal stability and chemical properties (azeotrope formation) of green solvents coupled with little experience or training to implement them in chemical processes could result in these new solvents not being recycled but instead disposed of in less sustainable ways, such as by incineration. Similarly, “green” polymers are being developed using cellulose, lignin, and their composites, blended with plasticizers. It is generally assumed that these materials will be biodegradable (at least in industrial composting), but this has not been robustly demonstrated (Science Advice for Policy by European Academics, 2020). The lack of research into how these materials might be recycled could also lead to these polymers ending up in landfill. The recycling and waste disposal industries have little expertise and infrastructure for handling these new bioderived products, and they lack the research and development capability to investigate them and develop end‐of‐life solutions, particularly recycling options. This limits industry's ability to adapt to the new challenges of the circular economy and sustainable production and consumption.
Unintentional breakdown products of biodegradable chemicals
Consumer demand for “green” products is increasing, with biodegradability often being a key marketing claim. Hence, industry is keen to design biodegradable products, which theoretically should break down into innocuous chemicals, replacing environmentally persistent current products; however, this is not well studied. For chemicals that are persistent in the environment, factors such as their bioaccumulation and toxicity are used to determine whether the substance is of concern (e.g., persistent, bioaccumulative, and toxic chemicals; European Chemicals Agency, 2021; US Environmental Protection Agency, 2021). However, current methods for the assessment of the biodegradability of bulk substances (chemicals) generally rely on mass‐based measurements, such as changing biochemical oxygen demand in a test system (Jouanneau et al., 2014). Hence, regulations based on these existing approaches (Wik, 2013) fail to account for what the substance may break down into and what the environmental impacts of metabolites and breakdown products could be (with the exception of legislation addressing pesticides, biocides, and pharmaceuticals, which also addresses metabolites and their toxicity). As more biodegradable substances enter use, acceptable timescales should be defined and breakdown products accounted for. Another important consideration is how the substances are used. The routes of emission into the environment are very different for pharmaceuticals (Puhlmann et al., 2021) compared with plastics used in packaging (Song et al., 2009). Hence, it is vital to understand how people interact with the chemicals and materials to ensure that they are designed with end‐of‐life and circular economy principles in mind, to facilitate responsible reuse and disposal by the general public, and to ensure that appropriate regulatory actions are taken. The potential impacts of biological components in waste streams (e.g., wastewater sludge, agricultural mulches) should also be considered early, especially for persistent substances.
Identification of chemical threats using artificial intelligence
Understanding the toxicity of the hundreds of thousands of chemicals on the market presents a significant and costly challenge for risk assessment. There is, therefore, a demand for in silico computational models to replace animal tests in chemical safety assessments and to provide more economic and time‐efficient methods (Pérez Santín et al., 2021). In the field of medicine, great progress has been made in using various forms of artificial intelligence (AI)—notably deep learning and neural networks (Ciallella et al., 2021)—to identify drugs that have biological effects beyond those for which they were originally developed. This has been extremely beneficial, not least during the COVID‐19 pandemic. With these recent advances in AI technology, new opportunities are on the horizon for predicting which chemicals are likely to cause ecological damage or threaten human health, either singly or in complex mixtures. Some studies suggest that AI may be more accurate and reliable in predicting toxicity than animal tests to meet regulatory safety requirements (Pérez Santín et al., 2021), and they could help chemists identify hazards early in designing safe and sustainable chemicals or identifying less toxic alternatives to existing products (Hartung, 2019). In addition, bringing together data from thousands of field studies of the impact of chemical pollutants on freshwater, marine, and terrestrial organisms, populations, and communities using AI techniques offers the potential to revolutionize environmental management, predicting exposure and effects in situ.
Chemicals that we have never heard of being imported via products
Chemical regulations only cover substances and preparations and, to some extent, the known chemicals in articles (the European Union's Registration, Evaluation and Authorisation of Chemicals [REACH] term for products). There are no provisions for assessing the risks from new chemicals produced outside of the jurisdiction and imported via articles. How would we identify them? This flow of materials through trade makes regional controls less effective and points toward the need for a global policy (Wang et al., 2020, 2021). Indeed, chemicals that have been restricted or banned in many regions but not universally can be incorporated into a product legally manufactured in one region. They may then complete their life cycle in another region without knowledge of their presence, resulting in human exposure and issues for disposal and recycling. As non‐OECD countries become more innovative in producing new chemicals, without necessarily having the legislation in place to assess the risks from their use, importing countries will be increasingly exposed to the unknown chemicals that have not been assessed. This issue is exacerbating another issue—that of value chain transparency. Companies putting articles on the market face difficulties in knowing their full chemical composition: Contamination and increased use of nonvirgin materials provide a potential to prolong exposure to complex mixtures. Countries will have to cooperate to improve the traceability of chemicals in articles (products) that are traded worldwide (Wang et al., 2021).
Developing a sustainable future fiber industry
Textiles and their constituent fibers are found in clothing, home furnishings, and the growing nonwoven sector (e.g., sanitary products; wet wipes; medical personal protective equipment; and construction, automotive, filtration, and agricultural materials). Annual global fiber production is >105 million tonnes (Textile Exchange, 2021), with the United Kingdom fashion industry alone directly contributing £29.7 billion to the United Kingdom's gross domestic product (GDP) in 2016 (Fashion Roundtable, 2018). Concerns have been raised for over two decades regarding the adverse environmental impact of fiber production and the associated releases into the environment (Turley et al., 2009). Environmental damage results from microfiber pollution, toxic chemical additives and fire retardants, excessive water usage, greenhouse gas emissions, and minimal recycling, with >70% of garments ending up in landfill. A newly recognized aspect of the fiber threat is the enormous increase in demand for “fast fashion” as clothing costs fall, demand rises, and the global population increases. Currently, 62 million tons of garments are bought globally each year. More sustainable consumption is required because textile production is projected to increase to 102 million tons by 2030 (Environmental Audit Committee, 2019), with the potential of an associated increase in environmental damage. New policies and regulations aimed at minimizing the chemical footprint of the textile industry would offer huge opportunities to drive better business and manufacturing practices using novel, sustainable materials and alternative feedstocks and supporting reduced chemical use, leakage, and fiber loss (European Parliamentary Research Service, 2019).
Maximizing synergies between chemical risk reduction and climate change mitigation
International action on climate change (COP27), biodiversity (COP15), and chemical management (e.g., the Strategic Approach to International Chemicals Management [SAICM]'s Beyond 2020) marks a new opportunity for global environmental protection and sustainable development. However, siloed decision‐making to mitigate one threat raises concerns of large‐scale unnecessary trade‐offs. Examples include the following: (1) while the prospect of greener vehicles provides opportunities to contribute to climate mitigation, the current focus on electric vehicles without redesigning models of mobility (e.g., better public transportation and reduced vehicle travel) raises other concerns, which include the unsustainable level of increased demand for raw materials mining and processing (Hund et al., 2020), with attendant environmental and social impacts, and resource constraints for some metals (Dominish et al., 2019) that could create a demand for damaging seabed mining (Miller et al., 2018); (2) a focus on manufacturing efficiency over sustainability, combined with authorizations for continued use of some of the most hazardous chemicals in commerce, will continue to impair pursuit of a circular economy because of contamination of recyclates; (3) controversial geoengineering technologies could in the future spread large amounts of chemicals into the environment without properly accounting for either climatic or polluting side effects; and (4) biobased feedstocks (see above, Transitioning to nonvirgin fossil feedstocks in the chemicals industry). As laid out in UNEP's Making Peace with Nature report (2021c), there are opportunities to jointly tackle climate change, biodiversity loss, and pollution within the framework of the SDGs. Breaking down siloes through systems thinking, working across disciplines and policy areas, and joining up international actions would be beneficial and support holistic decision‐making. Likewise, the safer‐by‐design concept and essential use criteria could help to ensure that chemicals have an effective role in climate mitigation with trade‐offs in pollution minimized.
Recent experiences with PFAS setting new precedents for data sharing and chemicals management
Lack of data on chemicals in use has long been a challenge for surveillance, monitoring, risk assessment, and management, in part because of claims of confidential business information. This is despite the principle that “information on chemicals relating to the health and safety of humans and the environment should not be regarded as confidential” (SAICM, 2006). A recent case featured a chemical manufacturer effectively halting research into the presence of the PFAS C604 in the environment through patent infringement claims to halt the sale of an analytical reference standard for the compound; C604 had previously been detected in the Po River, Italy, near factories that make, use, or process it (Bettenhausen, 2021). This raises concerns regarding access to the standards needed for research to better understand the risks posed by contaminants of concern. The high persistence of PFAS, due to a strong carbon–fluorine bond, has led to them being popularly termed “forever chemicals.” Their accumulation in the environment, including in drinking water, has been a particular cause for concern. To manage risks from PFAS, new criteria have been proposed for hazard classification, including persistent, mobile, and toxic and very persistent, very mobile substances (Hale et al., 2020), with some suggesting that such chemicals could be managed as a class based on persistence alone (Cousins et al., 2019). Indeed, grouping approaches to manage PFAS as a class are being taken under the European Chemicals Strategy for Sustainability and through legislation in the United States (European Commission, 2020; Hogue, 2021). To achieve source reduction of hazardous chemicals like PFAS, limiting their consumption to essential use only is also being explored (Cousins et al., 2019), aligned with the European Union (EU) chemicals strategy. These precedents provide new options and approaches for management of chemical risks, particularly those posed by these classes of chemicals, that may be applied in the future.
Changes in landfill technology and waste composition may increase the need for effective leachate treatment to reduce release of persistent chemicals
Although an increasing number of global studies (Busch et al., 2010; Lang et al., 2017) have reported the presence of PFAS in leachates, data from landfills in the United Kingdom remain scarce (Hurst, 2019). In a study carried out by the Irish Environmental Protection Agency, concentrations of some polybrominated diphenyl ethers and PFAS have been found to be significantly higher in the leachate from newer, lined landfills than in samples from older, unlined landfills because they are designed to retain such contaminants (Harrad et al., 2020). Because of their high water solubility, PFAS were found to have high concentrations. These modern engineered landfills collect and remove leachates to prevent emissions to the environment. Some leachates can be treated within on‐site leachate treatment plants (LTPs) prior to discharge to WWTPs or directly to waterways. However, the mass flow of some of these chemicals increases following the treatments at LTPs or WWTPs, indicating a net formation of these compounds, possibly due to the degradation of precursors such as fluorotelomer alcohols, perfluoroalkyl phosphates, or fluorotelomer sulfonates (Schultz et al., 2006; Yan et al., 2015). Novel treatment technologies and updated permitting controls can abate/remove these substances (Rodríguez et al., 2004; Yin et al., 2017). Inside the landfill, limited, or a lack of, environmental degradation pathways coupled with continuing changes to the composition of waste (in the United Kingdom less organic matter is expected by 2035; HM Government, 2018b) could negatively affect the breakdown of these chemicals in landfill. This could increase their presence in leachates discharged to the environment if treatment technologies do not have a high enough removal efficiency. Further research and collaboration with landfill and wastewater industries will promote better understanding of the long‐term effects of persistent chemicals (Hurst, 2019; Rodríguez et al., 2004; Schultz et al., 2006; UNEP, 2001; Yan et al., 2015; Yin et al., 2017).
The need for a structured “weight‐of‐evidence” decision‐making approach to assess the significance of chemicals in the environment
For the past 30 years, European decision‐making on the risk and significance of chemical challenges has centered on laboratory ecotoxicity studies combined with field measurements of chemical residues. The resulting evidence is unfortunately not definitive when trying to establish real risks to wildlife and indeed can be misleading (Fahlman et al., 2021). There is uncertainty in translating laboratory observations to the field where both costressors and compensating factors exist (Johnson & Sumpter, 2016). Retrospective wildlife and chemical monitoring has the potential to overcome this (Milner & Boyd, 2017). Long‐term data sets showing changes in biodiversity and chemical concentrations over time can be used to identify associations between pollution and population changes. However, few countries currently have access to such extensive monitoring data. There is therefore an opportunity for existing monitoring programs to be better utilized and new ones created to identify effects of pollution on wildlife. When such data are used to test hypotheses through a series of transparent epidemiological criteria, we have an extraordinarily potent tool. Such criteria were proposed for human health in 1965 and include “strength of association,” “consistency,” and “temporality” (Hill, 1965). Such criteria, now more frequently described as “weight of evidence,” have been incorporated into chemical risk‐assessment procedures in a number of jurisdictions (see Agerstrand & Beronius, 2016; Johnson et al., 2021; Suter & Cormier, 2011). Importantly, the weight‐of‐evidence approach for decision‐making is the one most likely to achieve buy‐in from multiple stakeholders, including industry.
Advanced materials
There is a need to support and guide innovation in “smarter” technologies and materials to ensure that they have safe and sustainable roles in a green economy. Advanced materials have complex, innovative three‐dimensional structures and can be composites of organic and inorganic chemicals, including in the nano‐size range. They have many potential future applications in medicine, food, and consumer and engineering products. However, there is concern that these new materials have not been assessed for their safety and that the long‐term consequences are unknown. Many contain (eco‐)toxic substances that could leach from them, and some are highly persistent (Giese et al., 2020). How the advanced materials can be covered under existing chemical regulatory frameworks (e.g., REACH) remains unclear, and there is a lack of tools available for industry and regulators to test their safety. The nano‐scale sizes and the composite nature of many advanced materials also create challenges for end of life, limiting their recyclability and making them difficult to separate from the waste stream in a circular economy, as well as creating pathways for uncontrolled emissions to the environment. Some are simply examples of a single‐use, throwaway technology, such as plastic microchips (“How to Build a Disposable Microchip,” 2019). Applying the precautionary principle, the safe and sustainable by design concepts, a holistic scrutiny of risk across the life cycle, and effective risk management will reduce the potential for unexpected impacts of advanced materials on environment or human health and technological lock‐in, in the future.
Environmental impacts of emissions of chemicals from tire and road wear
Nonexhaust particle emissions from road traffic, specifically from tire and road wear, are of increasing concern (OECD, 2020b). Tire particles can release chemicals and their transformation products. In addition, environmental transformations can produce other chemicals of concern. There is awareness and regulation of pollution from exhaust emissions; however, nonexhaust emissions, such as chemicals and tire particles, are largely unregulated (Tkaczyk et al., 2021). Organic pollutants from nonexhaust emissions can end up in road runoff (Markiewicz et al., 2017). While the environmental and human health impacts from the use and release of chemicals used in the manufacture of tires are assessed as part of industrial chemicals legislations such as REACH, they are currently not a high priority for review. Tires and the bitumen‐based asphalt of road construction can release a range of chemicals, substantially aggravated by the thermolysis processes involved in wear. Research is continually identifying specific pollution problems. For example, environmental transformation of chemicals leaching from tires (Walsh et al., 2005) such as N′‐(1,3‐dimethylbutyl)‐N″‐phenyl‐para‐phenylenediamine (6PPD) produces the highly toxic 6PPD‐quinone, which was implicated in the mortality of coho salmon (Oncorhynchus kisutch) in urban creeks contaminated by stormwater (Tian et al., 2021). Despite the reactivity of these compounds (e.g., antioxidants), their transformation products are often unknown. Emerging evidence suggests a potential for road dust to contribute to the dispersal of pathogenic bacteria and their transfer between environmental compartments and between areas of high and low population density (Wang et al., 2022).
The purposeful and safe use of complex nanotechnology in food production and packaging
Food industries are aiming to improve metrics around food production and waste and reduce plastic usage in packaging in response to the drive for a greener economy and sustainability policies. Innovation in food security has seen an increase in the use of nanomaterials to enhance food sustainability through crop protection, food processing, packaging, and preservation with their efficient applications in functional food development (Nile et al., 2020). Adoption of these technologies will see increased quantities of nanomaterials added to products intended for human consumption and their release into the environment, with the long‐term impact unknown (Naseer et al., 2018; Quik et al., 2020). Hazard data are often limited for new technologies and replacement chemicals. The hazards of as‐produced nanomaterials over short time frames are relatively well understood; however, the effects of environmental transformations of nanomaterials on their long‐term effects need to be investigated (Svendsen et al., 2020), including their fate within food systems and the resulting potential for an indirect exposure. End of life for these products and use of contaminated sewage sludge as agricultural fertilizer potentially pose challenges when considering the fate of new nano‐forms in the environment, highlighting a need for full–life cycle analysis. The challenge with nanotechnology‐based foods for government and industry is to ensure consumer confidence and acceptance of foods produced utilizing nanotechnologies and precision agriculture (Zhang et al., 2021) and to develop and implement robust regulatory rules for the safe utilization of nanomaterials in food products (Zhao et al., 2021).
Heavy metal contamination of food products traded internationally
There are alarming reports of chemical contaminants, such as heavy metals and pesticides, being found at concerning levels in food products globally (European Food Safety Authority et al., 2021; Sarkar et al., 2021). Cereals, seafood, chocolate, and vegetables are convincingly shown to bioaccumulate lead, arsenic, cadmium, and mercury from either local contamination or long‐range aerosol deposition (Burney & Ramanathan, 2014). Accumulation in less varied diets, such as the infant diet, is a particular concern, with a recent report from the US House of Representatives (2021) detailing alarming levels of heavy metals in well‐known baby food brands. Countries' local food standards do not fully protect consumers from internationally traded products contaminated at source. For instance, Europe is the main importer of South American chocolate, which may explain the high lead and cadmium content of EU chocolate products (Argüello et al., 2019; WHO, 2011). China and India are the largest rice suppliers in the global market, and their rice also contributes the highest cancer risk from arsenic exposure (Meharg et al., 2013). Severely high concentrations of lead have been found in spices imported to New York and North Carolina from India (Hore et al., 2019). Further challenges, beyond harmonization of food protection regulations, include the need for reduction of pollution globally, especially at the sources of food production, and to tackle long‐range diffuse pollution, to avoid continued contamination of food products threatening global food security (Hou et al., 2020).
Continuing persistence of childhood lead poisoning
Despite the phase out of lead in gasoline and improvements in reducing lead in paint, one third of all children globally are lead‐poisoned (>5 µg/dl; Rees & Fuller, 2020). There is no safe level of lead exposure (Vorvolakos et al., 2016); even low‐level exposure results in significant negative health impacts and reduced lifetime prospects, often to the poorest in society, further enhancing inequality. Lead permanently reduces intelligence, affecting school outcomes, limiting job potential, and reducing lifetime earnings (WHO, 2019). Children poisoned by lead are more likely to exhibit violent and antisocial behavior (Aizer & Currie, 2019), while reductions in lead poisoning are closely associated with drops in murder, rape, and violent crimes. Health impacts are also severe: Lead poisoning leads to heart and kidney damage and causes 900,000 premature deaths each year. The economic impact is substantial, costing the G20 countries up to 2% of GDP every year; in Africa that rate exceeds 4% (Rees & Fuller, 2020). The main sources of current world exposure come from unsafe recycling and smelting of up to 50% of all used lead acid batteries from vehicles and lead in food and consumer products, including spices, baby food, ceramics, and aluminum cookware, among others. These products are traded internationally, despite their toxicity. Preventing exposure is the only effective way to stop this ongoing damage. Without intervention, lead poisoning will continue to increase, along with the associated burden of diseases, often in the most vulnerable populations.
DISCUSSION
Chemical strategies are typically conceived based on today's world rather than tomorrow's, with environmental policies often being reactive, responding to problems after they have occurred, rather than prospective, pragmatic, and risk‐based. New issues constantly emerge, drawing the public eye and prompting political debate, which can divert attention—and resourcing—away from relevant health and environmental chemical threats. Chemicals are not equal when it comes to relative impact on health or the environment, and many only become a significant concern long after they first enter widespread use, as evidenced by the current regulatory focus on single‐use plastics and the PFAS family of chemicals. New chemicals are entering the market with little or no public awareness regarding potential adverse impacts. The world is changing around us, particularly in response to the COVID‐19 pandemic, obviating the need to tackle deteriorating environmental quality. More proactive policymaking, regulation, and innovation that mitigates or develops resilience to future risks and harnesses new opportunities is facilitated by identifying issues early and engaging with them. This horizon scan identified 15 cross‐cutting chemical pollution issues that present opportunities or threats for tackling future, chemical‐related environmental challenges, both in the United Kingdom and globally. Their interconnections are highlighted in Figure 2, where the major themes that emerged include the need for a holistic approach to environmental risk management that takes a precautionary approach and considers chemicals risks in the broadest manner throughout their life cycle and in their intersections with each other (as mixtures) and with the circular economy and climate change and that considers an inclusive framing of the narrative around the issues to reflect societal needs.
While our focus was mainly the United Kingdom, all issues were considered by the panel to have global relevance. This is unsurprising because many countries face common chemical management issues, pollutants do not respect borders, and supply chains are highly globalized (e.g., heavy metals in internationally traded food, import of products containing unknown and unregulated chemicals). This highlights the ongoing importance of international cooperation on chemicals management via, for example, multilateral environmental agreements, the OECD, and the new framework to promote sound management of chemicals and wastes (the legacy of SAICM) under the UNEA science‐policy panel to contribute further to the sound management of chemicals and waste and to prevent pollution (adopted March 2, 2022; UNEA, 2019). These provide important opportunities for policymakers, industrialists, and scientists, among other stakeholders, to share experiences, develop new tools and approaches, and drive international action. The development of new 2030 targets for international chemicals and waste management is key, especially because such targets are being set in a decade where the size of the global chemicals industry is expected to double (UNEP, 2019) and petrochemicals are projected to drive 60% of oil demand growth (IEA, 2018). At the 2020 OECD Global Forum on the Environment “Towards Cost‐Effective Management Systems for Industrial and Consumer Chemicals,” participants determined that the generation of scientific knowledge on emerging issues and its dissemination are important first steps to help countries implement sound management of chemicals and waste (OECD, 2020a). Despite this, there is a recognized absence of effective horizon scanning and early warning processes among international bodies to identify future issues with potential impacts on chemical pollution (Wang et al., 2021). Bodies such as the OECD, professional organizations such as the Society for Environmental Toxicology and Chemistry, frameworks such as SAICM, and the new UNEA intergovernmental science policy interface for chemicals, waste, and pollution (UNEA resolution 5/8) would all benefit from their ability to bring together international representation from multiple stakeholders and could consider how to integrate horizon scanning into their future work plans in a collaborative way with each other.
The 2020s have been framed as a decisive decade for environmental policy because the international community urgently needs to tackle climate change and biodiversity loss. Pollution has previously been described as an issue neglected by both governments and the international development agenda (Landrigan et al., 2018). However, pollution is inherently interlinked with biodiversity loss and climate change as part of three planetary crises that should be tackled together (UNEP, 2021c). This was strongly reflected in this horizon scan, with one issue focusing specifically on the need to maximize synergies between climate action and chemical risk reduction. Another important point that emerged from the horizon scan considered the implications of the use of nonvirgin feedstocks in the chemicals industry and its potential trade‐offs on the potential for achieving the Paris Agreement's 1.5 °C target. Use of alternative feedstocks and biodegradable chemicals emerged from the horizon scan as concerns due to as yet uncertain implications for wildlife health (e.g., breakdown products of biodegradable chemicals, release of persistent chemicals from landfill leachates). Likewise, land‐use change associated with the growing of biofuels remains a concern for biodiversity conservation and applies also to the generation of alternative, non‐fossil‐fuel feedstocks. Internationally, the IPCC and the Intergovernmental Science‐Policy Platform on Biodiversity and Ecosystem Services have recently collaborated to consider the interlinkages between climate change and biodiversity loss (Pörtner et al., 2021). The secretariats of the Basel, Rotterdam, Stockholm, and Minamata conventions, alongside UNEP, have reported on the interlinkages between chemicals, wastes, climate change, and biodiversity loss (UNEP 2021a, 2021b). A key theme that emerged from this research is that horizon scanning, combined with whole‐systems thinking and the development of a stronger, holistic understanding of the interlinkages between these three environmental crises (chemicals, climate, and biodiversity) among scientists and decision makers, is crucial for reducing unanticipated trade‐offs and identifying win–win solutions to these crises.
Many of the issues identified in the present study are related to new technologies and innovations, which provided a mix of opportunities and threats (e.g., advanced materials and “green” chemicals). The eventual impacts of some issues on the environment were considered to depend on how the issue is approached. The biodiversity conservation horizon scans have also recently identified novel chemicals (new refrigerants and neonicotinoid replacements) as issues of concern (Sutherland et al., 2022). This indicates a continuing misalignment between development, comprehensive assessment, and regulation such that new compounds are developed and permitted before the full suite of risks has been assessed, adding to the legacy of chemicals that continue to have impacts decades after they were banned. The horizon scan identified a clear need to support innovation with safe and sustainable by‐design principles, including sustainable chemistry, to move toward “smarter” technologies and chemicals with useful new properties that can meet the requirements of a low‐ and/or zero‐carbon, toxic‐free circular economy while avoiding trade‐offs. Regrettable substitutions must be avoided, to avoid a repeat of past problems.
A key theme that emerged from the discussions and prioritization activities was that there are great opportunities for multistakeholder, cross‐sector, transdisciplinary work across the chemical life cycle to provide this support, which would benefit from more systems and holistic thinking. Foresight itself has an important role to play in the design and application of new materials and innovations to minimize harm, and clear lessons should be learned from past issues where the early warnings of threats (environmental persistence, transboundary effects, hazards) were not heeded soon enough (e.g., lead in petrol, asbestos, and DDT; EEA, 2001, 2013). New technologies and processes (e.g., AI) present opportunities for chemicals regulation and management, and precedents set from experience in managing specific chemical groups (e.g., PFAS) enable consideration of new ways of managing other classes of chemicals. Alongside innovations, the horizon scan provided new perspectives on old themes. The continued persistence and worsening of childhood lead poisoning, for example, provides a new take on one of the oldest anthropogenic pollutants whose human health effects were first observed by the first‐century Greek physician Pedanius Dioscorides, who observed that “Lead makes the mind give way” (EEA, 2013). Perfluorinated chemicals were identified by Sutherland et al. (2011). These pollutants have been studied for over 70 years (since their discovery in the 1940s and 1950s) but have garnered significant interest more recently with high‐profile lawsuits, new regulations, and the release of the film Dark Waters; and as we learn more about perfluorooctane sulfonic acid/PFAS, novel themes emerge such as new “hot spots.” A key goal for chemicals management is to avoid another situation like that of PFAS, where the issues were known but action was not taken in a timely manner. Thus, PFAS is considered by the panel of experts as an example of “what not to do” in the management of chemicals and the environment as a result of poorly thought‐through substitutions but where emerging ideas around their management and regulation as a class of chemicals is an interesting case study (Kwiatkowski et al., 2020). Issues surrounding nanomaterials have also been raised previously (e.g., nanosilver in wastewater in 2010 [Liang et al., 2010] and impacts on terrestrial ecosystems in 2013 [Colman et al., 2013]), but innovation and application of these technologies have continued to develop, while a new generation of advanced materials (e.g., black phosphorus–carbon black nanocomposite as a lithium‐ion battery electrode material; Park & Sohn, 2007) has arisen that pose similar regulatory challenges to the nanomaterial experience (Schwirn et al., 2021). While horizon scanning clearly has a role in identifying issues relating to new technologies and supporting safer future innovations, there is also a value in continuing to reflect on the lessons from the older and emerged themes to foresee new issues and unexpected legacies from the past.
It is important to note that the issues identified through Delphi methods, employed in the present study, are the product of the expertise of the panel who presented and shortlisted them. As such, the prioritized issues should be seen as the most pertinent of those submitted by a particular panel: A panel of different experts may have submitted and reached consensus on a different set of issues, and certainly a panel containing experts from developing nations may have identified different issues and priorities. A Delphi panel should consist of individuals with knowledge about the substantive area of research (Day & Bobeva, 2005). Biases are also reduced through confidential assessments and scoring to shortlist issues (Sutherland et al., 2019) as we did in the present study. Considering the heterogenous nature of our panel's expertise from within the chemicals field (and beyond), we believe that the shortlisted issues resulting from the present study certainly merit wider consideration, while acknowledging that there may be other issues not considered and not prioritized in the present study (see the long list of topics in Supporting Information, Table S3), which may wield greater future significance for the chemical pollution sphere.
Analysis of the popularity of the keywords in the 48 submissions (with those keywords identified in all 15 prioritized issues highlighted in red in Figure 2) indicated an emphasis on source reduction and a holistic approach by the majority of the panel members (see Supporting Information, Table S4, for full details of the keywords). Correspondingly, analysis of the co‐occurrence between these keywords identified the vast number of interconnections between the different topics and themes, as shown schematically in Figure 2. The importance of understanding synergies and holistic assessment, breaking siloes, and cross‐sector work to assist with risk management and to support innovations with minimal trade‐offs emerges clearly. Thus, from Figure 2, it becomes increasingly clear that a whole‐systems approach is needed to both scrutinize risks and horizon‐scan. The “One Health” approach, where multiple disciplines—working locally, nationally, and globally—unite in the quest to attain optimal health for humans, animals, and the environment, recognizing that each of these entities are integrated within a system, has been endorsed by the WHO, the Food and Agriculture Organization of the United Nations, the World Organization for Animal Health, and numerous national governments (Lombi et al., 2019). This approach has proven particularly valuable in the areas of (microbiological) food safety, zoonotic diseases, and antimicrobial resistance and has recently been proposed as a means to support agri‐nanotechnologies, which brings both the increased opportunities and increased intersectionality that cannot adequately be addressed by current discipline‐specific approaches alone (Lombi et al., 2019). The role of planetary boundaries and overconsumption, itself a major driver of climate change, also emerged strongly, not just to the production of chemicals themselves but throughout the life cycle and disposal of the products that the chemicals end up in.
We acknowledge that the issues shortlisted do not contain many great surprises for environmental scientists, except perhaps that supposedly legacy issues are not in fact addressed and are still causing problems even in industrialized economies. This is likely a feature of the fact that this is the first horizon scan focused on chemicals and pollution, and as such, it would be interesting to track the progress up the policy agenda also of the long‐listed topics (see Supporting Information, Table S3) which did highlight some less documented issues such as emissions from electric vehicles and solar panels and the potential negative impacts of recycled materials solutions, such as heavy metals from biochars and crushed silicates used as soil amendments, and from tree planting in riparian strips as a means of mitigating climate change: Thus, many of the issues that were long‐listed will be important to reflect on also as part of holistic policymaking to ensure that the seemingly “green” solutions do not lead to unintended consequences in the longer term. This further emphasizes the need for regular horizon‐scanning activities to track emerging issues of concern, allowing policy interventions to be applied before they become significant environmental pollutants.
Because the priority issues change continuously, there is value in proactively conducting further, regular horizon‐scanning exercises to provide ongoing global, regional, and national foresight as new issues emerge, to maintain vigilance. Horizon scanning and implementation of an early warning methodology to identify future issues with potential impacts on chemical pollution would be well placed as a regular function of the new global intergovernmental science‐policy panel for chemicals, waste, and pollution (Wang et al., 2021). As this is negotiated at the UNEA, in the short term, professional bodies with a wide membership and subsidiary science bodies of intergovernmental mechanisms could take on such a task in a collaborative way through regional hubs, as has been done previously to identify key research questions (Van den Brink et al., 2018). However, such an approach would have the greatest gain through follow‐up integration to ensure collaboration across regions to produce a truly global horizon scan. The relevance of the findings of a horizon scan could be further increased by broadening participation through engaging more early career researchers, more researchers from the Global South, and indigenous people with rich local knowledge into the nomination, discussion, and shortlisting processes. Thus, a key message from this first chemical horizon scan is to think broadly, consult widely, and act on the results, whereby the horizon‐scanning outputs could and should shape policies and influence chemical management.
CONCLUSIONS
Our horizon scan emphasizes the central role of chemicals in society and its response to global challenges and to meeting the United Nations SDGs, which could be compromised without a holistic understanding (in policymaking) of their risks during production, use and reuse (in an increasingly circular economy), and disposal, as illustrated by the cross‐cutting nature of the emerging issues that were prioritized. Both the long list of 48 issues and the 15 issues that were shortlisted should be of interest to a broad range of stakeholders, with the potential to generate discussion in the United Kingdom and internationally around emerging issues relating to chemicals and pollution. The identified issues of greatest concern for the environment include potential challenges arising from new chemical manufacturing approaches (including transitioning to non‐fossil‐fuel feedstocks), from novel materials, and from globalized trade challenging regulatory oversight of chemicals as well as opportunities from new tools for regulation including use of next‐generation risk assessment, greater data transparency, and the weight‐of‐evidence approach. The need for a precautionary approach and holistic systems‐wide and whole–life cycle approaches were evident in all 15 of the shortlisted topics. It is up to organizations to consider whether they need to monitor the discussed issues, conduct further research to better understand them, develop policy actions, or do nothing. Having awareness of these emerging issues may be the key to making effective decisions further down the line. Either way, the cross‐cutting nature of these issues calls for more collaborative decision‐making, and it is hoped that studies such as the present one will promote interdisciplinarity, a whole‐systems approach, and a crosstalk between sectors, researchers, policy teams, and other stakeholders to develop more coordinated approaches/actions to future chemical‐related environmental issues and greater consideration of interlinkages between chemicals and other environmental issues. Given the intersectionality of chemicals, climate, public health, and biodiversity, there is also a need for enhanced coordination of the national agencies tasked with the protection of human health and the environment.
There are a multitude of issues that will impact the future of chemicals, and the perceived importance of the various issues identified will vary based on individual perspective and circumstance, including degree of industrialization and policy focus. Nevertheless, the Delphi process concludes in a group consensus: a collective envisioning of the top issues that could have a significant impact on the future of chemical pollution. We strongly advise that those involved in the development of chemical strategy, policy, regulation, and chemical management give them equal consideration to other issues already on their radar.
Supporting Information
The Supporting Information is available on the Wiley Online Library at https://doi.org/10.1002/etc.5620.
Acknowledgments
We thank the Chemicals, Pesticides and Hazardous Wastes Team within the UK Department for Environment, Food and Rural Affairs (Defra) and the Environment Agency's Horizon Scanning & Futures Team for supporting this exercise. We thank the Defra steering group for their comments throughout the process. We also thank additional experts engaged by panelists and involved in the nomination of issues: the Sustainable Consumption Institute's Sustainable Materials for Consumer Products Working Group (biodegradable chemicals and nonvirgin feedstocks); M. Scheringer, N. V. Urho, Z. Wang, M. Diamond, and D. Santillo (maximizing synergies); I. Cousins and K. Bridgden (precedents set by the perfluoroalkyl substances experience); L. Schaefli (childhood lead poisoning); R. Blackburn and P. Goswami (development of a sustainable future fibers industry); and the Royal Society for Chemistry (green‐bioderived chemicals). The findings and opinions expressed are those of the authors and do not necessarily reflect the official views of their organizations.
Disclaimer
The authors declare no conflict of interest.
Author Contributions Statement
Christopher Green: Conceptualization; Formal analysis; Investigation; Methodology; Project administration; Writing—original draft; Writing—review & editing. Antoaneta Bilyanska: Data curation; Formal analysis; Project administration; Writing—original draft; Writing—review & editing. Mags Bradley: Data curation; Formal analysis; Project administration; Writing—original draft; Writing—review & editing. Jason Dinsdale: Formal analysis; Methodology; Writing—original draft; Writing—review & editing. Lorraine Hutt: Conceptualization; Formal analysis; Investigation; Writing—original draft; Writing—review & editing. Thomas Backhaus: Investigation; Writing—original draft; Writing—review & editing. Frank Boons: Investigation; Writing—original draft. David Bott: Investigation; Writing—original draft. Chris Collins: Investigation; Writing—original draft. Sarah E. Cornell: Investigation; Writing—original draft. Mark Craig: Investigation; Writing—original draft. Michael Depledge: Investigation; Writing—original draft; Writing—review & editing. Bob Diderich: Investigation; Writing—original draft; Writing—review & editing. Richard Fuller: Investigation; Writing—original draft; Writing—review & editing. Tamara S. Galloway: Investigation; Writing—original draft; Writing—review & editing. Gary R. Hutchison: Investigation; Writing—original draft; Writing—review & editing. Nicola Ingrey: Investigation; Writing—original draft. Andrew C. Johnson: Investigation; Writing—original draft; Writing—review & editing. Rachael Kupka: Investigation; Writing—original draft; Writing—review & editing. Peter Matthiessen: Investigation; Writing—original draft; Writing—review & editing. Robin Oliver: Investigation; Writing—original draft; Writing—review & editing. Stewart Owen: Investigation; Writing—original draft; Writing—review & editing. Susan Owens: Investigation; Writing—original draft; Writing—review & editing. John Pickett: Investigation; Writing—original draft; Writing—review & editing. Sam Robinson: Investigation; Writing—original draft; Writing—review & editing. Kerry Sims: Investigation; Writing—original draft; Writing—review & editing. Svetlana Tretsiakova–McNally: Investigation; Writing—original draft; Writing—review & editing. Pete Smith: Investigation; Writing—original draft. John P. Sumpter: Investigation; Writing—original draft; Writing—review & editing. Mengjiao Wang: Investigation; Visualization; Writing—original draft; Writing—review & editing. Tom Welton: Investigation; Writing—original draft. Katherine J. Willis: Investigation; Writing—original draft. Iseult Lynch: Formal analysis; Project administration; Writing—original draft; Writing—review & editing.
Data Availability Statement
All data are included in the Supporting Information for publication online.