-
PDF
- Split View
-
Views
-
Cite
Cite
Magali Houde, Zofia E. Taranu, Xiaowa Wang, Brent Young, P. Gagnon, Steve H. Ferguson, Michael Kwan, Derek C.G. Muir, Mercury in Ringed Seals (Pusa hispida) from the Canadian Arctic in Relation to Time and Climate Parameters, Environmental Toxicology and Chemistry, Volume 39, Issue 12, 1 December 2020, Pages 2462–2474, https://doi.org/10.1002/etc.4865
- Share Icon Share
Abstract
Mercury is found in Arctic marine mammals that are important in the diet of northern Indigenous peoples. The objectives of the present long‐term study, spanning a 45‐yr period, were to 1) investigate the temporal trends of total mercury (THg; muscle and liver) and selenium (Se; liver) in ringed seals (Pusa hispida) from different regions of the Canadian Arctic; and 2) examine possible relationships with age, diet, and climate parameters such as air temperature, precipitation, climatic indices, and ice‐coverage. Ringed seals were collected by hunters in northern communities in the Beaufort Sea, Central Arctic, Eastern Baffin Island, Hudson Bay, and Ungava/Nunatsiavut regions (Canada) between 1972 and 2017. Mercury levels did not change through time in seal liver, but THg levels in muscle decreased in seals from Hudson Bay (−0.91%/yr) and Ungava/Nunatsiavut (−1.30%/yr). Carbon stable isotope values in seal muscle decreased significantly through time in 4 regions. Selenium‐to‐THg ratios were found to be >1 for all years and regions. Variation partitioning analyses across regions indicated that THg trends in seals were mostly explained by age (7.3–21.7%), climate parameters (3.5–12.5%), and diet (up to 9%); climate indices (i.e., Arctic and North Atlantic Oscillations, Pacific/North American pattern) explained the majority of the climate portion. The THg levels had a positive relationship with Arctic Oscillation for multiple regions. Associations of THg with air temperature, total precipitation, and sea‐ice coverage, as well as with North Atlantic Oscillation and Pacific/North American pattern were found to vary with tissue type and geographical area. Environ Toxicol Chem 2020;39:2462–2474. © 2020 Her Majesty the Queen in Right of Canada. Environmental Toxicology and Chemistry published by Wiley Periodicals LLC on behalf of SETAC. Reproduced with the permission of the Minister of Fisheries and Oceans Canada.
Abstract
INTRODUCTION
Mercury (Hg) is of particular concern in the Arctic given the bioaccumulation and the toxicity of its methylated species, its high concentrations in marine mammals, and the importance of these animals as country foods for Indigenous peoples (Kirk et al. 2012; Arctic Monitoring and Assessment Programme 2015). The high levels of Hg in animals have led to health advisories for children, pregnant women, and women of childbearing age for the consumption of ringed seal liver in Nunavut and beluga whale meat (Delphinapterus leucas) in Nunavik, Canada (Nunavut Tunngavik Incorporated 2012; Lemire et al. 2014). Similarly, in the Faroe Islands, the general population is advised to limit consumption of pilot whale (Globicephala melas) meat to only one main meal of meat weekly and to avoid liver and kidney, in which Hg bioaccumulation is suggested to be most pronounced (Arctic Monitoring and Assessment Programme 2015).
The ringed seal (Pusa hispida) is a keystone species in Arctic food webs and has been an important biomonitoring animal for evaluating circumpolar trends of persistent organic pollutants and Hg. In Inuit Nunangat (homeland of the Inuit in Canada), a long‐term monitoring of Hg in seals has been supported by Government of Canada's Northern Contaminants Program for the past 25 yr. This program works closely with the Arctic Monitoring Assessment Programme, which contributes to international initiatives to reduce the global threats from contaminants and climate change, such as the Minamata Convention on Mercury (United Nations Environment Programme 2020). Effects of Hg in marine mammals, including Arctic species, have been previously summarized (Dietz et al. 2013, 2019; Scheuhammer et al. 2015; Desforges et al. 2016; Kershaw and Hall 2019) and include neurological changes (Krey et al. 2015; Ostertag and Chan 2018) as well as liver and kidney lesions (see Kershaw and Hall 2019 for review).
Reviews of Hg in Arctic biota, which have included results for ringed seals, have generally reported no consistent trends over time for circumpolar samples collected from the 1970s up to the early 2010s despite international action on Hg contamination (Rigét et al. 2011; Braune et al. 2015a). Patterns of accumulation are very species and site specific, and increases in Hg tissue concentrations have mostly been observed in marine mammals (Rigét et al. 2011; Braune et al. 2015a). Levels of Hg in marine mammals from the southern Beaufort Sea region of northern Canada have been reported to be higher than levels from Eastern Canadian and Western Greenland locations (Rigét et al. 2011, 2012; Braune et al. 2015a; Vorkamp and Muir 2016; Brown et al. 2018). Moreover, trends of Hg in Arctic biota have not been found to be representative of trends observed in the atmosphere, where slow declines of gaseous elemental Hg were observed over the 2000 to 2014 period (Hung et al. 2017; Steffen et al. 2017; Sharma et al. 2019; Wang et al. 2019). The recycling of legacy or previously emitted Hg between oceans, terrestrial environments, and the atmosphere is predicted to result in stable concentrations in marine biota on a global scale despite declines in the rate of primary emissions (Sunderland and Selin 2013; Wang et al. 2019).
Climate change can impact environmental factors, such as air and water temperatures, water chemistry, snow and rain precipitation rates, as well as sea‐ice coverage and thickness, which can lead to modifications in food web structures and dynamics. These changes can consequently alter the storage, transformation, transport, and accumulation of contaminants in environmental matrices and biota (Alava et al. 2017; Wang et al. 2019). More rapid biogeochemical and ecological changes have been observed in the Arctic, and Stern et al. (2012) have reviewed the influence of climate variability on Hg cycling, processes, and exposure in this polar region. For example, warmer temperatures and the loss of sea‐ice may allow for greater direct absorption of gas‐phase contaminants into surface waters, where they can be readily incorporated into the marine food chain. Longer ice‐free seasons can also influence the bioenergetics of primary consumers and fish, potentially leading to altered Hg transformation processes, influencing its distribution and transport, as well as higher uptake of Hg by biota (Stern et al. 2012; Wang et al. 2019).
A few studies have reported associations between Hg concentrations in marine mammal tissues (i.e., ringed seals and beluga) and environmental factors such as sea‐ice coverage and climate indices (Gaden et al. 2009; Rigét et al. 2012; Loseto et al. 2015). Associations have also been found between Hg levels and stable isotope values; isotopic ratios of nitrogen (15N/14N, expressed as δ15N) and carbon (13C/12C, expressed as δ13C) have been used to estimate wildlife trophic levels and feeding location, respectively. For example, a shift in diet (inferred from δ15N values) has been found to influence the accumulation of Hg in eggs of thick‐billed murres (Uria lomvia) from Nunavut, Canada (Braune et al. 2014). Moreover, correlations between total Hg (THg) concentrations and stable isotope values were reported in hair of polar bears from Beaufort Sea, Hudson Bay, and Barents Sea (Canada) and were associated with changing foraging patterns over time (McKinney et al. 2017; Lippold et al. 2020; Yurkowski et al. 2020).
Climatic indices (e.g., Arctic and North‐Atlantic Oscillations), which characterize surface atmospheric pressure/mass and wind patterns, could also influence the transport of contaminants to the Arctic. All this information underlines the important of investigating the influence of climate parameters on Hg accumulation in high‐trophic‐level Arctic organisms.
The main objectives of our long‐term study were to 1) evaluate the temporal trends of THg between 1970s and 2017 in liver and muscle tissues of ringed seals from different regions of the Canadian Arctic, and 2) investigate the possible relationships between THg bioaccumulation in seals, available biological/ecological information (e.g., age, sex, carbon and nitrogen stable isotopes), and climate parameters such as sea‐ice coverage, precipitation, and climatic indices. Seal liver and muscle tissues were collected from the same individuals because both tissues are consumed by Inuit. Trends of decreasing THg concentrations in time were expected in seals; associations between tissue THg concentrations, age of animals, and stable isotope values as well as environmental factors were also anticipated.
Mercury is mainly transferred in food webs in its organic form, methylmercury (MeHg), which is more efficiently assimilated into tissues following absorption. Marine mammals are capable of detoxifying, to some extent, MeHg through hepatic demethylation. Mercury can bind to selenium (Se) to form insoluble molecular groups, which may protect animals against Hg toxicity (Kershaw and Hall 2019). Selenium is an essential element that is incorporated into proteins and thus plays important roles within cells. In addition to forming a complex with Hg, Se can also facilitate the detoxification process by mediating the demethylation of organic Hg to inorganic Hg and reducing the digestive absorption of Hg (Spiller 2017). Calculating the ratio between these essential and nonessential elements represents a simple metric to evaluate imbalance. Temporal trends of Se and Se:THg ratios, based on molecular concentrations, were examined in the liver of a majority of seals.
MATERIALS AND METHODS
Sample collection
Liver and muscle samples were collected during local subsistence hunts from 22 sites in the Canadian Arctic (Figure 1). All sampling for this project was done by local hunters and was generally coordinated by Hunters and Trappers Associations/Committees. Hunting periods varied with locations, with usually summer hunts for communities further north and fall hunts for southern sites. Overall, >1500 ringed seals were collected between 1972 and 2017. For seal collection of unknown date, the time of capture was estimated, when possible, based on hunting periods from other years for specific sites. Sex, length, girth, and blubber thickness were determined in the field for a large proportion of the animals (Supplemental Data, Table S1). Samples were stored at −20 to −35 °C until analysis for THg and Se in liver and muscle.
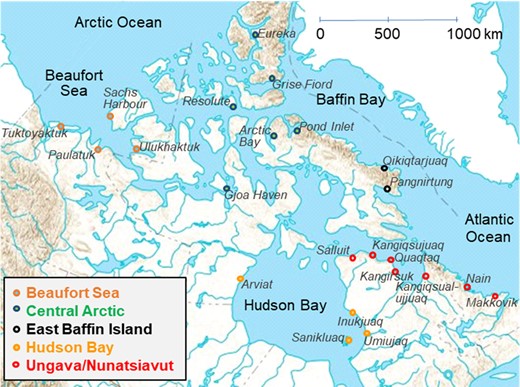
Ringed seal sampling sites across the Canadian Arctic (1972–2017). Results through time were analyzed according to regions, which are indicated by colors for each community.
Age and sex determination
Age of seals was determined by the Nunavik Research Centre (from 1998 to 2004; Nunavik, QC, Canada) and the Matson's Laboratory (after 2004; Milltown, MT, USA) by counting annual cementum growth layers of sectioned canine tooth using a compound microscope and transmitted light. Starting in 2006, DNA markers were analyzed in freeze‐dried muscle samples at the Wildlife Genetics International laboratory (Nelson, BC, Canada) to confirm sex of seals. Sex was determined based on the co‐amplification of a DNA segment from the sex‐chromosome–specific ZFX and ZFY genes. The presence/absence of the SRY gene, which is involved in male development, was used to confirm sex. The DNA results were in good agreement (>90%) with information from hunters in the field.
Stable isotope analyses
Stable carbon isotope δ13C and δ15N ratios were determined in non‐lipid–extracted muscle at the Environmental Isotope Laboratory, University of Waterloo (Waterloo, ON, Canada) as described in Houde et al. 2017; (Supplemental Data, Table S2). A subset of muscle samples (n = 45) were lipid extracted for comparison, and the results indicated minor differences (0.28‰ for δ13C and 0.25‰ for δ15N).
Chemical analyses
Analytical methodology and quality assurance approaches used for the determination of THg and Se in seal tissues collected over the period 1972 to 2017 are described in the Supplemental Data and in Supplemental Data, Table S3. The THg and Se were analyzed by 4 laboratories over the study period. Results for THg in liver and muscle samples from the 1970s were previously reported by Smith and Armstrong (1975) and for the mid‐1980s to mid‐1990s by Wagemann et al. (1995, 2000). The present results for THg in seal tissues from selected locations have been previously used in spatial trend studies: 1998 to 2001 data from 5 sites in Rigét et al. (2005), 2007 to 2010 data from 10 sites in Brown et al. (2016), and 2003 to 2015 results for Arviat in Yurkowski et al. (2020).
In brief, acid digestion followed by cold vapor atomic absorption spectrometry (CVAAS) was used for analysis of THg in all liver samples throughout the 45‐yr period as well as for THg in muscle until 2007. The detection limit for CVAAS was approximately 0.002 µg/g. Seal muscle tissue from 2007 to 2017 was analyzed for THg using US Environmental Protection Agency method 7473 (1998; using a Direct Mercury Analyzer; Milestone, Shelton, CT, USA; detection limit: <0.001 µg/g). Concentrations of Se in liver for samples from 1987 to 2009 were determined by atomic absorption spectrometry following acid digestion (Wagemann et al. 1996; Kwan 2011). From 2009 to 2017, Se was determined using inductively coupled plasma–mass spectrometry (detection limit of 0.01 µg/g) by the National Laboratory for Environmental Testing inorganic laboratory (Burlington, ON, Canada) following Environment and Climate Change Canada procedures (Environment Canada 2015).
All laboratories participated in interlaboratory quality assurance testing for THg starting in the early 1970s (Uthe et al. 1971) and including Se in the 1980s (Wagemann and Armstrong 1988). Beginning in the early 1990s, the participating laboratories took part in the Northern Contaminants Program Quality Assurance Program, which has been operating on an annual basis since 1993 (see the Supplemental Data for details). Quality assurance steps utilized by all laboratories included the analysis of certified reference materials for THg and Se and reagent blanks with each batch of samples. Seven standard reference materials: dogfish liver (DOLT‐2, ‐3, ‐4, and ‐5), dogfish muscle (DORM‐2, ‐3, ‐4, ‐5), and TORT‐2, NIST‐1566B, and 2976 mussel tissue were run during analyses; the percentage of recovery ranged between 87 and 112% (Supplemental Data, Table S4).
Environmental parameters
Results from individual communities were grouped by geographical regions for analyses (i.e., Beaufort Sea, Central Arctic, Eastern Baffin Island, Hudson Bay, and Ungava/Nunatsiavut). These regions were characterized by environmental parameters such as ice coverage, air temperature, total precipitation, and climate indices (i.e., Arctic and North Atlantic Oscillations; Pacific/North American pattern), for which information for the capture period was available. The Canadian Ice Service archive was utilized for site‐specific information on the monthly mean sea‐ice coverage as well as new, first‐year, and multiyear ice coverage (Canadian Ice Service 2020; see the Supplemental Data for definition of ice types). The same database was consulted for the annual total accumulated ice‐coverage for the year of capture as well as for the year preceding the hunts. Historical air temperature and total precipitation (rain and snow) data for arctic communities were obtained for the months of capture from the Government of Canada Historical Climate Data website (Government of Canada 2019a). Climatic oscillation indices including Arctic Oscillation (AO), North Atlantic Oscillation (NAO), and Pacific/North American pattern (PNA) for years of capture and years before sample collection were obtained from the US National Weather Service Climate Prediction Centre website (National Oceanic and Atmospheric Administration 2020). Data for air temperature and precipitation were found to be available for the period 1987 to 2017 and data from 1972 to 2017 for ice‐coverage and climate indices. Definitions of the climatic indices can be found in the Supplemental Data.
Data analyses
Analyses aimed to evaluate whether 1) Hg and Se contamination in seals were similar among geographical regions; 2) Hg and Se levels were associated in time with biological parameters such as age, sex, blubber thickness, and stable isotopes values; and 3) relationships/interactions between chemical and environmental variables were similar among regions. Statistical analyses were conducted using R Ver 3.5 (R Core Team). Statistical tests showing a probability <0.05 were considered significant. When multiple tests were conducted on a given response variable (i.e., trend and correlation tests across all regions as well as within regions), the false discovery rate (FDR; Benjamini and Hochberg 1995) was applied to correct the significance values (using the p. adjust function in R with method = “BH” for Benjamini and Hochberg, also called the false discovery rate).
Metal concentrations were summarized by sex, year, and region using geometric means and 95% confidence intervals for statistical robustness. Concentrations of THg and Se were compared among regions for 2016 to 2017 with a Wilcoxon rank score test proposed by Koenker (2005) because values were not normally distributed. Temporal trends for chemical, biological, and environmental variables were examined with years by computing linear regression. Metal concentrations were found through regression analyses to be linearly increasing with age (up to age 15, which represented 85% of aged animals); therefore age was considered during time trend analyses by regressing THg/Se concentration data versus both time (in years) and age. The unique effect and significance of time for these age‐adjusted trend analyses were visually represented using the effects function and package in R (Fox and Weisberg 2018). Associations of stable isotopes and environmental conditions (current and previous year) with metal concentrations were assessed with Spearman correlations and visually represented using the corrplot function and package in R (Wei and Simko 2017). Variation partitioning analysis (varpart function from the vegan package in R; Bocard et al. 1992; Legendre and Legendre 2012) was then used to quantify the unique and joint proportion of variation in THg accumulation in seal liver explained by each variable—age, time, diet (δ15N and δ13C), and environmental parameters using data collected after 1999. The venneuler function in R (Wilkinson 2011) was used for the visual representation (Venn diagrams) of the variation partitioning results, where overlapping portion of the circles indicate variation jointly explained by 2 (or more) variables, and non‐overlapping portions illustrate the unique variation explained by a given variable.
RESULTS AND DISCUSSION
Biological parameters
Co‐analysis of biological and chemical data indicated that, overall, sex of seals did not influence the accumulation of THg and Se in tissues (p value for THg in liver: 0.0852, Hg in muscle: 0.5272, and Se in liver: 0.0802). Such observations have been reported before in ringed seals (Dietz et al. 1998; Rigét et al. 2012). Sex differences in Hg accumulation in liver and muscle of adult Lake Saimaa ringed seals have, however, been reported (Lyytikäinen et al. 2015). The results also indicated that metal concentrations in liver and muscle increased with age of animals to approximately 15 yr of age for THg and 20 yr for Se in liver (age of all seals ranged between <1 and 42 yr‐old; p < 0.0001). Trends for older animals, which constituted approximately 15% of the samples, were inconsistent. Dehn et al. (2005) observed a similar pattern with age for accumulation of THg in liver of seals from Alaska and the Beaufort Sea. Differences between life stages of ringed seals and different species of Hg have also been previously reported (Wagemann et al. 2000; Ewald et al. 2019). Accumulation in liver may be explained by continuous uptake of Hg and MeHg through a changing diet over time as well as relatively slow elimination resulting in half‐lives estimated to be approximately 50 d for THg in blood of sled dogs (Lieske et al. 2011) and humans (Yaginuma‐Sakurai et al. 2012) and 50 d for MeHg in ringed seals (Ewald et al. 2019). Increased concentrations with age were also observed in muscle of seals but to a lesser extent. Furthermore, it was observed that age of seals collected during hunts decreased in time at all sites, indicating a possible bias in sampling by easier access to young‐of‐the‐year and juvenile seals in more recent years or taste preference of hunters for younger animals (Supplemental Data, Table S1).
Links between metal accumulation and feeding ecology (i.e., trophic levels and carbon source), were reported by the Brown et al. (2016) and Yurkowski et al. (2020) for Canadian Arctic ringed seals. Age and trophic position have also been found to influence Hg accumulation in Greenland ringed seals (Rigét et al. 2012). In the present study, THg concentrations in liver (p = 0.0024) and muscle (p < 0.0001) were found to significantly increase with δ15N values (mean stable isotope ratios for each year can be found in the Supplemental Data, Table S2). Increases in δ15N values were observed over time in seals from the Central Arctic and Hudson Bay, but trends were, however, not statistically significant; δ15N levels were also stable through time for the other regions (Figure 2). Constant piscivorous diet and trophic level position were also recently reported for ringed seals from Hudson Bay (Yurkowski et al. 2020). The diet of ringed seals at higher latitudes has been shown to consist mainly of Arctic cod (Boreogadus saida) compared with capelin (Mallotus villosus) and sand lance (Ammodytes sp.) in southern regions where isotopic niches are larger and prey opportunity greater (Yurkowski et al. 2016a). Ringed seals from higher latitudes, where the ice‐free season is shorter and interannual variability in ice phenology greater, have also been found to spend less time in a resident state associated with foraging behavior than animals from lower latitudes (Yurkowski et al. 2016b). Changes in sea‐ice type and length of open water periods related to climate changes may affect the foraging ecology and isotopic niches of seals and the distribution of their prey in the High Arctic (Yurkowski et al. 2015, 2016b).
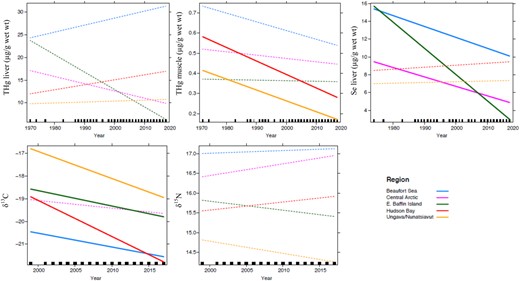
Age‐adjusted time trends of total mercury (THg) concentrations (µg/g wet wt) in liver and muscle as well as selenium (Se) in liver of ringed seals from different regions of the Canadian Arctic. Trends of carbon (δ13C) and nitrogen (δ15N) stable isotopes in seal muscle are also presented through time (with age correction as well). Solid lines = significant trends (p < 0.05); dashed lines = nonsignificant trends (p > 0.05). Note: ≤5 years of data available for liver analyses at East Baffin Island.
Moreover, δ13C values in seal muscle decreased significantly through time in 4 regions (Figure 2; p < 0.0001), which could suggest a change in feeding habits and a shift in diet toward pelagic and/or offshore preys. The decline in δ13C values could also be linked to the anthropogenic combustion of fossil fuel and deforestation, which decrease the δ13C value of surface ocean carbon reservoirs and increase the oceanic CO2 concentrations, a phenomenon known as the Suess effect (Keeling 1979; Newsome et al. 2007; Dietz et al. 2009). The annual rates of δ13C decline in seals were estimated to be −0.006 ± 0.23% for the Central Arctic, −0.008 ± 0.33% for Beaufort Sea, −0.031 ± 0.60% for East Baffin Island, −0.057 ± 0.32% for Hudson Bay, and −0.110 ± 0.43% for Ungava/Nunatsiavut. These rates were similar or larger than the Suess effect predicted values (−0.006 to −0.008%.yr; Tagliabue and Bopp 2008) or reported value (−0.011 ± 0.001%/yr; de la Vega et al. 2019) for the Arctic. In addition to carbon availability, baseline δ13C values in Arctic waters may further be affected by other factors such as the phytoplankton physiology, community composition, and changing sea‐ice conditions (de la Vega et al. 2019). The increasing use of carbon associated with phytoplankton was suggested to explain decreasing δ13C trends observed in Hudson Bay seals (Yurkowski et al. 2020). Values for δ13C were, however, not found to influence THg accumulation in seals from that study. Decreasing trends in muscle THg and δ13C were observed in belugas from Western Hudson Bay (Gaden and Stern 2010). The authors suggested that the increasing temperatures and longer duration of the ice‐free period over time in Hudson Bay may favor offshore foraging on less contaminated prey.
Regarding general body condition, the blubber thickness of animals from the Hudson Bay and Ungava/Nunatsiavut regions was found to increase over the study period (p = 0.0006 and 0.0009, respectively), and positive associations between blubber thickness and THg concentrations in liver (p = 0.0024), muscle (p < 0.0001) and Se in liver (p = 0.0379) were found over time. Previous studies have reported that ringed seals from Western Hudson Bay (Arviat, Nunavut) and Nunatsiavut consume lower trophic level organisms (Yurkowski et al. 2015; Brown et al. 2016) compared with other Canadian Arctic sites. This finding suggests that seals from the 2 most southerly regions may have been finding prey more easily or may have had more time to forage for food and build up fat reserves in more recent years. In relation to sea‐ice, it was recently reported that ringed seal body condition decreased with later sea‐ice breakup date in Hudson Bay (Yurkowski et al. 2020). Moreover, in the Beaufort Sea, a decreasing mean annual body condition of ringed seals has been reported from 1994 to 2011, and negative correlations were found between body condition and the timing of later ice clearance in spring (Fisheries and Oceans Canada 2015; Harwood et al. 2015). Decreasing blubber thickness was also observed in seals from Ulukhaktok (Northwest Territories, Canada; 1972–2010; Addison et al. 2014). Results suggest that ringed seals across Inuit Nunangat are responding to changing climatic factors that influence prey availability and environmental conditions (Harwood et al. 2012; Yurkowski et al. 2020).
Spatial trends
Recent results for samples collected in 2016 and 2017 indicated that liver THg concentrations in seals followed the west–east trend Beaufort Sea > Central Arctic ~ Hudson Bay > East Baffin Island > Ungava/Nunatsiavut in seals (Table 1). Spatial trends for THg in muscle were slightly different and followed the order Central Arctic > Beaufort Sea > Hudson Bay > East Baffin Island > Ungava/Nunatsiavut. The highest hepatic THg levels reported for the western Canadian Arctic in the present study were similar to early assessments conducted in the 1980s to 1990s (Wagemann et al. 1995; Muir et al. 1999) and in the more recent study by Brown et al. (2016) for ringed seal samples collected between 2007 and 2011. Rigét et al. (2005) found a similar geospatial trend in THg based on ringed seals samples collected during the period 1995 to 2001 from 11 Arctic locations ranging from northern Alaska to the White Sea in Western Russia; highest THg levels were found in samples from the southeastern Beaufort Sea. Information on Hg in Canadian Arctic marine mammals summarized by Brown et al. (2018) also corroborates this west–east trends in hepatic burden.
Most recent mean concentrations (±standard deviation; µg/g wet wt) of total mercury (THg) and selenium (Se) measured in liver and muscle of ringed seals at different sites across Inuit Nunangata
Region | Site | Year of sampling | THg (liver) µg/g wet wt | THg (muscle) µg/g wet wt | Se (liver) µg/g wet wt | Se:THg (liver) |
Beaufort Sea | Sachs Harbour | 2016 | 22.3 ± 20.1 | 0.32 ± 0.22 | 10.0 ± 5.88 | 1.34 ± 0.36 |
2017 | 9.60 ± 7.50 | 0.23 ± 0.12 | 6.03 ± 2.90 | 2.33 ± 1.25 | ||
Paulatuk | 1993 | 4.20 ± 1.30 | 0.17 ± 0.07 | 3.99 ± 1.25 | 2.63 ± 0.68 | |
Tuktoyaktuk | 2005 | 17.3 ± 23.9 | 0.21 ± 0.05 | 11.2 ± 9.1 | 3.69 ± 3.92 | |
Ulukhaktok | 2010 | 21.6 ± 13.0 | 0.52 ± 0.14 | 11.6 ± 6.26 | 1.80 ± 1.84 | |
Hudson Bay | Arviat | 2016 | 11.9 ± 13.5 | 0.37 ± 0.39 | 7.0 ± 5.4 | 2.81 ± 2.13 |
2017 | 15.0 ± 18.9 | 0.21 ± 0.99 | 8.4 ± 7.9 | 3.59 ± 3.98 | ||
Inukjuak | 2007 | 2.85 ± 3.66 | 0.14 ± 0.09 | na | na | |
Central Arctic | Arctic Bay | 2009 | 5.31 ± 5.76 | 0.31 ± 0.17 | 3.49 ± 2.58 | 3.12 ± 2.03 |
Eureka | 1994 | 27.8 ± 39.6 | 0.69 ± 0.42 | 14.7 ± 17.5 | 2.06 ± 1.54 | |
Gjoa Haven | 2009 | 6.49 ± 7.79 | 0.31 ± 0.23 | 5.22 ± 3.96 | 5.55 ± 5.22 | |
Grise Fiord | 2008 | 9.26 ± 10.5 | 0.13 ± 0.12 | 5.10 ± 4.87 | 3.10 ± 2.70 | |
Pond Inlet | 2009 | 6.78 ± 7.21 | 0.29 ± 0.21 | 4. 37 ± 3.27 | 3.78 ± 3.18 | |
Resolute Bay | 2016 | 13.4 ± 9.77 | 0.62 ± 0.23 | 5.85 ± 3.87 | 1.29 ± 0.43 | |
2017 | 8.56 ± 5.04 | 0.51 ± 0.19 | 4.63 ± 2.61 | 1.84 ± 1.43 | ||
Eastern Baffin Island | Pangnirtung | 2011 | 5.89 ± 5.01 | 0.25 ± 0.11 | 3.84 ± 2.16 | 2.12 ± 0.72 |
Qikiqtarjuaq | 2005 | 9.11 ± 9.32 | 0.49 ± 0.12 | na | na | |
Ungava/Nunatsiavut | Kangiqsualujjuaq | 2002 | 5.65 ± 2.50 | na | 7.54 ± 2.41 | 3.68 ± 0.92 |
Kangiqsujuaq | 2002 | 1.82 ± 1.58 | na | 3.89 ± 1.53 | 8.27 ± 4.92 | |
Kangirsuk | 1998 | 20.3 ± 33.5 | na | 10.2 ± 13.4 | 2.34 ± 0.93 | |
Makkovik | 1998 | 10.4 ± 5.24 | na | 7.52 ± 2.59 | 2.18 ± 0.87 | |
Nain | 2016 | 6.30 ± 8.03 | 0.10 ± 0.04 | 4.17 ± 3.48 | 2.64 ± 1.30 | |
2017 | 14.9 ± 19.6 | 0.16 ± 0.15 | 7.78 ± 7.54 | 2.83 ± 2.11 | ||
Quaqtaq | 2002 | 8.20 ± 6.72 | na | 10.2 ± 6.84 | 3.21 ± 1.27 | |
Salluit | 1998 | 6.95 ± 8.32 | na | 4.77 ± 3.99 | 5.27 ± 3.34 |
Region | Site | Year of sampling | THg (liver) µg/g wet wt | THg (muscle) µg/g wet wt | Se (liver) µg/g wet wt | Se:THg (liver) |
Beaufort Sea | Sachs Harbour | 2016 | 22.3 ± 20.1 | 0.32 ± 0.22 | 10.0 ± 5.88 | 1.34 ± 0.36 |
2017 | 9.60 ± 7.50 | 0.23 ± 0.12 | 6.03 ± 2.90 | 2.33 ± 1.25 | ||
Paulatuk | 1993 | 4.20 ± 1.30 | 0.17 ± 0.07 | 3.99 ± 1.25 | 2.63 ± 0.68 | |
Tuktoyaktuk | 2005 | 17.3 ± 23.9 | 0.21 ± 0.05 | 11.2 ± 9.1 | 3.69 ± 3.92 | |
Ulukhaktok | 2010 | 21.6 ± 13.0 | 0.52 ± 0.14 | 11.6 ± 6.26 | 1.80 ± 1.84 | |
Hudson Bay | Arviat | 2016 | 11.9 ± 13.5 | 0.37 ± 0.39 | 7.0 ± 5.4 | 2.81 ± 2.13 |
2017 | 15.0 ± 18.9 | 0.21 ± 0.99 | 8.4 ± 7.9 | 3.59 ± 3.98 | ||
Inukjuak | 2007 | 2.85 ± 3.66 | 0.14 ± 0.09 | na | na | |
Central Arctic | Arctic Bay | 2009 | 5.31 ± 5.76 | 0.31 ± 0.17 | 3.49 ± 2.58 | 3.12 ± 2.03 |
Eureka | 1994 | 27.8 ± 39.6 | 0.69 ± 0.42 | 14.7 ± 17.5 | 2.06 ± 1.54 | |
Gjoa Haven | 2009 | 6.49 ± 7.79 | 0.31 ± 0.23 | 5.22 ± 3.96 | 5.55 ± 5.22 | |
Grise Fiord | 2008 | 9.26 ± 10.5 | 0.13 ± 0.12 | 5.10 ± 4.87 | 3.10 ± 2.70 | |
Pond Inlet | 2009 | 6.78 ± 7.21 | 0.29 ± 0.21 | 4. 37 ± 3.27 | 3.78 ± 3.18 | |
Resolute Bay | 2016 | 13.4 ± 9.77 | 0.62 ± 0.23 | 5.85 ± 3.87 | 1.29 ± 0.43 | |
2017 | 8.56 ± 5.04 | 0.51 ± 0.19 | 4.63 ± 2.61 | 1.84 ± 1.43 | ||
Eastern Baffin Island | Pangnirtung | 2011 | 5.89 ± 5.01 | 0.25 ± 0.11 | 3.84 ± 2.16 | 2.12 ± 0.72 |
Qikiqtarjuaq | 2005 | 9.11 ± 9.32 | 0.49 ± 0.12 | na | na | |
Ungava/Nunatsiavut | Kangiqsualujjuaq | 2002 | 5.65 ± 2.50 | na | 7.54 ± 2.41 | 3.68 ± 0.92 |
Kangiqsujuaq | 2002 | 1.82 ± 1.58 | na | 3.89 ± 1.53 | 8.27 ± 4.92 | |
Kangirsuk | 1998 | 20.3 ± 33.5 | na | 10.2 ± 13.4 | 2.34 ± 0.93 | |
Makkovik | 1998 | 10.4 ± 5.24 | na | 7.52 ± 2.59 | 2.18 ± 0.87 | |
Nain | 2016 | 6.30 ± 8.03 | 0.10 ± 0.04 | 4.17 ± 3.48 | 2.64 ± 1.30 | |
2017 | 14.9 ± 19.6 | 0.16 ± 0.15 | 7.78 ± 7.54 | 2.83 ± 2.11 | ||
Quaqtaq | 2002 | 8.20 ± 6.72 | na | 10.2 ± 6.84 | 3.21 ± 1.27 | |
Salluit | 1998 | 6.95 ± 8.32 | na | 4.77 ± 3.99 | 5.27 ± 3.34 |
Results from all other years can be found in the Supplemental Data, Table S1. Selenium‐to‐THg molar ratios in liver were calculated using µg/g wet weight concentrations converted to nM/g wet weight.
na = not analyzed.
Most recent mean concentrations (±standard deviation; µg/g wet wt) of total mercury (THg) and selenium (Se) measured in liver and muscle of ringed seals at different sites across Inuit Nunangata
Region | Site | Year of sampling | THg (liver) µg/g wet wt | THg (muscle) µg/g wet wt | Se (liver) µg/g wet wt | Se:THg (liver) |
Beaufort Sea | Sachs Harbour | 2016 | 22.3 ± 20.1 | 0.32 ± 0.22 | 10.0 ± 5.88 | 1.34 ± 0.36 |
2017 | 9.60 ± 7.50 | 0.23 ± 0.12 | 6.03 ± 2.90 | 2.33 ± 1.25 | ||
Paulatuk | 1993 | 4.20 ± 1.30 | 0.17 ± 0.07 | 3.99 ± 1.25 | 2.63 ± 0.68 | |
Tuktoyaktuk | 2005 | 17.3 ± 23.9 | 0.21 ± 0.05 | 11.2 ± 9.1 | 3.69 ± 3.92 | |
Ulukhaktok | 2010 | 21.6 ± 13.0 | 0.52 ± 0.14 | 11.6 ± 6.26 | 1.80 ± 1.84 | |
Hudson Bay | Arviat | 2016 | 11.9 ± 13.5 | 0.37 ± 0.39 | 7.0 ± 5.4 | 2.81 ± 2.13 |
2017 | 15.0 ± 18.9 | 0.21 ± 0.99 | 8.4 ± 7.9 | 3.59 ± 3.98 | ||
Inukjuak | 2007 | 2.85 ± 3.66 | 0.14 ± 0.09 | na | na | |
Central Arctic | Arctic Bay | 2009 | 5.31 ± 5.76 | 0.31 ± 0.17 | 3.49 ± 2.58 | 3.12 ± 2.03 |
Eureka | 1994 | 27.8 ± 39.6 | 0.69 ± 0.42 | 14.7 ± 17.5 | 2.06 ± 1.54 | |
Gjoa Haven | 2009 | 6.49 ± 7.79 | 0.31 ± 0.23 | 5.22 ± 3.96 | 5.55 ± 5.22 | |
Grise Fiord | 2008 | 9.26 ± 10.5 | 0.13 ± 0.12 | 5.10 ± 4.87 | 3.10 ± 2.70 | |
Pond Inlet | 2009 | 6.78 ± 7.21 | 0.29 ± 0.21 | 4. 37 ± 3.27 | 3.78 ± 3.18 | |
Resolute Bay | 2016 | 13.4 ± 9.77 | 0.62 ± 0.23 | 5.85 ± 3.87 | 1.29 ± 0.43 | |
2017 | 8.56 ± 5.04 | 0.51 ± 0.19 | 4.63 ± 2.61 | 1.84 ± 1.43 | ||
Eastern Baffin Island | Pangnirtung | 2011 | 5.89 ± 5.01 | 0.25 ± 0.11 | 3.84 ± 2.16 | 2.12 ± 0.72 |
Qikiqtarjuaq | 2005 | 9.11 ± 9.32 | 0.49 ± 0.12 | na | na | |
Ungava/Nunatsiavut | Kangiqsualujjuaq | 2002 | 5.65 ± 2.50 | na | 7.54 ± 2.41 | 3.68 ± 0.92 |
Kangiqsujuaq | 2002 | 1.82 ± 1.58 | na | 3.89 ± 1.53 | 8.27 ± 4.92 | |
Kangirsuk | 1998 | 20.3 ± 33.5 | na | 10.2 ± 13.4 | 2.34 ± 0.93 | |
Makkovik | 1998 | 10.4 ± 5.24 | na | 7.52 ± 2.59 | 2.18 ± 0.87 | |
Nain | 2016 | 6.30 ± 8.03 | 0.10 ± 0.04 | 4.17 ± 3.48 | 2.64 ± 1.30 | |
2017 | 14.9 ± 19.6 | 0.16 ± 0.15 | 7.78 ± 7.54 | 2.83 ± 2.11 | ||
Quaqtaq | 2002 | 8.20 ± 6.72 | na | 10.2 ± 6.84 | 3.21 ± 1.27 | |
Salluit | 1998 | 6.95 ± 8.32 | na | 4.77 ± 3.99 | 5.27 ± 3.34 |
Region | Site | Year of sampling | THg (liver) µg/g wet wt | THg (muscle) µg/g wet wt | Se (liver) µg/g wet wt | Se:THg (liver) |
Beaufort Sea | Sachs Harbour | 2016 | 22.3 ± 20.1 | 0.32 ± 0.22 | 10.0 ± 5.88 | 1.34 ± 0.36 |
2017 | 9.60 ± 7.50 | 0.23 ± 0.12 | 6.03 ± 2.90 | 2.33 ± 1.25 | ||
Paulatuk | 1993 | 4.20 ± 1.30 | 0.17 ± 0.07 | 3.99 ± 1.25 | 2.63 ± 0.68 | |
Tuktoyaktuk | 2005 | 17.3 ± 23.9 | 0.21 ± 0.05 | 11.2 ± 9.1 | 3.69 ± 3.92 | |
Ulukhaktok | 2010 | 21.6 ± 13.0 | 0.52 ± 0.14 | 11.6 ± 6.26 | 1.80 ± 1.84 | |
Hudson Bay | Arviat | 2016 | 11.9 ± 13.5 | 0.37 ± 0.39 | 7.0 ± 5.4 | 2.81 ± 2.13 |
2017 | 15.0 ± 18.9 | 0.21 ± 0.99 | 8.4 ± 7.9 | 3.59 ± 3.98 | ||
Inukjuak | 2007 | 2.85 ± 3.66 | 0.14 ± 0.09 | na | na | |
Central Arctic | Arctic Bay | 2009 | 5.31 ± 5.76 | 0.31 ± 0.17 | 3.49 ± 2.58 | 3.12 ± 2.03 |
Eureka | 1994 | 27.8 ± 39.6 | 0.69 ± 0.42 | 14.7 ± 17.5 | 2.06 ± 1.54 | |
Gjoa Haven | 2009 | 6.49 ± 7.79 | 0.31 ± 0.23 | 5.22 ± 3.96 | 5.55 ± 5.22 | |
Grise Fiord | 2008 | 9.26 ± 10.5 | 0.13 ± 0.12 | 5.10 ± 4.87 | 3.10 ± 2.70 | |
Pond Inlet | 2009 | 6.78 ± 7.21 | 0.29 ± 0.21 | 4. 37 ± 3.27 | 3.78 ± 3.18 | |
Resolute Bay | 2016 | 13.4 ± 9.77 | 0.62 ± 0.23 | 5.85 ± 3.87 | 1.29 ± 0.43 | |
2017 | 8.56 ± 5.04 | 0.51 ± 0.19 | 4.63 ± 2.61 | 1.84 ± 1.43 | ||
Eastern Baffin Island | Pangnirtung | 2011 | 5.89 ± 5.01 | 0.25 ± 0.11 | 3.84 ± 2.16 | 2.12 ± 0.72 |
Qikiqtarjuaq | 2005 | 9.11 ± 9.32 | 0.49 ± 0.12 | na | na | |
Ungava/Nunatsiavut | Kangiqsualujjuaq | 2002 | 5.65 ± 2.50 | na | 7.54 ± 2.41 | 3.68 ± 0.92 |
Kangiqsujuaq | 2002 | 1.82 ± 1.58 | na | 3.89 ± 1.53 | 8.27 ± 4.92 | |
Kangirsuk | 1998 | 20.3 ± 33.5 | na | 10.2 ± 13.4 | 2.34 ± 0.93 | |
Makkovik | 1998 | 10.4 ± 5.24 | na | 7.52 ± 2.59 | 2.18 ± 0.87 | |
Nain | 2016 | 6.30 ± 8.03 | 0.10 ± 0.04 | 4.17 ± 3.48 | 2.64 ± 1.30 | |
2017 | 14.9 ± 19.6 | 0.16 ± 0.15 | 7.78 ± 7.54 | 2.83 ± 2.11 | ||
Quaqtaq | 2002 | 8.20 ± 6.72 | na | 10.2 ± 6.84 | 3.21 ± 1.27 | |
Salluit | 1998 | 6.95 ± 8.32 | na | 4.77 ± 3.99 | 5.27 ± 3.34 |
Results from all other years can be found in the Supplemental Data, Table S1. Selenium‐to‐THg molar ratios in liver were calculated using µg/g wet weight concentrations converted to nM/g wet weight.
na = not analyzed.
Many factors such as geological influences, river and atmospheric input, and long‐range transport of Hg emissions from Asian sources may be influencing the region‐specific accumulation of Hg in seals (Kirk et al. 2012; Cole et al. 2014; Brown et al. 2018). Wang et al. (2018) have also suggested that differences in MeHg enrichment in subsurface waters may explain the higher biotic Hg concentrations in western regions where the MeHg peaks have been found to occur at shallower depth. Moreover, climate can be a major influence on Hg bioavailability and accumulation in biota (Wang et al. 2019). Given the relatively high THg hepatic levels, adult ringed seals from several regions of the Canadian Arctic (e.g., Sachs Harbour, Ulukhaktok, Resolute Bay, and Arviat) have been found to be at severe or high risk of Hg‐mediated health effects (Dietz et al. 2019).
In recent years, concentrations of Se were also significantly higher in liver of seals captured in the Beaufort Sea compared with other regions (Table 1; p = 0.0002–0.0453). Selenium levels in Hudson Bay seals were also greater than in animals from Central Arctic, East Baffin Island, and Ungava/Nunatsiavut (p < 0.0001–0.014). The nutrient–toxicant complex formation between Se and Hg has been considered protective against the direct toxic effects of Hg exposure. Calculation of the molar Se:THg ratio may be indicative of the overall protection of the animals; a ratio >1 is assumed to counteract the adverse effects of Hg (Kehrig et al. 2013). In the present study, Se:THg ratios were found to be >1 for all years/sites (means/site of 1.3–8.3; Table 1 and Supplemental Data, Table S1). Ratios were also found to decrease with age in seals from the Central Arctic (p < 0.01) and to be the lowest in Beaufort Sea and Central Arctic seals where the greater THg tissue contamination was found. Previous studies have also reported that Se:THg ratios exceeded 1:1 in liver of most ringed seals collected from Alaska and the Beaufort Sea between 1996 and 2001 and that ratios were negatively correlated with age (Dehn et al. 2005). Moreover, Wagemann et al. (2000) found a 1:1 ratio between Se and Hg in ringed seal livers from Ulukhaktok (Beaufort Sea; 1996) and Arviat (1992); the latter datasets were included in the present study. Modeling suggests that the Hg–Se complex formation occurs across life stages and may be higher in mature seal livers compared with young‐of‐the‐year seals (Ewald et al. 2019). The effect of the Hg–Se interaction depends on the form of Hg and Se, the organ, and the dose (Spiller 2017). The strong binding affinity of Hg with Se sites on proteins can also lead to the disruption of their functions (Spiller 2017) and to Se deficiency (Gajdosechova et al. 2016).
Temporal trends
Analyses indicated that levels of THg and Se significantly increased with age of seals, and therefore this variable was considered during time trend analyses. Temporal trends observed for THg and Se in seals were region specific (Table 2 and Figure 2). Results indicated that THg in liver of Canadian Arctic ringed seals generally did not vary significantly over time (Table 2 and Figure 2). In ringed seals from Greenland, Hg liver levels were found to increase at Avanersuaq and Ittoqqortoormiit between 1980s and 2010, with annual increases of 2%/yr and 10%/yr, respectively (Rigét et al. 2012); a decrease was observed in seals from Qeqertarsuaq (–3.1%/yr; 1994–2010). Global temporal trends of Hg in Arctic biota have not been consistent across species and regions (Rigét et al. 2011; Braune et al. 2015b). In general, higher numbers of time‐series with increasing THg in liver were found for arctic marine mammals based on results up to 2009 (Rigét et al. 2011).
Percentage (%/yr ±standard deviation) increase or decrease of total mercury (THg) and selenium (Se) concentrations in ringed seals collected in different regions of the Canadian Arctica
Beaufort Sea 1972–2017 | Central Arctic 1983–2017 | Eastern Baffin Island 1999–2011 | Hudson Bay 1989–2017 | Ungava/Nunatsiavut 1989–2017 | |
No. of yr of sampling | 20 | 20 | 8 | 19 | 13 |
THg (liver) | 0.73 (2.85) | −1.65 (7.28) | nab | 1.56 (8.52) | 1.32 (6.22) |
THg (muscle) | −2.29 (6.64) | −4.29 (19.4) | −2.29 (7.45) | −0.91 (4.10) | −1.30 (4.40) |
Se (liver) | −0.86 (2.77) | −1.30 (5.83) | nab | 0.06 (2.02) | −0.21 (3.70) |
Beaufort Sea 1972–2017 | Central Arctic 1983–2017 | Eastern Baffin Island 1999–2011 | Hudson Bay 1989–2017 | Ungava/Nunatsiavut 1989–2017 | |
No. of yr of sampling | 20 | 20 | 8 | 19 | 13 |
THg (liver) | 0.73 (2.85) | −1.65 (7.28) | nab | 1.56 (8.52) | 1.32 (6.22) |
THg (muscle) | −2.29 (6.64) | −4.29 (19.4) | −2.29 (7.45) | −0.91 (4.10) | −1.30 (4.40) |
Se (liver) | −0.86 (2.77) | −1.30 (5.83) | nab | 0.06 (2.02) | −0.21 (3.70) |
Numbers in bold indicate statistically significant trends (p < 0.05) of metal concentrations versus time based on age‐adjusted modeling and where p values were corrected for the multiple testing across all 5 regions using the false discovery rate (FDR).
na = value could not be calculated because data were available for ≤5 yr for this tissue.
Percentage (%/yr ±standard deviation) increase or decrease of total mercury (THg) and selenium (Se) concentrations in ringed seals collected in different regions of the Canadian Arctica
Beaufort Sea 1972–2017 | Central Arctic 1983–2017 | Eastern Baffin Island 1999–2011 | Hudson Bay 1989–2017 | Ungava/Nunatsiavut 1989–2017 | |
No. of yr of sampling | 20 | 20 | 8 | 19 | 13 |
THg (liver) | 0.73 (2.85) | −1.65 (7.28) | nab | 1.56 (8.52) | 1.32 (6.22) |
THg (muscle) | −2.29 (6.64) | −4.29 (19.4) | −2.29 (7.45) | −0.91 (4.10) | −1.30 (4.40) |
Se (liver) | −0.86 (2.77) | −1.30 (5.83) | nab | 0.06 (2.02) | −0.21 (3.70) |
Beaufort Sea 1972–2017 | Central Arctic 1983–2017 | Eastern Baffin Island 1999–2011 | Hudson Bay 1989–2017 | Ungava/Nunatsiavut 1989–2017 | |
No. of yr of sampling | 20 | 20 | 8 | 19 | 13 |
THg (liver) | 0.73 (2.85) | −1.65 (7.28) | nab | 1.56 (8.52) | 1.32 (6.22) |
THg (muscle) | −2.29 (6.64) | −4.29 (19.4) | −2.29 (7.45) | −0.91 (4.10) | −1.30 (4.40) |
Se (liver) | −0.86 (2.77) | −1.30 (5.83) | nab | 0.06 (2.02) | −0.21 (3.70) |
Numbers in bold indicate statistically significant trends (p < 0.05) of metal concentrations versus time based on age‐adjusted modeling and where p values were corrected for the multiple testing across all 5 regions using the false discovery rate (FDR).
na = value could not be calculated because data were available for ≤5 yr for this tissue.
In the present study, decreasing trends of muscle THg were observed in ringed seals from the Hudson Bay (–0.91%/yr) and Ungava/Nunatsiavut (–1.30%/yr) regions (Table 2 and Figure 2). In other Canadian studies, decreasing trends of THg levels in seal muscle were also reported in ringed seals from Arviat (Western Hudson Bay; 2003–2015; –3.8%/yr; Yurkowski et al. 2020) as well as in muscle from Arviat beluga (Gaden and Stern 2010). Moreover, stable THg concentrations have been reported in muscle of ringed seals from Ulukhaktok, southeastern Beaufort Sea, between 1973 and 2007 (Gaden et al. 2009). Total Hg has also been reported to be stable or decreasing, depending on the age group, in muscle and liver of migratory beluga whales from the Beaufort Sea collected between 2002 and 2012 (Loseto et al. 2015).
The decreasing trends of muscle THg we found in ringed seals from the Hudson Bay and Ungava/Nunatsiavut regions may reflect the decline in gaseous elemental Hg concentrations observed in the period 2006 to 2014 at Alert, in Nunavut (Cole et al. 2013; Sharma et al. 2019; Wang et al. 2019). Over the past decades, atmospheric Hg concentrations and deposition fluxes have declined in North America and Europe generally following the declining Hg point‐source emissions. However, in the past 10 yr, levels have been shown to stabilize and even increase in some of these regions (Wang et al. 2019). The presence of legacy Hg, and the diverse biogeochemical processes and metabolisms, could partly explain the differences in accumulation between tissues and geographical regions. Selenium concentrations have also decreased in seals from the Beaufort Sea and the Central Arctic regions. The Se:THg ratios in liver have, however, been found to be stable through time (nonsignificant data not shown). The fraction of THg inactivated by Se was estimated to be 53% in ringed seals liver (Wagemann et al. 2000; Dehn et al. 2005), leaving approximately half of the THg available for potentially toxicity.
Associations with climate parameters
Relationships were investigated between changing climate variables and THg and Se accumulation in ringed seals in regions where more than 8 yr of data were available (the East Baffin Island region was therefore excluded). Because environmental factors such as atmospheric patterns may have global influence, overall trends across the Canadian Arctic were evaluated as well as more specific trends by regions. A review of the few studies reporting ecological impacts of climate on Hg in Arctic biota has previously been published by McKinney et al. (2015).
Variation partitioning analyses by regions showed that age was the dominant factor explaining THg accumulation in seal liver (7.3–21.7% unique variation explained; 11.3% for the overall Canadian Arctic), climate accounted for 3.5 to 12.5% (unique variation; 4% for all regions combined when considering all climate factors included in the present study), and diet for 4.1% overall (0–9.1% unique variation by regions; Figure 3). Note that many variables co‐explained the variation in THg (i.e., 3.2% of the variation overall, 5.3% in Beaufort Sea, 3.1% in the Central Arctic, 10.2% in Hudson Bay, and 24.7% in Ungava/Nunatsiavut; see overlaps in Figure 3). It is known that climate has been changing through time, and an overlap of climate and year explained 0.2% of THg variation in liver when all regions were considered (0–16.5% by region; Figure 3).
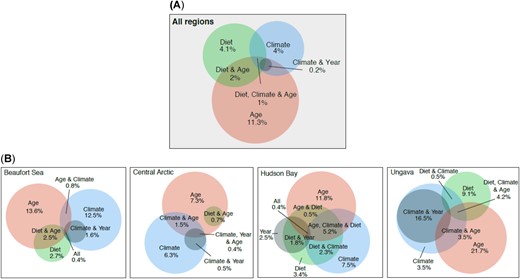
Venn diagrams presenting factors influencing total Hg concentrations in liver of ringed seals. (A) Overall Canadian Arctic. (B) Beaufort Sea, Central Arctic, Hudson Bay, and Ungava/Nunatsiavut.
Analyses by environmental factor groups (i.e., air temperature/total precipitation; total accumulated sea‐ice, monthly coverage for all types of ice; climate indices) indicated that climate indices (AO/NAO/PNA) explained the majority of the climate portion, with percentages ranging between 0.5 to 7% (mean of 3.8%; Supplemental Data, Figure S1). Ice‐coverage recorded on the month of capture also influenced THg accumulation in seals from the Beaufort Sea, Central Arctic, and Hudson Bay (3.5–4.1%).
Air temperature and total precipitation
When all regions were considered, the mean air temperature was found to be positively related to THg levels in liver and muscle of seals (Figure 4). The same observation was also made for Se in liver (Supplemental Data, Figure S2). A negative trend with air temperature was, however, observed for muscle THg levels in Central Arctic seals when specific regions were considered (Figure 4 and Supplemental Data, Table S5). In northern Canada, annual mean air temperatures have increased by 2.3 °C (range 1.7–3.0 °C) from 1950 to 2016, approximately 3 times the global mean warming rate, with the largest increases in the northwestern part of the country (Government of Canada 2019b).

Correlations between total mercury concentrations (THg) in liver and muscle of ringed seals and environmental factors for specific regions of the Canadian Arctic as well as overall. The size of the circles indicates the strength of the relationships. Gray indicates positive correlations and red negative associations. * = Based on the available data for the month of capture; AO = Arctic Oscillation; PNA = Pacific/North‐American pattern; NAO = North Atlantic Oscillation. Spearman correlations and p values are available in the Supplemental Data, Table S5.
The annual mean precipitation has also increased in Canada in the past decades, with larger relative increases in northern regions (Government of Canada 2019b). Total precipitation rates (including rain and snow) were found to be negatively correlated with THg muscle levels in seals in 3 regions (Beaufort Sea, Central Arctic, and Hudson Bay). Total Hg liver concentrations in seals from the Hudson Bay region were also negatively correlated with the total precipitation. A positive relationship was, however, found for THg levels in liver of seals from the Beaufort Sea. In the future, climate models project that temperature and precipitation in Canada will increase by 1.8 °C and 7%, respectively, in the current century under a low emission scenario and will increase by more than 6 °C and 24% under a high emission scenario, with more important changes in the north and during winter (Government of Canada 2019b). These environmental factors will therefore need to be monitored in conjunction to Hg in wildlife to better understand linkages.
Climate indices
Total Hg concentrations in seal liver were positively related to AO for multiple sites (Figure 4 and Supplemental Data, Table S5) when the data recorded for the years of seal capture were considered (see Supplemental Data, Table S1 for years of sampling). The AO index has also been found to be positively associated with Hg in liver of Greenland seals (Rigét et al. 2012) and in liver/muscle of beluga whales from the Beaufort Sea, for which correlations between THg concentrations and the Pacific Decadal oscillation were also found (Loseto et al. 2015). The AO is a see‐saw pattern characterized by winds circulating counterclockwise around the Arctic. The positive phase produces a strong polar vortex, with the midlatitude jet stream shifted northward. The AO variability affects the winds, and thus variations in the ocean circulation and ice drift in the Arctic and northern North Atlantic are closely tied to the index. The oscillation has been trending to more of a positive phase since the 1990s (National Oceanic and Atmospheric Administration 2014). The AO is known to be related to the NAO, which describes the strength and direction of westerly winds and location of storm tracks across the North Atlantic (Cohen and Barlow 2005). Similar to the AO, positive correlations were found between THg concentrations in both liver and muscle and NAO (Figure 4). Trends were, however, negative for THg in muscle in seals from Hudson Bay and Ungava/Nunatsiavut. The NAO index was not found to be linked with THg levels in muscle/liver of beluga, walrus (Odobenus rosmarus), or narwhal (Monodon monoceros) from the Hudson Bay region (Gaden and Stern 2010), although these researchers' dataset was limited to a maximum of 10 sampling years. Temperature and NAO were found to be important factors in THg accumulation through time in eggs of Arctic seabirds when time lags of 2 to 7 years were considered (Foster et al. 2019).
Moreover, THg tissue concentrations in seals were negatively associated with the PNA in the Hudson Bay and Ungava/Nunatsiavut regions when data from the year preceding capture were considered (Figure 4). Overall, results from associations with oscillation indices suggest that these patterns may influence Hg accumulation in seals. The AO seems to have a global positive link with THg in seals, whereas for other indices relationships are more complex and vary with tissue and site.
Type of ice and coverage
Total annual sea‐ice coverage recorded during the year of sampling, or the preceding year, was positively linked with liver/muscle THg when all regions were considered. These results suggest that, globally, higher ice‐coverage contributes to higher accumulation of Hg in seals, an observation also made for Se in seal liver (Supplemental Data, Figure S2). Region‐specific analyses indicated, however, some negative correlations for muscle. In addition, positive correlations were also found between the sea‐ice coverage during the month of seal capture and liver/muscle THg levels except for the Beaufort Sea region, where the trend was opposite (negative; similar observation for Se). Specific types of ice (i.e., first‐year and multiyear ice coverage; see the Supplemental Data for definition of ice types) were also evaluated and indicated similar results.
Beaufort Sea and the Central Arctic archipelago usually remain partly covered by ice in the summer because they contain a mix of multiyear and seasonal ice, whereas Hudson Bay and the Northern Labrador Sea are typically seasonal ice regions (Environment and Climate Change Canada 2019). Significant decreasing trends in sea‐ice have been observed throughout the Canadian Arctic from the 1950s to 2018, ranging from –4.3%/decade in the Central Arctic region to –15.6% in the Northern Labrador Sea. Multiyear ice has also been observed to decrease in Beaufort Sea over that period (–8.8%/decade in Beaufort Sea, –6.5% in Central Canadian Arctic; Environment and Climate Change Canada 2019).
Other studies have reported relationships between lengths of ice‐free season and THg concentrations in marine mammals. Gaden et al. (2009) found that THg in muscle of ringed seals from Ulukhaktok in the Western Canadian Arctic were higher following short and long ice‐free seasons. Tissue‐specific influence of sea‐ice concentrations have also been reported on accumulation of THg in Beaufort Sea beluga whales (Loseto et al. 2015). Moreover, relationships of Hg levels in ringed seals in Greenland and ice conditions were found to vary from site to site (Rigét et al. 2012), for example, positive associations were found between the global Arctic ice‐coverage index in seals from Avanersuaq. The influence of sea‐ice on the composition and the availability of prey items have been suggested to explain differences in associations and Hg accumulation among sites (Gaden et al. 2009; Rigét et al. 2012).
CONCLUSIONS
The results of the present study, spanning 45 yr of sampling, indicate that overall temporal trends of Hg concentrations in tissues of ringed seals in the Canadian Arctic have shown very limited declines over the last 5 decades. The only significant decreasing trends of THg were observed in muscle of seals from 2 regions (~1%/yr). Overall, the seal THg tissue concentrations are declining more slowly than reported declines in atmospheric gaseous elemental Hg in the Arctic, reflecting the complexity associated with the transformation and bioaccumulation of Hg in the marine environment. The THg concentrations in seal liver were found to be positively related to AO in multiple regions, whereas associations with other climate parameters were found to vary with tissue and geographical areas. Relationships between climate change and Hg levels in Arctic ecosystems are complex and multidirectional; integrating environmental and climate parameters in future analyses is therefore of importance to better understand and predict the impacts of a warming climate on Hg accumulation.
Supplemental Data
The Supplemental Data are available on the Wiley Online Library at https://doi.org/10.1002/etc.4865. They include information on the locations, years, numbers, and biological parameters of ringed seals; mean concentrations of THg (liver and muscle) and Se (liver) as well as Se:THg molar ratios in liver; mean stable isotopes values; analytical methods and quality assurance used through time; % of recovery for Hg and Se analyses; definitions of climate indices and types of ice; Venn diagrams for partitioning variations; and Spearman correlations between metal concentrations and climate factors.
Acknowledgment
We are grateful to the hunters in each northern community for their long‐term participation in this project. We thank B. Wolki and J. Kuptana (Sachs Harbour), T. Smith and J. Alikamik (Ulukhaktok), and L. Pijogge and R. Laing (Nain) for their help in sample collection and coordination as well as the Nunavik Hunters, Fishers and Trappers Association for coordinating sample collecting in Nunavik and the Nunavik Nutrition and Health Committee for its support and approval of the project. Thanks to the Hunters and Trappers Associations of Resolute Bay, Sachs Harbour, and Arviat, as well as the Nunavut Environmental Contaminants Committee, the Northwest Territories Regional Contaminants Committee, and the Environment Division of the Nunatsiavut Government for their support throughout the years. We also thank L. Harwood of Department of Fisheries and Oceans for provision of samples, L. Lockhart (Department of Fisheries and Oceans, retired) for providing the data for samples from the 1970s to 1990s, S. Tanabe (Ehime University, retired) for providing samples from Pangnirtung 1999, and J. Côté for help with data processing. This project was funded by the Northern Contaminants Program (Crown‐Indigenous Relations and Northern Affairs Canada) and by Environment and Climate Change Canada.
Disclaimer
The authors have no competing interests to declare.
Data Availability Statement
Data, associated metadata, and calculation tools are available from the corresponding author ([email protected]).