-
PDF
- Split View
-
Views
-
Cite
Cite
Paul J. Van den Brink, Alistair B.A. Boxall, Lorraine Maltby, Bryan W. Brooks, Murray A. Rudd, Thomas Backhaus, David Spurgeon, Violaine Verougstraete, Charmaine Ajao, Gerald T. Ankley, Sabine E. Apitz, Kathryn Arnold, Tomas Brodin, Miguel Cañedo‐Argüelles, Jennifer Chapman, Jone Corrales, Marie‐Agnès Coutellec, Teresa F. Fernandes, Jerker Fick, Alex T. Ford, Gemma Giménez Papiol, Ksenia J. Groh, Thomas H. Hutchinson, Hank Kruger, Jussi V.K. Kukkonen, Stefania Loutseti, Stuart Marshall, Derek Muir, Manuel E. Ortiz‐Santaliestra, Kai B. Paul, Andreu Rico, Ismael Rodea‐Palomares, Jörg Römbke, Tomas Rydberg, Helmut Segner, Mathijs Smit, Cornelis A.M. van Gestel, Marco Vighi, Inge Werner, Elke I. Zimmer, Joke van Wensem, Toward sustainable environmental quality: Priority research questions for Europe, Environmental Toxicology and Chemistry, Volume 37, Issue 9, 1 September 2018, Pages 2281–2295, https://doi.org/10.1002/etc.4205
- Share Icon Share
Abstract
The United Nations’ Sustainable Development Goals have been established to end poverty, protect the planet, and ensure prosperity for all. Delivery of the Sustainable Development Goals will require a healthy and productive environment. An understanding of the impacts of chemicals which can negatively impact environmental health is therefore essential to the delivery of the Sustainable Development Goals. However, current research on and regulation of chemicals in the environment tend to take a simplistic view and do not account for the complexity of the real world, which inhibits the way we manage chemicals. There is therefore an urgent need for a step change in the way we study and communicate the impacts and control of chemicals in the natural environment. To do this requires the major research questions to be identified so that resources are focused on questions that really matter. We present the findings of a horizon‐scanning exercise to identify research priorities of the European environmental science community around chemicals in the environment. Using the key questions approach, we identified 22 questions of priority. These questions covered overarching questions about which chemicals we should be most concerned about and where, impacts of global megatrends, protection goals, and sustainability of chemicals; the development and parameterization of assessment and management frameworks; and mechanisms to maximize the impact of the research. The research questions identified provide a first‐step in the path forward for the research, regulatory, and business communities to better assess and manage chemicals in the natural environment. Environ Toxicol Chem 2018;37:2281–2295. © 2018 The Authors. Environmental Toxicology and Chemistry published by Wiley Periodicals, Inc. on behalf of SETAC.
INTRODUCTION
On 1 January 2016, the 2030 Agenda for Sustainable Development and its 17 Sustainable Development Goals came into force (United Nations 2015). The aim of the Sustainable Development Goals is to end poverty, protect the planet, and ensure prosperity for all; and their delivery depends on a healthy and productive environment. Europe, like many other parts of the world, is facing a number of major environmental challenges. These include habitat loss and degradation, climate change and associated extreme weather events, environmental contamination resulting from urbanization, agricultural intensification, and increased per capita consumption of natural resources. These environmental challenges, which are a consequence of human activities, are resulting in biodiversity loss; increasing natural hazards; threatening food, water, and energy security; impacting human health; and degrading environmental quality (e.g., Civantos et al. 2012; Leip et al. 2015). The European Environment Agency (2015) has highlighted environmental impacts and health risks from chemicals and climate change as areas of major concern. It also states that, whereas industrial pollutant emissions in Europe have declined following implementation of more stringent European Union policies, they still cause considerable damage to the environment and human health (European Environment Agency 2015).
However, our understanding of how chemicals impact the environment and human health is still poorly developed. For example, most research on and regulation of chemicals considers the impacts of individual substances, yet in the real environment chemicals will co‐occur with hundreds or thousands of other substances and stressors. Laboratory ecotoxicological studies, to support research and regulation, tend to explore impacts on single species rather than populations and communities. Variations in the nature of the environment in time and space, which will affect chemical impacts, are hardly accounted for in research and risk assessments. To achieve the Sustainable Development Goals, a step change is therefore needed in the way in which we study and regulate chemicals in the environment. However, many questions need to be addressed about the risks of chemicals in the environment, and it will be impossible to tackle them all. There is therefore an urgent need to identify the research questions that matter most to the broad community across sectors and multiple disciplines so that research and regulatory efforts can be focused on the most pressing ones.
One approach to identifying key issues in a topic area is to perform horizon‐scanning exercises that promote engagement of researchers and stakeholders from a broad range of sectors (e.g., Fleishman et al. 2011; Rudd et al. 2011; Sutherland et al. 2011; Boxall et al. 2012). In September 2013, the Society of Environmental Toxicology and Chemistry (SETAC) launched a global horizon‐scanning project to identify geographically specific research needs to address stressor impacts on sustainable environmental quality by drawing on the diverse experience and insights of its members. This project employed a key‐questions model in which research questions were widely solicited from SETAC members and subsequently ranked by experts. Key‐questions exercises were performed in all of SETAC's geographic units: Africa, Asia‐Pacific, Europe, Latin America, and North America. Conclusions from the Latin American exercise have recently been published (Furley et al. 2018). We report the results and conclusions of the European key‐questions exercise. We anticipate that the findings will be invaluable in the setting of agendas for regulatory and business communities in Europe and elsewhere.
METHODS
Questions were initially solicited from the membership of the European branch of SETAC in 2014 and 2015. Members (2029 individuals from a range of sectors and disciplines) were invited, via e‐mail, to submit questions to the project. Guidance was provided on what would make an ideal question (Sutherland et al. 2011): it should address important knowledge gaps, be answerable within approximately 5 yr given sufficient research funding (∼ €10 million), be answerable through a realistic research design, have a factual answer that does not depend on value judgments, cover a spatial and temporal scale that could realistically be addressed by a research team, not be answerable by “it all depends” or “yes” or “no,” and should contain a subject, an intervention, and a measurable outcome. The submitted questions were reviewed by the project team to remove duplicate questions and questions outside the scope of the exercise. The final list of questions was then taken forward for discussion at a horizon‐scanning workshop.
The workshop was held in conjunction with the 2015 SETAC Europe Annual Meeting in Barcelona, Spain, and combined plenary and working group discussions. The submitted questions were allocated to 9 themes that were discussed in 3 breakout sessions by 37 participants with multidisciplinary expertise from the government, academia, and industry sectors. Two themes addressed questions related to aquatic and terrestrial ecotoxicology; 2 addressed ecosystem responses to multiple stressors or chemical mixtures; 2 addressed risk assessment, regulation, and public perception; and the final 3 themes addressed nanomaterials: contaminant analysis, fate and behavior, and modeling and predictive toxicology. The workshop participants were tasked with identifying 2 to 5 priority research questions in each theme: breakout group members were free to rephrase or combine candidate questions or to propose new questions to address issues not directly covered by candidate question submissions. The combined list of priority questions was then discussed and agreed at a final plenary session to generate the priority questions.
Finally, an internet‐based survey of the broader SETAC Europe membership was used to rank the priority questions using the best‐to‐worst scaling approach described in Rudd et al. (2014). E‐mails were sent out to all SETAC Europe members asking them to participate in the survey. We asked respondents to repeatedly examine subsets of 4 questions drawn from the full priority list. For each set of 4 questions they were asked to select which of the questions were of greatest and least importance. Ranking questions in this way is cognitively less challenging than full ranking exercises and offers one of the few approaches to effectively and fully rank large lists of items. It also allowed us to rank‐order every question for each respondent and to subsequently calculate the overall rank of all research questions for the entire sample.
RESULTS
A total of 183 questions was submitted by the SETAC Europe membership (see Supplemental Data). The removal of duplicate and invalid questions reduced the number to 90, which were discussed at the workshop. The workshop participants identified 22 of these that they considered as top priority.
The results of the best‐to‐worst ranking analysis, based on 299 responses, are shown in Table 1. The top ranked questions relate to developing an understanding to deal with complexity in the environmental risk assessment process such as understanding the impacts of multiple stressors over time and space. Mid‐ranked questions deal with issues about mitigation, extrapolation between endpoints, chemical prioritization, and predictive ecotoxicology. The lowest ranked questions covered areas such as risk communication, risks from emerging and future stressors, and identification of hotspots of risk around the globe.
The top 22 research questions arising from the European horizon‐scanning workshop and their ranking and scores
Rank | Question | Mean | 95% Lower | 95% Upper |
1 | How can interactions among different stress factors operating at different levels of biological organization be accounted for in environmental risk assessment? | 7.41 | 7.07 | 7.76 |
2 | How do we improve risk assessment of environmental stressors to be more predictive across increasing environmental complexity and spatiotemporal scales? | 7.03 | 6.70 | 7.36 |
3 | How can we define, distinguish, and quantify the effects of multiple stressors on ecosystems? | 6.68 | 6.27 | 7.08 |
4 | How can we develop mechanistic modeling to extrapolate adverse effects across levels of biological organization? | 6.13 | 5.67 | 6.59 |
5 | How can we properly characterize the chemical use, emissions, fate, and exposure at different spatial and temporal scales? | 5.32 | 4.95 | 5.69 |
6 | Which chemicals are the main drivers of mixture toxicity in the environment? | 5.24 | 4.81 | 5.68 |
7 | What are the key ecological challenges arising from global megatrends? | 5.20 | 4.84 | 5.57 |
8 | How can we develop, assess, and select the most effective mitigation measures for chemicals in the environment? | 5.01 | 4.58 | 5.44 |
9 | How do sublethal effects alter individual fitness and propagate to the population and community levels? | 5.00 | 4.53 | 5.48 |
10 | Biodiversity and ecosystem services: What are we trying to protect, where, when, why, and how? | 4.57 | 4.10 | 5.05 |
11 | What approaches should be used to prioritize compounds for environmental risk assessment and management? | 4.34 | 3.95 | 4.72 |
12 | How can monitoring data be used to determine whether current regulatory risk‐assessment schemes are effective for emerging contaminants? | 4.17 | 3.81 | 4.53 |
13 | How can we improve in silico methods for environmental fate and effects estimation? | 4.07 | 3.66 | 4.47 |
14 | How can we integrate evolutionary and ecological knowledge to better determine vulnerability of populations and communities to stressors? | 3.95 | 3.57 | 4.33 |
15 | How do we create high‐throughput strategies for predicting environmentally relevant effects and processes? | 3.82 | 3.42 | 4.21 |
16 | How can we better manage, use, and share data to develop more sustainable and safer products? | 3.79 | 3.39 | 4.20 |
17 | Which interactions are not captured by currently accepted mixture toxicity models? | 3.79 | 3.46 | 4.11 |
18 | How can we assess the environmental risk of emerging and future stressors? | 3.26 | 2.89 | 3.64 |
19 | How can we integrate comparative risk assessment, life cycle analysis, and risk–benefit analysis to identify and design more sustainable alternatives? | 3.10 | 2.66 | 3.53 |
20 | How can we improve the communication of risk to different stakeholders? | 2.98 | 2.57 | 3.39 |
21 | How do we detect and characterize difficult‐to‐measure substances in the environment? | 2.80 | 2.41 | 3.19 |
22 | Where are the hotspots of key contaminants around the globe? | 2.34 | 1.94 | 2.73 |
Rank | Question | Mean | 95% Lower | 95% Upper |
1 | How can interactions among different stress factors operating at different levels of biological organization be accounted for in environmental risk assessment? | 7.41 | 7.07 | 7.76 |
2 | How do we improve risk assessment of environmental stressors to be more predictive across increasing environmental complexity and spatiotemporal scales? | 7.03 | 6.70 | 7.36 |
3 | How can we define, distinguish, and quantify the effects of multiple stressors on ecosystems? | 6.68 | 6.27 | 7.08 |
4 | How can we develop mechanistic modeling to extrapolate adverse effects across levels of biological organization? | 6.13 | 5.67 | 6.59 |
5 | How can we properly characterize the chemical use, emissions, fate, and exposure at different spatial and temporal scales? | 5.32 | 4.95 | 5.69 |
6 | Which chemicals are the main drivers of mixture toxicity in the environment? | 5.24 | 4.81 | 5.68 |
7 | What are the key ecological challenges arising from global megatrends? | 5.20 | 4.84 | 5.57 |
8 | How can we develop, assess, and select the most effective mitigation measures for chemicals in the environment? | 5.01 | 4.58 | 5.44 |
9 | How do sublethal effects alter individual fitness and propagate to the population and community levels? | 5.00 | 4.53 | 5.48 |
10 | Biodiversity and ecosystem services: What are we trying to protect, where, when, why, and how? | 4.57 | 4.10 | 5.05 |
11 | What approaches should be used to prioritize compounds for environmental risk assessment and management? | 4.34 | 3.95 | 4.72 |
12 | How can monitoring data be used to determine whether current regulatory risk‐assessment schemes are effective for emerging contaminants? | 4.17 | 3.81 | 4.53 |
13 | How can we improve in silico methods for environmental fate and effects estimation? | 4.07 | 3.66 | 4.47 |
14 | How can we integrate evolutionary and ecological knowledge to better determine vulnerability of populations and communities to stressors? | 3.95 | 3.57 | 4.33 |
15 | How do we create high‐throughput strategies for predicting environmentally relevant effects and processes? | 3.82 | 3.42 | 4.21 |
16 | How can we better manage, use, and share data to develop more sustainable and safer products? | 3.79 | 3.39 | 4.20 |
17 | Which interactions are not captured by currently accepted mixture toxicity models? | 3.79 | 3.46 | 4.11 |
18 | How can we assess the environmental risk of emerging and future stressors? | 3.26 | 2.89 | 3.64 |
19 | How can we integrate comparative risk assessment, life cycle analysis, and risk–benefit analysis to identify and design more sustainable alternatives? | 3.10 | 2.66 | 3.53 |
20 | How can we improve the communication of risk to different stakeholders? | 2.98 | 2.57 | 3.39 |
21 | How do we detect and characterize difficult‐to‐measure substances in the environment? | 2.80 | 2.41 | 3.19 |
22 | Where are the hotspots of key contaminants around the globe? | 2.34 | 1.94 | 2.73 |
The top 22 research questions arising from the European horizon‐scanning workshop and their ranking and scores
Rank | Question | Mean | 95% Lower | 95% Upper |
1 | How can interactions among different stress factors operating at different levels of biological organization be accounted for in environmental risk assessment? | 7.41 | 7.07 | 7.76 |
2 | How do we improve risk assessment of environmental stressors to be more predictive across increasing environmental complexity and spatiotemporal scales? | 7.03 | 6.70 | 7.36 |
3 | How can we define, distinguish, and quantify the effects of multiple stressors on ecosystems? | 6.68 | 6.27 | 7.08 |
4 | How can we develop mechanistic modeling to extrapolate adverse effects across levels of biological organization? | 6.13 | 5.67 | 6.59 |
5 | How can we properly characterize the chemical use, emissions, fate, and exposure at different spatial and temporal scales? | 5.32 | 4.95 | 5.69 |
6 | Which chemicals are the main drivers of mixture toxicity in the environment? | 5.24 | 4.81 | 5.68 |
7 | What are the key ecological challenges arising from global megatrends? | 5.20 | 4.84 | 5.57 |
8 | How can we develop, assess, and select the most effective mitigation measures for chemicals in the environment? | 5.01 | 4.58 | 5.44 |
9 | How do sublethal effects alter individual fitness and propagate to the population and community levels? | 5.00 | 4.53 | 5.48 |
10 | Biodiversity and ecosystem services: What are we trying to protect, where, when, why, and how? | 4.57 | 4.10 | 5.05 |
11 | What approaches should be used to prioritize compounds for environmental risk assessment and management? | 4.34 | 3.95 | 4.72 |
12 | How can monitoring data be used to determine whether current regulatory risk‐assessment schemes are effective for emerging contaminants? | 4.17 | 3.81 | 4.53 |
13 | How can we improve in silico methods for environmental fate and effects estimation? | 4.07 | 3.66 | 4.47 |
14 | How can we integrate evolutionary and ecological knowledge to better determine vulnerability of populations and communities to stressors? | 3.95 | 3.57 | 4.33 |
15 | How do we create high‐throughput strategies for predicting environmentally relevant effects and processes? | 3.82 | 3.42 | 4.21 |
16 | How can we better manage, use, and share data to develop more sustainable and safer products? | 3.79 | 3.39 | 4.20 |
17 | Which interactions are not captured by currently accepted mixture toxicity models? | 3.79 | 3.46 | 4.11 |
18 | How can we assess the environmental risk of emerging and future stressors? | 3.26 | 2.89 | 3.64 |
19 | How can we integrate comparative risk assessment, life cycle analysis, and risk–benefit analysis to identify and design more sustainable alternatives? | 3.10 | 2.66 | 3.53 |
20 | How can we improve the communication of risk to different stakeholders? | 2.98 | 2.57 | 3.39 |
21 | How do we detect and characterize difficult‐to‐measure substances in the environment? | 2.80 | 2.41 | 3.19 |
22 | Where are the hotspots of key contaminants around the globe? | 2.34 | 1.94 | 2.73 |
Rank | Question | Mean | 95% Lower | 95% Upper |
1 | How can interactions among different stress factors operating at different levels of biological organization be accounted for in environmental risk assessment? | 7.41 | 7.07 | 7.76 |
2 | How do we improve risk assessment of environmental stressors to be more predictive across increasing environmental complexity and spatiotemporal scales? | 7.03 | 6.70 | 7.36 |
3 | How can we define, distinguish, and quantify the effects of multiple stressors on ecosystems? | 6.68 | 6.27 | 7.08 |
4 | How can we develop mechanistic modeling to extrapolate adverse effects across levels of biological organization? | 6.13 | 5.67 | 6.59 |
5 | How can we properly characterize the chemical use, emissions, fate, and exposure at different spatial and temporal scales? | 5.32 | 4.95 | 5.69 |
6 | Which chemicals are the main drivers of mixture toxicity in the environment? | 5.24 | 4.81 | 5.68 |
7 | What are the key ecological challenges arising from global megatrends? | 5.20 | 4.84 | 5.57 |
8 | How can we develop, assess, and select the most effective mitigation measures for chemicals in the environment? | 5.01 | 4.58 | 5.44 |
9 | How do sublethal effects alter individual fitness and propagate to the population and community levels? | 5.00 | 4.53 | 5.48 |
10 | Biodiversity and ecosystem services: What are we trying to protect, where, when, why, and how? | 4.57 | 4.10 | 5.05 |
11 | What approaches should be used to prioritize compounds for environmental risk assessment and management? | 4.34 | 3.95 | 4.72 |
12 | How can monitoring data be used to determine whether current regulatory risk‐assessment schemes are effective for emerging contaminants? | 4.17 | 3.81 | 4.53 |
13 | How can we improve in silico methods for environmental fate and effects estimation? | 4.07 | 3.66 | 4.47 |
14 | How can we integrate evolutionary and ecological knowledge to better determine vulnerability of populations and communities to stressors? | 3.95 | 3.57 | 4.33 |
15 | How do we create high‐throughput strategies for predicting environmentally relevant effects and processes? | 3.82 | 3.42 | 4.21 |
16 | How can we better manage, use, and share data to develop more sustainable and safer products? | 3.79 | 3.39 | 4.20 |
17 | Which interactions are not captured by currently accepted mixture toxicity models? | 3.79 | 3.46 | 4.11 |
18 | How can we assess the environmental risk of emerging and future stressors? | 3.26 | 2.89 | 3.64 |
19 | How can we integrate comparative risk assessment, life cycle analysis, and risk–benefit analysis to identify and design more sustainable alternatives? | 3.10 | 2.66 | 3.53 |
20 | How can we improve the communication of risk to different stakeholders? | 2.98 | 2.57 | 3.39 |
21 | How do we detect and characterize difficult‐to‐measure substances in the environment? | 2.80 | 2.41 | 3.19 |
22 | Where are the hotspots of key contaminants around the globe? | 2.34 | 1.94 | 2.73 |
We provide a brief description of each question and the drivers behind the question. We do not provide a detailed review of an area but attempt to highlight the potential approaches for answering a question, the likely challenges, and the interdependency of each question with other questions coming out of the exercise. An analysis of the questions indicated that the priority questions were grouped into 3 broad categories (Figure 1), so we have ordered the questions by category.
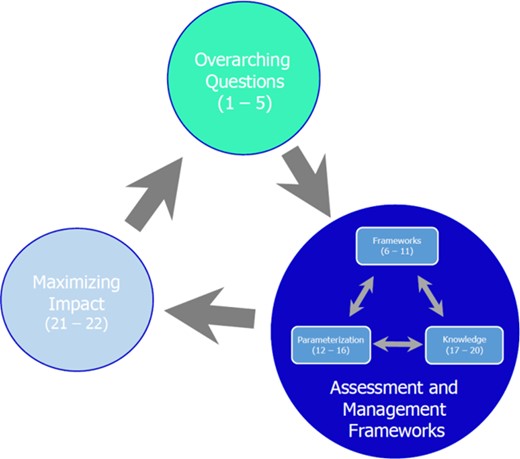
Broad categorization of the 22 priority questions, showing the interlinkages between the questions.
Overarching questions
Five “overarching questions” covered aspects of which chemicals are negatively impacting the environment and the identification of regions most heavily impacted, the impacts of global megatrends on chemical impacts, the identification of the most sustainable pathways for chemical use, and the definition of protection goals.
1. What are the key ecological challenges arising from global megatrends? (Rank #7)
The accelerating changes in urbanization, climate, and demographics were highlighted in a recent assessment of the impact of global megatrends on European environments (European Environment Agency 2015). Urbanization generates multiple environmental stressors, the sources and effects of which are complex and difficult to untangle (questions 3, 8, and 10; Johnson and Sumpter 2014). Understanding climate‐induced changes in the abundance and distribution of species (including pests and disease organisms), coupled with an understanding of how climate change affects the exposure characteristics and impacts of multiple stressors, is essential for effective risk assessment and risk management (Stahl et al. 2013). Renewable energy sources (solar, wind, tidal, biofuels) are key to mitigating the effects of climate change but are not without environmental consequences (Spellman 2014), which also need to be assessed and managed. Europe's population is aging rapidly, and resulting shifts in housing, transport, technology, and infrastructure, as well as changes in pharmaceutical and energy use (Government Office for Science 2016), may have significant environmental impacts. These large‐scale challenges can only be addressed via interdisciplinary approaches that account for the complexity and connectivity of environmental systems and incorporate appropriate spatial and temporal scales (questions 11 and 16). In addition to developing a systems‐based approach to environmental risk assessment that incorporates multiple stressors, it is necessary to consider environmental risk in a global context, to ensure that national policies do not have unintended adverse global consequences (questions 4 and 12; Lenzen et al. 2012).
2. Biodiversity and ecosystem services: What are we trying to protect, where, when, why, and how? (Rank #10)
Central to effective land management and environmental protection is a clear articulation of what is being protected in a specific location/habitat type (where), over what time scales the protection applies (when), and what the justification for the protection is (why). Only once the protection goal has been articulated can the correct management (how) be instigated. Biodiversity is essential to human well‐being and provides many benefits (ecosystem services; Mace et al. 2012). However, it is not possible to protect everything, everywhere, all of the time (Holt et al. 2016). Because ecosystems are managed to meet human demands (e.g., water provision, food production, raw materials), trade‐offs between protecting ecosystem integrity and guaranteeing human welfare need to be considered. The societal and policy challenge is deciding which ecosystem services are desired in specific habitats over specified time periods (question 22). The scientific challenge is understanding which species and processes (i.e., service providing units) deliver the desired ecosystem services and how stress‐induced changes in these ecological components translate into changes in ecosystem service delivery (questions 6 and 7; Maltby 2013). Robust ecological production functions that translate changes in service providing unit attributes to changes in ecosystem service delivery and outcomes that people value are essential to an ecosystem services–based approach to environmental risk assessment (questions 10 and 20; Bruins et al. 2017). The adoption of an ecosystem services–based approach to environmental risk assessment would provide a framework for landscape‐scale risk management, enabling the development of spatially explicit protection goals and more targeted risk‐management measures (question 5). Systematic conservation‐planning approaches (Margules and Pressey 2000) may play a role here by allowing ecological knowledge to be incorporated into practice and ecosystem functions and services to be considered into the design of protected areas (Adame et al. 2015).
3. Which chemicals are the main drivers of mixture toxicity in the environment? (Rank #6)
Ecosystems, including humans, are exposed to mixtures of chemicals and not single compounds (e.g., Moschet et al. 2014). However, the ecotoxicity and toxicity of these mixtures of chemicals in the environment are often driven primarily by a few compounds (e.g., Vallotton and Price 2016). Consequently, the development of methodologies for the identification of such “mixture toxicity drivers” is a European research priority (European Commission 2012). The use of effects‐directed analysis methods (Brack 2003), where a combination of toxicity testing and sample manipulation is used to home in on the chemical drivers of toxicity, which are then identified through chemical analysis methods, could help identify mixture toxicity drivers. The use of cutting‐edge chemical analysis techniques such as time‐of‐flight mass spectrometry for nontargeted analysis of a sample coupled with in silico models for estimating the toxicity (question 18) of the identified chemicals (Hollender et al. 2017) and the use of chemical prioritization approaches (question 13) may also be part of the solution. Chemical composition of environmental mixtures will vary in time and space, and different compounds will affect different organisms in different ways. To fully address the question of drivers of mixture toxicity will therefore likely require intense sampling campaigns at high temporal and spatial resolutions and the development of high‐throughput approaches (question 19) for characterizing the toxicity of mixtures to key taxonomic groups and for identifying key toxicants.
4. Where are the hotspots of key contaminants around the globe? (Rank #22)
Much of our understanding of the concentrations of contaminants relates to the North American, European, and Chinese situations, with limited or no data available for many other countries around the globe (e.g., Aus Der Beek 2016). More global‐scale initiatives are needed to identify pollution hotspots so that mitigation efforts can be focused on these areas (Kroeze et al. 2016). This could be achieved through global‐scale environmental monitoring studies of key classes of contaminants. For select contaminants this may need new analytical methodologies (question 17). These studies would require global collaborations, possibly coordinated by organizations such as SETAC. The use of citizen science–based approaches, similar to the FreshWater Watch program on water quality across the globe (Scott and Frost 2017) or on microplastic contamination of European beaches (Lots et al. 2017), could be part of the solution. Even using these mass sampling methods, it will be impractical to monitor everywhere, so any monitoring activities will likely need to be complemented by modeling activities to provide high‐resolution information on levels of contamination in different regions. The use, use patterns, fate, behavior, and exposure pathways of chemicals are likely to differ across regions within a country and across countries (question 16). Consequently, the identification of contaminant hotspots using modeling approaches will require a concerted effort to collate information on chemical emissions and local practices (e.g., for disposal of waste and wastewater), as well as the characteristics of the receiving natural environment (altitude, weather conditions, soil maps, distribution of water bodies, and hydrological regimes; Keller et al. 2014).
5. How can we develop, assess, and select the most effective mitigation measures for chemicals in the environment? (Rank #8)
Mitigation measures are becoming increasingly important to protect the environment from future pollution and to abate current pollution. A range of approaches are available to limit the risks of chemicals in the environment, including policy interventions (e.g., banning of a substance), environmental stewardship, existing and novel treatment technologies, and the application of green chemistry (Schwarzenbach et al. 2006). The development of effective mitigation methods will require the identification of contaminant classes that cause environmental effects (question 3) and the locations across the globe at greatest risk (question 4). It is likely that combinations of approaches will be needed and that these combinations will need to be tailored to a particular pollution problem and the location of interest. Selection of a method will need to consider not only the efficacy of a method for reducing environmental exposure but also affordability for the area of interest, social acceptability, ease of use, and the broader environmental costs of an approach such as increased CO2 emissions. Selection of an approach will likely require the use of cost–benefit analyses to weigh up the environmental benefits of reducing the levels of contamination against the economic, social, and other environmental costs of adopting the method. The ecosystem services concept could be used to frame and assess trade‐offs inherent in such evaluations (Nienstedt et al. 2012; question 2). How well an approach works could be assessed through the use of environmental monitoring and the use of social science methodologies such as public surveys, pre‐ and postadoption of a mitigation approach. These studies may need to run for some time to determine the long‐term sustainability of a particular solution.
Assessment and management frameworks
Seventeen questions were related to the design, parameterization, and validation of “assessment and management frameworks.” These questions fit within 3 subdivisions: generation of fundamental knowledge, development of frameworks, and parameterization of frameworks.
Fundamental knowledge
6. How can we integrate evolutionary and ecological knowledge to better determine vulnerability of populations and communities to stressors? (Rank #14)
The vulnerability of populations and communities to stressors is a function of exposure, inherent sensitivity, and recovery (De Lange et al. 2010). Exposure is dependent on the spatiotemporal co‐occurrence of stressor and species, which in turn is a function of habitat suitability and the ecological processes driving community assembly and species coexistence (i.e., dispersal, colonization, competition, predation; question 11; HilleRisLambers et al. 2012). Differences in the inherent sensitivity of species derive from phylogenetic differences in morphological, physiological, and ecological traits (Rubach et al. 2012), which are shaped by evolutionary processes (Dallinger and Höckner 2013). The internal recovery of populations is dependent on the reproductive output of surviving individuals, whereas external recovery is dependent on immigration processes and the presence of local source populations (Gergs et al. 2016a). The recovery of communities is dependent on recolonization order (e.g., prey available for predators), the degree of niche specialization of the recolonizing species, and the ecological and evolutionary processes that generate the local species pool (question 10; Mittelbach and Schemske 2015). Traits commonly associated with vulnerable species include restricted distribution and limited dispersal ability, long generation times and low reproductive rates, specialized habitats and dietary requirements, and narrow physiological tolerances (Pacifici et al. 2015). However, the relative importance of specific traits in determining vulnerability and how evolutionary and ecological processes shape them requires further investigation (question 11).
7. How do sublethal effects alter individual fitness and propagate to the population and community levels? (Rank #9)
Primarily, environmental risk assessment is concerned with protecting populations of species and the communities and ecosystems to which they belong. However, most information is available on the lethal and sublethal effects of chemicals on individual organisms, and therefore, the scientific challenge is understanding and predicting the population‐ and community‐level implications of (sub)individual‐level effects. The use of molecular and cellular responses to chemical exposure in environmental risk assessment (i.e., biomarkers) has been criticized as being unlikely to be predictive of adverse effects at the level of the whole organism, let alone at the population or community level (e.g., Forbes et al. 2006). The adverse outcome pathway (AOP) addresses this criticism by identifying the chain of causality between chemically induced molecular initiating events and adverse outcomes at levels of biological organization relevant to environmental risk assessment (Ankley et al. 2010). Quantitative AOPs have a potentially important role to play in screening and monitoring programs (questions 15 and 19), but considerable resources are needed to generate the mechanistic understanding required (Conolly et al. 2017).
Individual‐level effects, either predicted from AOPs or measured experimentally, can be extrapolated to population‐level effects and beyond, using mechanistic effect models (Forbes and Galic 2016; question 20). Whether chemical‐induced reductions in vital rates (e.g., survival, growth, and reproduction) result in population declines depends on the physiological processes affected by the chemical (Martin et al. 2013) and density‐mediated compensatory mechanisms operating in natural populations (Rohr et al. 2016). At the community level, adverse effects on species may be counteracted by changes in biotic interactions (i.e., reduced competition or predation), and adverse effects on ecological processes may occur despite little effect on the abundance of individual populations (Galic et al. 2017) or species richness (Spaak et al. 2017). Greater mechanistic and ecological understanding is needed to reduce the uncertainties associated with extrapolating from what we measure ([sub]individual‐level responses) to what we want to protect (populations, communities, and the ecosystem services they provide).
8. How can we define, distinguish, and quantify the effects of multiple stressors on ecosystems? (Rank #3)
Ecosystems face an increasing complexity of anthropogenic and natural stressors (see question 1), and understanding, quantifying, and predicting their interactive effects remain challenging (Segner et al. 2014; Jackson et al. 2016). Distinguishing the effects of multiple stressors on ecosystems requires multiple lines of evidence that can be generated from a range of approaches, including in situ toxicity identification and evaluation (Steigmeyer et al. 2017), molecularly based diagnostic tools (Dafforn et al. 2016), eco‐epidemiology (Posthuma et al. 2016), and Bayesian network relative risk models (Landis et al. 2017). Our limited understanding of the combined effects of multiple stressors on ecosystems is hampering the development of sound risk‐assessment and management strategies (Van den Brink et al. 2016; question 10). One reason for our poor understanding is the limited availability of detailed ecological information over sufficient spatial and temporal scales (question 11) to distinguish chemical effects from natural variability and to identify robust associations between exposure and effect (question 10). The use of emerging technologies such as remote sensing and high‐throughput genomic sequencing techniques (question 19) will enable a more rapid and economical collection of ecological data sets on a similar or greater scale, when compared to physical and chemical monitoring (Chariton et al. 2016). However, as these methods evolve, care must be taken to ensure that the granularity and scale, as well as relevance and narrative intent, of different measures are properly taken into account. Field surveys and weight‐of‐evidence approaches alone cannot definitively establish causality (Stevenson and Chapman 2017); what is required is a combination of comprehensive field surveys (covering a wide range of stressor interactions) and experimental studies.
9. Which interactions are not captured by currently accepted mixture toxicity models? (Rank #17)
The standard mixture toxicity models, concentration addition and independent action (also known as “response addition”), are based on the assumption that the components in a mixture do not interact (Backhaus and Faust 2012). However, in the real world, chemicals can interact in a mixture, at the chemical, organismal, and/or ecological level. Such interactions are sometimes pronounced enough to lead to deviations from predictions based on the concentration addition or independent action model, patterns that are often termed “synergism” or “antagonism” (respectively, higher or lower toxicity than the sum of single toxic effects). Given that concentration addition as well as independent action are exceptionally coarse simplifications of complex biological and ecological systems, deviations from concentration addition‐ or independent action‐based mixture toxicity predictions are to be expected. The crucial question is therefore whether the observed deviations are unacceptably high, which depends on the specific protection goal, the endpoint studied, and how often such deviations occur. A systematic exploration of interactions to identify which combinations of chemicals deviate from the independent action or concentration addition model is a major challenge because an enormous number of different biological receptors and biochemical pathways from myriad organisms with different life cycles and traits, interacting with each other in complex ecological communities, are involved. Meeting this challenge will likely need to involve the use of high‐throughput screening approaches discussed in question 19. The assessment of the mechanisms and consequences of interactions between chemicals on an ecological level closely resembles the analysis of multiple stressor effects discussed in question 10.
Development of assessment and management frameworks
10. How can interactions among different stress factors operating at different levels of biological organization be accounted for in environmental risk assessment? (Rank #1)
One of the most difficult and evasive goals of environmental risk assessment is the understanding of the effects of multiple stressors on individuals, populations, and ultimately groups of interacting species at different spatial scales (e.g., Kapo et al. 2014). Prospective environmental risk assessments primarily focus on a single stressor or a limited number of stressors in a few model species, under (semi)controlled conditions over limited timescales (Hommen et al. 2010). Retrospective environmental risk assessments are inevitably concerned with multiple stressor impacts on dynamic and complex ecosystems, which may have been exposed over many years and for which assignment of causality is difficult (question 8; Fischer et al. 2013). Ecosystems are subject to a multitude of chemical (e.g., pH), physical (e.g., temperature, sedimentation), and biological (e.g., parasitism, invasive species) stressors that may enhance or reduce the impact of anthropogenic chemical exposures. Stressor interactions can influence chemical bioavailability and uptake (Karlsson et al. 2017) as well as detoxification and other defence mechanisms (Janssens and Stoks 2017), which may result in antagonistic or synergistic effects on individual organisms. Stressor‐induced changes in phenology, species tolerance, community composition, and biotic interactions can result in ecosystems being more or less resilient to anthropogenic chemicals (question 6; Rohr et al. 2016).
Accounting for multistressor effects in environmental risk assessment requires the development of mechanistic exposure and effects models that capture stressor interactions at relevant spatiotemporal scales and enable extrapolation across levels of biological organization (question 20). This will require greater understanding of stressor interactions in natural systems, information from manipulative experiments at appropriate temporal and spatial scales, and field surveys spanning wide gradients of focal stressors at multiple locations (Beketov and Liess 2012). Model development and implementation will be facilitated by the development of environmental scenarios for combined exposure and effect assessment (question 11).
11. How do we improve risk assessment of environmental stressors to be more predictive across increasing environmental complexity and spatiotemporal scales? (Rank #2)
Stressors may be distributed across multiple habitats and transported considerable distances from the point of release. Spatiotemporal variation in stressor exposure is superimposed on variation in the distribution of biological species, ecological processes, and the ecosystem services they provide. Risk is therefore variable and context‐dependent; it varies according to the location, type, and quality of habitats and the exposure to stressors within the landscape (Landis et al. 2017). Current environmental risk assessment frameworks do not account explicitly for the environmental complexity that drives spatiotemporal variation in risk at different scales (Scientific Committee on Health and Environmental Risks et al. 2013a; question 10), but how important is this for environmental decision‐making? Current approaches adopt “realistic worst‐case” assumptions and are designed to be conservative rather than realistic. How appropriate are these assumptions, and what is the degree of over‐ or underprotection? A more spatially defined environmental risk assessment would allow for targeting of interventions (e.g., restrictions, mitigation measures) where protection is most needed, while limiting opportunity costs of overprotection elsewhere.
How much of this complexity needs to be incorporated into assessments of risk? Overly simple models do not represent important aspects of the system's dynamics and have large model bias. Overly complex models require detailed knowledge of species and environmental interactions and need a large number of parameters to specify detailed dynamics; they have large parameter uncertainty (Collie et al. 2016). An alternative approach to building complex models is to develop scenarios that are defined in terms of landscape structure and environmental conditions, incorporate spatial and temporal variability, and link to protection goals (Rico et al. 2016; question 2). Landscape ecotoxicology provides a conceptual framework for bringing together mechanistic exposure and effect modeling, and the increasing availability of spatially‐ and temporally explicit data sets provides an exciting opportunity to develop mapping and modeling tools that both are spatially defined and make predictions in real time (Focks 2014).
12. How can we assess the environmental risk of emerging and future stressors? (Rank #18)
Over the past decade, there has been increasing interest in the environmental risks of the so‐called emerging contaminants. Emerging contaminants encompass a broad range of substances including those that have been used for some time (e.g., pharmaceuticals and personal care products, veterinary medicines, and plastics) and their transformation products and new technologies such as nanomaterials and biologicals (Boxall, 2012). The main concern is that existing paradigms and models used for environmental risk assessment may not be appropriate because the drivers of their environmental fate, behavior, and effects differ from those of traditional chemicals (question 15). For example, for nanomaterials and microplastics, the partitioning concept used in risk assessment for assessing the distribution of “traditional” chemicals between environmental compartments is inappropriate for use on particulate material (Praetorius et al. 2014). Exposure models are therefore needed that take into account processes relevant for particles (e.g., Praetorius et al. 2012). Approaches for combining exposure predictions with data from effects studies for particles are also poorly developed. For pharmaceuticals and veterinary medicines, many compounds are ionized at environmental pH values, so models for estimating sorption, uptake, and toxicity that are embedded into risk‐assessment schemes are inappropriate. New approaches are also needed for assessing the risks of micro‐ and nano‐encapsulated bioactive materials such as nanopesticides (Kookana et al. 2014). A wealth of data and knowledge have been generated over the past few years on the fate and effects of many classes of emerging contaminants, and numerous models and tools are being proposed for assessing the properties, exposure, and effects of these substances. These approaches now need to be evaluated and, where appropriate, then embedded into environmental risk assessment processes. In instances where models are not available for key substance classes and endpoints, these need to be developed. Much of the existing data are held by industry, so the development of new models could be facilitated through improvements in approaches to share data (question 21).
13. What approaches should be used to prioritize compounds for environmental risk assessment and management? (Rank #11)
It is estimated that approximately 120 000 chemicals are manufactured and imported in Europe (European Chemicals Agency 2018a). During use and following emission to the natural environment, these chemicals can be metabolized or degraded to transformation products (Boxall et al. 2004), so the environment will be exposed to an even greater number of chemicals. However, we only have data on the environmental occurrence, fate, effects, and risks of a small proportion of these substances; and even fewer are regulated. Methods have been proposed to prioritize chemicals for testing and risk assessment (i.e., substances with limited data); the methods are typically reliant on predictive models and algorithms or read‐across approaches (Burns et al. 2018; question 18). The objective of prioritizing chemicals requires inputs from most of the priority questions identified in the present report. A better understanding of the distribution, exposure, effects, and relevance of multiple chemicals to a range of endpoints, in the context of a changing environment, multiple stressors, and evolving expectations of landscapes and services, must be integrated to develop regionally relevant priority lists (question 16). The current approaches have shortcomings when it comes to focusing on “what matters.” They, however, constitute a good starting point that can be complemented with experience and existing exchanges on prioritization approaches between different regulatory systems. Further efforts could, for example, be directed toward better understanding and application of commonalities between approaches. The effects‐directed analysis approaches, discussed in question 3, could also be used to identify those contaminants in an area of concern that require management.
14. How can we integrate comparative risk assessment, life cycle analysis, and risk–benefit analysis to identify and design more sustainable alternatives? (Rank #19)
Synthetic chemicals are essential to modern life, but they may have unacceptable environmental or human health impacts. There is therefore a strong desire to substitute the most hazardous chemicals with nonhazardous alternatives that have the same function (European Chemicals Agency 2018b). Chemical risk assessment and management in Europe is fragmented and single chemical–focused. Different research communities drive forward advances in risk assessment, life cycle analysis, and risk–benefit analysis, with little interaction or awareness of each other's activities. However, the integration of comparative risk assessment, life cycle analysis, and risk–benefit analysis is essential for effective decision‐making. A holistic approach is needed to consider all stages of a chemical's life cycle and to minimize the risk of unintended consequences, including the loss of socioeconomic benefits of chemical use and regional displacement of environmental impacts attributable to shifts in global production. A more integrated approach will facilitate the identification and design of less hazardous chemicals or chemical alternatives, while maintaining intended functions and represents an opportunity to fuel innovation and economic growth while protecting public health and the environment (Zimmerman and Anastas 2015; DeVito 2016). In particular, incorporating toxicology into the molecular design process, possibly using the tools developed in response to question 18, provides the potential to produce safer chemicals, but further multidisciplinary research is needed to ensure that this potential is realized (Coish et al. 2016).
15. How can monitoring data be used to determine whether current regulatory risk‐assessment schemes are effective for emerging contaminants? (Rank #12)
As discussed under question 12, there is concern that existing experimental and modeling methods, used to support environmental risk assessment, may not be appropriate for many classes of emerging contaminants, especially particulate contaminants such as nanomaterials and microplastics. Chemical and biological monitoring of exposed environments could help identify whether current risk‐assessment schemes are effective and, if not, where the frameworks fall down. This could be achieved through monitoring studies of an emerging contaminant of interest at the different stages in the source–pathway–receptor relationship. The results could then be used to evaluate exposure models and laboratory fate and effects studies used in the risk‐assessment process. Because many emerging contaminants are difficult to measure, to answer this question will require robust and sensitive analytical methods to be developed for many of these compounds (question 17). Although this question focuses on emerging contaminants, it is also relevant to environmental contaminants more generally.
Parameterization
16. How can we properly characterize the chemical use, emissions, fate, and exposure at different spatial and temporal scales? (Rank #5)
Environmental assessment of chemicals is typically done without a specific spatial and temporal scale in mind. Obtaining data on the emissions, fate, and exposure of chemicals at high spatial and temporal resolutions would provide better information on which organisms are really exposed throughout their lifetime and what they are exposed to and help to answer many of the other priority questions (e.g., questions 4, 11, 13, 15). A wide range of technologies (including mobile phones, passive sampling devices, miniaturized sensing devices, high‐resolution spatial models, remote sensing, robotics, and state‐of‐the‐art analytical techniques such as time‐of‐flight mass spectrometry) are now available (e.g., INTCATCH 2018) that could provide new insights into chemical exposure. These technologies could allow assessors to 1) quantify levels of pollution at greater frequencies and spatial resolutions than is currently possible, 2) monitor locations that in the past have been difficult to sample (e.g., hostile environments or systems with accessibility issues), and 3) characterize human and ecological exposure to the plethora of chemicals that have never been monitored before. Effective application of various technologies will provide a much better understanding of the degree of exposure of humans and wildlife to pollutants and hence the risks these pollutants pose to the health of ecosystems and humans. These technologies have the potential to be used to inform mitigation measures, both in the short term and over longer timescales. The use of new technologies will, however, also raise challenges, like quality control, regulatory acceptance, social and ethical issues, and the analysis and interpretation of the resulting “big data” (Dafforn et al. 2016).
17. How do we detect and characterize difficult‐to‐measure substances in the environment? (Rank #21)
Robust and sensitive analytical methods have been available for metals, pesticides, and many persistent organic compounds for some time. However, for many contaminant classes, analysis is still challenging. Good examples are the products of unknown or variable composition, complex reaction products, and biological materials, nanomaterials, plastics, and other polymers. For example, unknown or variable composition, complex reaction products, and biological material substances are comprised of individual constituents, each of which may possess different physicochemical and fate properties. These substances cannot be sufficiently identified by their chemical composition, which creates complications for testing using standard guideline methodologies (European Chemicals Agency 2017). The potential toxicity, behavior, and fate of nanomaterials and microplastics are affected by a wide range of factors including particle number and mass concentration, surface area, charge, chemistry and reactivity, size and size distribution, state of hetero‐/homo‐agglomeration/aggregation, elemental composition, as well as structure and shape (Borm et al. 2006; Handy et al. 2008; Coutris et al. 2012; Benoit et al. 2013). Therefore, when analyzing nano‐ and microparticles in different matrices, it is not only the composition and concentration that will need to be determined but also the physical and chemical properties of the particles within the sample and the chemical characteristics of any capping/functional layer on the particle surface. A range of new analytical techniques, including microscopy‐based approaches, chromatography, centrifugation, filtration, fractionation, spectroscopic and related techniques, and single‐particle inductively coupled plasma mass spectrometry, have been reported in the literature that could be used (Hässellöv et al. 2008; Hildago‐Ruz et al. 2012). However, although many of these approaches work when used in controlled laboratory‐based studies, they can lack the sensitivity and specificity for application to environmental monitoring. Work therefore needs to continue on the development of methods that are able to measure these substances at concentrations that are expected to occur in the environment.
18. How can we improve in silico methods for environmental fate and effects estimation? (Rank #13)
In silico approaches, such as (quantitative) structure–activity relationships, (quantitative) structure–property relationships, read‐across, and expert systems have been available for some time for estimating the properties, persistence, and environmental effects of a chemical based on its chemical structure (European Commission 1998). Although these predictive approaches work well for select classes of chemicals (e.g., neutral organics) and endpoints (e.g., log KOW and acute toxicity), we are not yet at a stage where we have robust models for all classes of chemicals and all the environmental endpoints that we consider in the risk‐assessment process. In particular, we need improved models for chronic toxicity, biodegradation in environmental matrices, sorption and uptake of ionizable compounds, effects models for specifically acting compounds, and property and effect models for nanomaterials and microplastics (e.g., Winkler et al. 2013; Cronin 2017). The development of new models might be achieved through the adoption of new data‐mining technologies such as machine learning techniques (Devinyak and Lesyk 2016) and, for molecules like pharmaceuticals, mammalian to environmental read‐across approaches (Rand‐Weaver et al. 2013). To develop these new approaches in a timely manner will require generation of data for training and evaluation of models, perhaps using some of the high‐throughput methodologies discussed in question 19 as well as increased sharing of existing data (and metadata) that have been generated by the research community and industry over the years (question 21).
19. How do we create high‐throughput strategies for understanding environmentally relevant effects and processes? (Rank #15)
To experimentally establish the environmental properties and effects of a chemical will typically involve the use of Organisation for Economic Co‐operation and Development (OECD)–type test methodologies. These methods can be time‐consuming and costly and, in the case of ecotoxicity testing, involve the use of whole animals. The use of alternative high‐throughput strategies could allow us to generate information on the fate, behavior, and effects of large numbers of chemicals in a significantly shorter time than the traditional approaches. The availability of such approaches would enable us to generate the data to support work to answer other questions, such as questions 3, 9, and 18. Potential solutions include the adaptation of existing standard methods to shorten the study and/or reduce the number of animals used. A good example is the so‐called minimized bioconcentration study, which uses up to 70% fewer animals than the standard OECD approach and could be run over shorter time periods (Springer et al. 2008; Carter et al. 2014). Technologically led solutions include the use of in vitro and micro‐scale assays. High‐throughput testing routinely employs in vitro models used for pharmaceutical development and alternative animal systems (e.g., embryonic zebrafish) to rapidly collect information on bioactivity and toxic potential for diverse industrial and speciality chemicals. High‐throughput testing uses modern robotics, computing, and miniaturization and relies largely on batteries of in vitro bioassays that may effectively screen chemicals for their ability to exert specific biological activities or perturbations. High‐throughput testing has the attraction of being able to perform hundreds or thousands of biological determinations in relatively short times and with a potentially high degree of experimental standardization (Schroeder et al. 2016). We are still far from being able to predictively extrapolate high‐throughput testing results to ecologically important endpoints. However, AOPs may translate biological activities mapped at the molecular level to traditional and regulatory meaningful apical endpoints (such as growth or reproduction impairments). Efforts such as those recently described by Ankley et al. (2016) are needed to address the biological domain of applicability of high‐throughput testing data in the context of application to environmental risk assessment. Both the US National Research Council (2007) and the European Commission (Worth et al. 2014) advocate for moving away from the traditional reliance on whole‐animal toxicity testing and toward in vitro and micro‐scale bioassays (Krewski et al. 2010).
20. How can we develop mechanistic modeling to extrapolate adverse effects across levels of biological organization? (Rank #4)
Most regulatory toxicity studies measure the effect of chemicals on individual organisms and do not consider impacts on higher levels of biological organization and ecosystem services, which is what we want to protect (question 2). There is therefore a need to extrapolate effects across levels of biological organization, and mechanistic modeling is one way to do this (question 7). Mechanistic effect models include toxicokinetic–toxicodynamic models and AOPs that extrapolate chemical concentrations or molecular initiating events to individual‐level effects (Ankley et al. 2010; Ashauer et al. 2011; Ashauer and Jager 2018), dynamic energy budget models that extrapolate changes in physiological responses to vital rates (Kooijman 2010), individual‐based and population models that extrapolate individual‐level effects to population‐level consequences (Forbes et al. 2011; Martin et al. 2013), food web models that extrapolate effects on populations to community‐level consequences (Pastorok et al. 2002), and ecological production functions that extrapolate from changes in biophysical structure or process to ecosystem functions driving ecosystem services (Bruins et al. 2017). Recent advances include the development of good modeling practice (Grimm et al. 2014); the integration of the toxicokinetic–toxicodynamic, dynamic energy budget, and individual‐based model approaches (e.g., Gergs et al. 2016b); and the use of scenarios and trait‐based approaches to improve the general applicability of models (Van den Brink et al. 2013; Rico et al. 2016). In addition to approaches for extrapolating across levels of biological organization, there are emerging computational approaches for extrapolating across species based on the conservation of key biological traits and molecular processes (e.g., LaLone et al. 2016; Ankley et al. 2016; question 6). However, the use of these approaches in environmental risk assessment is limited, and considerable research is still required to make the models suitable for regulatory risk assessment (Forbes and Galic 2016; Hommen et al. 2016). In particular, there is a need for more in‐depth knowledge of mechanistic linkages between different levels of biological organization (question 7) and increased availability of trait data for species that are relevant to key protection goals (question 2).
Maximizing impact
Two questions were about “maximizing the impact” of the work of the community through better communication of risks and the more effective collation and sharing of data.
21. How can we better manage, use, and share data to develop more sustainable and safer products? (Rank #16)
A wealth of data on the environmental fate, behavior, and effects of chemicals have been produced over the years by the research community and the business sector. Exploitation of all this information could help us to much better assess the environmental risk of the chemicals in use today and to help identify safer alternatives. Significant resource investment has resulted in diverse toxicity data sets, available in both the public and private domains, for many environmental contaminants, such as the European Chemicals Agency's unique database on chemicals in Europe (2018a), the European Union Observatory for Nanomaterials (2018), and the US Environmental Protection Agency's ECOTOX database (US Environmental Protection Agency 2018). These can be used to develop quantitative structure–activity relationships (Cherkasov et al. 2014), to develop group chemicals by common modes of action (Barron et al. 2015), and to develop (Kostal et al. 2015) and evaluate (Connors et al. 2014) sustainable design guidelines for less hazardous chemicals. The databases probably only cover a small proportion of the data that have been generated, they differ in their contents, there are large differences in data quality, and they often do not contain the metadata needed for use in chemical assessment and the model development work (e.g., needed to address question 14). To fully exploit the wealth of data that are available will require new ways of working: researchers and the business sector need to be more transparent and open in sharing their data, improved mechanisms are needed to support data sharing, standardization is needed in the presentation of data and metadata, and assessment approaches are needed to determine the quality of the data. Learned societies such as SETAC could play an important role here.
22. How can we improve the communication of risk to different stakeholders? (Rank #20)
The environmental risk assessment of chemicals and other stressors is performed to inform risk management and therefore needs to be communicated in a way that enables effective science‐based decision‐making. This means that the risk assessment should address the protection goals that society values (question 2) and be relevant to the challenges it faces (question 1). The outcome of the assessment should be directly relevant to public and regulatory decision‐making (Scientific Committee on Health and Environmental Risks et al. 2013b) and be communicated in terms that are accessible to a range of stakeholders, including other risk assessors, risk managers, policy makers, and the general public. To establish trust in the risk‐assessment process, information needs to be robust, transparent, and reported objectively, without advocacy or hype (Calow 2014). Communication about risks based on environmental risk assessment methods is often challenged with the “so what?” question (Faber and Van Wensem 2012); for instance, what does it mean when threshold values for contaminants have been exceeded? How should a risk manager or a member of the general public interpret this type of information? If risk‐assessment specialists have difficulty in translating a laboratory toxicity value for a chemical or the exceedance of an environmental quality standard to actual changes in biodiversity or ecological processes in the field (e.g., questions 6, 7, and 11), how is a nonspecialist expected to use this information? What also puzzles stakeholders is that, despite robust prospective risk‐assessment and risk‐management processes, critical levels of chemicals may still occur in the environment. This may be attributable to either improper use or misuse of the chemical or be a consequence of the protection level used in the risk assessment (e.g., protection set at the population level but effects observed at the [sub]individual level). Reporting of these, sometimes high‐profile, events erodes trust in the risk‐assessment process and drives calls for precautionary, hazard‐based assessments or even the rejection of scientific evidence (Apitz et al. 2017). Several authors have suggested that a risk‐management process that is focused on the effects of stressors on natural capital and the ecosystem services it provides and that clearly articulates uncertainties, trade‐offs, and the consequences of chemical use/nonuse may provide an effective framework for risk communication and risk assessment (e.g., Nienstedt et al. 2012; Maltby 2013; question 2).
OUTLOOK
Europe faces significant challenges around the risk assessment and management of chemicals and other stressors. This constrains the region's ability to contribute to the achievement of the global goals for sustainable development. Both the environmental science and the regulatory communities are often working in apparent isolation. This study is the first attempt to set a research agenda for the European research community for the assessment and management of stressor impacts on environmental quality. The questions arising from this exercise are complex. To answer them, it will be necessary to adopt a systems approach for environmental risk assessment and management. In particular, it is important that we establish novel partnerships across sectors, disciplines, and policy areas, which requires new and effective collaboration, communication, and coordination.
This exercise is an important first step in a longer‐term process. The results of this project now need to be disseminated to the policy, business, and scientific communities. The output should be used for setting of research agendas and to inform the organization of scientific networking activities to discuss these questions in more detail and identify pathways for future work. Because there are strong interdependencies between the questions (Figure 2), one way forward would be to establish a large “chemicals in the environment” research program that extends from the “goals” through to the “solutions.” For example, a European Union framework program, involving a number of projects tackling different questions coming out of this exercise, would provide such an opportunity.
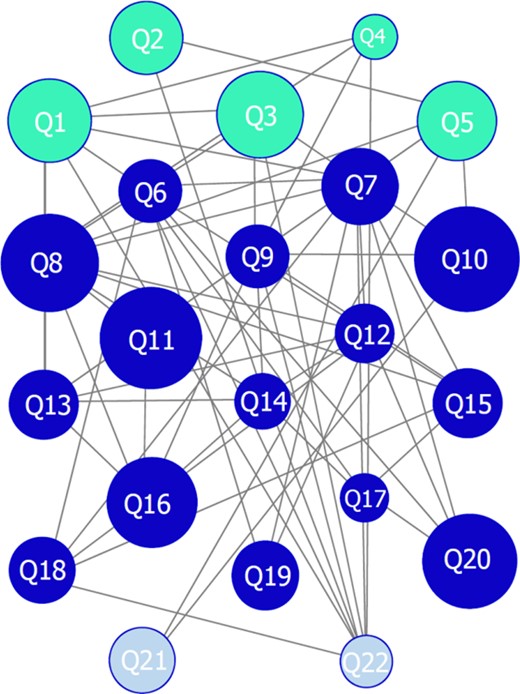
Network map indicating the interrelationships between the different priority questions.
The outputs from this European effort should increase the relevance of environmental research by decreasing scientific uncertainty in assessing and managing environmental risks and increasing the credibility of technical and policy responses to global environmental stressors. The research questions we have prioritized in the present report are not specific to Europe and should therefore be considered in the light of parallel horizon‐scanning activities that have taken place in Africa, Asia‐Pacific, Latin America, and North America. By answering the research questions identified, the European research community will play a pivotal role in achieving the Sustainable Development Goals.
Supplemental Data
The Supplemental Data are available on the Wiley Online Library at DOI: 10.1002/etc.4205.
Acknowledgments
We would like to thank the financial support provided by Baylor University, Tony and Donna Robert, the University of York, and the US Environmental Protection Agency. In kind support was provided by the Society of Environmental Toxicology and Chemistry. We deeply acknowledge all experts that anonymously submitted questions and provided their rankings of the priority questions, which made this exercise possible.
Disclaimer
C. Ajao is a staff member of the European Chemicals Agency. The views and opinions expressed in the present article represent exclusively the personal ideas of the author and do not represent the official position of the agency.
Data availability
All information has been provided in the Supplemental Data.