-
PDF
- Split View
-
Views
-
Cite
Cite
Paola Serio, Raffaella Nenna, Marco Di Maurizio, Stefano Avenali, Roberto Leone, Roberto Baggi, Luigi Arcieri, Bruno Murzi, Andrea Quarti, Marco Pozzi, Lorenzo Mirabile, Fabio Midulla, Outcome of long-term complications after permanent metallic left bronchial stenting in children, European Journal of Cardio-Thoracic Surgery, Volume 53, Issue 3, March 2018, Pages 610–617, https://doi.org/10.1093/ejcts/ezx374
- Share Icon Share
Abstract
We describe the way we treated 7 children with critical long-term complications after metallic balloon-expandable stenting in the left mainstem bronchus.
Endoscopic follow-up included a first bronchoscopy 3 weeks after stenting, then monthly for 3 months, every 4–6 months up to 1 year and at scheduled times to calibrate stent diameter up to final calibration. When major complications occurred, patients underwent chest computed tomographic angiography.
In 1 of the 7 children (median age 2.8 years), metallic left bronchial stenting served as a bridge to surgery. After a median 4-year follow-up, all 7 children experienced recurrent stent ovalizations with stent breakage in 3 and erosion in 1. In 4 children, computed tomographic angiography showed abundant peribronchial fibrous tissue, in 2 left mediastinal rotation and in 1 displacement along the left bronchus after pulmonary re-expansion as the cause of stent-related complication. Of the 7 children, 6 underwent surgery (5 posterior aortopexy and 1 section of the ligamentum arteriosus) and 3 required nitinol stents placement within the metallic ones. One patient completed the follow-up, and 1 patient was lost to follow-up. All 5 remaining children still have permanent bronchial stents in place, patent and re-epithelialized after a median 10.5-year follow-up. There were no deaths.
Satisfactory anatomical relationships when children have stents placed in the left mainstem bronchus alone do not guarantee the final success. Several mechanisms intervene to cause critical stent-related complications in children during growth. Permanent metallic stents should be used carefully, and only in selected patients.
INTRODUCTION
Infants and children occasionally suffer from respiratory symptoms and respiratory distress related to bronchial narrowing [1]. This airway defect may arise from primary stenosis or malacia [2] or from extrinsic compression by anomalous vascular structures. Several anatomical factors predispose the left mainstem bronchus to narrowing: its course is longer and more angled, its calibre is slightly smaller than that of the right bronchus and it lies in a vulnerable position between the pulmonary artery, oesophagus, vertebral bodies and descending aorta [3]. Whatever the cause, bronchial narrowing can make it difficult for children to clear secretions and to ventilate, thus favouring recurrent infections, atelectasis, deteriorated gas exchange and respiratory failure. Bronchial narrowing may also, to some extent, persist despite growth [4, 5], sometimes requiring extensive medical treatment, mechanical ventilatory support or surgery including tracheostomy.
Consensus recommends several surgical options as first-line attempts for managing severe left bronchomalacia causing severe respiratory symptoms in children [6, 7]. Most paediatric series therefore suggest that critically ill children should undergo airway stenting only as the last option [8], because the procedure often leads to fatal complications [9, 10]. Complications such as erosion and migration are more common in the mainstem bronchus than in the trachea, because the bronchial wall is thinner and the cartilage is less supportive [9].
Scanty data report the long-term complications after left mainstem bronchus stenting with permanent devices during growth, and the pathogenesis of these events remains unclear [9–15]. Having reliable information on changes in the spatial relationship between the left mainstem bronchus and the surrounding anatomical structures in a constantly growing chest would help physicians to predict the outcome of the device in the child’s airway and to clarify the indications for permanent metallic balloon-expandable stents in children.
In this study, we describe how we managed critical long-term stent-related complications arising in 7 children after metallic balloon-expandable stenting in the left mainstem bronchus. We also report the anatomical changes taking place in thoracic structures during long-term follow-up.
MATERIALS AND METHODS
We retrospectively reviewed clinical records and chest computed tomographic angiography (CTA) scans of 7 children who had severe stent-related complications after the placement of metallic balloon-expandable stent in the left mainstem bronchus at our Respiratory Endoscopy Unit in Meyer Children Hospital, Florence, Italy, over the period from January 2005 to December 2016.
Indications for metallic balloon-expandable stent placement were severe left bronchomalacia (lumen reduction >70%) causing persistent lung dysventilation areas that required prolonged invasive or non-invasive ventilation, repeated failed extubations and recurrent episodes of severe pneumonia with repeated hospital admissions, often requiring intubation.
Airway cross-section was evaluated visually in each child, through flexible (Karl Storz®, Germany; diameter 2.5/3.7/5.2 mm) or rigid bronchoscopy (Karl Storz; diameter 3.5/4/4.5/5.5 mm). We decided to place a stent in the left mainstem bronchus only after a CTA scan was negative for vascular compression. Stenting devices were chosen according to the bronchial diameter estimated by age and inserted with a rigid bronchoscope in the operating theatre with patients under general anaesthesia (sevoflurane inhalation ± intravenous propofol) and radiological guidance. Once the left segment was visualized, the balloon-expandable metallic stent (Multilink, Abbott®; Jostent, Abbott; Palmaz, Johnson & Johnson®) was expanded under radiographical guidance by filling a balloon using a standard angioplasty syringe equipped with a pressure gauge. The catheter was then removed by deflating the balloon, and bronchoscopic and radiological examinations immediately after stenting confirmed that the stent was correctly located. Technical success was defined as successful stenting in the appropriate site in 1 bronchoscopic session. Clinical success was considered as (i) clinical improvement when the child was weaned off mechanical ventilation or (ii) respiratory symptoms dramatically improved or (iii) the respiratory infection rate decreased after the procedure.
Stent follow-up included a first bronchoscopic examination 3 weeks after the placement and then monthly for the first 3 months. Follow-up then continued every 4–6 months up to 1 year and thereafter at scheduled times to calibrate stent diameter according to the airway growth up to final calibration (12 mm).
For recurrent stent ovalization, if an ad hoc CTA scan confirmed that the bronchial stent abutted vascular structures, the child underwent surgery [7] or the insertion of a silicone Dumon stent (Novatech®) or covered nitinol stent (Silmet®) within the metallic broken one to restore the radial force of the metallic stent.
Chest CTA included a single post-contrast phase scan, with an age and weight-adjusted low-dose protocol: 120 or 80 kV; 120 or 80 mA; collimation 1.5 or 0.75 mm and gantry rotation 0.5 s. Data were post-processed with maximum intensity projection, volume intensity projection, 3D volume rendering, minimum intensity projection and surface rendering of virtual bronchoscopic images and multiplanar reformations.
The study was approved by the research and institutional review board at Meyer Children’s Hospital, Florence, Italy (146/2014).
RESULTS
The study series comprised 7 children, with a median age of 2.8 years (age range 2.5 months–13 years) at the time of stenting. In all of them, left mainstem bronchus stenting immediately reached technical and clinical success. In 1 child whose conditions contraindicated surgery, metallic left bronchial stenting served as a bridge to surgery.
After a median 4-year follow-up (range 3 months–8 years), the 7 children experienced recurrent stent ovalizations with stent breakage in 3 children and erosion in 1. These complications were due to previously non-existing vascular compression on the left mainstem bronchus in all children except 1, in whom the compression was already detected at the time of stenting. CTA showed abundant peribronchial fibrous tissue in 4 children, left mediastinal rotation in 2 and an anatomical displacement of the left bronchial axis after pulmonary re-expansion, with ‘mass effect’ of the restored patent bronchus in 1 child.
Of the 7 children treated, 6 underwent surgery (5 posterior aortopexy and 1 section of the ligamentum arteriosus), 3 required covered nitinol stents within the metallic ones and in 1 patient, the parents declined surgery and referred their child to another centre.
One patient completed the follow-up, and 1 patient was lost to follow-up. The 5 remaining children still have re-epithelialized permanent bronchial stents in place, after a median 10.5-year follow-up (range 6.5–13.5 years), and 2 patients still have the nitinol stent within the broken metallic one (Fig. 1).
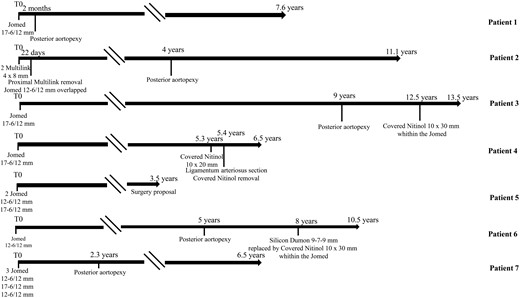
Timeline of the individual course of each patient (in years) after metallic stenting in the left mainstem bronchus (T0). \\indicates suspension of the graphic representation of follow-up when follow-up did not present significant events.
CASES
Patient 1 underwent corrective surgery at birth for complex congenital heart disease (double-inlet left ventricle and transposition of the great vessels), and at the age of 7 months, the Glenn procedure was performed. At the age of 1 year, the girl was endoscopically evaluated for severe recurrent wheezing, recurrent acute respiratory infections resistant to medical therapy and lung dysventilation areas requiring prolonged hospitalizations. Bronchoscopy and CTA documented severe malacia involving the left mainstem bronchus lying close to the descending aorta and left pulmonary artery with mild fibrous tissue interposition (Fig. 2).
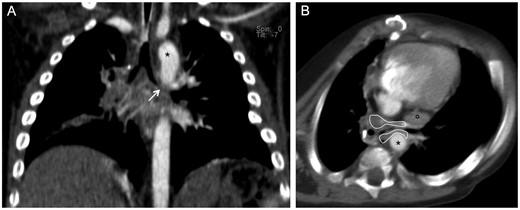
Computed tomographic angiography in Patient 1. (A) Multiplanar coronal reconstruction: severe malacia involving the left mainstem bronchus (white arrow). (B) Axial plane: the left mainstem bronchus lies close to the descending aorta (*) and left pulmonary artery (°) with peribronchial fibrous tissue (area enclosed by the continuous white line) causing severe bronchial narrowing.
Because the patient’s critical clinical status contraindicated surgery, we attempted a conservative approach by inserting a silicone stent (Dumon 6 × 15) in the left mainstem bronchus. One month later, when bronchoscopy showed that the stent had migrated into the trachea, it was replaced with a metallic (Jomed 17-6/12 mm) stent to ensure greater radial pressure. After this procedure, the clinical conditions of the child promptly improved, and 2 months later, she underwent a posterior aortopexy to eliminate the vascular compression on the stent.
At 7.6-year follow-up, the stent is still in place, calibrated to the final diameter, completely patent and re-epithelialized.
Patient 2, a child operated at birth for hypoplastic left heart syndrome, underwent bronchoscopy at the age of 5 months to investigate the causes of impossible weaning from mechanical ventilation after surgery and persistent and relapsing left lung dysventilation. Bronchoscopy showed severe left mainstem bronchial malacia that was treated by placing 2 coronary stents (Multilink 4 × 8 mm).
Proximal stent dislocation just 22 days after the placement, before re-epithelialization, led the endoscopist to remove the proximal coronary stent and to place a metallic expandable stent (Jomed 12-6/12 mm) overlapping the distal one. Soon after this procedure, lung compliance improved significantly, and the child was progressively weaned from mechanical ventilation.
Four years later, mild haemoptysis required an urgent bronchoscopy that disclosed stent ovalization and a pulsatile compression. CTA showed the stent, now deformed, with peribronchial fibrous tissue shifting the bronchus close to the descending aorta and left pulmonary artery and a dilated collateral vessel around the proximal stent (Fig. 3). During a posterior aortopexy, the ovalized bronchial stent was dilated and the airway patency restored. At 11.1-year follow-up, the stent is still in place, re-epithelialized, completely patent and without signs of bleeding.
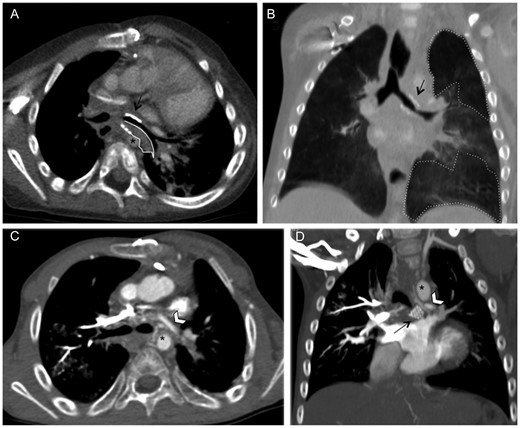
Computed tomographic angiography in Patient 2. (A) Axial plane: abnormal stent dilatation (black arrow) in the left mainstem bronchus with endobronchial and peribronchial fibrous tissue (area enclosed by the continuous white line) causing the bronchial stent to lie close to the centrally positioned descending aorta (*). (B) Coronal plane, minimum intensity projection reconstruction: stent deformation with severe bronchial narrowing (black arrow) and air trapping parenchymal areas (area enclosed by the dotted line). (C) Axial and (D) coronal planes: dilated collateral bronchial vessel around the proximal stent (white arrowhead) surrounded by multiple mediastinal vessels.
Patient 3 underwent bronchoscopy soon after birth for severe respiratory failure requiring mechanical ventilation due to multiple bronchial anomalies causing air trapping and atelectasis. The boy underwent multiple endoscopic procedures to treat several causes of airway obstruction: a congenital membrane obstructing the bronchus intermedius was endoscopically treated with balloon dilation and subsequently with balloon-expandable stent (Palmaz) placement; at 2.8 years of age, another metallic expandable stent (Jomed 17-6/12 mm) was needed to treat severe left bronchial malacia, and a laryngotracheoplasty and subsequent placement of a Montgomery tracheal cannula were needed to treat an acquired subglottic stenosis.
The child continued to have chronic respiratory symptoms, and at 7 years of age, he had biopsy-proven bronchiolitis obliterans organizing pneumonia.
Nine years after the left mainstem bronchus stenting, CTA showed multiple lung dysventilation areas and a stent breakage with abundant peribronchial fibrous tissue causing the bronchus to abut the descending aorta and left pulmonary artery (Fig. 4).
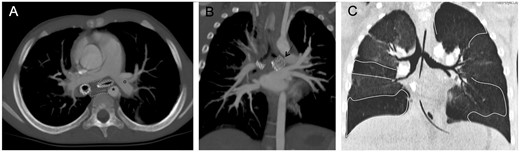
Computed tomographic angiography in Patient 3. (A) Axial and (B) coronal plane, maximum intensity projection reconstructions: the stent in the left mainstem bronchus completely ruptured (black arrow), the subcarinal space filled with fibrous peribronchial tissue (area enclosed by the continuous white line) and the stent in the right mainstem bronchus patent. (C) Coronal minimum intensity projection reconstruction: mosaic pattern of the lung parenchyma characterized by air trapping (area enclosed by the continuous line). The descending aorta (*) and left pulmonary artery (°).
A posterior aortopexy prevented fatal erosion due to the close proximity of the broken stent to vascular mediastinal structures, but left vascular compression was unrelieved, because the peribronchial fibrous tissue transmitted vascular pulsation to the stent.
At 12.5 years, after the left mainstem bronchus stenting, we placed a covered nitinol stent (10 × 30 mm) within the broken metallic one. At 13.5-year follow-up, the patient is in good clinical status and endoscopically monitored.
Patient 4, a 5.9-year-old girl, was referred to our centre for persistent left upper lobe atelectasis unresponsive to non-invasive ventilation. Bronchoscopy and CTA documented a left mainstem bronchial malacia unrelated to pulsatile compression (Fig. 5A). After a metallic stent (Jomed 17-6/12 mm) was inserted, lumen patency recovered and the symptoms improved.
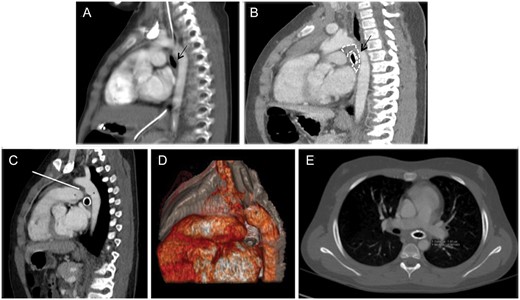
Computed tomographic angiography (CTA) in Patient 4. Pre-stent (A) and post-stent (B) sagittal multiplanar coronal reconstruction. Adequate space between the left mainstem bronchus (A, black arrow) and the vascular structures before stenting. (B) Segmental bronchial narrowing (black arrow) and peribronchial fibrous tissue (area enclosed by the dotted line). The descending aorta (*) and left pulmonary artery (°). Sagittal multiplanar (C) and 3D reconstruction (D) and axial (E) plane CTA. The bronchus is stapled between the left pulmonary artery and the hystmic aorta because of the traction of the ligamentum arteriosus (white arrow on C). Abundant peribronchial fibrous tissue between the aorta, the roof of the left atrium and the bronchus.
Scheduled endoscopic follow-up after 4.5 years disclosed a critical stent ovalization due to a posterior pulsatile compression. The CTA showed abundant peribronchial fibrous tissue interposed between the bronchus and the descending aorta and left pulmonary artery (Fig. 5B). Considering the child’s good health and the adequate respiratory function, we opted for a watchful waiting approach.
The girl was readmitted to our centre because of dysventilation of the left lung 5.3 years after stenting. During endoscopy, abundant granulations occluding the ovalized metallic stent were removed with diode laser (Dornier MedTech®), and a nitinol-covered stent (10 × 20 mm) was placed at the entrance of the bronchus. The girl required another admission to the hospital 1 month later for dyspnoea and pain in the left hemithorax and a CTA detected the left bronchus with abundant fibrous tissue, stapled between the left pulmonary artery and the hystmic aorta as a result of the traction of the ligamentum arteriosus on the left bronchus (Fig. 5C–E). Through a median sternotomy, the ligamentum arteriosus was sectioned, the bronchus was released from the surrounding fibrous tissue and an intraoperative bronchoscopy documented a reopening of the proximal left stented bronchus and the nitinol stent was successfully removed.
At 6.5-year follow-up, the metallic stent is re-epithelialized and the bronchus completely patent.
Patient 5, a 4-year-old child with June syndrome, previously operated for brachiocephalic trunk correction, was referred to our centre for recurrent left pneumonia. Endoscopy showed mild malacia in the distal trachea and proximal left mainstem bronchus. We opted for a conservative approach and scheduled clinical and endoscopic follow-up.
Two years later, we documented a severe obstruction in the left mainstem bronchus, with no signs of pulsatile compression, causing complete left lung atelectasis. Two overlapping metallic stents (Jomed 12-6/12, 17-6/12 mm) were placed in the left bronchus, but recurrent ovalization requiring dilation led to stent breakage. CTA showed an anteroposterior chest diameter reduced for age, mediastinal structures rotated to the left with left bronchial verticalization and the left bronchus lying close to the pulmonary artery (Fig. 6). We suggested surgery to expand the child’s chest, but the parents declined, and after 3.5 years, the patient was lost to follow-up.

Computed tomographic angiography in Patient 5. (A) Axial plane: reduced chest internal anteroposterior diameter (43 mm) for age, with cardiomediastinal structures rotated to the left (dotted and curved lines). (B) Coronal plane: partial left bronchial verticalization with left mainstem bronchus lies close to the left pulmonary artery (°). The descending aorta (*). (C) Parasagittal plane on 3D reconstruction: deformed stent in the left main bronchus, visible also in axial plane (A, black arrow).
Patient 6, a 13-day-old baby, with congenital upper left lobar emphysema, severe respiratory distress and diffuse bronchomalacia was first treated with a coronary stent (Multilink, Abbott) placed in the stenotic superior left lobar bronchus. As the lung condition remained severe, she underwent left superior lobectomy with concurrent stent removal.
At 2.5 months of age, because the baby could not be weaned from mechanical ventilation, a metallic stent (Jomed 12-6/12 mm) was placed to treat the left distal bronchial malacia.
About 5 years later, when bronchoscopy showed relapsing stent ovalizations, posterior aortopexy was undertaken. Three years later, the stent ovalized again and broke. CTA showed a deformed stent, the mediastinal structures appeared shifted and rotated to the left, where the upper left lung parenchyma was absent, so that the descending aorta and left pulmonary artery compressed the left bronchus (Fig. 7).
![Computed tomographic angiography in Patient 6. (A) Axial and parasagittal planes, volume intensity projection reconstruction: stent rupture. (B) Axial plane and corresponding 3D reconstruction: mediastinal shift and rotation to the left (dotted and curved lines) in the previous ipsilateral upper lobectomy; the stent is deformed and lies at an abnormal angle (dashed black arrow). (C) Axial plane: the silicon stent inside the metallic stent has caused the left main bronchus re-expansion touching (double arrows) the vascular structures [left pulmonary artery (°)]. (D) Coronal plane, minimum intensity projection reconstruction: the apical segment in the left lower lobe is overexpanded (area enclosed by the dotted line).](https://oup.silverchair-cdn.com/oup/backfile/Content_public/Journal/ejcts/53/3/10.1093_ejcts_ezx374/1/m_ezx374f7.jpeg?Expires=1749156017&Signature=r0hfQXvk30Ftyp09py55B3L656oB4wuQdA45dOL5lwb82STuunureF-fpqaiad0JTeKAETAARUwC7nVfDA1GImyNmL2yxJ09Vn~H6N104tAX1NYCU-C~UYocKJ5om7ueKmKovXmzPwwQHA-nKXErYwsxmYZN5~HzzMGMzpzCi1b~bC7RxuRXms4AAzj8trNQoKHeJIIVqQAiM5VycN-HrE0HIHsnbOzZEHDo~lakydCLzQ3qGMW93vjktPIvmZrNLMwnp4~7IUXudPn4u5MKteMjTaCFhe70PI4zx3JlJU23dmgiBMIsALipQRX1wdw72gJupjSMAysP-mKSvkmRsA__&Key-Pair-Id=APKAIE5G5CRDK6RD3PGA)
Computed tomographic angiography in Patient 6. (A) Axial and parasagittal planes, volume intensity projection reconstruction: stent rupture. (B) Axial plane and corresponding 3D reconstruction: mediastinal shift and rotation to the left (dotted and curved lines) in the previous ipsilateral upper lobectomy; the stent is deformed and lies at an abnormal angle (dashed black arrow). (C) Axial plane: the silicon stent inside the metallic stent has caused the left main bronchus re-expansion touching (double arrows) the vascular structures [left pulmonary artery (°)]. (D) Coronal plane, minimum intensity projection reconstruction: the apical segment in the left lower lobe is overexpanded (area enclosed by the dotted line).
The critical endoscopic condition of the child was clearly explained to the girl’s parents who chose a conservative approach considering the child’s optimal growth and respiratory status. We inserted a silicon stent (Dumon 9-7-9 mm) subsequently replaced by a covered nitinol stent within the broken metallic one, and this procedure completely restored bronchial patency.
At 10.5-year follow-up, the girl is always under closed clinical and endoscopic monitoring.
Following a perinatal diagnosis of diaphragmatic eventration, microgastria and asplenia, Patient 7, a baby, underwent surgical diaphragmatic plication at birth and required a long intensive care unit stay and mechanical ventilation with tracheostomy for 3 years.
At the age of 13 years, the girl was referred to our centre for recurrent left pneumonia requiring multiple hospitalizations. Spirometry suggested a fixed intrathoracic obstruction. Three metallic stents (Jomed 12-6/12, 17-6/12, 12-6/12 mm) were inserted to treat severe stenomalacia in the distal left mainstem bronchus and the left lung ventilation rapidly improved.
About 2.3 years later, bronchoscopy and CTA showed that when the left lung re-expanded after stenting the bronchus collided with the midline descending aorta and left pulmonary artery (Fig. 8). A posterior aortopexy relieved left bronchial compression avoiding the risk of fatal fistula due to the ‘mass effect’ of the restored patent bronchus.
![Computed tomographic angiography in Patient 7. Maximum intensity projection reconstruction (A) and standard acquisition (B) on the axial plane: completely patent stent (black arrow) collided with the left mainstream bronchus to the left pulmonary artery (°) and descending aorta [(*) middle positioned and shifted forward]; estimated diameter of the stented bronchus. Sagittal plane maximum intensity projection (C) and corresponding 3D reconstruction (D) on parasagittal plane.](https://oup.silverchair-cdn.com/oup/backfile/Content_public/Journal/ejcts/53/3/10.1093_ejcts_ezx374/1/m_ezx374f8.jpeg?Expires=1749156017&Signature=w0XuglyCe0sH6XaEamWkYZaEp0juYWmirWh1fnjTLA4KM9BbCrZts1x2n5I6a9BlIelsVy3DEUyLso~LnK-hkd~9rEsixGIRkk1BKtcG1J3W0p-M8Lh2RZ~cqGMfpgNCO3a6TNcqJWbWKIn76ddE33sSdWHCVVhg7JSOtHsQ7ocs1y79o2fId3JkpWzRNSohj-2u-BIvmkwoBxXJvgQNwetbXFJLdmooU~2QZAkEu4VfLHBP3VQrvmoElgM7G8CNpLfLV8mcNA8fVd0BvjINCW1N9TfJeVGU5C9-mFDQVec0ZtQ3T8DPti-R1tvAk3J4lMoEaFJ0aXFT5IHt37tbGA__&Key-Pair-Id=APKAIE5G5CRDK6RD3PGA)
Computed tomographic angiography in Patient 7. Maximum intensity projection reconstruction (A) and standard acquisition (B) on the axial plane: completely patent stent (black arrow) collided with the left mainstream bronchus to the left pulmonary artery (°) and descending aorta [(*) middle positioned and shifted forward]; estimated diameter of the stented bronchus. Sagittal plane maximum intensity projection (C) and corresponding 3D reconstruction (D) on parasagittal plane.
At 6.5-year follow-up, the girl, asymptomatic with the stent completely patent and calibrated to the final diameter, completed the follow-up.
DISCUSSION
In this series of 7 critically ill children, we provide previously unavailable, detailed information on how we managed long-term severe stent-related complications arising after permanent metallic balloon-expandable stenting in the left mainstem bronchus. Despite successful stenting, all the children in this series needed close endoscopic follow-up to detect and promptly treat potentially life-threatening complications. We also provide radiographic evidence detailing the anatomical changes in chest structures of permanent metallic stenting in the left mainstem bronchus. These severe complications arising after a long-term follow-up depended on factors such as peribronchial tissue formation, mediastinal rotation after lobectomy or owing to a small chest and ‘mass effect’ after restoring patent bronchus.
When we analysed the stent indications and outcomes, we found that in all 7 children, metallic stents were indicated for severe respiratory symptoms, and the left bronchial stenting promptly achieved clinical and technical success. Our findings further confirm that airway stents are a therapeutic option for treating airway obstruction in children, as reported in our previous study on 100 consecutive patients who underwent stent insertions between January 2005 and May 2012 [16]. Nevertheless, when extending the observation to December 2016, we noticed the occurrence of major complications only in the 7 patients included in this study of over 51 children who underwent the placement of 76 metallic balloon-expandable stents in the left mainstem bronchus at our centre in the same period.
As part of our clinical practice, to exclude pre-existing vascular collision or potentially dangerous proximity requiring surgery before stenting, all patients underwent a CTA [7, 17, 18]. The majority of reported complications usually occurred in children when the vascular compression had not been corrected or not completely eliminated prior to placement of a stent [9]. Recently, we published a favourable experience with metallic stenting in patients with severe symptomatic residual malacia after removal of vascular compression [7, 17]. This approach avoided the risk of potentially fatal fistulas due to stent radial pressure and compression from the adjacent structures causing high transmural pressure in the bronchus. Conversely, even though CTA clearly documented vascular compression on the left mainstem bronchus, in 1 child, we placed a metallic stent. We chose this high-risk procedure because the child’s precarious condition contraindicated other forms of surgery. The presence of mild fibrous tissue surrounding the mediastinal structures of this patient tipped the balance between the need for high radial pressure to reopen the bronchus and the danger that excessive transmural pressure might cause a fistula.
An especially important factor was the use of metallic balloon-expandable stents. Metallic stents can effectively relieve bronchomalacia [7, 16, 17], but despite their advantages [5, 17], many studies have already reported major and fatal complications such as migration, fistula, fractures and severe granulation specifically associated with their long-term use [9–15]. Once inserted, they must be considered as a permanent device. Removing a metallic stent is a highly dangerous manoeuvre to be avoided whenever possible [5, 8, 15]. Moreover, the longer a stent has been in place, the more it integrates into the airway wall, and the more difficult it is to remove [10]. To solve this problem, de Trey et al. [19] recently developed a technique where the stent is not completely inflated but just up to the point where it is not floating in the airway. In our series, we decided to remove a metallic stent 22 days after placement only in 1 patient, because it had failed to epithelialize.
Analysing the types of complications, we found that recurrent ovalizations developed in all the 7 children, stent breakage in 3 and initial mucosal erosion in 1. Ample evidence confirms ovalizations as the most frequent stent-related complications, usually treated by balloon dilation [10, 12, 15]. Repeated dilation and a persistent external compression can lead to stent breakage, and sharp parts of the device can lacerate the airway wall [20]. Insofar, if we considered the risk of stent removal to be extremely high, we inserted a removable stent within the broken ones to help restore the bronchus to its circular shape and to avoid internal wall erosion by broken metal meshes. In our study, the patient, who manifested mild haemoptysis due to initial erosion, suffered from cyanotic congenital heart disease and had a dilated collateral vessel close to the stented bronchus as reported by other authors [21, 22].
The major strength of our study is that we fully analysed the anatomical changes in thoracic structures after stenting and we described how we managed stent-related complications during long-term follow-up. How a permanent device influences bronchial growth, and how the stented left mainstem bronchus modifies the spatial relationship with the surrounding structures in a growing child, is the major concern [10, 12]. Our study showed that severe complications reflected factors related to stenting, such as peribronchial tissue formation and the ‘mass effect’ [23] of restoring patent bronchus, and child-related factors such as mediastinal rotation after lobectomy or due to a small thorax.
The most frequent pathological mechanism underlying stent-related complications was peribronchial fibrous tissue formation as shown at bronchial stent level in 4 patients. A florid inflammatory fibrovascular proliferation surrounding the stent, resembling foreign body reactions has been described. Furthermore, peribronchial fibrous tissue could also be due to scarring after previous thoracic surgery [24]. We suspect that although this tissue reduces the risk of a fatal fistula because it separates the airway from the mediastinal vessels, it increases the stent’s compressive load inducing relapsing ovalization and eventually leading to breakage. On the other hand, bronchial stenting within the chest may alter distances between the bronchus and the vessels. This pathogenetic mechanism is the well-known adverse ‘mass effect’ on adjacent vascular structures [23]. A posterior aortopexy was performed in 5 children to restore the correct anatomical distance between the airways and the vascular structures, and it avoided the risk of potentially fatal fistulas [7]. In 1 patient, the bronchus was stapled between the left pulmonary artery and the hystmic aorta because of the traction of the ligamentum arteriosus on the left bronchus. The section of this ligamentum resolved the compression.
Equally important mechanisms causing long-term stent-related complications in our series were mediastinal structure shift and distortion after lobectomy as found in 1 child or due to a small thorax in the child with June syndrome. Others have already reported postoperative tracheobronchial distortion related to fibrous scarring, particularly after cardiac surgery [24].
CONCLUSION
Satisfactory anatomical relationships existing when a balloon-expandable metallic stent is placed in the left mainstem bronchus alone cannot guarantee final success in a constantly growing chest. The decision to use a permanent device in this site must take into account the potential catastrophic clinical sequela occurrence after a long-term follow-up. A closed endoscopic, radiological and clinical follow-up in a specialized centre is needed to avoid life-threatening events and to guarantee appropriate management of possible long-term complications. For this reason, considering the peculiar anatomical feature in the left bronchus, for treating severe left bronchial narrowing, we suggest to reserve permanent stents only as ultima ratio in very critical conditions.
Conflict of interest: none declared.
REFERENCES
Author notes
Presented at the 26th International Congress of the European Respiratory Society, London, UK, 3–7 September 2016.