-
PDF
- Split View
-
Views
-
Cite
Cite
Lucio Cagini, Stefania Balloni, Vienna Ludovini, Marco Andolfi, Alberto Matricardi, Rossella Potenza, Jacopo Vannucci, Annamaria Siggillino, Francesca Romana Tofanetti, Guido Bellezza, Maria Bodo, Francesco Puma, Lorella Marinucci, Variations in gene expression of lung macromolecules after induction chemotherapy for lung cancer, European Journal of Cardio-Thoracic Surgery, Volume 52, Issue 6, December 2017, Pages 1077–1082, https://doi.org/10.1093/ejcts/ezx200
- Share Icon Share
Abstract
Preoperative chemotherapy may play a role in postoperative respiratory complications due to subclinical parenchymal damage. We investigated the gene expression of lung tissue components after neoadjuvant chemotherapy of alveolar–capillary membrane, extracellular matrix and membrane proteins.
The study group included 14 patients submitted to pulmonary resection for lung cancer after 3 cycles of gemcitabine–cisplatin, while the control group included 14 naive-treatment patients. RNA was extracted from frozen tissue obtained by healthy lung specimens using EZ1 RNA Universal Tissue kit and automatically purified by BioRobot EZ1 instrument. Three hundred nanograms of total RNA was reverse transcribed to complementary DNA and used to evaluate the gene expression of type I and III collagen, elastin, syndecan, metalloproteinase 13 and aquaporins (AQPs) in real-time polymerase chain reaction. Results were expressed as the mean ± standard deviation of 3 independent experiments. Analysis of variance followed by Sheffe’s F-test was performed.
Among the alveolar–capillary membrane and extracellular matrix genes, type I–III collagens and syndecan were significantly up-regulated (+645%, +327% and +261%, respectively), while elastin and metalloproteinase 13 were down-regulated in the study group versus control group (−46% and −77%, respectively). Furthermore, chemotherapy was associated with a significant up-regulation of AQP expressions (AQP1:+51% and AQP5:+36%).
We observed, in the treated group, increases in the mean values of gene expressions for macromolecules involved in the remodelling of both the alveolar septa and parenchyma scaffold, thereby supporting the hypothesis that induction chemotherapy may foster a fibrosing effect on the pulmonary parenchyma and lead to altering the alveolar–capillary membrane.
INTRODUCTION
Several trials over the past 2 decades have evidenced a greater benefit from induction chemotherapy and/or radiotherapy preceding surgery compared to surgery alone in patients with locally advanced non-small-cell lung cancer (NSCLC) [1].
However, the antineoplastic agents utilized in induction chemotherapy regimens have been reported to cause pulmonary toxicity and even respiratory failure [2].
This toxicity is often misdiagnosed due to its shared clinical and radiographic manifestations with other pulmonary diseases, and also changes in respiratory function are rarely clinically relevant [3]. Several authors have suggested that induction chemotherapy, especially prior to pneumonectomy, can lead to an increased rate of postoperative respiratory complications, possibly a role for platinum-based induction chemotherapy in increasing this risk [4–7].
Induction chemotherapy can provoke diffuse interstitial fibrotic alteration with remodelling of the alveolar septa and an increase in the content of proteins of the extracellular matrix (ECM) [8]. Specifically, excesses of collagen type I and III, which are components of both the thick portion of the air–blood barrier [9] and the ECM [10], are responsible for the development of fibrotic involvement.
Moreover, these alterations could play a role in an increased risk of postoperative respiratory complications following induction chemotherapy.
Therefore, the primary objective of this study was to assess the gene expressions of alveolar–capillary membranes (ACMs) (type III collagen, elastin and syndecan), ECM (type I collagen, elastin, syndecan and metalloproteinase 13) and membrane protein aquaporins (AQPs) by real-time polymerase chain reaction (PCR), of macroscopically healthy lung samples taken during surgery for NSCLC after 3 cycles of gemcitabine–cisplatin chemotherapy over at least 1 month. The secondary aim was to investigate the correlations between gene expressions and histological results following induction chemotherapy.
MATERIALS AND METHODS
Study design
From 1 March to 31 December 2015, we prospectively enrolled 14 consecutive patients affected by NSCLC scheduled for major pulmonary resection—lobectomies or pneumonectomies—after 3 courses of induction chemotherapy for at least 1 month. For each case, a control was matched for age, sex, smoking habits and type of surgical resection, from the series of patients scheduled for major pulmonary resection who did not receive induction chemotherapy over the same enrolment period. Given that it was a prospective study, it was meant to investigate gene expression changes. Moreover, as this had never been done before, to our knowledge (i.e. no previous data to infer from), we did not have any a priori indication of whether any difference was to be expected and the size of any possible changes. Accordingly, we could not formally pre-specify the sample size.
All 28 patients underwent elective pulmonary resection for NSCLC with curative intent. Inclusion criteria were age ≥18 years and an American Society of Anesthesiologists score ≤3, as well as informed consent to the use of surgical specimens for research purposes. Exclusion criteria were unwillingness to participate or refusal to sign the informed consent, pulmonary fibrosis or interstitial lung disease, radiological signs of atelectasis, preoperative radiotherapy, impossibility to perform surgical procedure at scheduled times, urgent or emergency surgical procedures and severe adverse events related to chemotherapy toxicity. Preoperative evaluation included a detailed anamnesis along with a cardiopulmonary assessment including blood gas analysis, a 12-lead electrocardiogram, spirometry and diffusing capacity of the lung for carbon monoxide (DLCO). Echocardiography was performed to exclude the presence of right ventricle overload, left ventricle diastolic dysfunction and cardiac impairments. All surgical procedures were performed using standard posterior–lateral thoracotomy or a 3-portal video-assisted thoracic surgery under general anaesthesia with selective one-lung ventilation and insertion of radial arterial catheters for constant blood pressure monitoring.
This study was approved by the local ethics committee and was conducted in accordance with ethical principles of the latest version of the Declaration of Helsinki.
Lung tissue specimens
A peripheral lung tissue sample approximately 2 × 2 cm and macroscopically normal, at least 5 cm from the tumour, was taken from the obtained surgical specimen. Subsequently, a portion of this tissue sample was immediately placed in a solution containing RNAlater (Qiagen S.p.A., Milan, Italy) and stored in a freezer at −80°C until RNA extraction. While the remaining formalin-fixed specimen was sent to the Anatomic Pathology and Histology laboratory of Perugia University for histological evaluation. Sections of formalin-fixed and paraffin-embedded tissues, 4 μm thick, were prepared from the surgical specimens for histological evaluation. Haematoxylin and eosin staining, as well as histochemical analysis with Masson’s trichrome, was performed. The pathologist was blinded to the preoperative treatments of the patients.
2.4 Gene expression analyses
Total RNA was extracted from frozen tissues after thawing and homogenizing by IKA Ultra-Turrax and QIAzol Lysis Reagent. RNA was extracted using the phenol chloroform method. From the aqueous phase, RNA was automatically purified by BioRobot EZ1using EZ1 RNA Universal Tissue kit instrument according to the manufacturer’s instructions (Qiagen S.p.A., Milan, Italy). RNA was eluted in 50 μl RNase-free water and stored at −80°C until use. The quality, integrity and quantity of the total RNA was evaluated with Experion™ Bioanalyzer (Bio-Rad Technologies, Milan, Italy). Three hundred nanograms of total RNA were subjected to reverse transcription in a final volume of 20 μl using 200 units of M-MLV (Moloney murine leukaemia virus) reverse transcriptase (Invitrogen) for reaction with an oligo-dT primer (Invitrogen). Real-time PCR was performed using 2 μl of cDNA prepared from the reverse transcription reaction. The primer sequences of each gene were obtained from Invitrogen and are listed in Table 1. Real-time PCR was carried out in an Mx3000P cycler (Stratagene, Amsterdam, Netherlands) using FAM for detection and ROX as a reference dye as described previously [11]. Negative controls were included to evaluate DNA contamination of isolated RNA and reagents. One-step PCR was performed in 25 μl of Brilliant SYBR(r) Green QPCR Master Mix (Stratagene, Amsterdam, Netherlands) according to the manufacturer’s instructions. Product formation was monitored continuously with the fluorescent double-stranded DNA binding dye SYBR(r) Green at each annealing step. The fold changes of the gene expressions of the samples were normalized to the housekeeping (endogenous) gene glyceraldehyde-3-phosphate dehydrogenase. Immediately after PCR, a melting curve was realized by raising the incubation temperature from 55°C to 95°C to confirm amplification specificity. All samples and templates for standard curves were run in triplicate. Quantitative analysis was performed using the comparative (ΔΔCT) method; the CT value is defined as the cycle number when the detected fluorescence exceeds the threshold value, ΔCT was considered the difference between the CT of a target gene and the endogenous control, whereas ΔΔCT was considered the difference between the ΔCT of the analysed sample and the calibrator (control sample).
mRNA . | Sequences (5ʹ–3ʹ) . | Product (bp) . | GenBank Accession No. . |
---|---|---|---|
Collagen type I | Fw: GTGAGACAGGCGAACAGG | 129 | NM_000088.3 |
Rv: GACCAGCAGGCACAGAGG | |||
Collagen type III | Fw: TAACACTGGTGCTCCTGGCA | 291 | NM_000090.3 |
Rv: GTACGGTCCAGGATCCCCTT | |||
Syndecan | Fw: TCTGACAACTTCTCCGGCTC | 210 | NM_001006946 |
Rv: CCACTTCTGGCAGGACTACA | |||
Elastin | Fw: CTGGAATTGGAGGCATCG | 200 | NM_000501.3 |
Rv: ACCTGGGACAACTGGAAT | |||
MMP13 | Fw: TTCCCAGTGGTGGTGATGAA | 128 | NM_002427.2 |
Rv: CATGGAGCTTGCTGCATTCT | |||
AQP 1 | Fw: TTGGACACCTCCTGGCTATTGACT | 98 | NM_198098 |
Rv: CCAGTGGTTGCTGAAGTTGTGTGT | |||
AQP 5 | Fw: CGCTCAACAACAACACAACGC | 180 | NM_001651 |
Rv: CCAGTGAAGTAGATTCCGACAAGG | |||
GAPDH | Fw: TGGTATCGTGGAAGGACTCATGAC | 188 | XM_001726954.1 |
Rv: ATGCCAGTGAGCTTCCCGTTCAGC |
mRNA . | Sequences (5ʹ–3ʹ) . | Product (bp) . | GenBank Accession No. . |
---|---|---|---|
Collagen type I | Fw: GTGAGACAGGCGAACAGG | 129 | NM_000088.3 |
Rv: GACCAGCAGGCACAGAGG | |||
Collagen type III | Fw: TAACACTGGTGCTCCTGGCA | 291 | NM_000090.3 |
Rv: GTACGGTCCAGGATCCCCTT | |||
Syndecan | Fw: TCTGACAACTTCTCCGGCTC | 210 | NM_001006946 |
Rv: CCACTTCTGGCAGGACTACA | |||
Elastin | Fw: CTGGAATTGGAGGCATCG | 200 | NM_000501.3 |
Rv: ACCTGGGACAACTGGAAT | |||
MMP13 | Fw: TTCCCAGTGGTGGTGATGAA | 128 | NM_002427.2 |
Rv: CATGGAGCTTGCTGCATTCT | |||
AQP 1 | Fw: TTGGACACCTCCTGGCTATTGACT | 98 | NM_198098 |
Rv: CCAGTGGTTGCTGAAGTTGTGTGT | |||
AQP 5 | Fw: CGCTCAACAACAACACAACGC | 180 | NM_001651 |
Rv: CCAGTGAAGTAGATTCCGACAAGG | |||
GAPDH | Fw: TGGTATCGTGGAAGGACTCATGAC | 188 | XM_001726954.1 |
Rv: ATGCCAGTGAGCTTCCCGTTCAGC |
PCR: polymerase chain reaction; MMP13: metalloproteinase 13; AQP: aquaporin; GAPDH: glyceraldehyde-3-phosphate dehydrogenase; Rv: reverse; Fw: forward.
mRNA . | Sequences (5ʹ–3ʹ) . | Product (bp) . | GenBank Accession No. . |
---|---|---|---|
Collagen type I | Fw: GTGAGACAGGCGAACAGG | 129 | NM_000088.3 |
Rv: GACCAGCAGGCACAGAGG | |||
Collagen type III | Fw: TAACACTGGTGCTCCTGGCA | 291 | NM_000090.3 |
Rv: GTACGGTCCAGGATCCCCTT | |||
Syndecan | Fw: TCTGACAACTTCTCCGGCTC | 210 | NM_001006946 |
Rv: CCACTTCTGGCAGGACTACA | |||
Elastin | Fw: CTGGAATTGGAGGCATCG | 200 | NM_000501.3 |
Rv: ACCTGGGACAACTGGAAT | |||
MMP13 | Fw: TTCCCAGTGGTGGTGATGAA | 128 | NM_002427.2 |
Rv: CATGGAGCTTGCTGCATTCT | |||
AQP 1 | Fw: TTGGACACCTCCTGGCTATTGACT | 98 | NM_198098 |
Rv: CCAGTGGTTGCTGAAGTTGTGTGT | |||
AQP 5 | Fw: CGCTCAACAACAACACAACGC | 180 | NM_001651 |
Rv: CCAGTGAAGTAGATTCCGACAAGG | |||
GAPDH | Fw: TGGTATCGTGGAAGGACTCATGAC | 188 | XM_001726954.1 |
Rv: ATGCCAGTGAGCTTCCCGTTCAGC |
mRNA . | Sequences (5ʹ–3ʹ) . | Product (bp) . | GenBank Accession No. . |
---|---|---|---|
Collagen type I | Fw: GTGAGACAGGCGAACAGG | 129 | NM_000088.3 |
Rv: GACCAGCAGGCACAGAGG | |||
Collagen type III | Fw: TAACACTGGTGCTCCTGGCA | 291 | NM_000090.3 |
Rv: GTACGGTCCAGGATCCCCTT | |||
Syndecan | Fw: TCTGACAACTTCTCCGGCTC | 210 | NM_001006946 |
Rv: CCACTTCTGGCAGGACTACA | |||
Elastin | Fw: CTGGAATTGGAGGCATCG | 200 | NM_000501.3 |
Rv: ACCTGGGACAACTGGAAT | |||
MMP13 | Fw: TTCCCAGTGGTGGTGATGAA | 128 | NM_002427.2 |
Rv: CATGGAGCTTGCTGCATTCT | |||
AQP 1 | Fw: TTGGACACCTCCTGGCTATTGACT | 98 | NM_198098 |
Rv: CCAGTGGTTGCTGAAGTTGTGTGT | |||
AQP 5 | Fw: CGCTCAACAACAACACAACGC | 180 | NM_001651 |
Rv: CCAGTGAAGTAGATTCCGACAAGG | |||
GAPDH | Fw: TGGTATCGTGGAAGGACTCATGAC | 188 | XM_001726954.1 |
Rv: ATGCCAGTGAGCTTCCCGTTCAGC |
PCR: polymerase chain reaction; MMP13: metalloproteinase 13; AQP: aquaporin; GAPDH: glyceraldehyde-3-phosphate dehydrogenase; Rv: reverse; Fw: forward.
Statistical analysis
Results shown in Fig. 1 are the mean ± standard deviations of 3 independent experiments, each performed in triplicate. Statistical analysis was performed by analysis of variance, followed by Scheffe’s F-test. A P-value of <0.05 was considered statistically significant.
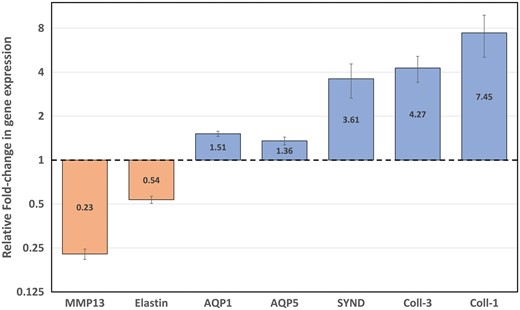
Expression (mRNA levels) of type I and III collagens, syndecan, AQP-1, AQP-5, elastin and MMP13 in healthy lung parenchyma specimens. mRNA levels were quantified by real-time quantitative PCR. Results are plotted on a log-2 scale and expressed as relative fold change in glyceraldehyde-3-phosphate dehydrogenase-normalized mRNA values. Values are the mean ± SD of 3 independent experiments, each performed in triplicate for each of the subjects. mRNA: messenger RNA; PCR: polymerase chain reaction; MMP13: metalloproteinase 13; AQP: aquaporin; Coll: collagen; SYND: syndecan.
Data are presented as means and SD unless specifically stated. Patients’ clinical characteristics were compared by the t-test for continuous variables and by the χ2 test for frequencies. ΔΔCTs (H0 = ΔΔCT equal to 0) and fold changes (H0 = fold change equal to 1) in gene expression were analysed by the Student’s t-test with Bonferroni adjustment to account for multiple comparisons. All analyses were performed with SAS 9.4 (SAS Insitute, Inc., Cary, NC, USA).
RESULTS
No statistically significant differences were observed between the 2 patient groups in terms of age, sex, side of surgery, the number of lobectomies or pneumonectomies performed and preoperative percentage forced expiratory volume in 1 s (Table 2). Regarding DLCO, we found that the study group had a mean lower value compared with the control group.
Characteristics . | Study group . | Control group . | P-value . |
---|---|---|---|
Age | 61 ± 10 | 64 ± 7 | 0.35 |
Male/female | 9/5 | 10/4 | 0.69 |
Smoke: no/former/yes | 2/5/7 | 3/3/8 | 0.68 |
FEV1% | 89 ± 20 | 104 ± 21 | 0.08 |
DLCO% | 78 ± 17 | 98 ± 9 | 0.008 |
PaO2 (mmHg) | 80 ± 9 | 84 ± 8 | 0.29 |
Lobectomies | 11 | 11 | |
Pneumonectomies | 3 | 3 | |
Histology | ADC: 8 | ADC: 9 | |
Squamous: 4 | Squamous: 4 | ||
Large cell: 2 | Large cell: 1 | ||
Stage | IIIA: 3 | IIIA: 7 | |
IIB: 4 | IIA: 3 | ||
IIA: 1 | IIB: 2 | ||
IB: 3 | IB: 2 | ||
IA: 3 |
Characteristics . | Study group . | Control group . | P-value . |
---|---|---|---|
Age | 61 ± 10 | 64 ± 7 | 0.35 |
Male/female | 9/5 | 10/4 | 0.69 |
Smoke: no/former/yes | 2/5/7 | 3/3/8 | 0.68 |
FEV1% | 89 ± 20 | 104 ± 21 | 0.08 |
DLCO% | 78 ± 17 | 98 ± 9 | 0.008 |
PaO2 (mmHg) | 80 ± 9 | 84 ± 8 | 0.29 |
Lobectomies | 11 | 11 | |
Pneumonectomies | 3 | 3 | |
Histology | ADC: 8 | ADC: 9 | |
Squamous: 4 | Squamous: 4 | ||
Large cell: 2 | Large cell: 1 | ||
Stage | IIIA: 3 | IIIA: 7 | |
IIB: 4 | IIA: 3 | ||
IIA: 1 | IIB: 2 | ||
IB: 3 | IB: 2 | ||
IA: 3 |
Pathological staging was classified according to the American Joint Committee on Cancer 2016 guidelines.
ADC: adenocarcinoma; Large cell: large cell carcinoma; Squamous: squamous carcinoma.
Characteristics . | Study group . | Control group . | P-value . |
---|---|---|---|
Age | 61 ± 10 | 64 ± 7 | 0.35 |
Male/female | 9/5 | 10/4 | 0.69 |
Smoke: no/former/yes | 2/5/7 | 3/3/8 | 0.68 |
FEV1% | 89 ± 20 | 104 ± 21 | 0.08 |
DLCO% | 78 ± 17 | 98 ± 9 | 0.008 |
PaO2 (mmHg) | 80 ± 9 | 84 ± 8 | 0.29 |
Lobectomies | 11 | 11 | |
Pneumonectomies | 3 | 3 | |
Histology | ADC: 8 | ADC: 9 | |
Squamous: 4 | Squamous: 4 | ||
Large cell: 2 | Large cell: 1 | ||
Stage | IIIA: 3 | IIIA: 7 | |
IIB: 4 | IIA: 3 | ||
IIA: 1 | IIB: 2 | ||
IB: 3 | IB: 2 | ||
IA: 3 |
Characteristics . | Study group . | Control group . | P-value . |
---|---|---|---|
Age | 61 ± 10 | 64 ± 7 | 0.35 |
Male/female | 9/5 | 10/4 | 0.69 |
Smoke: no/former/yes | 2/5/7 | 3/3/8 | 0.68 |
FEV1% | 89 ± 20 | 104 ± 21 | 0.08 |
DLCO% | 78 ± 17 | 98 ± 9 | 0.008 |
PaO2 (mmHg) | 80 ± 9 | 84 ± 8 | 0.29 |
Lobectomies | 11 | 11 | |
Pneumonectomies | 3 | 3 | |
Histology | ADC: 8 | ADC: 9 | |
Squamous: 4 | Squamous: 4 | ||
Large cell: 2 | Large cell: 1 | ||
Stage | IIIA: 3 | IIIA: 7 | |
IIB: 4 | IIA: 3 | ||
IIA: 1 | IIB: 2 | ||
IB: 3 | IB: 2 | ||
IA: 3 |
Pathological staging was classified according to the American Joint Committee on Cancer 2016 guidelines.
ADC: adenocarcinoma; Large cell: large cell carcinoma; Squamous: squamous carcinoma.
In the study group, chemotherapy-related toxicity was in line with that expected for the regimen used; i.e. drug-related adverse events were mild to moderate in intensity without any severe adverse events [12].
Overall, we recorded 1 postoperative pulmonary complication defined according to the Melbourne Group Scale Version-2 (MGS-2) score [13] and 1 case of atrial fibrillation in the study group and 2 cases of atrial fibrillations in the control group. There was no recorded case of mortality in either group.
Primary outcome measures
The real-time PCR analyses indicated that the messenger RNAs of both type I and III collagens and syndecan were markedly and significantly up-regulated (Bonferroni-adjusted P-value < 0.001) in the treated group, compared with the control group (+645%, +327% and +261%, respectively). Even though to a lesser extent, also the AQP transcription levels of the treated patients were up-regulated (AQP1: +51% and AQP5: + 36%; Bonferroni-adjusted P-value <0.001). On the other hand, elastin and metalloproteinase 13 messenger RNA expression levels were significantly down-regulated in the treated group versus the control group (−46% and −77%, respectively; Bonferroni-adjusted P-value <0.001) (Fig. 1).
Histological evaluation
The study group patients had subverted parenchyma architectures, characterized by diffuse fibrosing process, most often around vascular structures and along the septa and ACMs. In some cases, microcystic and emphysematous areas were evident in honeycombing patterns. Foci of bronchopneumonia with acute neutrophil-rich inflammation-filling bronchioles and alveolar spaces were observed; as shown in Fig. 2, fibrosis was more evident with Masson’s trichrome staining. In the control group, only focal lung alterations were observed, characterized by mild interstitial inflammation, few scattered neutrophils and isolated clusters of foamy macrophages in the alveolar spaces.
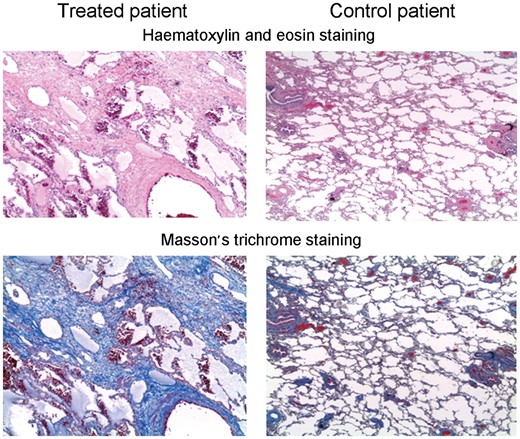
In the chemotherapy group, histological examination of lung parenchyma showed a completely subverted architecture, characterized mainly by a diffuse fibrosing process, particulary around vascular structures and along septa and alveolar–capillary membranes. In some cases, microcystic and emphysematous areas, in a likely honeycombing pattern, were evident. Fibrosis was more evident following Masson’s trichrome staining.
DISCUSSION
Treatment-related toxicity negatively contributes to overall survival in NSCLC patients. The impact of induction chemotherapy on postoperative morbidity and mortality, especially after pneumonectomy, still remains controversial [4, 5, 14–18].
In this regard, randomized trials, comparing surgery after neoadjuvant treatment with definitive radiotherapy as local curative treatment options, have reported that surgical arms were at a risk of treatment-associated mortality, mainly postoperative death, and overall mortality was significantly higher than after radiotherapy [19–21].
However, several studies have indicated a fibrosing effect on the pulmonary parenchyma [6, 8], lower tensile strengths of the pulmonary vessel and bronchi [22] as well as a decrease in the diffusion capacity of the ACM [6]. Moreover, other studies have reported that a reduction of DLCO can be a frequent event, mainly sub-clinical and asymptomatic. It has also been suggested that this event may indicate an impairment of the ACM due to chemotherapy [6], possibly exposing patients to a greater risk of lung injury from surgery [4, 23]. To date, it has been hypothesized that the inflammatory response to chemotherapy may be associated with a triggering of this event: alveolar inflammation with remodelling of the alveolar septa and an increase in the content of proteins of the ECM [8]. The final result being diffuse fibrotic involvement of the pulmonary parenchyma.
This study included patients submitted to pulmonary resection for lung cancer, and we compared the gene expression of macromolecules of both the ACMs and parenchyma scaffolds of macroscopically healthy non-tumoural lung samples. Both the patient groups were made up of those receiving and not receiving platinum-based neoadjuavant chemotherapy.
It has been suggested that platinum-based induction chemotherapy can be associated with diffuse fibrotic involvement of the pulmonary parenchyma [9]. Specifically, collagen types I and III are components of both the thick portion of the air–blood barrier [9] and the macromolecules of ECM whereby their excesses can lead to fibrotic involvement [10]. Regarding collagen types I and III levels, the treated group had significantly higher up-regulated mean levels compared to the non-treated group. Additionally, overall increases in the fibrotic components of the lung parenchyma (Fig. 2) and a reduction in the diffusion capacity of the ACM were present in the treated group only. Likewise, down-regulations of both elastin and the collagenase metalloproteinase 13 gene were observed only for the study group. Furthermore, we observed up-regulations for the gene expression of syndecan, AQP 1 and AQP 5. Syndecan controls the permeability of the pores through which water circulates at the level of the basement membrane [9], while AQP 1 and AQP 5 are selective water transporters. Being so, our finding may suggest an alteration of proteins and macromolecules involved in the physiological response to changes in both fluid volume and osmolarity [24, 25].
The results of our study suggest that, following platinum-based chemotherapy, NSCLC patients had an up-regulation of genes reliably predicting both interstitial lung oedema and lung fibrosis, along with a down-regulation of genes associated in elastic plasticity, thereby triggering an increase in the fibrous component of the pulmonary scaffold. Specifically, in the study group, histological examination evidenced diffuse pulmonary damage and a combination of subacute and chronic lung involvement, not evidencing a specific pattern of parenchymal involvement, but instead, a subverted architecture characterized by a diffuse fibrosing process, especially around the vascular structures and along the septa and ACMs having microcystic formations. Thus, this sub-clinical damage may have influenced the observed reductions in DLCO values following induction chemotherapy. In fact, we excluded patients with a pre-existing history of interstitial lung disease, respiratory symptoms and atelectasis from the study, in order to minimize the risk of patent selection bias. Finally, according to data collected and its distribution, smoking habit did not play a role in the onset of these alterations.
Limitations
Limits of this study included that complications were not an end-point and we could not evaluate the possible prognostic impact of our findings due to the reduced number of patients. Therefore, the results from this observational non-randomized study should be regarded as hypothesis generating, deserving further confirmatory studies.
CONCLUSION
In conclusion, we observed, in the treated group, increases in the mean values of gene expression for macromolecules involved in the remodelling of both the alveolar septa and parenchyma scaffold, thereby supporting the hypothesis that induction chemotherapy may foster a fibrosing effect on the pulmonary parenchyma and lead to an alteration of the ACM.
ACKNOWLEDGEMENTS
The authors wish to thank Thomas Kilcline for his suggestions regarding the final drafting of this manuscript.
Funding
This study was supported by a grant from the Italian Association for Cancer Research (AIRC) [No. 15713] and by the Basic Research Fund, University of Perugia, Assignment 2015.
Conflict of interest: none declared.
REFERENCES
Author notes
Presented at the 24th Annual Meeting of the European Society of Thoracic Surgeons, Naples, Italy, 29 May–1 June 2016.
- polymerase chain reaction
- gene expression
- extracellular matrix
- lung
- chemotherapy regimen
- water channels
- collagen
- dna, complementary
- elastin
- genes
- objective (goal)
- membrane proteins
- tissue membrane
- metalloproteases
- up-regulation (physiology)
- blood capillaries
- lung volume reduction
- lung cancer
- rna
- lung parenchyma
- respiratory complication
- syndecan
- macromolecule
- parenchyma
- preoperative chemotherapy
- chemotherapy, neoadjuvant
- cisplatin/gemcitabine
- aquaporin 1