-
PDF
- Split View
-
Views
-
Cite
Cite
Jeremie Jaussaud, Matthieu Biais, Joachim Calderon, Jean Chevaleyre, Pascale Duchez, Zoran Ivanovic, Thierry Couffinhal, Laurent Barandon, Hypoxia-preconditioned mesenchymal stromal cells improve cardiac function in a swine model of chronic myocardial ischaemia, European Journal of Cardio-Thoracic Surgery, Volume 43, Issue 5, May 2013, Pages 1050–1057, https://doi.org/10.1093/ejcts/ezs549
- Share Icon Share
Abstract
Cell loss during cardiac injection and hostility of the host-tissue microenvironment have the potential to diminish the overall effect of stem cell therapy. The purposes of this study were to evaluate the effect of a hypoxic preconditioning of mesenchymal stromal cells (MSC), to determine its safety and effectiveness, and to improve the efficacy of cell therapy using MSC in the setting of chronic myocardial ischaemia in swine.
Myocardial ischaemia was induced by an ameroid constrictor. Human MSC were cultured under normoxic (20% O2) or hypoxic conditions (1.5% O2) before transplantation. One month after ischaemia, pigs were randomly assigned to saline injection (sham), and 1 × 106/kg normoxic or hypoxic MSC transplantation into the ischaemic inferior-lateral zone.
Twenty-seven pigs were operated on and the mortality rate was 33.3%. The remaining 18 animals were randomly assigned to sham (n = 4), normoxic (n = 8) or hypoxic MSC (n = 6) treatment. Global systolic (left ventricle ejection fraction, P = 0.04) and diastolic (E/Ea, P = 0.008) functions were increased in the hypoxic group compared with other groups. The peak of 2-dimensional longitudinal strain was less altered in the hypoxic group compared with other groups (P < 0.001). Haemodynamic data showed that dP/dT max was improved in the hypoxic group compared with the other group (P < 0.01). Capillary density was increased in the hypoxic group (P = 0.001). MSC density was significantly higher in the ischaemic zone in the hypoxic group (P < 0.01).
MSC engraftment with hypoxic preconditioning significantly improves capillary density and cell survival, resulting in improvement in global, regional and diastolic left ventricular functions. This highlights the therapeutic potential of transplanting hypoxic-preconditioned MSC in the setting of chronic ischaemic heart failure.
INTRODUCTION
Cardiac stem cell transplantation may be a promising approach to improve cardiac regeneration and/or vascularization in the context of heart failure or myocardial ischaemia. From a clinical approach, bone marrow stromal or mesenchymal stromal cells (MSC) could be interesting candidate cells, as they are easily accessible, contain various multipotential progenitor cells, offer the capacity to be immune privileged and have been successfully transplanted into hearts without immunosuppression [1]. Both animal and human clinical studies have provided evidence that MSC transplantation can improve cardiac function through angiogenesis and possible myogenesis after myocardial infarction (MI) via a paracrine mechanism (including vascular endothelial growth factor, insulin growth factor, stromal cell-derived factor-1 secretion) [2]. A major dilemma is the low survival rate of transplanted cells in the ischaemic and peri-infarcted region where most implanted cells die in the first day after transplantation, limiting the potential effect of this therapy [3]. Understanding the importance of the tissue microenvironment and how it may be manipulated is critical to optimize the potential therapeutic effect of MSC [4, 5]. Interestingly, stem cells are located in bone marrow niches where the level of oxygen concentration is low [6] and as such, creating a hypoxic environment to mimic these stem cell niches [5, 7, 8], might be of interest. Oxygen plays a significant role in the control and regulation of many signalling pathways involved in cellular engraftment and host-tissue regeneration [5, 9, 10]. Oxygen tension in bone marrow compartments has been reported to be very low, as in injured tissue, suggesting that tissue hypoxia may be a fundamental mechanism governing stem cell recruitment and retention.
Our group has recently shown that hypoxic preconditioning of MSC before transplantation in a mouse model of hindlimb ischaemia improved vascular and skeletal muscle regeneration, implicating the Wnt/Frizzled pathway [11] in this process.
We demonstrated that hypoxic pretreatment could more effectively overcome the hurdle of cell death after implantation, than normoxic conditioning, promoting MSC survival and proliferation in situ.
In view of these findings, we developed a preclinical swine model using a non-necrosis model of myocardial ischaemia with the aim of our study being to compare the ability of hypoxic-preconditioned human MSC (Hyp-MSC) and non-preconditioned normoxic MSC (MSC) to engraft into ischaemic myocardial tissue and to assess the ability of these cells to participate in angiogenesis and cardiac regeneration. To mimic the conditions of clinical practice, we focused our evaluation utilizing tools frequently used in the clinic, such as 2-dimensional (2D) strain echocardiography (global and segmental deformation) and haemodynamic criteria, to investigate the potential effect of hypoxic preconditioning.
MATERIALS AND METHODS
This study was conducted in accordance with both institutional guidelines and those in force in the European community for experimental animal use (L358–86/609/EEC).
Design of study
Details of the design are illustrated in Fig. 1.
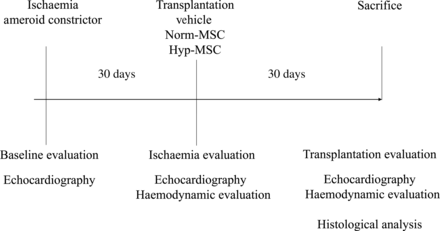
Mesenchymal stromal cells preparation
Human bone marrow MSC were isolated by culturing bone marrow from healthy donors obtained from filters used during the preparation of allogeneic haematopoietic grafts as previously described [12]. Bone marrow cells were cultured in ‘complete medium’ consisting of minimum essential medium, alpha modification (α-MEM; Lonza, Levallois Perret, France) supplemented with 10% foetal calf serum (Perbio Hyclone, France), glutamine (2 mmol/l; Lonza), streptomycin–penicillin (100 g/ml; Sigma-Aldrich, Saint Quentin Fallavier, France) and basic fibroblast growth factor (1 ng/ml; Peprotech, Neuilly sur Seine, France). Cultures were fed every 3–4 days until they reached confluence (∼10–12 days). Adherent cells were then trypsinized (Lonza), harvested and cultured at a concentration of 2 × 103/cm2 for 1 week. Final MSC conditioning was performed after cells were thawed, washed and resuspended in complete medium. Viable cell counting was performed 1 h after resuspension in medium by the trypan blue technique.
Conditioning of cells with respect to O2 concentration
1500 viable cells/cm2 (P2) were deposited in 500 cm2 triple flasks (Nunc, Brumath, France). Each flask compartment (floor) was seeded with 30 ml of cell suspension. Cells from the same initial sample were cultured for 7 days under two different conditions:
Atmospheric O2 concentration (‘normoxic’ 20%), 5% CO2, at 37°C in water-saturated atmosphere (Incubator Stericult 200, Thermo Scientific or IGO 150 Cell-life Jouan).
Low O2 concentration (‘hypoxia’ 1.5%), 5% CO2 at 37°C in water-saturated atmosphere (Proox Culture Chamber, Model C174 with the O2 regulator Pro-ox 110 and CO2 regulator Pro-CO2, Biospherix, Redfield, NY, USA).
After 7 days, cells were trypsinized and washed. In parallel, cells were analysed for viability (trypan blue exclusion) and CD45, CD73 and CD90 expression (Beckton-Dickinson, Le Pont de Claix, France). Sixty million viable cells were prepared in 24 ml of phosphate buffer serum for each sample and delivered to the surgeon to inject 1 × 106/kg per pig. The MSC were kept at room temperature and atmospheric pressure and injected within 1–2 h of preparation (with a necessary short period of reoxygenation).
Chronic myocardial ischaemia: ameroid constrictor placement
After anaesthesia, the proximal circumflex coronary artery was exposed after thoracotomy and the ameroid constrictor placed around it (3.5 mm, Research Instruments, Lebanon, Oregon, USA), just above the first lateral branch as previously described [13]. At 1 month, the stenosis observed on the circumflex branch was approximately 90%, with similar results observed at 2 months (time of transplantation). In general, the flow was scored at thrombolysis in myocardial infarction 2. As described, no demonstration of MI was found with this model [13]. The progressive constriction depends on three factors: absorption of water and slow swelling obstructing the vessel inside the lumen of the constrictor, growth of the coronary artery under a fixed metal sheath and inflammatory response in the site of implantation.
Study treatments
Thirty days after the ameroid constrictor placement, pigs were randomly assigned to one of three treatment groups prior to undergoing rethoracotomy and cell/saline injection into the ischaemic inferior-lateral zone. The sham (control) treatment group was injected with saline (no cells), the MSC group with 1 × 106/kg of MSC and the Hyp-MSC group with 1 × 106/kg of Hyp-MSC.
Injections were made with a 25 Gauge needle in 25 sites representing a total of 25 ml. All pigs that survived sham, MSC or Hyp-MSC treatment for 30 days were analysed and sacrificed. No immunosuppressive therapy was given to animals in this study.
Evaluation by echocardiography
All evaluations (A Vivid 7, GE Medical Systems, Horten, Norway) were performed at rest, by a trained echographist (J.J. and P.R.) blinded to the treatment group [13].
To assess myocardial function, a conventional echocardiogram of the ejection fraction was performed. Briefly, the Simpson's formula was used (TeleDiastolic Volume—TeleSystolic Volume/TeleDiastolic Volume) and results expressed in percentage.
Diastolic function was evaluated by the analysis of mitral Doppler inflow (E wave) and pulsed wave (e′) by tissue Doppler imaging at the lateral mitral annulus (E/e′ ratio).
Tools for quantifying 2D strain (speckle tracking) enabled us, through the recording of conventional black and white images in the various zones, to evaluate parameters of myocardial deformation. These deformations, depending on the orientation of acquisitions, were expressed in the form of circumferential, longitudinal and radial components closest to the orientation of the myocardial fibres. These components were exploited at rest as previously described [14]. The data were stored and transferred to a computer for post-processing analyses. The recordings were analysed using Echopac software (GE Medical Systems), by a trained echographist (S.L. and P.R.) blinded to the treatment group [13].
Haemodynamic analysis
Haemodynamic analyses were performed 1 month after constrictor placement, prior to transplantation, and 1 month after transplantation in non-blinded conditions. The right carotid artery and the right jugular vein were used for cardiac catheterization in a closed chest preparation. To evaluate the right-side pressures and to determine the cardiac output, a Swan-Ganz CCOmbo pulmonary artery catheter (Edwards Lifesciences, Irvine, USA) was inserted. The left ventricle (LV) pressures were recorded using a Millar probe (Millar Instruments, Houston, USA) [15]. After 10 min of stabilization, the heart rate, central venous pressure, cardiac output, pulmonary pressure, pulmonary arterial occluded pressure, left ventricle end-diastolic and end-systolic pressures, dP/dT max and min, and TauW were analysed and recorded using appropriate software (IOX, Emka technology, Falls Church, USA).
Histological analysis
After sacrifice, tissues from the lateral ischaemic zone, and healthy zones, were harvested, fixed in methanol, embedded in paraffin or cryopreserved, and 7 µm slides were cut and stained with Masson's Trichrome. The analysis of inflammatory reaction was carried out by labelling with myeloperoxydase (1/1000, Dako), CD45 (1/500, Serotec) and CD3 (1/100, Serotec). Capillary density was performed by immunostaining of endothelial cells (EC) with von Willebrandt factor (vWF; 1/100, Sigma). MSC and Hyp-MSC were both detected with immunostaining using an anti-human nuclei monoclonal antibody (1/100, Chemicon). The slides were photographed with a charge-coupled device camera (Nikon Microphot-FXA, France) connected to an IBM personal computer (Dell, USA). A minimum of 30 random digital photographs was taken at ×20 magnification for each specimen analysed. Positively stained cells were manually counted by a blinded observer (TC-CD), with the help of Sigma Scan Plot software.
The number of transplanted cells, capillary density and inflammatory cells per square millimetre were determined and recorded.
Statistical analysis
Results are expressed in mean ± standard deviation. All the analyses were carried out using relevant software (Statview 5-1, SAS institute Inc.). Comparisons of the continuous variables between the 2 groups were carried out using analysis of variance. If a statistical difference was found, a t-test was carried out. A value of P < 0 0.05 was considered to be statistically significant.
RESULTS
Mortality
Twenty-seven pigs underwent surgery, and two died during the constrictor placement procedure (one from a tear in the left atrium, one from ventricular fibrillation). This overall mortality rate of 33.3% is in accordance with a previously reported study [13].
Twenty-five pigs were randomly assigned in the three groups: 6 for sham, 10 for MSC and 9 for Hyp-MSC. Prior to intramyocardial saline/cell injection, seven animals died (two randomized to the sham group, two in the MSC group, three in the Hyp-MSC group) from congestive heart failure or cardiac rhythm disorder. A total of 18 animals underwent intramyocardial saline/cell injections without complications and were available for full final assessment: four animals in the sham group, eight in the MSC group and six in the Hyp-MSC group.
Hyp-MSC improves global systolic and diastolic function when compared with normoxic MSC: conventional echocardiographic assessment
As shown in Fig. 2, no differences were observed between the three groups at baseline with regard to left ventricle ejection fraction (LVEF), Telediastolic and Telesystolic LV volume, and diastolic function E/e′ ratio.
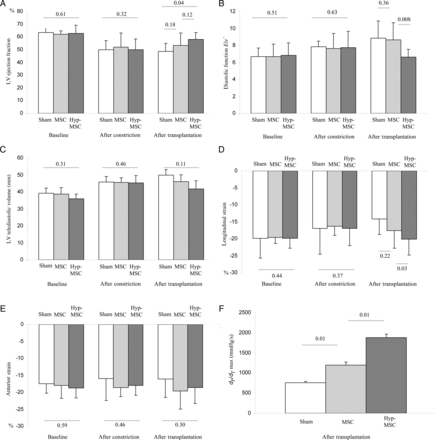
Global echocardiography analysis, regional deformation 2D strain and haemodynamic analysis. (A) LV ejection fraction: no differences were observed between the three groups at baseline and 30 days after constrictor placement. In contrast, 30 days after transplantation, LVEF significantly improved in the Hyp-MSC group. (B) Diastolic function: as observed with the systolic function, diastolic function, E/e′ ratio was less altered in the Hyp-MSc group. (C) Remodelling: no differences were observed in the three groups in terms of LV TeleDiastolic volume. (D) LS in the ischaemic inferior-lateral zone showed a significant improvement in the Hyp-MSC group compared with the other two groups. (E) Anterior strain did not show any difference in the healthy zone in any of the three groups. (F) The LV was catheterized with a Millar's probe. dP/dT showed significant improvement 30 days after transplantation in the Hyp-MSC group when compared with the other two groups.
One month after constrictor placement, LVEF was significantly reduced, remodelling markers as well as Telediastolic and Telesystolic LV volume were increased and diastolic function was altered in each group compared with their baseline evaluation (Fig. 2) as already described [13]. There was no statistical difference between the three groups prior to cell transplantation.
One month after transplantation, LVEF was stable in the sham and in the MSC groups, but was significantly improved in the Hyp-MSC group (Fig. 2A). Similar results were found for the diastolic function in which the E/e′ ratio was less altered in the Hyp-MSC group compared with the two other groups (Fig. 2B). However, no difference in TeleDiastolic and TeleSystolic LV volume (Fig. 2C) was found. In conclusion, in contrast to MSC alone, MSC preconditioned by hypoxia improved both global systolic and diastolic parameters after transplantation.
Hyp-MSC improves regional cardiac deformation when compared with normoxic MSC: 2D strain echocardiography assessment
At baseline, no statistical difference was found in the peak systolic longitudinal strain (LS), or in the inferior-lateral or anterior walls between the three groups (Figs 2D and E).
One month after constrictor placement, in the ischaemic zone (inferior-lateral), LS was significantly reduced in each group compared with baseline as already described [13], but there was no statistical difference between the three groups (Fig. 2D). Peak systolic measurement of the LS in the anterior wall, used as a control marker, was unchanged compared with baseline evaluation (Fig. 2E).
One month after transplantation, a significant improvement, in the ischaemic area, was noted in the LS in the Hyp-MSC group compared with the two other groups (Fig. 2D). The control anterior strain was not modified from baseline (Fig. 2E). In conclusion, MSC preconditioned by hypoxia improved regional cardiac deformation in the ischaemic zone.
Hyp-MSC reduces LV pressures and increases LV contractility indexes when compared with normoxic MSC: haemodynamic assessment
Cardiac catheterization evaluating cardiac output, LV pressures, dP/dT max and min, right-side pressures did not show any difference between the three groups at baseline, or 1 month after constrictor placement (data not shown). As shown in Fig. 2F and in Table 1, 1 month after stem cell transplantation, the MSC and Hyp-MSC groups revealed a significant improvement in haemodynamic parameters. Moreover, results were amplified in Hyp-MSC compared with the two other groups. These results strongly support the notion that preconditioning MSC with hypoxia improves haemodynamic parameters after transplantation.
. | Sham . | MSC . | Hyp-MSC . |
---|---|---|---|
Heart rate (per min) | 77 ± 17 | 81 ± 15 | 82 ± 4 |
Systolic blood pressure (mmHg) | 71 ± 2 | 105 ± 4 | 116 ± 6 |
Venous central pressure (VCP, mmHg) | 17 ± 3 | 9 ± 1 | 7 ± 0* |
Pulmonary arterial occluded pressure (PAOP, mmHg) | 22 ± 2 | 12 ± 3 | 8 ± 0* |
Cardiac output (l/min) | 2 ± 0 | 3 ± 0 | 3 ± 0* |
End-diastolic pressure (mmHg) | 7 ± 2 | 6 ± 2 | 3 ± 2* |
End-systolic pressure (mmHg) | 63 ± 2 | 98 ± 4 | 107 ± 5* |
TauW (m/s) | 26 ± 2 | 32 ± 3 | 47 ± 3* |
. | Sham . | MSC . | Hyp-MSC . |
---|---|---|---|
Heart rate (per min) | 77 ± 17 | 81 ± 15 | 82 ± 4 |
Systolic blood pressure (mmHg) | 71 ± 2 | 105 ± 4 | 116 ± 6 |
Venous central pressure (VCP, mmHg) | 17 ± 3 | 9 ± 1 | 7 ± 0* |
Pulmonary arterial occluded pressure (PAOP, mmHg) | 22 ± 2 | 12 ± 3 | 8 ± 0* |
Cardiac output (l/min) | 2 ± 0 | 3 ± 0 | 3 ± 0* |
End-diastolic pressure (mmHg) | 7 ± 2 | 6 ± 2 | 3 ± 2* |
End-systolic pressure (mmHg) | 63 ± 2 | 98 ± 4 | 107 ± 5* |
TauW (m/s) | 26 ± 2 | 32 ± 3 | 47 ± 3* |
Values are mean ± standard deviation.
*Means P < 0.01 compared with the MSC group.
. | Sham . | MSC . | Hyp-MSC . |
---|---|---|---|
Heart rate (per min) | 77 ± 17 | 81 ± 15 | 82 ± 4 |
Systolic blood pressure (mmHg) | 71 ± 2 | 105 ± 4 | 116 ± 6 |
Venous central pressure (VCP, mmHg) | 17 ± 3 | 9 ± 1 | 7 ± 0* |
Pulmonary arterial occluded pressure (PAOP, mmHg) | 22 ± 2 | 12 ± 3 | 8 ± 0* |
Cardiac output (l/min) | 2 ± 0 | 3 ± 0 | 3 ± 0* |
End-diastolic pressure (mmHg) | 7 ± 2 | 6 ± 2 | 3 ± 2* |
End-systolic pressure (mmHg) | 63 ± 2 | 98 ± 4 | 107 ± 5* |
TauW (m/s) | 26 ± 2 | 32 ± 3 | 47 ± 3* |
. | Sham . | MSC . | Hyp-MSC . |
---|---|---|---|
Heart rate (per min) | 77 ± 17 | 81 ± 15 | 82 ± 4 |
Systolic blood pressure (mmHg) | 71 ± 2 | 105 ± 4 | 116 ± 6 |
Venous central pressure (VCP, mmHg) | 17 ± 3 | 9 ± 1 | 7 ± 0* |
Pulmonary arterial occluded pressure (PAOP, mmHg) | 22 ± 2 | 12 ± 3 | 8 ± 0* |
Cardiac output (l/min) | 2 ± 0 | 3 ± 0 | 3 ± 0* |
End-diastolic pressure (mmHg) | 7 ± 2 | 6 ± 2 | 3 ± 2* |
End-systolic pressure (mmHg) | 63 ± 2 | 98 ± 4 | 107 ± 5* |
TauW (m/s) | 26 ± 2 | 32 ± 3 | 47 ± 3* |
Values are mean ± standard deviation.
*Means P < 0.01 compared with the MSC group.
Hyp-MSC increases capillary density in the ischaemic zone when compared with normoxic MSC
There was no fibrosis noted in histological analyses of the ischaemic inferior-lateral region, demonstrating the absence of necrosis and infarction in this model. As shown in Figs 3A and 4, the capillary density (as demonstrated by vWF staining) was significantly improved in the MSC group. Interestingly, these results were amplified in the MSC-Hyp group. No significant difference was observed in capillary density in the healthy anterior zone.
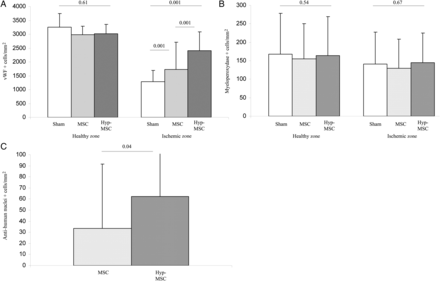
Histological analysis. (A) Thirty days after transplantation, pigs were sacrificed and hearts were harvested. Capillary density was analysed in the healthy and ischaemic zones with vWF immunostaining. Compared with the sham-treated group, there was a significant increase in vascular density after MSC transplantation, and results were further amplified in the Hyp-MSC group. (B) Analysis of the inflammatory response demonstrated that no indication of an immune rejection and no differences were observed between the three groups in the healthy or ischaemic zones after myeloperoxydase staining. (C) MSC homing in the ischaemic zone. After specific anti-nuclei human antibody, the presence of transplanted MSC was evaluated in the ischaemic zone. The number of transplanted cells detected at 30 days post-transplantation was significantly increased in the Hyp-MSC group compared with the MSC group.
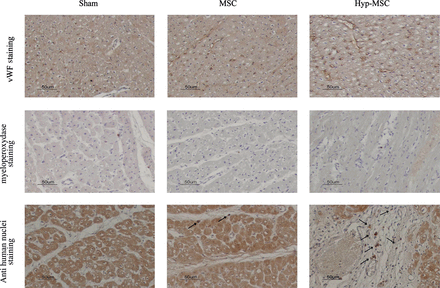
Histological analysis. Endothelial cells (vWF), inflammatory cells (myeloperoxydase) and MSC (human anti-nuclei antibody) staining. Arrows indicate viable transplanted MSC in the ischaemic zone.
No differences were detected in the inflammatory response (Fig. 3B). In conclusion, MSC improved capillary density, and these results were amplified when MSC were preconditioned by hypoxia.
Hypoxia preconditioning improves MSC survival and homing in the ischaemic zone
As illustrated in Figs 3C and 4, detection of MSC in the ischaemic zone by the anti-human nuclei monoclonal antibody staining 1 month after transplantation showed that the number of stained cells was significantly higher in the Hyp-MSC group compared with the MSC group. We were not able to demonstrate any trans-differentiation of MSC or Hyp-MSC into EC or cardiomyocytes. As expected, no MSC were detected in the sham group (Fig. 4).
DISCUSSION
Study objective
In the present work, we investigate the ability of Hyp-MSC, preconditioned in hypoxic conditions, to enhance angiogenesis when compared with non-preconditioned MSC in a preclinical swine model of chronic myocardial ischaemia. The goal of the current study was to focus on a new way to prepare cells (hypoxia preconditioning) before transplantation. The strategy was based on an improvement in capillary density and supported by previous mechanistic demonstration of the potential effect of Hyp-MSC [11]. Although practically identical to their counterparts preconditioned at 20% O2 with respect to the immunophenotype and the colony forming unit-F content, upon transplantation, the hypoxic-preconditioned allogeneic MSC (Hyp-MSC) increased capillary density, as well as global and segmental cardiac function, measured by 2D strain analysis and improved haemodynamic parameters. These observed effects are most likely associated with an increase in Hyp-MSC engraftment without demonstration of significant immune rejection.
Background and Wnt/Frizzled pathway implications
Our group has previously shown that MSC preconditioned with hypoxia are able to participate in vascular and muscular regeneration in an in vivo model of hindlimb ischaemia [11] and have a greater capacity for engraftment into ischaemic tissue compared with normoxic MSC. We further demonstrated that Hyp-MSC participate in remodelling of the stromal environment via structural interactions, first in encircling necrotic myocytes and then shaping a tight network around EC along growing neovessels. The distribution of hyp-MSC around necrotic myocytes suggested a potential macrophage-like, phagocytic function for these cells, which was demonstrated in vitro with coculture of necrotic or non-necrotic cell bodies of fluorescent cell line-labelled murine myoblasts [11]. Our data showed that Hyp-MSC expressed Wnt 4, and in vivo Wnt 4 transcripts were significantly increased in ischaemic muscles engrafted with Hyp-MSC. Wnt 4 in vitro stimulated EC migration, myogenic differentiation and MSC proliferation, but had no effect on EC proliferation. Importantly, the inhibition of Wnt 4 expression in MSC abrogated Hyp-MSC-induced vascular regeneration. These data provided strong evidence that Wnt signalling could be an important factor in the mechanism by which Hyp-MSC contribute to tissue regeneration, as well as engraftment, cell survival, cell proliferation, vessel formation and stabilization [11]. Hyp-MSC appeared to have a different pattern of engraftment when compared with normoxic MSC in ischaemic muscles and were evenly distributed in deep hypoxic zones around necrotic myocytes, whereas normoxic MSC were consistently detected at the periphery of ischaemic zones.
Potential role of low concentration in oxygen
Oxygen concentration in bone marrow compartments (niches) has been reported to be very low, as in injured tissue, suggesting that tissue hypoxia may be a fundamental mechanism governing stem cell recruitment and retention [5, 16]. Some studies have demonstrated a role of hypoxic gradients in stem cell trafficking in ischaemic tissue [16]. Whereas very low oxygen concentrations are involved in holding stem cells in a quiescent state in which they retain their plasticity [17], O2 concentrations between 1 and 3% oxygen are related to the maintenance of stem cells and/or directing their lineage commitment (most probably by an ‘asymmetrical’ mode of division) [18, 19]. Previously published data indicate that hypoxic (Hyp) preconditioning enhances the capacity of MSC to reduce cell death and apoptosis of implanted cells [20] and to increase gene expression of prosurvival and proangiogenic factors [21]. Our group has previously demonstrated that the 1.5% oxygen atmosphere, in which MSC were maintained, can condition them to better survive in a hypoxic environment as reported for in vivo [11] and in vitro [22] conditions. Hu et al. [23] found similar results in an in vivo model of myocardial ischaemia in mice: preconditioning of MSC by hypoxia increased the expression of prosurvival and proangiogenic factors (hypoxia inductible factor-1α, vascular endothelial growth factor and Angiopoietin) and decreased proapoptotic gene expression (bcl-2 and bcl-xL). This is in line with the results of Uemura et al. [24] showing that PI3K/Akt pathway activation reduced apoptosis and increased angiogenesis.
Translational objective and application
The majority of patients with severe ischaemic cardiomyopathy are not those with a large MI scar and severe LV dysfunction. Patients often present, after undergoing multiple percutaneous coronary intervention or coronary artery by-pass graft [25], with refractory angina, for which there is no adequate treatment. The development of new treatments to improve myocardial perfusion (angiogenesis) is required to reduce the symptomatology of patients [8]. To better translate the data from this study to a clinical setting, we utilized a previously characterized model of myocardial ischaemia in which there is progressive stenosis of the circumflex artery and a permanent regional inferior-lateral ischaemia without extensive necrosis. Most experimental studies in stem cell therapy have evaluated the improvement in LV function with conventional echocardiography, which is not sensitive enough to detect the subtle changes seen in non-necrotic ischaemic cardiomyopathy. The potential of the 2D strain in detecting ischaemia was clearly demonstrated, where abnormalities of deformation were particularly pronounced in the LS. To correlate the echocardiographic data with the extent of ischaemia, we previously demonstrated that abnormalities of 2D strain are associated with an important alteration in tissue perfusion after injection of microbubbles [13]. Moreover, these functional data are correlated with tissue histology, demonstrating a significant reduction in capillary density in the ischaemic zone. Moreover, we utilized a method of evaluation (segmental deformation) that is appropriate in a clinical approach, as it can be observed in severely ischaemic patients.
Limitation of the study
This is a descriptive study based on a recent published paper [11] in which we demonstrated the potential effect of the Hyp-MSC on vascular and muscular regeneration after ischaemia. Each group of animals is small and the relatively small sample size is still a major concern regarding our results. LVEF and 2D strain have a relatively high interobserver variability despite trained echocardiographists [13]. The therapeutic approach of the Hyp-MSC is not fully understood, and further studies are necessary and expected to place the cellular cardiomyoplasty as a standard therapy.
Conclusion
The present study suggests that implantation of MSC after 7 days of hypoxic preconditioning (1.5% O2) into chronically ischaemic myocardium is safe and effectively induces improvement in global and regional function assessed by speckle tracking technology, haemodynamic parameters, vascular density and cells survival [2, 11]. To our knowledge, this is the first report evaluating the potential effect of Hyp-MSC in a chronic phase of myocardial ischaemia, which could represent an important step to advance further preclinical evaluation. Using specific tools for ischaemia detection from a perspective of clinical practice, our results provide strong evidence that this strategy could be an attractive therapeutic approach for the treatment of ischaemic heart disease.
Conflict of interest: none declared.