-
PDF
- Split View
-
Views
-
Cite
Cite
Alessio Rungatscher, Alberto Merlini, Fabrizio De Rita, Gianluca Lucchese, Luca Barozzi, Giuseppe Faggian, Alessandro Mazzucco, Giovanni Battista Luciani, Diagnosis of infection in paediatric veno-arterial cardiac extracorporeal membrane oxygenation: role of procalcitonin and C-reactive protein, European Journal of Cardio-Thoracic Surgery, Volume 43, Issue 5, May 2013, Pages 1043–1049, https://doi.org/10.1093/ejcts/ezs524
- Share Icon Share
Abstract
Plasma concentration of procalcitonin (PCT) and its value in the diagnosis of infection in paediatric patients treated with extracorporeal membrane oxygenation (ECMO) are undefined. This study aimed to define the levels of PCT and C-reactive protein (CRP) in paediatric cardiac ECMO patients and to determine their role in predicting infection, severity of organ dysfunction and clinical outcome.
PCT and CRP plasma concentrations were measured daily in 20 consecutive infants and young children treated with veno-arterial ECMO. Each patient was examined daily for signs of infection and multiple organ dysfunction syndrome (MODS). A total of 139 patient days were classified for infection and MODS.
The median PCT and CRP plasma concentrations were not increased during infection: 2.4 vs 8.8 ng/ml and 223.8 vs 240.6 mg/l, in patients with vs without infection, respectively. PCT, but not CRP, was significantly elevated during MODS (10.9 vs 1.85 ng/ml) (P = 0.001). The area under the receiver operating characteristic (ROC) curve was 0.984 for PCT (95% confidence interval [CI], 0.962–1.000) compared with 0.347 for CRP (95% CI, 0.211–0.484) (P = 0.001). Only PCT differed significantly in patients weaned from ECMO who survived (2.6 ng/ml) vs patients not weaned from ECMO (10.5 ng/ml) (P = 0.001). The area under the ROC curve was 0.871 (95% CI, 0.786–0.956) compared with 0.261 for CRP (95% CI, 0.145–0.377) (P = 0.001).
Neither PCT nor CRP are reliable markers of infection in paediatric cardiac ECMO patients. However, high levels of PCT are associated with MODS. PCT may be used as a prognostic indicator of clinical outcome in this high-risk population.
INTRODUCTION
Extracorporeal membrane oxygenation (ECMO) is an established method of support for refractory cardiac and pulmonary failure in neonates and children [1]. Children who undergo surgery for congenital heart defects in the neonatal period or during infancy may require support with ECMO [2]. Despite its efficacy in many clinical scenarios, ECMO carries significant associated risks, including infections. Recently, the Extracorporeal Life Support Organisation reported a prevalence of acquired infections during ECMO of 17.4 and 22.8% in neonatal and paediatric patients, respectively [3]. Infections have significant consequences in the ECMO patient population, including a prolonged need for bypass support, an increased risk of mechanical and patient-related complications, a delay in post-ECMO lung recovery and an increased mortality [4].
The systemic inflammatory response syndrome constitutes a common finding in ECMO patients, making diagnosis of infection troublesome. The prolonged contact between blood and extracorporeal circuit induces activation of the inflammatory cascade as well as production of cytokines (including tumour necrosis factor alpha, TNF-α), which trigger complex humoral and cellular inflammatory responses. The latter leads to activation of the complement system and leukocytes, which reproduces, to a minor extent, the mechanisms found in sepsis [5].
Sensitive and specific markers enabling the differentiation between these two entities would be desirable and may have an impact on the therapeutic decision-making process.
Among several inflammatory markers, C-reactive protein (CRP) is the most often used to diagnose sepsis in clinical practice. However, CRP measurements appear far from ideal for this purpose, as a marked rise has been described in the post-operative setting, some non-infectious inflammatory conditions, and with several viral infections [6].
In the last decade, procalcitonin (PCT) has acquired an important role in the diagnosis of bacterial infection both in the paediatric and in the adult population [7]. A previous report from this institution showed that PCT plasma concentrations in an adult intensive care unit setting allow the differentiation of sepsis from non-infectious causes of SIRS and are very closely related to the severity of infection [8].
However, PCT diagnostic and prognostic utility still remains an issue of controversy. In addition, patient characteristics and clinical settings vary markedly, making the data difficult to interpret and extrapolation often inappropriate to clinical application [9]. A particular cause of misinterpretation of PCT results has been the PCT plasmatic levels after cardiopulmonary bypass. Even though different hourly cut-off values have been proposed for patients after cardiac surgery, the diagnostic relevance of PCT is still debated [10, 11].
Moreover, PCT plasmatic levels during paediatric ECMO treatment have not yet been established and may vary significantly depending on the prolonged inflammatory activation.
The aim of the present study was to define PCT and CRP levels and their relative roles in the diagnosis of infection, severity of organ dysfunction and outcome in paediatric cardiac ECMO patients.
MATERIALS AND METHODS
Setting and study population
Between 2006 and 2011, 20 consecutive paediatric patients assisted with veno-arterial ECMO for cardiac indication longer than 48 h in our Unit were prospectively included in the study. Approval by the Ethics Committee and informed consent were waived due to the observational nature of the study. In addition, levels of PCT and CRP were obtained as part of routine clinical care.
Hospital records of all children were examined for demographic, clinical, laboratory and perfusion variables. The ECMO circuit consisted of a PediVas blood pump (Levitronix LLC, Waltham, MA), D 902 Lilliput II ECMO oxygenator (Sorin Group, Mirandola, MO, Italy) and biocompatible circuit (A.g.i.l.e. Eurosets, Medolla, MO, Italy). Pump flow was maintained between 125 and 150 ml/kg/min and anticoagulation was managed by IV heparin infusion with target activated coagulation time between 170 and 200 s.
During ECMO support, PCT and CRP plasma concentrations were recorded daily and the first sample was performed within the first 24 h. TNF-α plasma concentration was determined daily in a subset of patients.
Diagnosis of infection and multiple organ dysfunction syndrome
Diagnosis of infection followed the criteria of the Centre for Disease Control and Prevention for specific type of infections in the acute care setting [12]. Daily blood, urine and tracheal aspirate samples were sent for culture. In addition, cultures of surgical wounds and removed catheters were systematically performed.
A Sequential Organ Failure Assessment (SOFA) [13] score was recorded daily in all patients. Considering all the patients in the study presented cardiac failure by definition and respiratory dysfunction secondary to cardiac disease and/or extracorporeal life support, multiple organ dysfunction syndrome (MODS) was arbitrarily defined as an SOFA score >9. The absence of MODS was identified with no organ dysfunction, other than heart and lungs (SOFA score <9).
Laboratory analysis
CRP was measured by direct immunoturbidimetry using the rabbit C-reactive protein method and dimension analyser (Dade Behring, Newark, DE). PCT was measured using a fully automated chemiluminescence analyser (Liaison, Byk Sangtec Diagnostica, Dietzenbach, Germany) and reagents provided by the same firm (Liaison Brahms PCT). In this immunoluminometric assay, based on the sandwich principle, two different specific monoclonal antibodies are used for the coating of the solid phase and for the tracer. After two incubations of 10 min each, the excess tracer is removed by a wash cycle, and the starter reagents are added to induce a chemiluminescence reaction that yields light that is proportional to the PCT concentration. TNF-α plasma concentration was also measured using conventional double-sandwich ELISA kits (Invitrogen) and correlated to the corresponding PCT value obtained from the same sample on the same day.
Statistical analysis
Statistical analysis was performed using Stata version 7.0 statistical software (Stata, College Station, TX). In order to compare PCT and CRP as markers of infection plasma levels in patient days with documented infection and in patient days without infection were analysed, a method previously published by our group [10]. To describe the relationship of PCT and CRP with MODS, the SOFA score was used. Finally, in order to evaluate PCT and CRP as early prognostic indicators of clinical outcome, the two markers were compared using receiver operating characteristic (ROC) curves and the respective areas under the curve. A comparison was performed using the Mann–Whitney U test. The Kruskal–Wallis rank sum was used for intergroup analyses and testing differences between the groups at different time points. Correlation between the numerical values was evaluated by using Spearman's rank correlation coefficient (r). A P value of <0.05 was considered to be significant.
RESULTS
Clinical outcome
Overall preoperative data, mechanical support data and outcomes are reported in Table 1. Eighteen (90%) patients were infants in the first year of life, 10 (50%) among them were neonates. Of note, the median weight was 3.3 kg, with 8/10 newborn weighing <2.5 kg. The indication for ECMO support was mainly post-cardiotomy shock (80%). Cannulation was central in 17 (85%) patients and peripheral in 3. The mean time for ECMO was 5.7 + 4.1 days (range 2–16). Weaning from ECMO was possible in 55% of patients and the survival-to-discharge rate was 35%. Overall morbidity was observed in 14 patients (70%): there were eight cases of renal failure requiring dialysis, six bleeding events needing surgical revision, five cases of sepsis and two neurological events consisting of intracranial haemorrhage. A total of 130 patient days were classified for infection and MODS.
. | N = 20 . | Mean + SD . | Median . | Range . |
---|---|---|---|---|
Patient characteristics | ||||
Female | 8 (40%) | |||
Male | 12 (60%) | |||
Age (months) | ||||
Neonate/infant <12 months | 18 (90%) | 7.8 ± 18.0 | 0.9 | 0.2–63 |
Weight (kg) | 5.6 ± 5.2 | 3.3 | 2.3–20 | |
ECMO indication | ||||
Post-cardiotomy | 16 (80%) | |||
Single ventricle stage I repair | 9 | |||
Complex LVOTO repair | 2 | |||
Truncus repair | 2 | |||
dTGA + intramural LMCA | 1 | |||
cTGA physiologic repair | 1 | |||
Recurrent PV stenosis s/p TAPVC | 1 | |||
Fulminant myocarditis | 2 (10%) | |||
Malignant SVT | 1 (5%) | |||
Fulminant haemophagocitosis syndrome | 1 (5%) | |||
ECMO setup | ||||
Weaning from CPB | 5 (25%) | |||
PICU initiation | 15 (75%) | |||
Duration (days) | 5.7 ± 4.1 | 5 | 2–16 | |
Central/peripheral cannulation | 17/3 | |||
Outcome | ||||
Weaning rate | 11 (55%) | |||
Hospital survival rate | 7 (35%) | |||
Overall morbidity | 14 (70%) | |||
Renal | 8 (42%) | |||
Bleeding | 6 (32%) | |||
Sepsis | 6 (30%) | |||
Neurological | 2 (11%) |
. | N = 20 . | Mean + SD . | Median . | Range . |
---|---|---|---|---|
Patient characteristics | ||||
Female | 8 (40%) | |||
Male | 12 (60%) | |||
Age (months) | ||||
Neonate/infant <12 months | 18 (90%) | 7.8 ± 18.0 | 0.9 | 0.2–63 |
Weight (kg) | 5.6 ± 5.2 | 3.3 | 2.3–20 | |
ECMO indication | ||||
Post-cardiotomy | 16 (80%) | |||
Single ventricle stage I repair | 9 | |||
Complex LVOTO repair | 2 | |||
Truncus repair | 2 | |||
dTGA + intramural LMCA | 1 | |||
cTGA physiologic repair | 1 | |||
Recurrent PV stenosis s/p TAPVC | 1 | |||
Fulminant myocarditis | 2 (10%) | |||
Malignant SVT | 1 (5%) | |||
Fulminant haemophagocitosis syndrome | 1 (5%) | |||
ECMO setup | ||||
Weaning from CPB | 5 (25%) | |||
PICU initiation | 15 (75%) | |||
Duration (days) | 5.7 ± 4.1 | 5 | 2–16 | |
Central/peripheral cannulation | 17/3 | |||
Outcome | ||||
Weaning rate | 11 (55%) | |||
Hospital survival rate | 7 (35%) | |||
Overall morbidity | 14 (70%) | |||
Renal | 8 (42%) | |||
Bleeding | 6 (32%) | |||
Sepsis | 6 (30%) | |||
Neurological | 2 (11%) |
ECMO: extracorporeal membrane oxygenation; LVOTO: left ventricular outflow tract; TGA: transposition of the great arteries; LMCA: left main coronary artery; PV: pulmonary veins; TAPVC: total anomalous pulmonary venous connection; SVT: supra-ventricular tachycardia; CPB: cardiopulmonary bypass; PICU: paediatric intensive care unit.
. | N = 20 . | Mean + SD . | Median . | Range . |
---|---|---|---|---|
Patient characteristics | ||||
Female | 8 (40%) | |||
Male | 12 (60%) | |||
Age (months) | ||||
Neonate/infant <12 months | 18 (90%) | 7.8 ± 18.0 | 0.9 | 0.2–63 |
Weight (kg) | 5.6 ± 5.2 | 3.3 | 2.3–20 | |
ECMO indication | ||||
Post-cardiotomy | 16 (80%) | |||
Single ventricle stage I repair | 9 | |||
Complex LVOTO repair | 2 | |||
Truncus repair | 2 | |||
dTGA + intramural LMCA | 1 | |||
cTGA physiologic repair | 1 | |||
Recurrent PV stenosis s/p TAPVC | 1 | |||
Fulminant myocarditis | 2 (10%) | |||
Malignant SVT | 1 (5%) | |||
Fulminant haemophagocitosis syndrome | 1 (5%) | |||
ECMO setup | ||||
Weaning from CPB | 5 (25%) | |||
PICU initiation | 15 (75%) | |||
Duration (days) | 5.7 ± 4.1 | 5 | 2–16 | |
Central/peripheral cannulation | 17/3 | |||
Outcome | ||||
Weaning rate | 11 (55%) | |||
Hospital survival rate | 7 (35%) | |||
Overall morbidity | 14 (70%) | |||
Renal | 8 (42%) | |||
Bleeding | 6 (32%) | |||
Sepsis | 6 (30%) | |||
Neurological | 2 (11%) |
. | N = 20 . | Mean + SD . | Median . | Range . |
---|---|---|---|---|
Patient characteristics | ||||
Female | 8 (40%) | |||
Male | 12 (60%) | |||
Age (months) | ||||
Neonate/infant <12 months | 18 (90%) | 7.8 ± 18.0 | 0.9 | 0.2–63 |
Weight (kg) | 5.6 ± 5.2 | 3.3 | 2.3–20 | |
ECMO indication | ||||
Post-cardiotomy | 16 (80%) | |||
Single ventricle stage I repair | 9 | |||
Complex LVOTO repair | 2 | |||
Truncus repair | 2 | |||
dTGA + intramural LMCA | 1 | |||
cTGA physiologic repair | 1 | |||
Recurrent PV stenosis s/p TAPVC | 1 | |||
Fulminant myocarditis | 2 (10%) | |||
Malignant SVT | 1 (5%) | |||
Fulminant haemophagocitosis syndrome | 1 (5%) | |||
ECMO setup | ||||
Weaning from CPB | 5 (25%) | |||
PICU initiation | 15 (75%) | |||
Duration (days) | 5.7 ± 4.1 | 5 | 2–16 | |
Central/peripheral cannulation | 17/3 | |||
Outcome | ||||
Weaning rate | 11 (55%) | |||
Hospital survival rate | 7 (35%) | |||
Overall morbidity | 14 (70%) | |||
Renal | 8 (42%) | |||
Bleeding | 6 (32%) | |||
Sepsis | 6 (30%) | |||
Neurological | 2 (11%) |
ECMO: extracorporeal membrane oxygenation; LVOTO: left ventricular outflow tract; TGA: transposition of the great arteries; LMCA: left main coronary artery; PV: pulmonary veins; TAPVC: total anomalous pulmonary venous connection; SVT: supra-ventricular tachycardia; CPB: cardiopulmonary bypass; PICU: paediatric intensive care unit.
PCT and CRP as markers of infection
The median PCT plasma concentrations in infected and non-infected patient days were 2.4 and 8.8 ng/ml (P = 0.015), respectively. The median CRP plasma concentrations in infected and non-infected patient days were 223.8 and 240.6 mg/l (P = 0.19). Not only were PCT and CRP levels not increased during infection, but PCT concentrations were paradoxically lower (Fig. 1). On the contrary, PCT levels significantly correlated with TNF-α levels (Fig. 2).
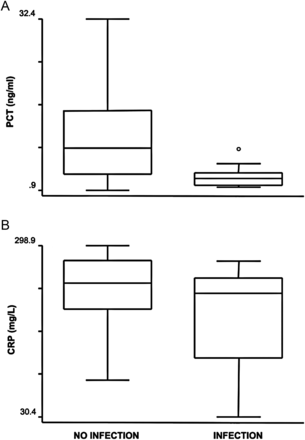
Box plots of plasma concentrations of PCT (A) and CRP (B) in the presence or absence of infection. PCT and CRP levels were not increased during infection and PCT concentrations were significantly lower during infection (P = 0.001).
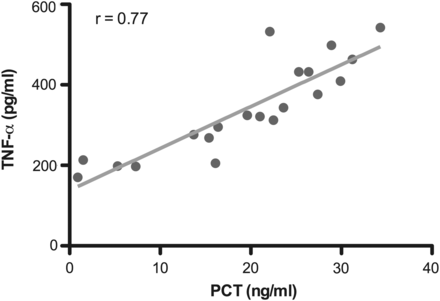
Linear regression analysis demonstrating direct correlation of PCT and TNF-α plasma concentrations (P = 0.001).
Relationship of PCT and CRP with severity of organ dysfunction
The median PCT plasma concentrations in patient days with and without MODS were 10.9 and 1.85 ng/ml (P = 0.001), respectively. Conversely, the median CRP plasma concentration was even lower in patient days with MODS (129.7 vs 234 mg/l), although the difference was not statistically significant (P = 0.09) (Fig. 3).
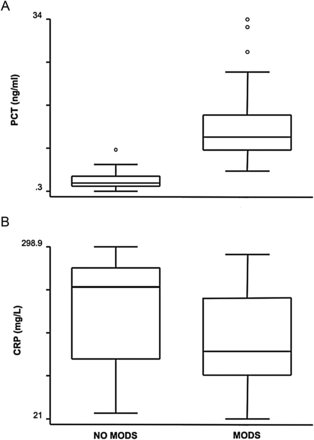
Box plots of plasma concentrations of PCT (A) and CRP (B) in the presence or absence of MODS. CRP levels were not significantly different during MODS than in the absence of it (P = 0.09). PCT levels were higher during MODS than in the absence of it (P = 0.001).
Figure 4 illustrates the sensitivity and specificity of PCT and CRP for the diagnosis of MODS. The area under the ROC curve was 0.984 for PCT (95% confidence interval [CI], 0.962–1.000) compared with 0.347 for CRP (95% CI, 0.211–0.484). The difference was statistically significant (P = 0.001).

ROC curve comparing AUC of PCT (circles) and CRP (linear) for prediction of MODS (P = 0.001).
PCT and CRP as predictors of clinical outcome
PCT plasma concentration differed significantly in patients who were weaned from ECMO and eventually survived to hospital discharge (2.6 ng/ml) vs patients not weaned from ECMO (10.5 ng/ml) (P = 0.001) (Fig. 5). On the contrary, CRP plasma concentrations did not correlate with the gross clinical outcome and were indeed lower in patients with a negative prognosis. In addition, the predictive value of PCT was assessed by following plasma levels over the first 5 days of ECMO support. Accordingly, PCT concentration differed significantly in patients who did not survive after ECMO starting with the second post-implant day (Fig. 6). The area under the ROC curve was 0.871 for PCT (95% CI, 0.786–0.956) compared with 0.261 for CRP (95% CI, 0.145–0.377). The difference was statistically significant (P = 0.001) (Fig. 7).
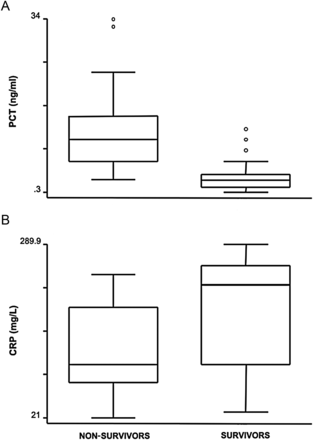
Box plots of plasma concentrations of PCT (A) and CRP (B) in patients subsequently weaned from ECMO compared with patients who were not weaned. CRP levels were not significantly different between the two groups (P = 0.07). PCT levels were significantly higher in the patients with negative prognosis (P = 0.001).
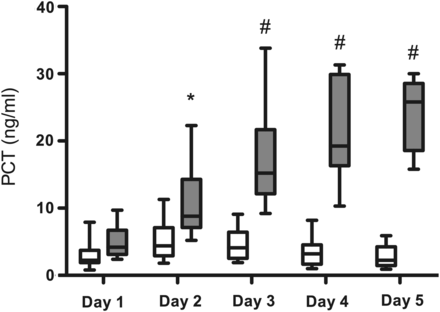
Kinetics of PCT plasma concentration after ECMO institution (day 1) and on the four consecutive days thereafter (days 2–5) in patients who survived (white boxes) or not-survived (gray boxes) after ECMO support. Data are expressed as minimum, maximum, median and interquartile range. Symbols indicate intergroup statistical differences (*P < 0.05; # P < 0.01).
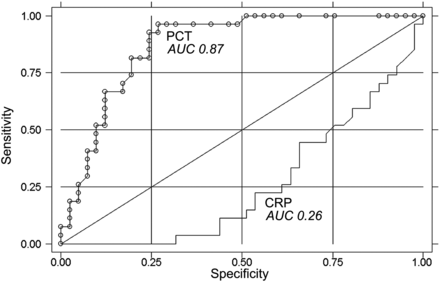
ROC curve comparing the AUC of PCT (circles) and CRP (linear) for prediction of survival (P = 0.001).
DISCUSSION
Early identification of infection has a major impact on the clinical course, management and outcome of paediatric patients supported with ECMO [4]. Mortality for sepsis is high and early therapeutic intervention can improve prognosis [1]. On the other hand, a broad use of antibiotics in all patients with systemic inflammatory response syndrome may lead to the selection of resistant strains with increasing toxicity and costs. ECMO induces a sustained systemic inflammatory response syndrome, which results from a combination of several factors including exposure to non-physiological surfaces, surgical trauma and creation of non-physiological condition, such as the continuous non-pulsatile flow [14]. ECMO-related inflammatory complications are more frequent in younger patients. Neonates and young infants are at greater risk because of the enormous disparity between the patient's circulating blood volume and that of the circuit, often 2- or 3-fold larger. This increases the activation of various blood components upon exposure to the foreign surface of the ECMO circuit [14]. In addition, this circulating volume disparity implies the need for frequent blood transfusion, a therapy that may enhance systemic inflammation.
The clinical similarities make differentiation between systemic inflammatory response syndrome and infectious complications difficult. Early differentiation between systemic inflammatory response syndrome during ECMO support and development of infection is crucial to institute appropriate therapy and to prevent subsequent morbidity. A diagnostic marker that helps us to discriminate between the systemic inflammatory response syndrome produced by ECMO and sepsis would be clinically relevant.
The superiority of PCT compared with CRP in the diagnosis of bacterial infection in children has been reported in several studies during the last 10 years [15, 16]. However, the accuracy of PCT in the diagnosis of infection after cardiopulmonary bypass has not been confirmed and its value in this setting remains controversial [10, 11]. Moreover, high plasma concentrations of PCT after cardiac surgery have already been correlated with complications other than infection [17]. Nevertheless, some authors demonstrated in the same setting, the superiority of PCT when compared with CRP in discriminating infections [18]. Accordingly, the dynamics of PCT levels, rather than the absolute values, could be important in identifying patients with infectious complications after cardiopulmonary bypass [18].
In the present study, PCT and CRP plasma levels were measured daily in paediatric patients supported with veno-arterial ECMO for cardiac indication. To the best of our knowledge, this provides the first ever description of the mean plasma concentration of PCT during paediatric ECMO. The current method was previously applied in a previous study from this Institution on PCT and CRP in a general medical–surgical intensive care unit [8]. Because the aim of this study was to establish the role of serum biomarkers in the diagnosis of infection in patients on ECMO, we studied the day-by-day changes in biomarker values in patients with or without pre-existing infection.
The results herein demonstrate that PCT is not a reliable marker of infection during paediatric ECMO support. Indeed, the PCT median levels were higher than the previously proposed cut-off value [8], independently of the presence of infection. Surprisingly, the median PCT plasma concentrations during days with infection were significantly lower than in days without infection. This apparently paradoxical finding suggests explanations other than infection for a sustained elevation of PCT plasma concentrations.
The current understanding of the inflammatory effects of extracorporeal circulation is primarily based on studies on adult patients treated with cardiopulmonary bypass during cardiac surgery. However, the extrapolation of data from cardiopulmonary bypass to ECMO is difficult because of the major pathophysiological differences between the two techniques. Existing clinical and experimental evidence on the mechanisms of ECMO-related systemic inflammatory response syndrome emphasises the activation of neutrophils, and leukocytes in general, as a pivotal event during ECMO [19, 20]. The activated neutrophils produce cytokines, arachidonic acid metabolites and reactive oxygen species, causing widespread microvascular injury and MODS [19]. The rapid rise in plasma concentrations of TNF-α and IL-8, almost immediately after the initiation of ECMO, suggests the importance of the role of these cytokines in the development of ECMO-related inflammation [20]. A recent experimental study identified mast cell degranulation and the release of performed stores of TNF-α in the intestine as early events in ECMO-related systemic inflammatory response syndrome [14].
Accordingly, one possible explanation for the increase of PCT plasma concentration in the absence of infection, as observed in the present study, could be related to the release of cytokines and, in particular, TNF-α. In fact, the values of PCT and TNF-α, from simultaneous sampling, exhibited a high correlation. Further support to this hypothesis comes from other clinical and experimental settings. Indeed, other authors have reported massively elevated PCT levels in kidney transplant patients with increased serum TNF-α after receiving pan-T-cell antibodies [21] and in patients after isolated limb perfusion using human recombinant TNF-α [22]. Furthermore, production of PCT has been induced in vitro in human liver slices in response to TNF-α [23].
In human neonates, higher plasma TNF-α concentrations have been correlated with the severity of systemic inflammatory response syndrome and have been noted in patients who died during ECMO when compared with survivors [24]. Furthermore, the severity of systemic inflammatory response syndrome during ECMO has been associated with MODS and consequently mortality [24]. Considering the evidence on the production of PCT in response to TNF-α and the role of TNF-α in predicting morbidity during ECMO, the results of the present study are not unexpected. In fact, PCT concentrations were associated with the severity of MODS as assessed by the SOFA score. More importantly, the PCT values demonstrated a close correlation with the clinical outcome, which was not found for CRP, as demonstrated by the sensitivity and specificity of the ROC analysis for severe MODS and mortality. Indeed, the observation that patients successfully weaned from ECMO, and who survived, presented lower PCT concentrations suggests that the severity of systemic inflammatory response syndrome plays a greater role than infection in the elevation of this biomarker. Furthermore, based on the different behaviour of PCT levels in survivors vs nonsurvivors after the first 48 h of support, plasma concentrations >10 ng/ml could be predictive of adverse clinical outcome (Fig. 6). The issue is critical because ECMO is used in children as a bridge to other potential therapeutic options, including organ transplant and long-term assist device implantation. An early sensitive marker of MODS and outcome could aid in critical clinical decisions, particularly when dealing with neonates and infants and their families. A recent report investigated the value of PCT and CRP in the diagnosis of infection in a heterogeneous cohort of adult patients treated with veno-venous or veno-arterial ECMO [25]. In the latter setting, the diagnostic role of PCT when combined with CRP was demonstrated in veno-arterial, but not in veno-venous ECMO. On the contrary, the results in the present study suggest that PCT could be misleading in the diagnosis of infection in paediatric patients treated with veno-arterial ECMO. The possible reason for this discrepancy is that PCT may be a marker of the severity of systemic inflammatory response syndrome rather than infection per se and it is well known that ECMO-related inflammatory complications are more frequent in neonates and young infants than in adults.
The limitations of this study are inherent in the relatively small number of patients and heterogeneity in diagnoses, albeit corrected by the fact of including only paediatric cardiac patients. In addition, PCT and CRP plasma concentrations' sensitivities were assessed daily in all paediatric patients treated with veno-arterial ECMO and not only at distinct time points, as done in most of the studies in the setting of cardiopulmonary bypass [10, 11, 18]. This allowed the prospective determination of a statistically relevant number of PCT values in a high-risk patient group, which were correlated to the presence or absence of infection, MODS and outcome.
CONCLUSIONS
This study provides the first evidence that neither PCT nor CRP is a reliable marker of infection in paediatric patients treated with veno-arterial ECMO. However, high levels of PCT are associated with MODS. Therefore, PCT may be used to assess the severity of the ECMO-related systemic inflammatory response syndrome and it could be a prognostic indicator of mortality in this high-risk clinical setting.
Funding
The work was supported by funds from Cariverona Foundation (Grant Cariverona 2007).
Conflict of interest: none declared.