-
PDF
- Split View
-
Views
-
Cite
Cite
Tord Fannelop, Geir Olav Dahle, Knut Matre, Christian Arvei Moen, Arve Mongstad, Finn Eliassen, Leidulf Segadal, Ketil Grong, Esmolol before 80 min of cardiac arrest with oxygenated cold blood cardioplegia alleviates systolic dysfunction. An experimental study in pigs, European Journal of Cardio-Thoracic Surgery, Volume 33, Issue 1, January 2008, Pages 9–17, https://doi.org/10.1016/j.ejcts.2007.10.011
- Share Icon Share
Abstract
Objective: Myocardial dysfunction after reperfusion can be a clinical problem in the early postoperative phase after on-pump cardiac surgery. The aim was, in an experimental setting, to investigate if administration of the β-adrenergic receptor blocker esmolol prior to cross-clamping for 80 min with cold oxygenated blood cardioplegia would improve myocardial protection and early postoperative function. Methods: Twenty-four anaesthetised pigs were randomly allocated into one of two equally sized groups and put on mild hypothermic cardiopulmonary bypass. Esmolol 1 mg kg−1 or saline was administered into the arterial line 4 min prior to aortic cross-clamp. Cardiac arrest during 80 min of cross-clamp was obtained with repeated antegrade cold oxygenated blood cardioplegia; the pigs were weaned from bypass following a standardised protocol. Left ventricular global and regional myocardial function and tissue blood flow were evaluated with conductance catheter, echocardiography and coloured microspheres at baseline and at 1, 2 and 3 h after declamping. Four animals did not fulfil the protocol and were excluded. Results: No significant differences between groups could be demonstrated for left ventricular global and local function and tissue blood flow at baseline. At 1 h after declamping the slope of preload recruitable stroke work (PRSWslope) averaged 73.7 ± 12.7 mmHg (SD) in controls and 72.7 ± 11.1 mmHg in esmolol-treated animals. In controls PRSWslope decreased to 62.1 ± 11.0 and 58.4 ± 12.7 mmHg after 2 and 3 h, respectively (p < 0.005 vs 1 h for both). In the esmolol-treated animals PRSWslope remained unchanged at 72.0 ± 11.4 and 73.7 ± 12.9 mmHg at 2 and 3 h after declamp and were significantly higher (p < 0.025 and <0.001) than the corresponding values in the control group. The slope of the end systolic pressure volume relationship did not differ between groups at 1 and 2 h after declamp, but were 1.85 ± 0.86 and 2.51 ± 0.96 mmHg ml−1 in controls and in esmolol-treated animals, respectively, after 3 h (p < 0.025). Conclusions: Esmolol administered prior to cold oxygenated cardioplegic arrest alleviates left ventricular dysfunction in the early hours after cardiopulmonary bypass.
1 Introduction
β-Adrenergic receptor antagonists reduce both ischaemic and reperfusion injury in the myocardium; this has been extensively studied and is well documented. Esmolol, an ultra-short acting β1-adrenoceptor blocker, has gained some special attention in relation to cardiac surgery. Since esmolol has an elimination half-life of 9 min [1], a protracted negative inotropic effect with a disadvantageous influence on weaning from cardiopulmonary bypass (CPB) can be avoided. In clinical settings esmolol has been used as sole cardioprotective agent, demonstrating favourable clinical outcome compared to potassium-based cardioplegia both with blood and with crystalloid solutions [2–4]. Esmolol has also proved beneficial during off-pump surgery [5]. Surprisingly, there are few studies reporting the use of esmolol as adjuvant to standard cardioplegic regimes.
Experimentally, some studies have focused on reduction of ischaemic and reperfusion injury modelling acute regional myocardial ischaemia and operative revascularisation with CPB. In dogs continuous coronary perfusion with esmolol in warm blood improved post-CPB global left ventricular function compared to continuous warm blood cardioplegia, but did not alter local function in the reperfused region [6]. In unloaded hearts (dogs on full CPB) esmolol added to warm oxygenated blood reduced infarct size after 2 h of reperfusion following 120 min of coronary occlusion compared to reperfusion without esmolol [7].
At our institution the standard routine for myocardial protection during on-pump cardiac surgery is multidose antegrade cold (10–12 °C) oxygenated blood cardioplegia and mild (∼34 °C) general hypothermia. Pre-treatment with esmolol as adjuvant to this protocol has a potential for being a safe and easily administered supplement to enhance myocardial protection in patients where long cross-clamp time is anticipated. In an experimental study with pigs on CPB, we hypothesised that one single loading dose of esmolol administered just prior to aortic cross-clamp and cardioplegic arrest with repeated cold oxygenated blood cardioplegia would reduce the ischaemic injury and thus improve global and regional cardiac function after declamping and weaning. In a standardised protocol for cardiac arrest and reperfusion, left ventricular function was evaluated in two groups of pigs randomised to administration of either esmolol or saline just prior to 80 min of aortic cross-clamping and cardioplegia. Left ventricular global and local function together with myocardial blood flow rate was measured by the conductance catheter method, by echocardiography and with coloured microspheres before and at 1, 2 and 3 h after declamping.
2 Materials and methods
2.1 Animals and anaesthesia
Twenty-four pigs (Norwegian Land Race) of both genders weighing 51 ± 5 kg (SD) and with a calculated body surface area of 1.25 ± 0.08 m2 were fasted overnight but had free access to water. The animals were acclimatised for at least 7 days in the animal facility under controlled temperature, lighting and humidity and were fed with a standard commercial young pig diet. The experimental protocol was registered and approved by the Norwegian Animal Research Authority (Project 2004220) and by the local responsible laboratory animal veterinarian, and was conducted in accordance with national and international laws controlling experiments in live animals.
Animals were randomised (drawing lots) into two groups. Excluded animals were replaced with the next animal using the same protocol without re-randomisation. After premedication with ketamine/atropine (20 mg kg−1/1 mg) i.m. in the neck animals were ventilated by mask with O2 and 3% (vaporiser setting) isoflurane (Rhodia, Bristol, England) for 2–3 min to allow cannulation of two ear veins, cleaning and monitoring of ECG and pulse oximetry. Animals were placed on a warm-water blanket on the operating table, and mechanical ventilation (Cato M32000, Drägerwerk, Lübeck, Germany) was commenced and continued with a mixture of N2O (56–57%) and oxygen. A tidal volume of 10 ml kg−1 and a frequency of 13–15 min−1 were used, only with small adjustments aiming at an end-tidal CO2 of 5% and arterial blood gases within physiological limits (AVL Opti1, AVL Scientific Corporation, Roswell, GA). Before tracheotomy and intubation, full surgical anaesthesia was induced by i.v. loading doses of fentanyl 0.02 mg kg−1, midazolam 0.3 mg kg−1, pancuronium 0.063 mg kg−1 and sodium pentobarbital 15 mg kg−1 and maintained with continuous infusions of fentanyl 0.02 mg kg−1 h−1, midazolam 0.3 mg kg−1 h−1, pancuronium 0.14 mg kg−1 h−1 and pentobarbital 4 mg kg−1 h−1. Fluid substitution was given as 15 ml kg h−1 of Ringer’s acetate with 20 mmol l−1 KCl added. Further details on this anaesthetic protocol can be found elsewhere [8].
2.2 Basic instrumentation
The right femoral artery and vein were surgically exposed in the groin, and catheters were advanced into the abdominal aorta and inferior caval vein for blood sampling and infusion purposes. A catheter with thermistor was surgically inserted into the urinary bladder for drainage and temperature monitoring. The heart was accessed through a midline sternotomy and a longitudinal pericardiotomy. After haemostasis, 125 IU kg−1 of heparin was given i.v. to prevent clotting of catheters. Central aortic pressure was measured with a pressure-tip catheter (Millar MPC-500, Houston, TX) advanced via the left mammarian artery. Cardiac output together with pulmonary artery and central venous pressures were assessed with a 7.5F balloon floating catheter (139H-7.5F, Baxter, Irvine, CA) with injection/pressure ports, advanced from the left mammarian vein through the right side of the heart into the pulmonary artery, and connected to a continuous cardiac output computer (Vigilance, Baxter, Irvine, CA) and two pressure sensors (SensoNor, Horten, Norway). The haemodynamic variables were sampled by a 16-channel Gould ES2000 system (Gould Instrument Systems, Valley View, OH), digitised, recorded and analysed by a specially designed program. A 7F dual field conductance catheter with an integrated micro-tip pressure sensor (Millar SPR 788, Houston, TX) was placed via an apical stab centrally into the left ventricle with the tip just above (∼1 cm) the aortic valve, secured with a tourniquet and connected to a Sigma 5 signal conditioner (CD Leycom, Zoetermeer, The Netherlands). Correct catheter positions were verified by obtaining typical pressure tracings and segmental volume signals and also with epicardial echocardiography (System Five, GE Vingmed Ultrasound, Horten, Norway). A snare loosely placed around the inferior caval vein allowed short periods of in-flow reduction. The left atrium was cannulated for microsphere injections. Instrumentation was completed after approximately 2–2.5 h. Animals were allowed to stabilise for ∼30 min before heparin, 500 IU kg−1 i.v. (full heparinisation) was administered.
2.3 Experimental protocol
After the stabilisation period, a baseline registration of arterial blood gases, blood conductivity, cardiac Troponin-T (Roche Diagnostics GmbH, Mannheim, Germany) and haemodynamic variables were obtained together with the first microsphere injection. During a short period (16–20 s) of respiratory shut-off in end-expiry, the ECG, left ventricular pressure and segmental volumes were sampled in a stable situation and during a dynamic preload reduction (inferior caval vein occlusion) by the Sigma 5 signal conditioner and digitised by a computer for later analysis. Left ventricular parallel conductance was assessed three times by injection of hypertonic (10%) saline into the pulmonary artery during respiratory shut-off and continuous registration with the Sigma 5 [9]. Using a 2 cm thick silicon pad as offset, epicardial tissue velocity images were obtained from the left ventricular anterior wall (short axis view) and from the middle part of the interventricular septum (four-chamber long axis view) with a phased array probe used at 4 MHz.
Additional heparin 250 IU kg−1 i.v. was given. With the circuit primed with 1.2 l of Ringer’s acetate animals were cannulated in the brachiocephalic artery (20 Fr, Medtronic Inc., Minneapolis, MN) and the right atrial appendage with a two-stage cannula (Medtronic 28/36 Fr). Mild hypothermic CPB (water temperature 34 °C) was initiated over 60 s after a standardised protocol with a flow aiming at cardiac output noted at baseline. Depending on the randomised group, esmolol (Brevibloc®, Baxter AS, Oslo, Norway) 1 mg kg−1 (esmolol group) or equal volume of 0.9% saline (control group) was administered into the arterial line. After four more minutes on full bypass the aorta was clamped and cardioplegia immediately induced with antegrade cold (12 °C) oxygenated blood cardioplegia in the aortic root for 3 min (high-dose) with an average flow of 354 ± 44 ml min−1 or 6.6 ± 0.4% of baseline cardiac output (Table 1 ). The left ventricle was vented with a 17 Fr catheter through the left atrial appendage and mitral valve. Body temperature was allowed to drift and was noted every 20 min. Repeated administration of cardioplegia (low-dose) was performed for 2 min after 20, 40, and 60 min of aortic clamping. Rewarming (water temperature 38 °C) was commenced after 60 min, and the aortic clamp was removed for reperfusion after 80 min. Except for electroconversion of ventricular fibrillation, no inotropic, chronotropic or antiarrhythmic interventions were allowed in the protocol. After 15 min of reperfusion, animals were gradually weaned from bypass following a standardised protocol during the next 10 min. The venous cannula and left ventricular vent were removed and protamine sulphate 0.1 mg kg−1 slowly administered i.v. The blood in the reservoir was returned observing left atrial visual pressure (the vent catheter repositioned into the left atrium) and remaining cannulaes removed.
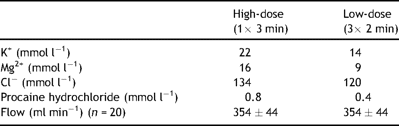
Final concentrations in cold oxygenated blood cardioplegia and flow rate ± SD
Three new data sets were sampled as described above for baseline following 1, 2 and 3 h after declamping and start of reperfusion. The heart was then arrested with saturated KCl and removed. Tissue samples from the left ventricular anterior wall were collected and divided into subendo and subepicardial half layers. Tissue samples from the right and left kidney cortex were obtained to evaluate adequate microsphere distribution.
2.4 Data acquisition
Tissue samples and reference blood were hydrolysed, microspheres isolated and processed; blood flow rate per gram tissue was calculated as earlier described [10]. The left ventricular pressure and volume variables were calculated from raw data files with Conduct 2000 acquisition/analysis software (CD Leycom, Zoetermeer, The Netherlands) by averaging five to eight consecutive heart beats in the stable situation and from consecutive heart beats during inferior caval vein occlusion. Volume signals were calibrated for blood conductance, for parallel conductance and for true cardiac output measured by the continuous cardiac output computer [11,12]. Volumes were indexed (normalised) for body surface area (BSA) calculated as BSA = k BW2/3/100 where BW is body weight in kg and k for pigs is 9 m2 kg−2/3[13].
Tissue velocity images were transferred to a work station, converted to strain rate images (SRI) and analysed for strain with commercially available software (EchoPacPC, GE Vingmed Ultrasound, Horten, Norway) as described earlier [14]. Radial peak ejection and postsystolic strain was calculated in the subendo and subepicardial halves of the anterior left ventricular wall with aortic valve opening and closure defined from pulsed wave Doppler recordings. Longitudinal strain in the mid-wall of the septum was measured from four chamber tissue velocity images. For radial strain measurements, strain length was set to 2 mm with a region of interest of 2 mm × 6 mm, first number denotes dimension along the beam. Corresponding values for longitudinal strain measurements in the septum were 8 mm and 8 mm × 6 mm, respectively.
2.5 Statistical analysis
Values are given as mean ± SD or median (75% quartile–25% quartile) unless otherwise noted. Statistical analyses were performed with the commercial software SPSS v. 15 (SPSS Inc., Chicago, IL). Baseline variables were tested for normality and equal variance by the Kolmogorov–Smirnov and the Levene median tests, respectively. Comparisons of baseline variables between groups were performed by two-sample t-test or Wilcoxon–Mann–Whitney test whenever appropriate. Variables obtained after declamp were studied by two-way analysis of variance (ANOVA) for repeated measurements with time as within-factor and esmolol versus control as grouping factor. If the Mauchly’s test of sphericity was significant (p < 0.05), a Greenhouse–Geisser adjustment of the degrees of freedom was selected when evaluating the significance of main effects. If a significant interaction effect was found, new analyses of variance for simple main effects within and between groups were performed with adjustment of the degrees of freedom [15]. When justified by the preceding ANOVA, means were compared with Newman–Keuls multiple contrast tests. In the two-way ANOVA a significant interaction was declared when p < 0.10, for all other analyses a p < 0.05 was regarded as significant.
3 Results
3.1 Experimental groups
Four animals were excluded for reasons not directly related to surgical and technical failures. In the control group two animals died due to pulmonary hypertension and hypoxia leading to cardiac failure; one shortly after declamp and one after approximately 1 h. In the esmolol group one animal could not be weaned from CPB (cardioversion resistant ventricular fibrillation) at reperfusion, one animal died 1 h after declamp due to pulmonary hypertension and severe and sustained arterial hypoxia followed by cardiac failure. In this animal an atrial septal defect was found. Results are reported for 20 animals, 10 in each group. There were no significant differences between groups with regard to body weight, calculated surface area, body temperature and respirator settings at baseline. Arterial blood gases did not differ and were within physiological limits. Although the mean values of some of the key variables were slightly higher in the esmolol than in the control group, no significant differences between groups could be demonstrated at baseline (Table 2 , see also Figs. 1 and 2 ).
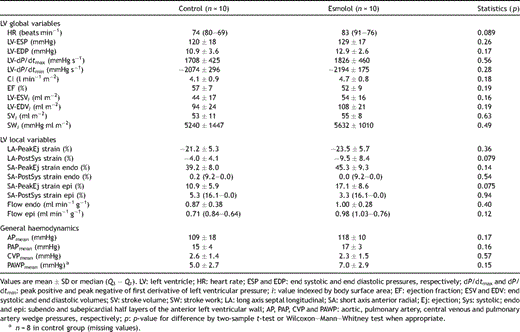
Global and local left ventricular function, regional tissue blood flow rate and haemodynamic variables measured at baseline
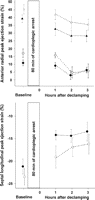
Radial peak ejection strain (upper panel) in the endocardial (triangles) and the epicardial (diamonds) half layer of the left ventricular anterior wall and longitudinal peak ejection strain in the interventricular septum (lower panel). For both: esmolol group, open symbols; control group, filled symbols. Error bars are SEM.
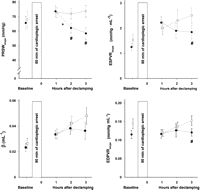
Slope of preload recruitable stroke work (PRSWslope), slope of the end systolic pressure volume relationship (ESPVRslope), left ventricular diastolic stiffness constant (β) and the slope of the linear end diastolic pressure volume relationship (EDPVRslope) obtained during dynamic preload reduction at baseline and 1, 2 and 3 h after reperfusion following cardioplegic arrest. Control: filled circles; esmolol: open rectangles; *: significantly different (p < 0.01) from value to the left in control; #: significantly different (p < 0.025) from corresponding value in control with Newman–Keuls multiple contrast tests. Error bars are SEM.
3.2 Cardiopulmonary bypass
At the start of CPB body temperature averaged 37.4 ± 0.9 °C (n = 20), decreasing to its minimum (34.2 ± 0.9 °C) after 60 min of cardiac arrest and increasing again during rewarming to 36.7 ± 0.9 °C at declamp, with no significant difference between groups. At the start of reperfusion the average mean aortic pressure was 64 ± 21 mmHg, s-K+ 5.0 ± 0.4 mmol l−1, s-Na+ 143 ± 4 mmol l−1 and Hb 9.7 ± 0.9 g dl−1 (n = 20 for all). Neither these nor any of the variables from arterial blood gas analyses at the time of declamping differed significantly between groups.
3.3 General haemodynamics
There was an overall decrease in left ventricular end systolic pressure between 1 and 3 h after declamping whereas heart rate and end diastolic pressure remained essentially unchanged (Table 3 ). The changes in LV-dP/dtmax did not differ between groups over time and averaged (n = 20) 1713 ± 532 mmHg s−1 1 h after start of reperfusion, decreasing to 1396 ± 429 mmHg s−1 (p < 0.001) after 2 h and with no further change after 3 h. In the control group LV-dP/dtmin was −1688 ± 617 mmHg s−1 1 h after start of reperfusion and increased after 2 and 3 h (p < 0.025 vs 1 h for both). In the esmolol group LV-dP/dtmin was unchanged. Furthermore cardiac index tended to decrease and EF decreased significantly over time, with no difference between groups. Left ventricular end diastolic volume and stroke volume also decreased with time. Stroke work decreased in the control group from 1 to 2 h and 3 h after declamp but remained fairly stable over time in the esmolol group. Even though mean arterial pressure tended to be higher compared to esmolol 1 h after declamp in control and decreased over time, no significant group differences could be demonstrated between individual means.
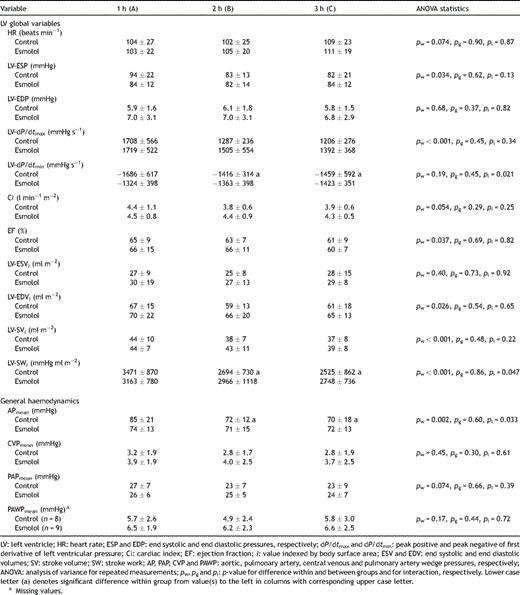
Global left ventricular function and general haemodynamics 1, 2 and 3 h after aortic declamping following 80 min of cardioplegic arrest (mean ± SD), n = 10
3.4 Regional tissue blood flow and function
Subendocardial tissue blood flow rate after start of reperfusion did not differ between groups (pg = 0.54); pooled values (n = 20) were 1.06 ± 0.40 ml min−1 g−1 after 1 h and 0.96 ± 0.19 and 1.00 ± 0.22 ml min−1 g−1 2 after 3 h, respectively (pw = 0.38). Nor in the subepicardium did blood flow differ between groups (pg = 0.99); 1 h after declamping blood flow rate (n = 20) averaged 1.18 ± 0.49 ml min−1 g−1, decreasing significantly in both groups to 0.97 ± 0.18 ml min−1 g−1 after 2 h (p = 0.041) and remained at 0.97 ± 0.22 ml min−1 g−1 after 3 h.
Subendocardial radial short axis peak ejection strain was clearly higher than in the subepicardial half layer in both groups at baseline as well as after declamping, representing more thickening in this wall layer (Fig. 1). During the first 3 h after start of reperfusion there was a borderline group difference (pg = 0.05), indicating a better-preserved subendocardial contraction in esmolol compared to the control group. Subendocardial postsystolic strain after declamping did not differ over time, pooled median values were 1.0% (1.2–0.0) for esmolol versus 6.2% (16.2–2.1) (pg = 0.038) for the control group. In the subepicardium, peak ejection strain did not differ between groups, but decreased significantly (pw < 0.01, n = 20) from 1 to 2 h after start of reperfusion. Subepicardial postsystolic strain did not differ between groups (pg = 0.23). Longitudinal peak ejection strain in the septum was equally negative at baseline (Fig. 1, lower panel), representing the same degree of shortening. After bypass, there was a weak tendency of less negative values in the control than in the esmolol group (pg = 0.12).
3.5 Load independent variables for left ventricular function
None of the load independent variables differed significantly between groups at baseline (Fig. 2). The slope of preload recruitable stroke work (PRSWslope) did not differ between groups 1 h after declamp. In control animals PRSWslope decreased significantly from 1 to 2 h (p < 0.01) but remained unchanged in the esmolol group. These values were significantly higher in the esmolol group compared to corresponding values in the control group after 2 h (p < 0.025) and after 3 h (p < 0.001). The volume axis intercept for PRSW did not change over time after declamp in either group (pw = 0.60) but differed with an average of 14.5 ± 6.0 ml for the control group compared to 23.3 ± 8.2 ml (pg = 0.014) for the esmolol group. The same indication of improved contractility in the esmolol group was observed for the slope of the end systolic pressure volume relationship (ESPVRslope), tending to decrease in the control group and increase in the esmolol group (pi = 0.014), but only differed significantly between groups at 3 h, being 2.51 ± 0.95 mmHg ml−1 in the esmolol group versus 1.85 ± 0.85 mmHg ml−1 in the control group (p < 0.025). The non-linear relationship between end diastolic pressure and volume, the left ventricular stiffness constant (β) did not differ significantly between groups during the first 3 h following declamping whereas the slope of the linear relationship (EDPVRslope) was found to be slightly higher in the esmolol group compared to the control group at 3 h (p < 0.025).
3.6 Tissue water content and troponin release
Myocardial tissue water content 3 h after declamping averaged 80.2 ± 0.6% in the endo and 80.8 ± 0.6% in the epicardium, with no group difference. Serum cardiac Troponin-T levels averaged 0.36 ± 0.19 and 0.40 ± 0.29 μg l−1 (p = 0.73) in the control and esmolol group after 3 h, respectively.
4 Discussion
We found that that pre-treatment with a single bolus of esmolol just prior to aortic clamping for 80 min and repeated antegrade cold oxygenated blood cardioplegia preserves global left ventricular contractility (PRSWslope) in pigs during the first 3 h after declamping and weaning from CPB. In the control group PRSWslope did not differ from the esmolol group at 1 h after declamp but decreased to lower levels after 2 and 3 h. The slope of the end systolic pressure volume relationship was also significantly higher 3 h after declamp in the esmolol group compared to controls.
Our findings demonstrate that esmolol alleviates the reduction of systolic function after cardiopulmonary bypass in the first 3 h after start of reperfusion following cardioplegic arrest. Both in experimental and clinical studies, adaptation to the high catecholamine levels found during CPB will occur, resulting in an agonist induced decrease in the β-receptor adenylate cyclase activity and depression of β-adrenergic receptor signalling [16,17]. The observed reduction of systolic cardiac function early after reperfusion in the control group could be explained by this β-adrenoceptor desensitisation to the high levels of catecholamines during CPB and explains why esmolol prevents reduction in PRSWslope and ESPVRslope, variables both describing systolic function and contractility. In a comparable experimental setting also involving acute regional ischaemia (severe LAD stenosis) followed by cardioplegic arrest, esmolol infusion during CPB and reperfusion enhanced β-adrenoceptor signalling and improved regional contractile function [18]. A reduction of myocardial oxygen metabolism caused by esmolol could be an alternative explanation [4]. A direct esmolol-mediated reduction of the myocardial ischaemic injury during cardioplegic arrest in the present study is less probable, since the serum cardiac Troponin-T values did not differ between groups.
In this model with repeated oxygenated blood cardioplegia it is not possible to distinguish between the beneficial effects of esmolol on the ischaemic and the reperfusion injury. Given the pharmacological properties of esmolol with its short elimination half-life [1], however, the effect caused by esmolol in the present study must primarily be related to the early ischaemic period during aortic cross-clamping. However, the beneficial effects may also be related to the repeated reperfusions with oxygenated blood cardioplegia. In patients, the clearance of a steady-state arterial blood concentration of esmolol is reduced during hypothermic CPB and is not influenced by the membrane oxygenator in the circuit [19].
If judged by the standard clinical variables like cardiac index, ejection fraction, heart rate, left ventricular pressures and volumes, the esmolol group did not demonstrate clear signs of improved cardiac function during the early hours after declamping and weaning compared to the control group (Table 3). Furthermore, the strain measurements demonstrated no clear improvement in regional systolic function (Fig. 1). Many of these variables are highly inter-correlated and also depend on preload and afterload in the individual animal. In the present study, therefore, efforts were made to standardise the conditions. No group difference was found for LV-EDP, LV-EDVi or APmean after weaning from CPB (Table 3). Increased pulmonary vascular resistance, as also observed here, is frequently seen in experimental studies on pigs with cardiopulmonary bypass and reperfusion. No group difference was found for PAPmean (pg = 0.66) after declamping, but the degree of pulmonary hypertension experienced after CPB varied between individual animals and was not liable to standardisation. Although deviating from the idealised linearity when studied over a wide volume range, the slopes of linear regressions for PRSW and ESPVR are regarded as relative load independent variables describing the systolic properties or contractility of the left ventricle [20]. However, a recent study demonstrated that acute pulmonary hypertension reduces left ventricular contractility judged by PRSWslope[21]. In the present study no such correlation (p > 0.43) was found between the individual changes in pulmonary artery pressure and changes in PRSWslope after declamping.
Diastolic dysfunction is believed to precede the systolic dysfunction following ischaemia and reperfusion. The small but significant increase in slope of the EDVPR caused by esmolol 3 h after start of reperfusion could indicate negative effect on left ventricular function (Fig. 2). In principle, the end diastolic pressure volume relationship is non-linear [20]. No significant difference (pg = 0.37) was found between groups with regard to the non-linear stiffness constant β. Tissue oedema following ischaemia and reperfusion is one factor causing increased diastolic stiffness. Tissue water content did not differ between groups in the present study.
4.1 Clinical considerations
Many clinical studies report favourable effects on cardiac function when using esmolol to obtain minimal myocardial contraction in comparison with potassium-mediated cardioplegia during CPB and cardiac surgery. In spite of this, esmolol used as a supplement to standard cardioplegic regimes has not gained much attention in the literature. Patients with continuous esmolol infusion during CPB and 10 min after reperfusion in addition to crystalloid cardioplegia demonstrated an improved cardiac function indices compared to a control group the first 30–60 min after CPB [22,23]. Esmolol in addition to cold repeated blood cardioplegia did not demonstrate biochemical signs of improved cardioprotection [24]. These clinical studies, however, do not necessarily contradict the main results in the present study.
4.2 Study limitations
A direct comparison of results from clinical studies and an experimental study like this may not be pertinent. Apart from the obvious differences related to species, the methodological aims of an experimental study are to standardise the conditions when comparing an intervention group to an untreated control group. The present protocol allowed only cardioversion of ventricular fibrillation at the time of reperfusion as an intervention. One animal was excluded due to therapy resistant fibrillation. In three animals, pulmonary hypertension after weaning from bypass with hypoxia and systemic hypotension leading to myocardial ischaemic injury in the reperfusion phase was the reason for exclusion. These are well known problems related to the use of pigs in experimental models involving cardiopulmonary bypass and myocardial ischaemia/reperfusion. In a clinical setting, however, pharmacological and respiratory interventions and provisional re-establishment of cardiopulmonary bypass might have solved such problems. This, however, can conceal or amplify beneficial or disadvantageous effects of the intervention under study, especially in the early phases of reperfusion after bypass.
5 Conclusion
In the present study adjuvant treatment with a single dose of esmolol 4 min prior to aortic cross-clamp and cardiac arrest with repeated blood cardioplegia did not alter cardiac function judged by clinical variables like cardiac index and ejection fraction, but prevented the development of reduced systolic function in the left ventricle within the first three hours after weaning from cardiopulmonary bypass. One possible explanation for this effect is the reduction by esmolol of β-adrenoceptor desensitisation.
Acknowledgements
We acknowledge the technical assistance from Lill-Harriet Andreassen, Cato Johnsen, Gry-Hilde Nilsen, Anne Aarsand, Inger Vikøyr and the staff at the Vivarium, University of Bergen.
References
Author notes
Funding: Locus for Cardiac Research, University of Bergen and Western Norway Regional Health Authority.
- aorta
- cardiac arrest
- myocardium
- esmolol
- cardiopulmonary bypass
- vascular flow
- cardiac surgery procedures
- hypothermia, natural
- systolic blood pressure
- cerebrovascular accident
- ischemic stroke
- heart arrest, induced
- left ventricle
- reperfusion therapy
- microspheres
- suidae
- systolic dysfunction
- bypass
- catheters
- normal saline
- experimental study
- saline solutions