-
PDF
- Split View
-
Views
-
Cite
Cite
Øyvind Jakobsen, Thor A. Stenberg, Ole Losvik, Sveinung Ekse, Dag G. Sørlie, Lars M. Ytrebø, Adenosine instead of supranormal potassium in cardioplegic solution preserves endothelium-derived hyperpolarization factor-dependent vasodilation, European Journal of Cardio-Thoracic Surgery, Volume 33, Issue 1, January 2008, Pages 18–24, https://doi.org/10.1016/j.ejcts.2007.10.013
- Share Icon Share
Abstract
Objective: We have recently shown that adenosine instead of supranormal potassium in cold crystalloid cardioplegia improves cardioprotection. Studies indicate that hyperkalemia has unfavorable effects on vascular endothelial function. Three pathways have been identified as major vasodilatory pathways: the nitric oxide (NO) pathway, the cyclooxygenase (COX) pathway, and the endothelium-derived hyperpolarization (EDHF) pathway, where the EDHF pathway, in particular, seems susceptible to hyperkalemia. We hypothesized that adenosine cardioplegia improves postcardioplegic endothelial function. Methods: Sixteen pigs were randomized to receive either cold (6 °C) hyperkalemic cardioplegia (n = 8) or cardioplegia where hyperkalemia was substituted with 1.2 mM adenosine (n = 8). After 1 h of cold ischemic arrest, coronary blood flow was monitored for the following 2 h. The LAD artery was then explanted, and cylindrical rings were mounted for isometric tension recordings in organ chambers. Vessels were preconstricted with U46610 (Thromboxane A2 analog) and then bradykinin-mediated relaxation was investigated. To differentiate between the vasodilatory pathways the relaxation was assessed in the absence and presence of inhibitors of the COX (indomethacin), NO (L-NAME + carboxy-PTIO), and EDHF (apamin + charybdotoxin) pathways. Results:Invivo: The adenosine group had, as distinct from the hyperkalemic group, a significantly increased coronary blood flow index 1 h after cross-clamp release (from (ml/min/100 g, mean ± SD) 50.9 ± 13.9 to 72.8 ± 21.9, p = 0.010). The difference was, however, not statistically significant between groups. Invitro: Maximal relaxation without blockers was 27.4 ± 10.1% of maximal tension in the adenosine group and 22.2 ± 7.5% in the hyperkalemic group. To investigate EDHF-dependent vasodilation the vessel rings were simultaneously treated with indomethacin, L-NAME, and carboxy-PTIO. Maximal relaxation in the hyperkalemic group was then reduced to 47.4 ± 17.4% of maximal tension, which was a significant reduction compared to the adenosine group with a maximal relaxation of 20.6 ± 8.7% (p = 0.028). Conclusion: Adenosine instead of supranormal potassium in cold crystalloid cardioplegia increases postcardioplegic myocardial blood flow and preserves EDHF-dependent vasodilation.
1 Introduction
Hypothermic hyperkalemic cardioplegia is still the most common modality in open chest heart surgery. However, a number of studies indicate that hyperkalemic cardioplegic solutions have unfavorable effects on vascular endothelial function [1,2]. The vascular endothelium is known to play an important role in the regulation of vascular homeostasis. To date, the nitric oxide (NO) pathway, the cyclooxygenase (COX) pathway, and the endothelium-derived hyperpolarization (EDHF) pathway have been identified as the major vasodilatory pathways. The relative contributions from these pathways vary significantly between different vascular beds and are also highly dependent on the vessel diameter [3],. Vascular dysfunction after the use of hypothermic hyperkalemic cardioplegia is mediated to a large extent through the EDHF pathway, while the NO and COX pathways seem to be less affected [4,5]. It is also shown that endothelial reperfusion injury following hyperkalemic cardioplegia in human myocardium is associated with microcirculatory disturbances with trapping of both red blood cells and neutrophils [6]. Cardioplegic solutions that might have a potential to preserve vascular endothelial function are therefore of interest.
Several studies have shown that adenosine in the cardioplegic solution, either alone or in combination with hyperkalemia, improves cardioprotection [7,8]. Adenosine is a potent coronary vasodilator and has been proven to be of importance in the regulation of vascular tone during increased metabolic demands and myocardial stress [9]. Four subtypes of adenosine receptors (A1, A2A, A2B, A3) have been characterized [10]. The A1 and A2 receptor subtypes are present in the coronary artery vascular smooth muscle [11], while both A2A and A2B receptors are present in coronary endothelial cells [12]. Activation of these receptors has previously been shown to induce coronary vasodilation through ATP-sensitive potassium channels (KATP) and NO release [13]. Furthermore, supplementation of adenosine in the reperfusion period following ischemia has been shown to reduce infarct size and improve endothelial function [14]. Adenosine has also been shown to attenuate functional and structural abnormalities in the microvasculature [15] together with inhibiting intra- and perivascular neutrophil accumulation and superoxide generation [16].
Corvera et al. [17] used the combination of adenosine and lidocaine in warm and cold blood cardioplegia, and investigated postexperimental left anterior descending coronary artery (LAD) endothelial and smooth muscle function. The problem with the use of adenosine in blood cardioplegia is, however, the rapid catabolism of adenosine in blood (half-life of 0.6 s) [18]. Corvera et al. also used low concentration of adenosine (400 μM), and did not differentiate between the different vasodilatatory pathways.
We have recently performed a randomized controlled study on the effects of administering adenosine instead of supranormal potassium in the cold crystalloid cardioplegic solution in a well-characterized pig model [8]. Data provided evidence for a satisfactory cardiac arrest, improvement in postcardioplegic left ventricular systolic function and efficiency, and an attenuation of myocardial damage [8]. As part of the study we also looked at the postischemic endothelial function as revealed by coronary blood flow and a standardized set of measurements on explanted parts of the left anterior descending coronary artery. We hypothesized that adenosine instead of supranormal potassium in the cold crystalloid cardioplegia would better preserve postcardioplegic endothelial function.
2 Materials and methods
2.1 Animal care
The experimental protocol was approved by the local steering committee of the Norwegian Animal Experiments Authority. The study was conducted in compliance with the Guide for the Care and Use of Laboratory Animals published by the US National Institute of Health 1996 (National Institute of Health Publication No 8523, revised 1996). Sixteen Norwegian landrace pigs of both sexes weighing 59.7 ± 6.6 kg (mean ± SD) were fasted overnight, but had free access to water before the experiment.
2.2 Anesthesia
The pigs were premedicated with intramuscular ketamine (20 mg/kg; Warner Lambert Nordic, Sweden) and atropine (2 mg/kg; Nycomed Pharma, Norway). Anesthesia was intravenously induced via an ear vein with pentobarbital (10 mg/kg; Nycomed Pharma, Norway) and fentanyl (0.02 mg/kg; Pharmalink, Sweden). The pigs were tracheostomized and mechanically ventilated (Servo Ventilator 900D, Elema-Schønander, Sweden) with 60% oxygen (100% during reperfusion and weaning off cardiopulmonary bypass). Ventilation was adjusted by repeated arterial blood gas analyses (Rapidlab 860, Chiron, USA) throughout the experimental period with PaCO2 within 4.0–5.0 kPa. Anesthesia was maintained with a continuous infusion of pentobarbital (4 mg/kg/h), fentanyl (0.02 mg/kg/h), and midazolam (0.3 mg/kg/h; Alpharma, Norway) via the left external jugular vein. Glucose-enriched normal saline for fluid replacement (1.25 g/l 0.9% saline) was given intravenously at a rate of 10 ml/kg/h via the left external jugular vein.
2.3 Surgical preparation
After a median sternotomy the pericardium was incised and transit time flow probes (Cardio-Med CM 4000, Medi-Stim AS, Norway) were applied around the proximal parts of the right, the circumflex, and the left anterior descending coronary arteries and the pulmonary trunk, to continuously measure coronary blood flow (CBF) and cardiac output (CO). Central venous pressure (CVP) was measured via the right external jugular vein, and mean arterial pressure (MAP) was measured in the descending aorta through the right femoral artery.
2.4 Cardiopulmonary bypass protocol
The pigs were block randomized in two rounds (equal number of pigs in both groups after 8 and 16 pigs) to receive either cold (6 °C) hyperkalemic cardioplegia (hyperkalemic group) or cardioplegia, where 1.2 mM adenosine was given instead of supranormal potassium (adenosine group). The concentrations of procaine and magnesium were similar in the two groups. The compositions of the cardioplegic solutions are given in Table 1 . The solutions were made at the Department of Pharmacy (University Hospital of North Norway) and blinded for the investigators. Body temperature was held at 38 ± 1 °C (normothermic for pigs) throughout the whole experiment. Before cannulation the pigs received 380 IE/kg heparin intravenously to keep the activated clotting time (ACT) > 480 s. ACT was repeatedly monitored while the pigs were on cardiopulmonary bypass (CPB). The pigs were cannulated through the right carotid artery with a pediatric arterial cannula (18 Fr, Jostra Arterial Cannula, Maquet, Germany), and venous drainage was obtained from a cavoatrial cannula (32/40 Fr, Dual Stage Venous Drainage Cannula, Edwards Lifescience, Canada). Normotherm cardiopulmonary bypass was initiated with a flow similar to baseline CO, using a roller pump (Stockert/Shiley Caps Roller Pump, Soma Technology, USA) and a membrane oxygenator (Synthesis, Sorin Biomedica, Italy). A non-heparin coated polyvinylchloride tubing was employed. A standard cardioplegic cannula (9 Fr, dlp-CB20012, Aortic root cannula, Medtronic, USA) with a side branch for venting of the left ventricle was placed in the ascending aorta. After cannulation, the aorta was cross-clamped for 1 h. The cardioplegia (6 °C) was given antegradely and intermittently: the first dose of 500 ml immediately after clamping the aorta, the second and third of 200 ml following 20 and 40 min after clamping. Infusion rate was 200 ml/min. Ice-slush was applied, and an isolation pad was put behind the heart to maintain low temperature between the infusions. After 1 h of cold ischemic arrest the aortic cross-clamp was released. Weaning from CPB was tried 20 min after cross-clamp release. If required, animals were given additional support for another 20 min before CPB was terminated. The pigs were monitored for 2 h following aortic cross-clamp release before the ramus descendens anterior of the left coronary artery (LAD) was rapidly explanted.
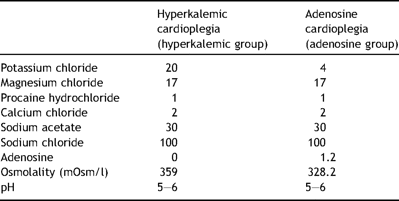
Composition of the cardioplegic solutions in final concentration (mmol/l)
2.5 Tissue preparation
After dissecting the arteries they were immediately transferred into oxygenized (95% O2, 5% CO2), modified ice-cold Krebs-Henseleit solution (in mmol/l: NaCl 118.3, KCl 4.7, CaCl2 2.5, MgSO4 1.17, KH2PO2 1.18, NaHCO3 25.0, EDTA 0.026, glucose 11.1) for subsequent handling. The vessels were cleaned from fat and connective tissue under a microscope, and the vessel integrity was maintained during preparation by protecting the endothelium from stretch and other mechanical injury. Next the arteries were cut into cylindrical rings of 4 mm length and transferred into 25 ml organ chambers containing oxygenized Krebs-Henseleit solution at 37 °C. Rings were mounted on tungsten wires and connected to isometric force transducers (FT-3; Grass Instrument Company, West Warwick, USA) coupled to a computer with software for online tension recordings (Grass Poly View, West Warwick, RI, USA). Initially, a resting tension of 30 mN was adjusted, and the vessels were allowed to normalize for a total period of 1 h. Thirty minute into the normalization period the organ chambers were perfused with oxygenized Krebs-Henseleit solution.
To ensure the viability of the vessels, they were preconstricted with 10 μM U46619 (a thromboxane A2 analog), and the tensions were recorded when stabilized. Subsequently, the chambers were perfused with oxygenized Krebs-Henseleit buffer several times until resting tension was reached. Antagonists of the COX, NOS, and EDHF vasodilatory pathways were then simultaneously applied to the bathing solution in each chamber according to Table 2 , and then allowed to stabilize for 30 min. Subsequently the vessel rings were contracted with 10 μM U46619. Once contraction of the vessels reached a stable plateau, bradykinin was added to the bathing solution and dose–response curves in the absence and presence of endothelium-dependent inhibitors were generated.

Blockers used in the different organ chambers for inhibiting vasodilatation pathways
2.6 Drugs
St. Thomas’ Hospital No. II cardioplegia was purchased from Ullevaal University Hospital, Norway. All other chemicals were purchased from Sigma Chemical Co. (St. Louis, MO, USA).
2.7 Statistics
Data are expressed as mean ± SD. Cardiac index (CI) was calculated as cardiac output divided by preoperative weight. Coronary blood flow index was calculated as total coronary blood flow per 100 g heart weight. Left ventricular coronary blood flow (LVCBF) was calculated as the sum of blood flow through the circumflex and the left anterior descending coronary arteries. Statistical analyses were performed using the Statistical Package for the Social Sciences, version 11.0 for Windows (SPSS, Chicago, Ill., USA). Two-way ANOVA was applied to test for differences within and between groups over time for coronary blood flow, coronary blood flow index, and coronary vascular resistance. To test for differences in maximal relaxation response in the isolated vessels Student’s t-test was applied. Probability values p ≤ 0.05 were considered significant for all tests applied.
3 Results
3.1 In vivo measurements
Cardiac hemodynamic variables are given in Table 3 . The hyperkalemic group had an overall higher coronary flow at baseline, but the groups were not significantly different. The adenosine group had, as distinct from the hyperkalemic group, a significantly increased left and right ventricular coronary blood flow and coronary blood flow index 1 h after cross-clamp release. Furthermore, blood flow remained significantly increased in the right coronary artery 2 h after cross-clamp release in the adenosine group. The differences were, however, not statistically significant between groups. Except from a lowered coronary vascular resistance there were no significant shifts in coronary blood flow in the hyperkalemic group. Both groups had a significant decrease in coronary vascular resistance 1 and 2 h after cross-clamp release, but without any significant differences between the groups when we compared individual changes (Δ) from baseline values.
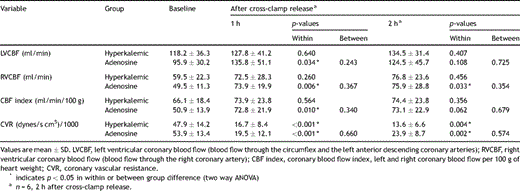
In vivo measurements of coronary blood flow and vascular resistance at baseline and after cross-clamp release
3.2 In vitro measurements
A summary of organ chamber data is displayed in Fig. 1 , and comparisons of the two groups are given in Fig. 2 . The maximal relaxation with all vasodilatory pathways intact (no inhibitors) was 27.4 ± 10.1% of maximal tension for the adenosine group and 22.2 ± 7.5% for the hyperkalemic group (Fig. 2a). Inhibiting COX with indomethacin had no significant effect on the maximal relaxation (adenosine group 26.5 ± 8.8%, hyperkalemic group 29.8 ± 13.5%; Figs. 1 and 2b). Treatment with indomethacin, L-NAME, and carboxy-PTIO, leaving EDHF as the only intact vasodilatory pathway through inhibition of COX and NOS, resulted in a maximal relaxation of 47.4 ± 17.4% of the maximal tension for the hyperkalemic group. This is a significant reduction when compared to the adenosine group where the maximal relaxation was 20.6 ± 8.7% of maximal tension (p = 0.028; Fig. 2c). Fig. 2d illustrates the effect of inhibiting all endothelium-derived pathways. There was no difference in relaxation between the groups (adenosine group 86.9 ± 12.7% vs hyperkalemic group 86.6 ± 11.3%).
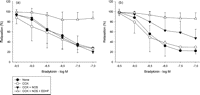
Vasodilatory response to increasing concentrations of bradykinin with and without blockers of vasodilatory pathways in LAD arteries from pigs, following 1 h of cold global ischemia with (a) adenosine cardioplegia and (b) hyperkalemic cardioplegia.
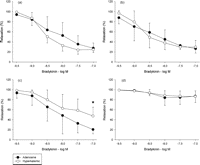
Vasodilatory response to increasing concentration of bradykinin without and with simultaneous blockers of the three different vasodilatory pathways; (a) without any blockers (b) COX blocker (indomethacin); (c) COX + NOS blockers (indomethacin + L-NAME + carboxy-PTIO); (d) COX + NOS + EDHF blockers (indomethacin + L-NAME + carboxy-PTIO + charybdotoxin + apamin), following 1 h of cold global ischemia with hyperkalemic or adenosine cardioplegia. (*) Indicates p < 0.05 in between group difference (Student’s t-test).
4 Discussion
In vivo examination of coronary blood flow patterns revealed significantly increased postcardioplegic coronary blood flow in both the right and the left coronary arteries in the adenosine group, as distinct from the hyperkalemic group, but without significance between group differences. In vitro studies of isolated LAD arteries suggest that adenosine cardioplegia has favorable effects compared to standard hyperkalemic cardioplegia, as the vasodilatory ability was better preserved in the adenosine group after adding blockers of the COX and NO pathways (EDHF-dependent vasodilation).
The present study adds to the ongoing discussion as to whether high potassium concentrations should be used in cardioplegic solutions. During cardiac surgery the heart is arrested and subjected to ischemia–reperfusion injury. Endothelial function during and after cardioplegic arrest is therefore of specific interest in relation to development of novel protective strategies [19]. The NO pathway is known to be particularly susceptible to ischemia–reperfusion, whereas the EDHF pathway may be altered by hyperkalemic cardioplegia [19]. Several studies have shown that exposure of the endothelium to hyperkalemia gives impaired endothelial function through decreased EDHF-mediated relaxation in coronary arteries [5,19]. However, causes for the detrimental effects following cardiopulmonary bypass are combined, and ischemia per se is likely to affect several endothelium-dependent vasodilatory pathways. Accordingly, the findings in the present study are of particular interest as we were able to show that bradykinin-induced vasodilation in the presence of COX and NOS inhibitors was preserved in the adenosine group. This observation supports previously published studies where impaired endothelial function have been shown to be linked to the EDHF pathway, while the COX and NO pathways seem to be less affected after exposure to high concentrations of potassium [19].
The hallmark of EDHF is vasodilation that is insensitive to agents that block the production of NO and prostacyclin (PGI2), but is blocked by a combination of charybdotoxin and apamin [3]. This is taken as proof of the involvement of intermediate-conductance Ca2+-activated K+ channels (IKCa) and small-conductance Ca2+-activated K+ channels (SKCa), which are almost exclusively expressed on endothelial rather than smooth muscle cells. The opening of these channels is responsible for initiating a hyperpolarization of vascular endothelial cells, which is then transferred to the vascular smooth muscle cells (VSMC) via myoendothelial gap junctions or evokes the release of a hyperpolarizing factor that acts directly on the smooth muscle layer. The exact nature of EDHF remains controversial and, apart from gap junctions, several different EDHFs have been proposed as being responsible for the transfer of hyperpolarization from the endothelial cells to VSMC. These include (1) metabolites of the cytochrome P450 pathway, such as an epoxyeicosatrienoic acid (EET); (2) the monovalent cation K+; (3) hydrogen peroxide; and (4) C-natriuretic peptide [3]. In addition, the effect of many other well-characterized vasodilatory mechanisms, such as NO, PGI2, and carbon monoxide, has been shown to be at least partly mediated via opening of potassium channels on VSMC and thereby induce hyperpolarization [20].
We did not perform any specific NO-blocking study, and hence we cannot really estimate the relative contribution from the different pathways. However, we did get a fairly good estimate by blocking the COX pathway, as this reflects the vasodilatory potential of the combined NO and EDHF pathways. The present study was not designed to pinpoint the vasodilator that is most important for the vasodilatory response, but data allow us to speculate on potential mechanisms. Improved vasodilatory response to EDHF might be explained by either increased EDHF response or change in NO status. However, more work is needed to determine the relative contributions from these two main vasodilatory pathways.
Adenosine is known to attenuate functional and structural abnormalities in the microvasculature [15], which might have important implications for autoregulation of regional blood flow. We found increased postcardioplegic coronary blood flow when adenosine was used, indicating a beneficial effect on vasodilatory function. This might partly be due to EDHF pathway involvement distal to LAD, but the present study was not designed to explore this any further.
Adherence of neutrophils to the endothelium is reported to be one of the initiating factors leading to the cascade of inflammatory events after myocardial ischemia, and it has been shown that endothelial dysfunction occurs initially upon reperfusion of the previously ischemic heart and is aggravated by superoxide radicals produced by activated neutrophils [7,21]. Adenosine is shown to inhibit both superoxide radical generation by neutrophils and adherence-activated neutrophils [22], primarily through the A2A receptor [7]. Furthermore, A2A receptor activation is shown to act directly on the endothelium through reducing postischemic leukocyte–endothelium interaction [23]. Reperfusion injury is also attenuated by A2 receptor activation through inhibition of neutrophil accumulation, superoxide generation, and coronary endothelial adherence [16]. We can therefore conclude that neutrophil-mediated endothelial injury is an important contributor to reperfusion injury, and that adenosine, primarily through A2 receptor activation, attenuates reperfusion injury through inhibiting neutrophil-related pathways. The present study shows that endothelial dysfunction is present several hours after the ischemic insult and that adenosine, even when administered in the ischemic period, seems to protect against endothelial reperfusion injury. Since adenosine has a very short half-life, this indicates an immediate protective effect against the ischemic–reperfusion endothelial injury. However, adenosine has other well-known cardioprotective properties, both when used as a pharmacologic pre- and postconditioning agent [24]. Administration of adenosine during the reperfusion period has been shown to reduce infarct size [14] and inhibit neutrophil-mediated processes resulting in endothelial injury [16]. Prolonged administration of adenosine therefore might provide additive protective effects.
Baseline measurements showed an overall higher coronary blood flow in the hyperkalemic group, but the groups were not significantly different. Since the groups were randomized, blinded for the researchers, and the baseline measurements were done before any administration of cardioplegia, this difference could only be explained through a coincidence due to individual variations. Since the pigs, however, are their own control, the groups should be comparable. In the present study we could not detect any significant differences between groups in vivo, while the in vitro part demonstrated a better preserved postcardioplegic endothelial function in the adenosine group. A probable explanation for this difference is that the in vivo results are only observed parameters dependent on several other physiological factors, while the in vitro part exclusively and with higher sensitivity reflects the postcardioplegic endothelial function.
The present study has certain limitations; we used intact adolescent healthy pigs, with healthy coronary arteries without any atherosclerosis. Since responses can vary both between species, be age-related, and depend upon pathological conditions in the coronary arteries, our observations cannot be directly translated into the senescent, diseased human heart with pathological coronary arteries. However, we used a well-established large animal model, which has been used for many years to study novel cardioprotective strategies in our laboratory [25], with a cardiopulmonary bypass protocol very much similar to the daily clinical setting. We only used preparations from LAD in the in vitro study. LAD was chosen because this artery was deemed to be the most important coronary artery, together with being the easiest coronary artery to dissect rapidly and carefully. After seeing the in vivo results it might have been interesting to investigate also the right coronary artery, but because these results were available only after completion of the experiments and since the endothelial physiology is assumed similar in the right and left coronary artery we only investigated in vitro effects of the LAD artery. Furthermore, relative contribution of the different vasodilatory pathways might very well differ between different vascular beds and between species. It is generally believed that EDHF is more important for regulation of vascular tone in smaller arteries, while the NO pathway is thought to be important in larger conduit arteries. Accordingly, the present results must be interpreted with caution.
5 Conclusion
Adenosine instead of supranormal potassium in cold crystalloid cardioplegic solution increases postcardioplegic myocardial blood flow and preserves endothelial function through the EDHF pathway after global cardioplegic ischemia in pigs on cardiopulmonary bypass.
Acknowledgements
We appreciate the skillful technical assistance at the Surgical Research Laboratory at the University of Tromsø.
References
Author notes
This study was supported with a grant from the Norwegian Council on Cardiovascular Diseases.