-
PDF
- Split View
-
Views
-
Cite
Cite
Oliver J. Liakopoulos, Nils Teucher, Christian Mühlfeld, Peter Middel, Gerd Heusch, Friedrich A. Schoendube, Hilmar Dörge, Prevention of TNFα-associated myocardial dysfunction resulting from cardiopulmonary bypass and cardioplegic arrest by glucocorticoid treatment, European Journal of Cardio-Thoracic Surgery, Volume 30, Issue 2, August 2006, Pages 263–270, https://doi.org/10.1016/j.ejcts.2006.04.019
- Share Icon Share
Abstract
Objective: Cardiac surgery on cardiopulmonary bypass (CPB) results in progressive myocardial dysfunction, despite unimpaired coronary blood flow, and is associated with increased myocardial tumor necrosis factor-α (TNFα) expression. We investigated whether anti-inflammatory treatment prevents increased TNFα expression and myocardial dysfunction after CPB. Methods and results: Baseline systemic hemodynamics, myocardial contractile function, aortic and coronary blood flow were measured in anesthetized pigs. Then, placebo (PLA; saline; n = 7) or methylprednisolone (MP; 30 mg/kg; n = 6) was infused intravenously and CPB was instituted. Global ischemia was induced for 10 min by aortic cross-clamping, followed by 1 h of cardioplegic cardiac arrest. After declamping and reperfusion, CPB was terminated after a total of 3 h. Measurements were repeated at 15 min, 4 h, and 8 h following termination of CPB. Systemic TNFα-plasma concentrations and left ventricular TNFα expression were analyzed. With unchanged coronary blood flow in both groups, a progressive loss of myocardial contractile function to 38 ± 2% of baseline (p ≪ 0.01) and cardiac index to 48 ± 6% of baseline (p ≪ 0.01) at 8 h after CPB in PLA was attenuated in MP (myocardial function: 72 ± 3%, p ≪ 0.01 vs PLA; cardiac index: 78 ± 6%, p ≪ 0.05 vs PLA). Systemic TNFα was increased at 8 h in PLA compared to MP (243 ± 34 vs 90 ± 34 pg/ml, p ≪ 0.05). Myocardial TNFα was increased at 8 h after CPB compared to baseline and MP (p ≪ 0.05). Myocardial TNFα immunostaining was more pronounced in PLA than in MP (p ≪ 0.05), with TNFα-mRNA localization predominantly to cardiomyocytes. Conclusions: Methylprednisolone attenuates both systemic and myocardial TNFα increases and progressive myocardial dysfunction induced by cardiac surgery, suggesting a key role for TNFα.
1 Introduction
Postoperative low cardiac output due to myocardial dysfunction is associated with substantial morbidity and mortality, especially in patients with unstable angina and impending myocardial infarction referred for urgent coronary artery bypass grafting [1,2]. Simulating this clinical scenario, we have recently demonstrated a progressive myocardial dysfunction despite unimpaired coronary blood flow in an experimental model of cardiac surgery with cardiopulmonary bypass (CPB) [3]. The progressive pattern of myocardial dysfunction was associated with an increase of systemic and myocardial tumor necrosis factor-α (TNFα) expression and suggested underlying mechanisms at least partially different from those of myocardial stunning.
The concept of a systemic inflammatory response syndrome induced by cardiac surgery and CPB is well established (for review see Ref. [4]), and numerous studies have demonstrated an association between systemic release of pro-inflammatory cytokines and both hemodynamic instability and clinical outcome [5–9]. Beyond systemic inflammation, local myocardial inflammation after cardiac surgery and its sequelae have been investigated less extensively [10,11]. Although negative effects of cytokines on myocardial function have been demonstrated in ischemia–reperfusion [12], coronary microembolization [13], and heart failure, data on the relationship between local myocardial inflammation induced by cardiac surgery and postoperative myocardial dysfunction are still lacking.
In the present study, we investigated whether or not anti-inflammatory treatment with glucocorticoids prevents myocardial inflammation – with focus on TNFα – and myocardial dysfunction after cardiac surgery.
2 Materials and methods
All animals received humane care in compliance with the European Convention on Animal Care. The experimental protocol used in this study was approved by the bioethical committee of the district of Braunschweig, Germany. Experimental preparation, hemodynamic data analysis, measurement of systemic TNFα concentration and immunohistochemical, morphological and electron microscopic analysis were described in detail previously [3].
2.1 Experimental preparation, hemodynamic data analysis
Thirteen pigs (body weight 62 ± 5 kg) were anesthetized with ketamine (6 mg/(kg h)) and pentobarbital (6–8 mg/(kg h)) intravenously, and instrumented with venous, arterial, left atrial and left ventricular (Model SPC-350, Millar Instruments Inc., Houston, TX, USA) catheters for measurement of systemic hemodynamics. Aortic and coronary blood flow were recorded by transit time flow probes (Model 20A/3S, Transonic Systems Inc., NY, USA) placed around the ascending aorta and the proximal left anterior descending coronary artery. Sonomicrometric piezoelectric crystals (WT 5-2, Hugo Sachs Elektronik GmbH, March-Hugstetten, Germany) were implanted into the anterior myocardial wall for measurement of myocardial wall thickness.
After systemic anticoagulation with heparin (300 IU/kg, Hoffmann-La Roche AG, Grenzach-Wylen, Germany), CPB was instituted using a standard clinical setup with cannulation of the ascending aorta and right atrial appendage. Flow index was 2.5–3.3 l/(min m2) with mean arterial pressure maintained at about 60 mmHg. On CPB, animals were systemically cooled to moderate hypothermia (32 °C) and rewarmed to 37 °C beginning 30 min before CPB termination. After CPB, anticoagulation was reversed by 1:1 protamine administration and body temperature was maintained then at 37 °C throughout the remaining protocol using a heating table.
Hemodynamic data, blood flow data, and sonomicrometric dimensions (Triton Technology Inc., San Diego, CA, USA) were digitized and recorded at a rate of 500 Hz throughout the experiment using a 16-channel hemodynamic setup (Hugo Sachs Elektronik-Harvard Apparatus GmbH, March-Hugstetten, Germany) with a dedicated software for data acquisition and signal analysis (HSE Haemodyn Version 1.0, Hugo Sachs Elektronik-Harvard Apparatus GmbH). Heart rate (HR), mean arterial pressure (MAP), left ventricular systolic pressure (LVPsys), and its maximal first derivative (dP/dtmax), left ventricular end-diastolic pressure (LVPed), left atrial pressure (LAP), central venous pressure (CVP), and coronary blood flow (CBF) are reported. Cardiac index (CI) was calculated from aortic blood flow and body surface area. Regional myocardial contractile function was determined as systolic wall thickening (WT%) given as systolic wall excursion in percent of end-diastolic wall thickness (Wted). End-systole was defined as the point of return to zero of the aortic flow signal. End-diastole was defined as the point of the initial upslope of left ventricular dP/dt after crossing zero. Hemodynamic data were analyzed over 12 consecutive seconds.
2.2 Experimental protocol
After baseline measurements, transmural left ventricular biopsies (3 mm × 3 mm × 12 mm) were obtained, and saline (PLA; n = 7) or 30 mg/kg methylprednisolone (MP; n = 6) was infused intravenously. CPB was instituted and global ischemia was achieved by aortic cross-clamping for 10 min. Then, without intermittent declamping cardioplegic cardiac arrest was established with a single infusion of cold (4 °C) crystalloid cardioplegic solution (HTK solution, 30 ml/kg, Köhler Chemie GmbH, Alsbach-Hähnlein, Germany) into the aortic root. After 60 min, the aorta was declamped and heart rhythm was restored with defibrillation (10–20 J). All animals were weaned from CPB after a total duration of 3 h. The chest was left open until the end of the experiment. No inotropic support or allogenic blood transfusions were given throughout the protocol. Central venous pressure and left atrial pressure were kept constant at baseline values by crystalloid fluid administration. Hemodynamic measurements were performed after stabilization prior to CPB (baseline) and at 15 min, 4 h, and 8 h after termination of CPB.
2.3 Systemic TNFα and IL-6 concentration
TNFα-plasma concentrations were measured from arterial blood samples at baseline, 15 min and 8 h after termination of CPB using a pig-specific enzyme-linked immunosorbent assay (Quantikine® P, R&D Systems Inc., Minneapolis, USA). The minimum detectable dose according to the manufacturers’ manual is 5 pg/ml for TNF and 10 pg/ml for IL-6.
2.4 Morphology
Using standard light microscopy (40× magnification) transmural tissue specimens of 5 mm × 5 mm × 12 mm size obtained postmortem from the anterior and posterior left ventricular wall were analyzed after hematoxylin and eosin staining. Tissue areas exhibiting hypereosinophilia, contraction bands, and thinning and waviness of fibers were appreciated as necrotic.
For electron microscopy, transmural biopsies randomly obtained from the left ventricular free wall at baseline and 8 h after CPB were fixed by immersion with 1.5% paraformaldehyde and 1.5% glutaraldehyde in 0.15 M Hepes buffer. After postfixation in 1% osmium tetroxide buffered in 0.1 M cacodylate, tissues were rinsed in distilled water, dehydrated in an ascending acetone series and embedded in araldite. From each pig, two tissue blocks (baseline and at 8 h after CPB) were chosen randomly for cutting. Ultrathin sections were stained with uranyl acetate and lead citrate. Three ultrathin sections per block each with an average myocardial area of 40,000 μm2 were mounted on a copper grid and investigated qualitatively using an EM 900 (Zeiss, Oberkochen, Germany). At magnification levels between ×3,000 and ×20,000, the myocardium was examined with respect to signs of irreversible cellular damage. All light and electron microscopic analysis were performed in blinded fashion by two investigators.
2.5 Myocardial TNFα protein
Transmural left ventricular biopsies taken at baseline, 15 min and at 8 h after termination of CPB were snap-frozen in liquid nitrogen and stored at −80 °C until Western blot. Total protein homogenates (50 μg) were denatured and separated on 12% SDS–polyacrylamide electrophoresis gels and transferred to nitrocellulose membranes (Protran, Schleicher-Schüll GmbH, Dassel, Germany). For immunoblotting, membranes were blocked with 5% nonfat dried milk in 0.1% TRIS-buffered saline (TBS, 0.5 M TRIS, 1.5 M NaCl, 1000 ml distilled water, pH 7.6) and incubated over night at 4 °C. After washing with TBS, primary antibodies against TNFα (1:1000, polyclonal goat anti-human TNFα, Acris Antibodies GmbH, Hiddenhausen, Germany) and ß-actin (1:5000, polyclonal goat anti-human ß-actin, Santa Cruz Biotechnology, CA, USA) were added over night at 4 °C. Thereafter, membranes were washed in TBS and incubated with secondary peroxidase-conjugated antibody (1:5000, Pierce Biotechnology Inc., Rockford, IL, USA) for 2 h. The immunoreaction was visualized using a chemiluminescence system (Super Signal West Pico Chemiluminescence, Pierce Biotechnology Inc.) and densitometric analysis of blots was performed using Biodoc-Analyze software (Whatman-Biometra GmbH, Göttingen, Germany). Protein signals for TNFα were normalized for ß-actin and are reported in percent change to baseline values.
2.6 Immunohistochemistry for TNFα
Transmural left ventricular biopsies were taken at baseline and 8 h after termination of CPB. Immunohistochemistry was performed using a polyclonal goat anti-human TNFα antibody (1:1000, Acris Antibodies GmbH) and anti-goat IgG as secondary antibody (1:400, DakoCytomation GmbH, Hamburg, Germany). Visualization was enhanced with EnVision® Dual Link HRP System (EnVision® HRP System, DakoCytomation GmbH, Hamburg, Germany). The sections were evaluated semi-quantitatively by light microscopy (BH2-Olympus, Hamburg, Germany) using a 1–5 scale as follows: 1, no staining; 2, rare staining; 3, moderate staining; 4, marked staining; 5, extensive staining. All immunohistochemical analyses were performed blinded by two independent investigators.
2.7 TNFα in situ hybridization
Snap-frozen myocardial biopsies were homogenized with TRI Reagent® (Sigma-Aldrich Chemie GmbH, Munich, Germany), and total RNA isolated by chloroform extraction and purified using the RNeasy Mini Kit (Qiagen GmbH, Hilden, Germany). A total of 1 μg mRNA was reversely transcribed into cDNA by using conventional recombinant DNA techniques with PCR (Mastercyler®, Eppendorf AG, Hamburg, Germany) and SuperScript II reverse transcriptase (Invitrogen GmbH, Karlsruhe, Germany). Two primers (MWG Biotech AG, Munich, Germany; TNFα sense; T3 = 5′-ATT AAC CCT CAC TAA AGG GAC TGT GCC TCA GCC TCT TCT C-3′; TNFα antisense; T7 = 5′-GGA TCC TAA TAC GAC TCA CTA TAG GGA GGC AGG AGG GCA TTG GCA TAC-3′) were used to amplify the fragment of pig TNFα cDNA (GenBank Acc. No. X57321) by PCR and the PCR-amplified fragment was purified (QIAquick gel extraction kit, Qiagen GmbH) and used as template in the subsequent in vitro transcription reaction. Digoxigenine (DIG)-labeled probes for in situ hybridization were generated according to manufacturer’s recommendations (DIG RNA labeling kit; Roche Diagnostics, Mannheim, Germany). Labeling kit included DIG-UTP in the nucleotide mix, T3 or T7 RNA polymerase (Ambion Inc., Austin, TX, USA) and 5 μl RNA template.
For non-radioactive in situ hybridization, formalin-fixed, paraffin-embedded myocardial tissue sections were rehydrated, digested with proteinase K for 3 min at room temperature and after washing, dehydrated in 100% alcohol for 1 min. The probes were diluted in hybridization solution (1:100; DakoCytomation GmbH) and hybridized onto sections in a humidified chamber overnight at 47 °C. After hybridization, sections were placed into a low stringency wash for 2 × 5 min (1% saline sodium citrate, SSC) at room temperature and a high stringency wash for 2 × 10 min (0.2% SSC) at 50 °C. Slides were then placed for 3 × 5 min in 1% TBS-Tween buffer (Roth GmbH, Karlsruhe, Germany), followed by peroxidase-blocking (DakoCytomation GmbH) for 20 min. After washing, antibody diluent (DakoCytomation GmbH) was added for 30 min, and slides were incubated overnight at 4 °C in a humidified chamber with polyclonal rabbit Anti-DIG/HRP (1:300; DakoCytomation GmbH). After washing, slides were covered with 3,3′-diaminobenzidine for 2–3 min and staining was stopped with distilled water. The slides were counterstained with hemalaun, coverslipped and analyzed using light microscopy (BH2, Olympus, Hamburg, Germany).
2.8 Statistical analysis
Data were expressed as mean ± standard error of the mean (mean ± SEM). Two-way analysis of variance (ANOVA) with Fisher’s LSD procedure for post hoc repeated measurements was used to analyze intra- and intergroup differences. Myocardial TNFα immunohistochemical score was compared by t-test between regions. p-values ≪0.05 were considered statistically significant. Data were analyzed with Statistica 6.1 (Statsoft GmbH, Hamburg, Germany).
3 Results
3.1 Systemic hemodynamics and coronary blood flow
HR was increased after CPB but not different between groups (Table 1 ). CVP, LAP, LVPed, and CBF remained unchanged throughout the protocol in both groups. MAP and LVPsys in the PLA group decreased at the end of the protocol. In the PLA group, dP/dtmax was decreased at 4 h and 8 h after CPB and at 8 h also lower than in the MP group. Cardiac index progressively declined in the PLA group after termination of CPB, and was greater in the MP group at 4 h and 8 h after termination of CPB (Fig. 1 ).
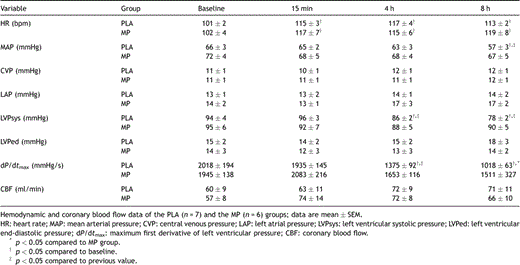
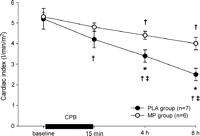
Cardiac index decreased progressively in the PLA group after CPB and was lower at 4 h and 8 h after termination of CPB compared to the MP group; data are mean ± SEM; †p ≪ 0.05 compared to baseline; ‡p ≪ 0.05 compared to previous value, *p ≪ 0.05 compared to MP.
3.2 Regional myocardial contractile function
Systolic wall thickening progressively decreased in the PLA group. In the MP group, systolic wall thickening decreased at 15 min after CPB and remained constant thereafter (Table 2 , Fig. 2 ).
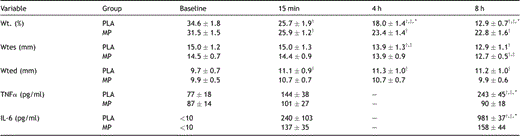
Regional myocardial contractile function and systemic cytokine concentrations
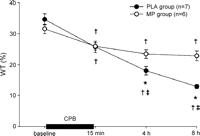
Systolic wall thickening (WT%) decreased progressively in the PLA group after CPB but remained unchanged after CPB in the MP group; data are mean ± SEM; †p ≪ 0.05 compared to baseline; ‡p ≪ 0.05 compared to previous value, *p ≪ 0.05 compared to MP.
3.3 Systemic cytokine concentrations and myocardial TNFα
Baseline systemic TNFα plasma concentrations were similar in both groups and remained at baseline levels 15 min after CPB (Table 2; Fig. 3 ). At 8 h after termination of CPB, systemic TNFα remained unchanged in the MP group, but increased in the PLA group (Fig. 3). Time course of systemic IL-6 release was similar to TNFα. Before CPB, IL-6 concentration was not detectable (≪10 pg/ml) but increased at 15 min in both groups without reaching statistical difference (Table 2). At 8 h after CPB, IL-6 was significantly increased in PLA when compared to baseline and the MP group (p ≪ 0.05).

Systemic TNFα-plasma concentrations increased at 8 h in PLA, but not in MP; data are mean ± SEM; †p ≪ 0.05 compared to baseline; ‡p ≪ 0.05 compared to previous value; *p ≪ 0.05 compared to MP.
Normalized TNFα/ß-actin ratio at 15 min after CPB increased in PLA without statistical difference when compared to baseline and MP group (PLA 1.75 ± 0.89 vs MP 0.38 ± 0.10; p = 0.33). In the PLA group, myocardial TNFα increased at 8 h after CPB. Treatment with methylprednisolone abolished the TNFα increase at 8 h after CPB (Fig. 4 ).

Normalized, myocardial TNFα protein (TNFα/ß-actin ratio) increased at 8 h after CPB in PLA, but not in MP; data are mean ± SEM; †p ≪ 0.05 compared to baseline; *p ≪ 0.05 compared to MP group.
3.4 Immunohistochemistry for TNFα
Semi-quantitative immunohistochemical scoring for myocardial TNFα at baseline was from absent to rare in both groups. At 8 h after CPB, TNFα was demonstrated predominantly in cardiomyocytes. Scoring for TNFα was marked in the PLA group (p ≪ 0.05 compared to baseline) and rare to moderate in the MP group (n.s. compared to baseline) at 8 h after CPB (Fig. 5 ).
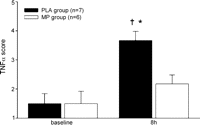
Immunohistochemical scoring for TNFα showed marked myocardial TNFα staining in the PLA group at 8 h after CPB; data are mean ± SEM; †p ≪ 0.05 compared to baseline; *p ≪ 0.05 compared to MP group.
3.5 In situ hybridization for TNFα mRNA
To investigate the origin of TNFα, immunolocalization in myocardial tissue was performed by in situ hybridization for TNFα mRNA. In situ hybridization demonstrated TNFα mRNA staining with predominant localization to cardiomyocytes, which was more pronounced in the PLA group than in the MP group (Fig. 6 ).
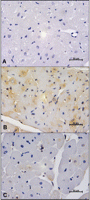
Representative photomicrographs of in situ hybridization of TNFα in left ventricular tissue at 8 h after CPB. (A) In situ hybridization with sense probe (negative control) in PLA group; (B) strong TNFα mRNA staining was revealed in cardiomyocytes of PLA with antisense probe; (c) less pronounced myocardial TNFα mRNA staining in MP group with antisense probe.
3.6 Morphology
In both groups, light microscopy did not reveal any areas of necrotic foci or infarction. Using electron microscopy, a slight intracellular edema was detected in cardiomyocytes in both groups without further ultrastructural alterations. There was no evidence of irreversible cellular damage 8 h after CPB.
4 Discussion
Compromised left ventricular function due to acute ischemia in patients with unstable angina referred for urgent coronary artery bypass grafting is a strong predictor of postoperative low cardiac output failure [1,2]. To investigate this clinical scenario we established an experimental model with progressive loss of myocardial contractile function in the absence of impaired coronary blood flow and irreversible myocardial damage [3].
In the present study, we observed during the first hours after CPB and cardioplegic cardiac arrest a progressive loss of myocardial contractile function in the placebo group which resulted in low cardiac output failure 8 h after CPB. This myocardial dysfunction was associated with increases of systemic and myocardial TNFα. Anti-inflammatory pre-treatment with methylprednisolone attenuated progressive myocardial dysfunction and abolished the increases in TNFα.
CPB and cardioplegic cardiac arrest are known to induce a systemic inflammatory response [4] which has been associated with myocardial and respiratory dysfunction, higher postoperative inotropic support and perioperative complications [5,6]. In patients with preoperatively compromised left ventricular function, a more pronounced release of pro-inflammatory cytokines has been observed, resulting in further deterioration of postoperative myocardial function and low cardiac output syndrome [7]. In addition to a systemic inflammatory response, a local myocardial inflammation has been reported after cardiac surgery with CPB [11]. Myocardium is a major source of inflammatory cytokines and capable of producing TNFα after ischemia–reperfusion [12], coronary microembolization [13], and after cardiac surgery [10,11]. In the present study, myocardial dysfunction was accompanied in the placebo group by an elevation of systemic TNFα-plasma concentrations at 8 h after CPB. Thus myocardial dysfunction can be partially attributed to the systemic IL-6 and TNFα-release. Furthermore, elevated TNFα in myocardial biopsies, as measured by immunohistochemical scoring and Western blot, indicate a pronounced local, myocardial inflammation in the placebo group after CPB. Increased myocardial expression of TNFα has been observed after CPB in human atrial and ventricular tissue [10,11] with localization to the interstitial cells and cardiomyocytes [10]. Immunohistochemistry and immunolocalization of TNFα in the placebo group revealed a predominant localization of TNFα to the cardiomyocytes, implying an autocrine response and ongoing myocardial inflammation with potential effects on postoperative myocardial function. With coronary microembolization, a perfusion–contraction mismatch with a similar time course of contractile dysfunction has been observed [14]. A causal role for myocardial TNFα [13], localized to infiltrating leucocytes and more markedly to viable cardiomyocytes, in the progressive dysfunction has been demonstrated, acting through a signal transduction sequence of nitric oxide and sphingosine [15]. In these experiments, intracoronary infusion of exogenous TNFα induced dysfunction in the absence of microembolization and conversely pre-treatment with TNFα-antibodies prevented dysfunction due to microembolization [13]. Indeed, TNFα inhibits cardiac myocyte adrenergic responsiveness and induces a negative inotropic effect on the hamster papillary muscle [16]. Direct infusion of recombinant human TNFα exerts a biphasic depression of myocardial contractility in conscious dogs [17], which is mediated in the early phase by sphingosine with inhibition of calcium (Ca2+) release by the sarcoplasmatic reticulum and in the late phase through a NO-dependent decrease of sensitivity of myofilaments to Ca2+[18]. Furthermore, TNFα enhances myocardial dysfunction by activating the ubiquitous transcription nuclear factor-κB (NF-κB) which leads to expression of genes, including those encoding synergistically acting cytokines such as interleukin-1β and interleukin-6 [19].
Glucocorticoids have been used due to their potential cardioprotective and anti-inflammatory effects in the treatment of a wide variety of diseases. In cardiac surgery steroid administration has been suggested to prevent postoperative low-cardiac-output syndrome and to reduce ischemia–reperfusion injury after cardiac surgery [5,9]. However, their role in improving myocardial performance and clinical outcome after cardiac surgery still remains controversial [20]. In the present study, treatment with methylprednisolone prevented progressive myocardial dysfunction and a decrease of cardiac index, and it preserved global and left ventricular hemodynamics after CPB and cardioplegic cardiac arrest. Additionally, systemic TNFα-plasma concentrations and myocardial TNFα-accumulation were attenuated by methylprednisolone. Consistent with our results, TNFα-induced progressive myocardial dysfunction after coronary microembolization was prevented by anti-inflammatory treatment with methylprednisolone in acutely and chronically instrumented dogs [21]. Intramyocardial activation of NF-κB with degradation of the inhibitory protein-κB (IκB) occurs after cardiac surgery [11,19] and is associated with an increased myocardial expression of TNFα, which in turn may contribute to postoperative myocardial depression and injury [11,19]. Thus, beyond inhibition of the systemic inflammatory cytokine release [5,9], attenuation of the postoperative intramyocardial inflammation observed in this study after glucocorticoid treatment may be explained by the direct inhibitory properties of steroids on the NF-κB signalling pathway through stabilization of IκB [22]. Additionally, steroids reduce leukocyte activation [5,9] and induce IL-10, an anti-inflammatory cytokine that is known to block NF-κB activity through inhibition of IκB phosphorylation and NF-κB DNA binding [4].
The present study demonstrates that progressive myocardial contractile dysfunction after CPB and cardioplegia is associated with a pronounced systemic inflammatory response and myocardial release of TNFα and that it can be attenuated by methylprednisolone treatment. Whether the local myocardial anti-inflammatory effects of glucocorticoid treatment are mediated through the NF-κB signalling pathway and if this experimental scenario can be exploited in patients undergoing cardiac surgery with cardiopulmonary bypass and cardioplegic cardiac arrest remain to be established in further studies.
Acknowledgment
This study was partially funded by a research grant (Nr. 1400750/2002) of the University of Goettingen, Germany.
References
- anti-inflammatory agents
- aorta
- cardiac myocytes
- cardiac arrest
- myocardium
- ischemia
- cardiopulmonary bypass
- hemodynamics
- cardiac surgery procedures
- immunohistochemistry
- left ventricle
- reperfusion therapy
- physiologic reperfusion
- myocardial dysfunction
- glucocorticoids
- methylprednisolone
- muscle contraction
- necrosis
- plasma
- rna, messenger
- suidae
- coronary artery flow
- cardiac index
- normal saline
- aortic cross clamping
- myocardial neoplasms
- saline solutions
- attenuation
- prevention