-
PDF
- Split View
-
Views
-
Cite
Cite
Tor Steensrud, Øyvind Jakobsen, Kirsti Ytrehus, Dag G. Sørlie, Contractile recovery of heart muscle after hypothermic hypoxia is improved by nicorandil via mitochondrial KATP channels, European Journal of Cardio-Thoracic Surgery, Volume 30, Issue 2, August 2006, Pages 256–262, https://doi.org/10.1016/j.ejcts.2006.04.033
- Share Icon Share
Abstract
Background: The ATP-sensitive potassium channel (KATP) opener nicorandil used instead of potassium in hypothermic cardioplegia significantly improves preservation of cardiac function and energetics in the in situ heart preparation. The present study, therefore, examines the effect of nicorandil at different temperatures and the role of sarcolemmal and mitochondrial KATP channels under ex vivo conditions using contractile force (CF) and action potential duration (APD) as end points. Methods: Guinea-pig papillary muscles at 37, 27, or 22 °C (1 Hz) were exposed to nicorandil 0.2–1.1 mM. The contributions of KATP channel subtypes in cardioprotection were examined using mitochondrial (mito) (0.1 mM) or non-selective (1.0 mM) concentrations of nicorandil, mito KATP blocker 5-hydroxyl decanoate (5HD, 300 μM) or sarcolemmal (sarc) KATP blocker HMR1098 (30 μM) before and during 140 min of hypothermic (22 °C) glucose-free hypoxia. Results: Nicorandil >0.5 mM shortened the APD, and this was abolished by hypothermia and HMR1098 but not by 5HD. Nicorandil in both tested concentrations preserved contractile force after hypoxia–reoxygenation significantly better than control (73.7 ± 4.4% and 75.8 ± 3.9% vs 40.6 ± 2.6%, n = 6 in each group). Protection was blocked by 5HD but not by HMR1098. 5HD and HMR1098 alone did not change recovery of contractile force compared to control. Conclusion: Shortening of APD and activation of sarc KATP by nicorandil were not related to myocardial protection. Thus, the mito KATP seems to play a significant role in cardioprotection compared to the sarc KATP also when substrate depletion and hypoxia are combined with hypothermia.
1 Introduction
Hypothermic, hyperkalemic cardioplegic arrest continues to be the standard method for myocardial protection in cardiac surgery when using cardiopulmonary bypass. The heart is arrested by partial depolarization of the cell membranes using high extracellular concentrations of potassium (16 mmol/l). Cooling is normally applied, both systemically (∼32 °C) and locally at the heart (8–12 °C). Hypothermia has several effects on the electrophysiology of the heart. With respect to cardiomyocytes, the action potential duration (APD) is increased and the resting membrane potential is slightly decreased (towards a more positive value) [1]. The potassium channel opener nicorandil has demonstrated cardioprotective effects [2] and has also been used as an additive to [3] and pretreatment [4] before cardioplegic solutions. With respect to cellular electrophysiology, potassium channel opening in general have been reported to have the opposite effects of those of hypothermia, namely a shortening of the APD and (hyper)polarizing the membrane potential (towards a more negative value). The KATP channel activator bimakalim reverses the hypothermia-induced action potential lengthening [5].
Cardiac myocytes contain two distinct types of KATP channels: those of the sarcolemma (sarc KATP) and those of the mitochondria (mito KATP) [6]. Activation of these channels with potassium channel openers has potent cardioprotective properties in a number of models and species [7]. This cardioprotective effect was initially attributed to sarcolemmal KATP channels and their ability to shorten the action potential duration and to polarize the membrane potential. However, others have shown that the cardioprotective effect of the KATP channel openers could be separated from the shortening of the action potential duration [8,9]. More recent studies, therefore, suggest that the mito KATP channels rather than the sarc KATP channels are involved in the cardioprotection offered by potassium channel openers [7,10]. It has been shown that the protection against ischemia-induced arrhythmias and cell death by KATP channel activator nicorandil is blocked by the mitochondrial KATP blocker 5-hydroxy decanoate (5HD) but not by the sarcolemmal blocker HMR1098 [11]. In another study, however, both sarc KATP channels and the mito KATP channels were involved in the anti-infarct effect afforded by nicorandil [12].
Nicorandil has a concentration-dependent mode of action in which 0.1 mM activates mito KATP channels only and 1 mM activates both sarc and mito KATP channels [11]. Nicorandil is a hybrid agent that also has nitrate properties [13]. We have previously shown that 0.1 mmol/l nicorandil instead of high concentration of potassium in a cold cardioplegic solution improved recovery of cardiac function and energetics in the in situ pig heart [14].
The aim of the present study was to investigate the cardioprotective mechanisms at the cellular level by studying the effect of nicorandil upon contractile force (CF) and action potential duration under substrate-free hypoxia in combination with hypothermic conditions and under hypothermia alone in an in vitro heart muscle preparation.
2 Methods
2.1 Preparation
The experimental protocol was approved by the Norwegian Animal Experiments Authority and in accordance with ‘The European convention for the protection of vertebrate animals used for experimental and other scientific purposes’ (Council of Europe No.125, Strasbourg 1985). Guinea pigs weighing 250–500 g (Dunkin Hartley, Taconic M&B) were anesthetized with intraperitoneal injection of pentobarbital (100 mg/kg). The hearts were rapidly excised and transiently perfused via the aorta with an oxygenated Krebs–Henseleit bicarbonate buffer of the following composition (in mM); Na+ 141, K+ 5, Ca2+ 2, Mg2+ 1, Cl− 131, H2PO4− 1.2, HCO3− 20 and Glucose 10. This solution was gassed with a mixture of 95% O2 and 5% CO2 to give a pH of 7.4 at 25 °C. Buffer O2 saturation and pH were controlled throughout the experiments regularly with a blood gas analyzer (Ciron Diagnostics, Ciba, MA, USA). A slim right ventricular papillary muscle (diameter ≪ 1mm) was rapidly dissected and pinned with a platinum electrode in a 5-ml tissue bath (37 °C) for superfusion (2 ml min−1) with Krebs–Henseleits buffer. The other end of the preparation was connected with a steel hook to a miniature quartz force transducer (FORT 10, WPI, Hertfordshire, UK) and a signal amplified (Universal amplifier, Gould Instrument Services, Madison, WI, USA). Preparations were paced at 1 Hz using a function generator (Velleman PCG10, Gaverre, Belgium) with accompanying software (Pc-Lab 200™). Stimuli of 1 ms duration were given throughout the experiment via a pair of closely spaced platinum electrodes, and the amplitude was set at 1.5 times above that of the minimum activation current. The preparations were stretched to produce maximum amplitude of contractile force. Transmembrane action potentials were recorded using conventional glass microelectrodes filled with 3 M KCl and having tip resistances about 20 MΩ. The microelectrodes were connected using an Ag–AgCl half-cell (MEH2R15, WPI, Sarasota, FL, USA) to the input of a high impedance electrometer (Model M-4A, W-P Instruments Inc., New Haven, CT, USA). Action potentials and developed force were displayed on an oscilloscope and stored on a personal computer and further processed with an in-house developed software program (Arild Danielsen). Preparations were allowed to stabilize at 37 °C for 60 min during which a cell within each preparation was impaled with a microelectrode. Inclusion criteria were as follows: baseline contractile force amplitude between 0.4 and 1.6 mN, action potential amplitude minimum 110 mV and resting potential minimum −70 mV. The temperature of the superfusate was controlled with a thermocouple in the bath connected to a digital thermometer and regulated using a circulating water bath (RTE210, Neslab, Newington, NH, USA) capable of heating and cooling.
2.2 Protocols
2.2.1 Protocol 1
This protocol was designed to observe changes in action potential duration and contractile force with varying concentrations of nicorandil during normothermia (37 °C), moderate (27 °C), and deep hypothermia (22 °C). APD and contractile force were recorded. In this protocol, six preparations were used in each group. After recording of baseline function, preparations were exposed to increasing concentrations of nicorandil from 0.1 to 1.1 mM. These preparations were not exposed to hypoxia.
2.2.2 Protocol 2
This protocol involved hypoxia–reoxygenation. After recording of the baseline action potential characteristics and contractile force, preparations were divided into eight groups: (1) no drug (control group n = 6); (2) 0.1 mM nicorandil (n = 5); (3) 1 mM nicorandil (n = 6); (4) 0.1 mM nicorandil and 300 μM of the selective mitochondrial KATP blocker sodium 5-hydroxy decanoate (n = 5); (5) 1 mM nicorandil and 300 μM 5HD (n = 4); (6) 300 μM 5HD alone (n = 4); (7) 1 mM nicorandil and 30 μM of the selective sarcolemmal KATP blocker HMR1098 (n = 6); and (8) 30 μM HMR1098 alone (n = 3). The KATP channel blockers 5HD and HMR1098 were allowed to superfuse for 10 min before adding nicorandil, which then was perfused for another 10 min [15].
Preparations were then rapidly cooled (5 min) to 22 °C and exposed to 140 min of substrate free hypoxia at 22 °C. Pilot experiments showed that 140 min paced hypoxia was necessary to achieve an approximately 40% reduction of contractile force in controls. Hypoxia in combination with substrate depletion was induced by using a buffer gassed with 95% N2 and 5% CO2 where glucose was exchanged with d-sorbitol. The superfusion chamber was covered with Argon gas. These interventions lowered pO2 to ≪5 kPa. Drug superfusion was continued throughout the hypoxic period. Electrical stimulation was maintained throughout the experiment. After 140 min, each preparation was re-warmed in the course of 30 min to 37 °C while again superfused with oxygenated glucose containing buffer (Krebs-Henseleit). Recovery of contractility was assessed 30 min after reoxygenation.
2.3 Chemicals
Nicorandil was a gift from Torrent Pharmaceuticals Inc., India. HMR1098 was a gift from Aventis, Germany. All other chemicals were purchased from Sigma-Aldrich Chemical Co.
2.4 Statistics
Data are presented as mean ± SD unless stated otherwise. Normality distribution of data was assessed with normal score plots of residuals. Data were analyzed using analysis of variance (ANOVA) with Tukey post hoc test and analysis of variance for repeated measurements (RANOVA) when appropriate, using the statistical software package SPSS (SPSS10.0©, SPSS Inc., IL, USA). Values of p ≪ 0.05 were considered significant.
3 Results
3.1 Protocol 1
At 37 °C and normoxic conditions, increasing the concentration of nicorandil shortened the APD significantly. Reducing the temperature from 37 to 27 °C and 22 °C resulted in a marked increase in APD90 and to a reduced effect of nicorandil on APD shortening (Fig. 1a ).
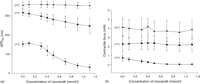
(a) Relationship between duration of action potential (APD90) in milliseconds (ms) and increasing concentrations of nicorandil from 0.1 to 1.1 mmol/l at three different temperatures: 37 °C (circles), 27 °C (squares) and 22 °C (triangles), protocol 1. n = 6 for each concentration and temperature tested, bars denote SD. (b) Relationship between contractile force (CF) in millinewton (mN) and increasing concentrations of nicorandil from 0.1 to 1.1 mmol/l at three different temperatures; 37 °C (circles), 27 °C (squares) and 22 °C (triangles), protocol 1. n = 6 for each concentration and temperature tested, bars denote SD.
In normothermic and normoxic preparations, the contractile force was reduced when the concentration of nicorandil was increased. The reduction was from 0.74 ± 0.13 mN (n = 6) at 0 mM nicorandil to a minimum of 0.04 ± 0.003 mN (n = 6) at 0.9 mM nicorandil. The effect of the compound upon CF disappeared when the temperature was lowered to 27 and 22 °C (Fig. 1b).
3.2 Protocol 2
When adding 1 mM nicorandil with or without mito KATP channel blocker 5HD the APD90 shortened significantly (Table 1 ). The control group, the 0.1 mM nicorandil group and the 1 mM nicorandil + sarc KATP channel blocker HMR1098 group behaved similarly with respect to the APD90. In these groups the APD was not shortened when the drug was added and there was no negative inotropy in the pre-hypoxic period. Adding the sarc KATP channel blocker HMR1098 inhibited both APD shortening and the negative inotropy observed in the 1 mM nicorandil-treated group (Fig. 2a and b , Table 2 ). The mito KATP channel blocker 5HD did not affect the action potential shortening or the negative inotropic effect of 1 mM nicorandil. The blockers HMR1098 or 5HD alone did not affect the action potential duration or the contractile force. The resting membrane potential was in the 1 mM nicorandil group at baseline −79.7 ± 2.9 mV, 5 min after starting mM nicorandil −82.0 ± 2.5 mV and 10 min after −83.0 ± 2.7 mV.
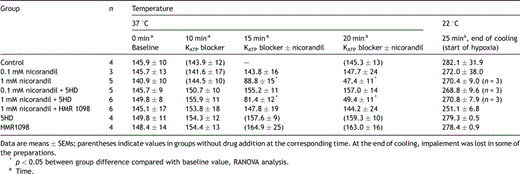
Action potential duration (ms) at 90% repolarization (APD90) at 1 Hz: effects of nicorandil and/or blockers followed by cooling to 22 °C against control in guinea pig papillary muscles
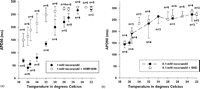
Changes in action potential duration (APD90) during cooling in guinea pig papillary muscle. Data are means ± SDs, n denotes number of papillary muscles with stable microelectrode impalement at the corresponding time-point (protocol 2, see text). (a) Effects of 1 mM nicorandil with (open circles) or without (closed circles) sarcolemmal KATP channel blockade (HMR1098). (b) Effects of 0.1 mM nicorandil with (open squares) or without (closed squares) mitochondrial KATP channel blockade (5HD).
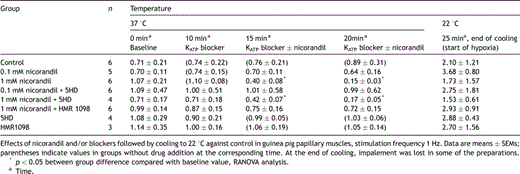
Nicorandil in both tested concentrations (0.1 and 1.0 mM) preserved contractile force after hypoxia–reoxygenation significantly better than control (73.7 ± 13.11% and 75.8 ± 7.4% vs 40.6 ± 2.6% of baseline, n = 6 in each group). When the mito KATP channel blocker 5HD (300 μM) was added 10 min prior to nicorandil, the preservation of contractile recovery was abolished. Recovery of baseline values were 42.9 ± 12.3% in the 0.1 mM nicorandil + 5HD group and 40.7 ± 13.1% in the 1.0 mM nicorandil + 5HD group. The sarc KATP channel blocker HMR1098 (30 μM) did not alter the cardioprotection offered by 1.0 mM nicorandil significantly. Contractile force was in the HMR1098 + 1.0 mM nicorandil group 63.6 ± 3.7% of baseline, not significantly different from the 0.1 mM nicorandil group or the 1.0 mM nicorandil group (Fig. 3 , Table 3 ).
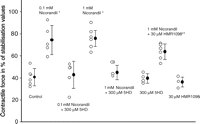
Contractile force in percentage of stabilization values after 140 min of substrate (glucose) free hypothermic hypoxia followed by 30 min recovery. Open circles denote individual experiments, closed circles mean and bars are SD. ANOVA with Tukey post hoc analysis showed **p = 0.002 versus control, *p ≪ 0.001 versus control (protocol 2, see text).
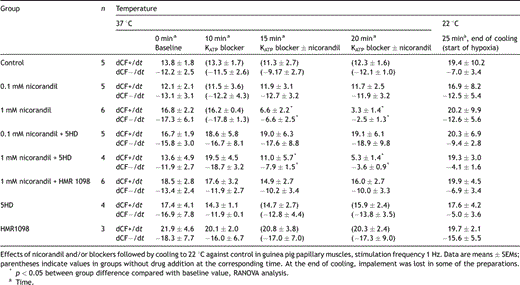
The maximum rate of contraction (dCF+/dt) and relaxation (dCF−/dt)
4 Discussion
Nicorandil at a concentration of 0.1 or 1.0 mM preserves contractile force in the guinea pig papillary muscle after hypoxia and reoxygenation also when combined with hypothermia. This protection was abolished with the mitochondrial KATP channel specific blocker 5HD but not with the sarcolemmal KATP channel blocker HMR1098 indicating that opening of mitochondrial KATP channels are responsible for the protection. Examination of action potential duration supported this conclusion since protection was independent of shortening of action potential duration.
Adding 1 mM nicorandil during normothermia (37 °C) markedly shortened APD, but when 30 μM HMR1098 was present this shortening of APD was abolished. This suggests that 1 mM nicorandil activate channels in the cell membrane, probably the sarc KATP channels, and that HMR1098 blocks these channels. The 10-fold lesser concentration of nicorandil (0.1 mM) had no effect on the APD. This finding supports the statement by Sato et al. [11] that 0.1 mM nicorandil is insufficient to affect the sarc KATP channels.
The mechanism of cardioprotection by KATP channel activation has been debated. To begin with, cardioprotection was believed to be due to shortening of the action potential and thereby reducing calcium overload and hence improve functional recovery after reperfusion. The ability to (hyper) polarize the cell membrane has also been proposed as a potential mechanism of cardioprotection since the vessel wall smooth muscle cell relaxation, which characterize compounds classified as KATP channel activating compounds is due to hyperpolarization [16]. With respect to cardioplegic arrest, maintaining ionic cellular balance at resting membrane potential could be favorable since lesser energy has to be used in the reperfusion period to restore ionic homeostasis compared with standard hyperkalemic depolarized arrest. Several studies including this one are challenging these hypotheses. Pre-treatment with 0.1 mM nicorandil was as good as ischemic preconditioning in preserving myocardial function after cold cardioplegic arrest in isolated rat hearts [17]. Other studies have shown that sarc KATP channels contribute to cardioprotection; a study of isolated hearts from Kir6.2-knockout mice lacked the protective effects of the KATP channels activator diazoxide [18], and a study of isolated rat myocytes showed sarc KATP channels to be necessary for diazoxide protection [19]. A question of a role of Ca2+-activated K-channels in smooth muscle cell relaxation as well as in cardioprotection has also been raised and related to nicorandil in the case of vasodilatation [20]. In our study the myocardial protection offered by nicorandil was not significantly changed when the sarc KATP channels were blocked with the selective blocker HMR1098. Also, action potential shortening was not necessary to induce myocardial protection as shown in the 0.1 mM nicorandil group (Fig. 3). A mito KATP channel selective, lower concentration of nicorandil (0.1 mM) was sufficient for cardioprotective effects. Our study thus defies the hypothesis that action potential shortening, resting membrane hyperpolarization and sarcolemmal KATP channel activation are dominant mechanisms in the cardioprotection offered by nicorandil. The mechanism behind protection afforded by mitochondrial KATP channel opening has been studied at the cellular and mitochondrial level [10,21]. Proposed mechanisms are mitochondrial inner matrix swelling due to potassium flux and reactive oxygen species (ROS)-signalling followed by cell adaptation and protection against mitochondrial permeability transition caused by ischemic injury.
Contractile force was substantially reduced during normothermia (37 °C) with 1 mM nicorandil and with 5HD plus 1 mM nicorandil. In the groups with 0.1 mM nicorandil or 1 mM nicorandil with HMR1098, contractile force was not significantly altered. The concentration-dependent negative inotropism observed when adding 1 mM nicorandil is comparable to the findings of others [22] and in concordance with the shortening of the action potential duration. The dose–response relationship with respect to the vasculature and smooth muscle cell relaxation triggered by cell membrane potassium channels and the activation of cardiomyocyte sarcolemmal KATP channels responsible for shortening action potential duration seems to be corresponding [16].
During normothermia (37 °C), nicorandil alone at concentrations above 0.5 mM depress contractile force. However, when the temperature was lowered nicorandil did not affect contractile force even at high concentrations. Nicorandil is thus cardiodepressive during normothermia but this effect diminishes at 27 and 22 °C (Fig. 1b). Nicorandil at concentrations above 0.3 mM shortens the APD during normothermia and at 27 °C above 0.9 mM (Fig. 1a). However, when the temperature was lowered to 22 °C nicorandil did not shorten the APD even at concentrations up to 1.1 mM. Thus, there is dissociation between the effect upon APD and contractile force during temperature reduction. No effects of nicorandil were detected at deep hypothermia. Hypothermia affects APD and CF markedly and any possible effect of the compound could have been masked or counteracted. It is also noteworthy that KATP-dependent vessel wall relaxation is temperature dependent [23].
This experimental model and the protocol used have some limitations. To simulate a clinical setting of heart surgery, cooling was fast (5 min) and re-warming was slow (30 min). The APD and CF measurements at 22 °C displayed a large variability within and between groups. One explanation could be that some preparations were not stable due to the rapid cooling. The present study assessed the duration of the action potential (APD) better than the resting membrane potential. We detected hyperpolarization when adding 1.0 mM nicorandil at 37 °C but not with 0.1 mM. The cooling after drug addition increased the force and interfered with the resting membrane potential measurements. The membrane potentials increased to a level of about −60 mV during hypothermia (data not shown). Thus, the extent of possible hyperpolarization during cooling due to the sarc KATP channel opening by nicorandil was not addressed directly in this study. It was, however, no change in resting membrane potential with 0.1 mM nicorandil and with 1.0 mM nicoradil plus HMR1098 during normothermia, and we conclude that the protection most likely is independent of hyperpolarization. Another limitation of the present study when related to the clinical situation is the use of a preparation stimulated to contract during the hypothermic hypoxia. This was done to reach approximately 40% reduction in contractile force at reoxygenation within the timeframe of the overall stability of the preparation, but may have introduced an extra calcium load. Also, since the endpoint of this study was contractile force we were not able to tell whether the improved contractile recovery was due to reduction of stunning or a reduction of cell death. This distinction is important for future clinical use. Based on multiple previous studies showing that observed protection against ischemic injury which depends on mito KATP channels is strongly related to reduction in cell death it is tempting to suggest that the improved contractile force is due to reduced cell death also in our preparation [7,24]. It was difficult to obtain stable monoimpalements during cooling in all preparations due to the sudden increase in contractile force induced by fast cooling. Results given are from preparations with stable impalement, and in some experiments a new impalement was made at the end of the reperfusion. In some experiments we were not able to measure APD at every interval and therefore the numbers reported vary between groups. We started in all groups with six preparations except in groups with blocker HMR1098 and 5-HD, in which we had only four.
Aortic cross clamping followed by release in cardiac surgery is a situation with planned hypothermia, hypoxia and reperfusion and therefore an ideal situation for the use of a cardioprotective agent such as nicorandil. However, Galinanes et al. [25] have shown that lemakalin, a potassium channel opener, was cardioprotective when given alone but not when added to a potassium-containing cardioplegic solution. We have shown that recovery after hypothermic substrate free hypoxia was improved by the nicorandil 0.1 mM in spite of no detectable functional effects of the compound at hypothermic temperature. Also, 0.1 mM nicorandil add cardioprotective effects during hypothermia in guinea pig papillary muscle ex vivo corresponding to results from anesthetized in situ pig hearts. Therefore, nicorandil as an adjunct to a hypothermic cardioprotective solution may have important clinical applications in providing added myocardial protection despite not having cardioplegic effects by itself in this situation.
5 Conclusion
Nicorandil in both tested concentrations (0.1 and 1.0 mM) preserved contractile force after hypothermic hypoxia and reoxygenation significantly better than in controls and in groups treated with the mito KATP blocker 5HD. Nicorandil in concentrations up to 1.1 mM did not arrest the papillary muscle during hypothermia (27 or 22 °C). Neither the sarcolemmal effects of nicorandil, the shortening of the action potential, nor the negative inotropism at higher concentrations, seem related to the cardioprotective effect. The mito KATP play a major role compared to the sarc KATP in cardioprotection also when the myocytes have been subjected to hypothermia.
Acknowledgements
This study was supported in part by a grant from the Norwegian Council of Cardiovascular Diseases. We would like to thank the staff at the Department of Medical Physiology at the University of Tromsø for technical assistance.