-
PDF
- Split View
-
Views
-
Cite
Cite
Tara Karamlou, Ross M. Ungerleider, Bahaaldin Alsoufi, Grant Burch, Michael Silberbach, Mark Reller, Irving Shen, Oversizing pulmonary homograft conduits does not significantly decrease allograft failure in children, European Journal of Cardio-Thoracic Surgery, Volume 27, Issue 4, April 2005, Pages 548–553, https://doi.org/10.1016/j.ejcts.2004.12.054
- Share Icon Share
Abstract
Objective: Placement of oversized pulmonary ventricle–pulmonary artery conduits is routinely performed to decrease conduit failure in children. However, this practice has recently been challenged as somatic outgrowth may not be the main determinant of allograft failure in children. Our objective was to determine whether placement of oversized homografts for extracardiac pulmonary ventricle (PV) outflow tract reconstruction improves longevity in young children. Methods: We reviewed 102 consecutive PV–PA conduits inserted in 70 patients less than 18 years between 1984 and 2003. Conduits placed in an anatomic position (n=23) as part of a Ross operation, were excluded. Conduits were initially stratified into two age groups: Group 1, those placed in patients ≦10 years, and Group 2, those placed in patients ≫10 years. Normalization of conduit size to patient's body surface area at the time of insertion (z-value) was then performed to divide the conduits into oversized (O/S) and non-oversized (NO/S) groups. Determinants of conduit failure and allograft longevity were then compared between groups. Results: Seventy-nine extracardiac conduits were placed, and 57 of these were in patients under 10 years of age. The majority had a diagnosis of tetralogy of Fallot (n=38), truncus arteriosus (n=19), pulmonary atresia with ventricular septal defect (n=12), or D-TGA with pulmonary stenosis and ventricular septal defect (n=7). Thirty-seven conduits were oversized (O/S) based on z-value, and 42 were non-oversized (NO/S), and the mean age at initial homograft placement was 7.0±7.5 years. Overall, oversizing conferred no significant advantage with respect to actuarial freedom from homograft replacement at 1, 5, or 10 years (96, 79, and 21%, O/S vs 93, 60, and 24%, NO/S), P=0.44. Oversizing was more frequent in Group 1 than Group 2 (53 vs 32%), and conduit failure was also more frequent with 49% requiring reoperation during the study period vs 38% in Group 2. In the subset of patients ≦10 years, both homograft explantation rate (50% O/S vs 48% NO/S) and median interval to conduit failure were similar between the O/S and NO/S patients (7.1 vs 4.8 years), P=0.340. Risk factors for conduit failure identified in multivariable regression analysis included the presence of pulmonary artery branch stenosis, lack of previous definitive repair, a diagnosis of pulmonary atresia, the need for percutaneous intervention. Conclusions: There is no significant benefit to placement of an oversized PV–PA homograft in this series of patients from a single institution. Even in young patients with rapid somatic growth, normalizing extracardiac allografts to BSA provides excellent conduit longevity and outcomes.
1 Introduction
Multiple studies have reported determinants of pulmonary allograft longevity in children during pulmonary ventricle outflow tract reconstruction [1–7]. Many of these earlier studies reported that smaller conduit size, and younger patient age at the time of implantation are significant risk-factors for homograft failure [1,3–5,8]. However, recent reports suggest that the routine placement of discordantly large grafts may not decrease the reintervention rate, as somatic outgrowth may not be the main determinant of conduit failure in children [9,10]. Thus, the recommended practice of placing larger homograft in young children might not improve homograft longevity. There is also increasing evidence that large conduits, especially in certain anatomic variants such as L-TGA, are more susceptible to external compression, and perhaps earlier allograft failure [3,10]. The objective of this study is therefore to determine whether oversizing of pulmonary ventricle to pulmonary artery conduits promotes increased durability in an age-stratified population that includes those at high-risk for somatic outgrowth.
2 Materials and methods
2.1 Patient characteristics
Between 1984 and 2003, 102 pulmonary homograft conduits were implanted in 70 children for reconstruction of the RVOT or to replace the pulmonary valve in conjunction with the Ross procedure at Oregon Health & Science University (OHSU). The patients were retrospectively selected from review of a large prospectively maintained database within the Cardiology division at our institution. Those patients over 18 years of age, those receiving heterografts or valveless conduits and those without follow-up were excluded from this study. We also excluded those patients receiving anatomic reconstruction as part of a Ross procedure. Demographic data, information regarding congenital and acquired cardiac malformations, preoperative treatment and interventions, operative details, and postoperative outcomes were abstracted from hospital and clinic charts. Patients, families, cardiologists, and primary care physicians were contacted when necessary to acquire current follow-up information. Transthoracic echocardiography and cardiac catheterization reports were also analyzed to determine conduit hemodynamic properties and functional status. Permission for this study was obtained from the Institutional Review Board at OHSU prior to its induction.
Normalization of homograft size to the patient's body surface area at the time of conduit insertion was then performed to divide the conduits into oversized (O/S) and non-oversized (NO/S) groups. Our protocol for normalization is described below. Then, to further explore the relationship between age and the performance of discordantly sized conduits, conduits were then stratified based on the age of the patient at the time of insertion as follows: Group 1, age ≦10 years, and Group 2, age ≫10 years. The 10-year designation was chosen based on previous data demonstrating a linear increase in homograft diameter up to age 10 years, at which point a plateau occurs [4].
2.2 Homograft sizing and survivial
Patients' height and weight was recorded at the time of initial implantation. The following formula was then used to obtain the body surface area (BSA) [11]:Fig. 5
Using a historically validated normogram [12] for pulmonary valve size indexed to BSA, a predicted pulmonary annulus size was determined. The homograft was described as oversized if it was ≫1 standard deviation from this value, and undersized or normally sized if it was either ≪1 standard deviation of this value, or within the normal range.
To determine if conduit longevity was associated with the sequence of insertion, we also stratified conduits using an ordinal variable coding for whether the homograft was the first, second, or third conduit, and compared groups using the Kaplan–Meier method followed by the log-rank test.
Homograft survival was defined as the interval between allograft implantation and the date of reoperation for homograft replacement. Patients not requiring homograft replacement for obstruction or important stenosis were treated as censored events at either the time of the last clinical follow-up or patient death. Because many patients had multiple homograft replacements, the same patient may have provided data for more than one interval, but each homograft survival interval was treated as an independent event. Homograft failure was defined as homograft replacement for stenosis or valve-related death. Important stenosis was defined as a mean Doppler gradient ≧50mmHg, or the presence of symptoms and a gradient ≧35mmHg.
2.3 Statistical analysis
Categorical variables were compared with the χ2 test or Fisher's exact test, when appropriate. Continuous variables were either dichomtomized, as in the case of age-group stratification and homograft sizing, or compared withunpaired t-tests. Survival between groups was then determined using the Kaplan–Meier method followed by the log-rank test. Two different Cox multivariable regression models were then constructed to determine those risk-factors associated with the time-related event, conduit reoperation, and two compare homograft survival between the OS and NOS groups after adjustment for significant covariates. Only those variables associated with greater than five events were selected for inclusion into the multivariable model to prevent model overdetermination. In the final model, variables with a P≪0.10 using backward selection were retained. Statistical anlaysis was performed using SAS software, v. 8.2 (Cary, North Carolina) and GraphPad Prism 4.0. A P value ≪0.05 was considered significant.
3 Results
3.1 Patient characteristics
Between 1984 and 2003, 102 cryopreserved pulmonary homografts were implanted in 70 children under 18 years of age at the Oregon Health and Science University. Of these, 79 were extracardiac conduits. Fifety-seven implantations were in patients ≦10 years of age. Within this subset, 30 were oversized allografts and 27 were non-oversized grafts. The general characteristics after age-based stratification into the two groups are shown in Table 1. Group 1, with oversized conduits, had larger allografts implanted (17.9 vs 13.7), P=0.0006 despite being utilized in younger and smaller patients, and were more likely to be placed in those with prior surgical intervention, including previous conduit implantation than those with non-oversized conduits (87 vs 59%), P=0.03. Similarly, in Group 2, the oversized conduits were utilized in patients who were slightly younger and significantly smaller than in the non-oversized group (24 vs 22.3), P≪0.0001.
3.2 Homograft survival
Actuarial event-free curves for homgraft failure for the entire cohort are illustrated in Fig. 1. The 1, 5, 10, and 15 year freedom from failure was 90, 65, 18, and 8%, respectively. Homograft replacement occurred 7 months to 23.7 years (mean 6.7 years) postoperatively in 32 patients (41%). There were six deaths during the follow-up period, for an overall mortality rate of 7.6%. Percutaneous intervention, either balloon or stent placement, was required in 11 patients for significant obstruction between 7 months and 10.9 years (mean 4.4 years). No patients with replacement homografts have required subsequent catheter-based revision of their conduit.
Actuarial event-free curves for the age-based strata are shown in Fig. 2. Freedom from homograft replacement was slightly shorter in Group 1 than Group 2 at 1, 5, and 10 years (95, 69, and 16% vs 100, 83, and 42%), P=0.13.
There was no significant advantage with respect to freedom from homograft replacement for patients having extracardiac reconstruction at 1, 5, or 10 years (96, 79, and 21%, O/S vs 93, 60, and 24%, NO/S), P=0.44, Fig. 3. In the subset of patients less than 10 years, oversizing was more frequent than in the older cohort (53%, Group 1 vs 32%, Group 2), and conduit failure was also more frequent, with 49% requiring replacement during the study period. Within Group 1, however, mean interval to conduit failure was not different between the O/S and NO/S patients (7.1 vs 4.8 years), P=0.340, Fig. 4.
Homograft longevity was independent of the sequence of insertion, Fig. 5. For an initial conduit (n=58), the median survival was 8.1 years, for a second conduit (n=28), the median survival was 7.3 years, and for a third conduit (n=5), the median survival was 6.8 years.
3.3 Multivariable analysis
The variables utilized for the Cox regression model fit for conduit reoperation included: PA branch stenosis, previous cardiac surgery, age at conduit insertion, operation before 1992, BSA, conduit size, use of an oversized conduit, presence of associated defects, sex, presence of percutaneous intervention, diagnosis of pulmonary atresia, diagnosis of tetralogy of Fallot, and initial pulmonary conduit placed (sequence 1). Oversized conduits were not associated with improved homograft longevity after adjusting for other influential factors. The results of the multivariable model are listed in Table 2.
Because oversized conduits were generally inserted in patients that were younger, were more likely to have experienced prior cardiac operation, including previous homograft implantation, a second Cox regression model was constructed to compare the two groups, OS and NOS, after adjusting for significant covariates. The same variables used in the first model, excluding oversized grafts, were entered, and the groups were stratified by the presence or absence of oversizing. Using this model, the presence of PA branch stenosis (Hazard ratio 3.3; P=0.02) and the presence of previous operation (protective) (Hazard ratio 0.09; P≪0.001) were associated with conduit reoperation. Estimated survival functions after risk-factor adjustment at 1,5, and 10 years were similar for both groups (OS: 97, 75, and 14% vs NOS: 96, 62, and 11%). The estimated survival curves (Fig. 6) were generated by substituting the mean values for these covariates, and are similar to those generated by non-parametric analysis.
4 Discussion
Homograft conduits have made the complex repair of many congenital defects possible [1–6,13–15]. However, their widespread use has focused attention on the finite lifespan of these allografts, as most patients will require multiple operations for replacement over the course of their lifetime [1–6,9,10]. Controversy exists regarding the ideal size and composition of homografts, and whether modifiable conduit-specific or patient-specific characteristics exist that could extend the freedom from allograft replacement in children.
Many studies have shown that young patients are at increased risk for failure [1–6,13]. Other authors have shown that small pulmonary arterial diameter, smaller homografts, and lower recipient weight are also important predictors of the need for conduit replacement [1–5,13,15]. These observations have led many to recommend the placement of larger conduits in small patients to compensate for somatic growth [1,4,16]. However, conflicting data is present in other reports [6,7,9,10]. Heineman and colleagues [9] reported a series of 43 neonates undergoing homograft repair of truncus arteriosus. They had only seven replacements with a mean follow-up of 31 months. They concluded that very small homografts (7–9mm) were not a risk factor for reintervention, and suggested that oversizing was not indicated even in neonates. Other studies have failed to demonstrate that young patient age (≪3 years) is an independent risk factor for allograft failure when examined by multivariable analysis [4,6,7].
It is likely therefore that other factors, other than somatic outgrowth, contribute to the observed variability in allograft longevity [9,10,13]. The recent report by Wells and colleagues [10] supports the idea that somatic outgrowth is not the main determinant of conduit failure, as only 8% of 40 patients undergoing explantation for stenosis had actually outgrown their allograft. In this series, contracture and shrinkage occurred in 96% and was the overwhelming reason that patients presented for replacement. These authors suggested that the placement of oversized conduits might actually predispose the patient to external compression and earlier repair [10]. Our results agree with these findings, as the placement of oversized conduits conferred no significant advantage with respect to longevity in younger patients at high risk for somatic outgrowth. Despite that fact that more patients in Group 1 had discordantly large homografts, there was no difference in freedom from replacement between the O/S and NO/S groups.
Increasing evidence shows that immune-related mechanisms play a crucial role in allograft survival and may provide alternative explanations why conduit failure is more frequent in younger children [17–20]. Heightened cell-mediated responses exist in children, and have recently been correlated with accelerated degeneration of cryopreserved homografts [17,20]. In addition, conduits harvested from younger donors may be more immunologically active, thus predisposing younger recipients to early failure [6,13]. Extracardiac placement of the allograft, as opposed to anatomic reconstruction as part of a Ross procedure, might also accelerate conduit degeneration and contracture, as external compressive forces increase turbulence and annular distortion [10]. Discordantly large homografts are vulnerable to such distortion from sternal compression, kinking, and posterior shelf impingement, especially in certain anatomic variants, which require substernal placement [10].
The location of prosthesis failure, although important, could not be determined from our data. However, in our clinical experience, the failures most commonly occur at the level of the valve, as a result of progressive calcific stenosis. This has been confirmed by other reports [21], and lends support to the idea that reduction in turbulence and distortion, potentially increased in oversized grafts, may improve homograft longevity.
In the present report, homograft durability was independent of the sequence of insertion. This agrees with previous data from Williams et al. [22] who demonstrated homologous findings in their series of 930 patients.
There are several obvious limitations to this retrospective review from a single institution. The use of conduit replacement as the definition of allograft failure introduces potential bias as criteria for conduit replacement were not uniform over the course of the study period, and patients with pulmonary hypertension or residual defects may tolerate equivalent levels of homograft stenosis less well than others. In addition, although data stratification improves homogeneity, and thus allows more meaningful comparison of outcomes, it also results in loss of statistical power and introduces the possibility that Type II error accounted for the failure to detect differences between study groups.
In the present report, we demonstrate that oversizing pulmonary homografts does not decrease conduit failure in young patients undergoing rapid somatic growth. Indexing the allograft size to patient BSA at the time of implantation provides equivalent outcomes and acceptable freedom from reintervention. Further study exploring the concordant relationship between recipient age and conduit longevity is warranted.
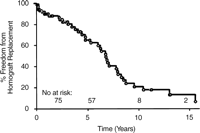
Kaplan–Meier freedom from allograft replacement for all 79 extracardiac conduits placed during the study period.
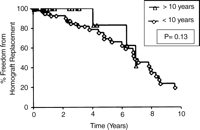
Kaplan–Meier freedom from homograft replacement for age-based strata. Group 1: conduits placed in those ≦10 years; Group 2: conduits placed in those ≫10 years.
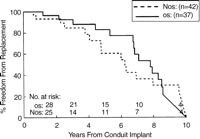
Actuarial freedom from homograft replacement (n=79) stratified by size. For the oversized grafts (OS) the 1 year, 5 year, and 10 year freedom from replacement was 96, 79, and 21%, respectively. For the non-oversized (NOS) grafts, the 1 year, 5 year, and 10 year freedom from replacement was 93, 60, and 24%, respectively. There was no difference between groups, by the log-rank statistic, P=0.44.
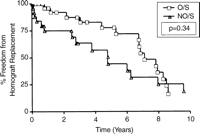
Kaplan–Meier freedom from homograft replacement for patients less than 10 years of age (Group 1) stratified by allograft size.
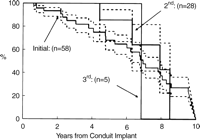
Actuarial freedom from conduit replacement stratified by sequence of insertion. The dashed lines enclose 95% confidence intervals.
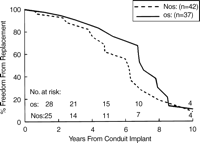
Freedom from homograft replacement (n=79) stratified by conduit size after adjustment for covariates utilized within the Cox regression model. Using the mean values of these covariates, the estimated survival functions were calculated for each subgroup. For the oversized grafts (OS) the 1 year, 5 year, and 10 year freedom from replacement was 97, 75, and 14%, respectively. For the non-oversized (NOS) grafts, the 1 year, 5 year, and 10 year freedom from replacement was 96, 62, and 11%, respectively.
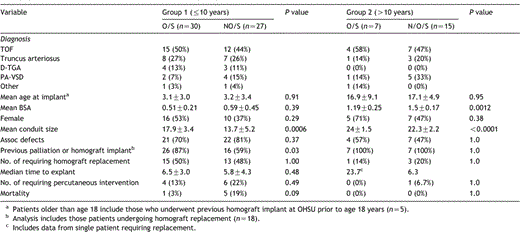
Demographics for the extracardiac cohort stratified according to age
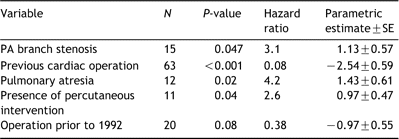
Multivariable analysis of factors associated with homograft explantation
Presented at the joint 18th Annual Meeting of the European Association for Cardio-thoracic Surgery and the 12th Annual Meeting of the European Society of Thoracic Surgeons, Leipzig, Germany, September 12–15, 2004.