-
PDF
- Split View
-
Views
-
Cite
Cite
Tamotsu Yasuda, Hiroyuki Kamiya, Yoko Tanaka, Go Watanabe, Ultra-short-acting cardioselective beta-blockade attenuates postischemic cardiac dysfunction in the isolated rat heart, European Journal of Cardio-Thoracic Surgery, Volume 19, Issue 5, May 2001, Pages 647–652, https://doi.org/10.1016/S1010-7940(01)00658-3
- Share Icon Share
Abstract
Objectives: We sought to test the effectiveness of ultra-short-acting cardioselective beta-blockade, landiolol hydrochloride, for warm heart surgery. Methods: The isolated perfused rat heart preparation was used. After preischemic measurement of cardiac function, 3 min of coronary infusion of crystalloid cardioplegic solution (37°C) with landiolol hydrochloride of various concentrations (1, 2.5, 5, and 10 mmol/l) or without it (control group) was performed, followed by 30 min of warm ischemic arrest. Finally, postischemic function was measured. Results: The percentage recoveries of heart rate in hearts receiving 0, 1, 2.5, 5, and 10 mmol/l landiolol hydrochloride were 89.4±3.4%, 90.9±1.7%, 89.6±1.8%, 83.4±3.3%, and 74.3±1.9% (P≪0.05 vs. 0, 1, and 2.5 mmol/l groups), respectively. The percentage recoveries of aortic flow were 55.6±3.1%, 62.8±3.3%, 75.0±4.2% (P≪0.05 vs. 0 and 10 mmol/l groups), 65.3±5.3%, and 51.6±4.0%, respectively. Similar recovery profiles were observed with the first derivative of the rise in aortic pressure, stroke volume and stroke work. The total amount of coronary effluent in the hearts receiving 5 or 10 mmol/l was lower than in the other groups. Conclusions: Landiolol hydrochloride has the potential to enhance postischemic cardiac function after the warm cardioplegic arrest. The optimal concentration for maximum postischemic functional recovery was 2.5 mmol/l, and recoveries of aortic flow and heart rate decreased in hearts receiving 5 mmol/l or more.
1 Introduction
Continuous warm blood cardioplegia is a useful technique for myocardial protection, avoiding ischemia and subsequent reperfusion injury and eliminating adverse effects of hypothermia. However, continuous cardioplegic infusion into the aortic root or the coronary sinus results in inadequate visualization of the operating field, and the infusion often needs to be interrupted for short intervals. Intermittent warm blood cardioplegia has been recently proposed as an alternative method of achieving myocardial protection and a better surgical field. However, it can lead to irretrievable myocardial ischemia. Several studies have been performed to determine the maximum time of interruption, and it is considered that the safe duration of interruption is between 10 and 20 min [1,2]. This duration is suitable for coronary artery bypass, but slightly longer interruption is needed for other surgical procedures such as valve replacement and congenital heart disease.
Myocardial ischemia is associated with marked accumulation of catecholamine in the ischemic tissue [3]. In vitro study suggested that 30 min of warm ischemia led to a 130-fold increase in norepinephrine (NE) overflow, and that this phenomenon is caused by non-exocytotic release of NE from the cardiac sympathetic nerve [4]. It is well known that beta-adrenergic mechanisms may play an important role in arrhythmogenesis and may contribute to myocyte injury during myocardial ischemia [5]. From this viewpoint, administration of beta-blockade before warm ischemia is an appropriate method for cardioprotection. In clinical practice, prospective analysis suggests that prior beta-blockade therapy has a cardioprotective effect in limiting creatine kinase-MB release after coronary intervention [6]. Various studies examining pharmacological prevention for ischemia-reperfusion injury in the isolated rat heart have been reported in relation to myocardial protection [7,8]. Wechsler and Abd-Elfattah [9] suggested that mechanisms and strategies contributing to myocardial protection were preconditioning, inhibition of adenosine triphosphate catabolism, the critical role of adenosine, management of myocardial edema, endothelial cell injury, antioxidant therapy, and the interaction between activated leukocytes and the endothelium. However, there have been no reports about the above-mentioned association in relation to myocardial protection.
Landiolol hydrochloride is a highly cardioselective (beta1/beta2=255) beta-blockade with little alpha-blocking action. This agent is approximately nine times more potent in beta-blocking activity in vivo and eight times more cardioselective in vitro than esmolol. In addition, it has a short duration (half-life of 4 min) of activity, enabling rapid recovery after cessation of administration [10]. The effectiveness of this agent, added to a commonly used cardioplegic solution, on postischemic cardiac function was investigated using the isolated perfused rat heart in the present study.
2 Materials and methods
Hearts were obtained from male Wistar rats weighing 300–350 g. All animals received humane care in compliance with the ‘Guide for the Care and Use of Laboratory Animals’ prepared by the Institute of Laboratory Animal Resources, National Research Council, and published by the National Academy Press, revised 1996.
The isolated perfused rat heart preparation was used for the present study. In brief, it is a left-sided heart preparation in which oxygenated Krebs–Henseleit bicarbonate buffer at 37°C enters the cannulated left atrium at a pressure equivalent to 20 cm H2O. The perfusate passes to the left ventricle from which it is spontaneously ejected through an aortic cannula against a hydrostatic pressure equivalent to 100 cm H2O. The exclusion criteria imposed in this study rejected hearts that produced an aortic flow of less than 50 ml/min or a coronary flow in excess of 25 ml/min after 20 min of preischemic working period. Coronary flow from the right side of the heart can be pooled and recirculated with the aortic outflow. Water-jacketed chambers maintained the heart under normothermic conditions. The myocardial temperature was measured using a thermometer, and the temperature was maintained at 37°C. Krebs–Henseleit bicarbonate buffer contained (in millimoles per liter) NaCl 118, NaHCO3 25, KCl 4.7, KH2PO4 1.2, MgSO4 1.2, CaCl2 2.5, and glucose 11 at a pH of 7.4 when gassed with 95% oxygen and 5% carbon dioxide at 37°C. The cardioplegic solution was St. Thomas' Hospital No. 2 which contained (in millimoles per liter) NaCl 110, NaHCO3 10, KCl 16, MgCl2 16, and CaCl2 1.2 [11].
After cannulation of the aorta, the heart was perfused with Krebs–Henseleit bicarbonate buffer in the Langendorff mode for a 5 min stabilization period. During the stabilization period, the pulmonary artery was cut and the left atrium was cannulated. The heart was then converted to a 20 min working mode. After preischemic measurement of cardiac function, a 3 min period of coronary infusion of cardioplegic solution with ultra-short-acting cardioselective beta-blockade, landiolol hydrochloride (Ono Pharmaceutical Co., Ltd., Osaka, Japan), of various concentrations (1, 2.5, 5, and 10 mmol/l) or without it (control group), was achieved by the use of a reservoir located 60 cm above the heart and attached to the side arm of the aortic cannula. The heart was then subjected to 30 min of ischemic arrest at 37°C by clamping the aortic cannula. Finally, hearts were reperfused in the Langendorff mode for 20 min and in the working mode for 20 min (37°C) for postischemic functional measurement (Fig. 1) .
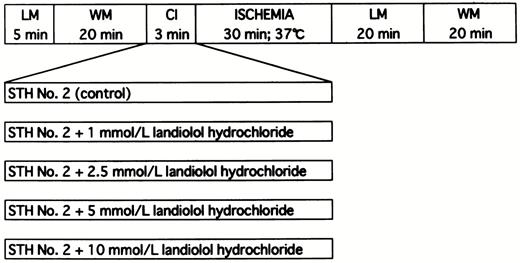
The experimental protocol represented diagrammatically. LM, Langendorff mode; WM, working mode; CI, cardioplegic infusion; STH No. 2, St. Thomas' Hospital cardioplegic solution No. 2.
Aortic flow, coronary flow and the total amount of coronary effluent during 3 min of cardioplegic infusion were measured by collecting the effluent from the aorta and venae cavae, respectively. Aortic pressure was constantly monitored by a pressure transducer (Life Scope 8; Nihon Kohden Co., Tokyo, Japan) connected to the aortic cannula and was recorded on a strip chart recorder. Heart rate and the first derivative of the rise in aortic pressure were calculated from records of aortic pressure. Cardiac output was derived from the sum of aortic flow and coronary flow, stroke volume was calculated by dividing cardiac output by heart rate, and stroke work was calculated by multiplying cardiac output by aortic pressure divided by heart rate.
Six rats were used for all groups. Results were expressed as the mean±standard error of the mean. To determine differences in recovery of function between groups, data were subjected to analyses by a one-way analysis of variance and if statistical significance was achieved Dunnett's (for group versus control comparison) and Turkey's (for group versus group comparison) modified t-tests were used. Differences were considered significant at the 95% confidence limit. All statistical analysis was conducted on a Macintosh computer using Statview (Abacus Concepts Inc., Berkeley, CA).
3 Results
Table 1 shows the preischemic function and postischemic recovery of cardiac function in hearts receiving various concentrations of landiolol hydrochloride. No significant differences were detected among all preischemic groups in cardiac function. The percentage recoveries of heart rate in hearts receiving 0, 1, 2.5, 5, and 10 mmol/l landiolol hydrochloride were 89.4±3.4% (P≪0.05 vs. 10 mmol/l group), 90.9±1.7% (P≪0.05 vs. 10 mmol/l group), 89.6±1.8% (P≪0.05 vs. 10 mmol/l group), 83.4±3.3%, and 74.3±1.9%, respectively (Fig. 2) . The percentage recoveries of heart rate in the hearts receiving 5 and 10 mmol/l were lower than in those receiving 0, 1, and 2.5 mmol/l, and the 10 mmol/l group reached statistical significance. The percentage recoveries of aortic flow in hearts receiving 0, 1, 2.5, 5, and 10 mmol/l landiolol hydrochloride were 55.6±3.1%, 62.8±3.3%, 75.0±4.2% (P≪0.05 vs. 0 and 10 mmol/l groups), 65.3±5.3%, and 51.6±4.0%, respectively (Fig. 3) . The percentage recovery of aortic flow was highest in the hearts receiving 2.5 mmol/l, and appeared to be bell-shaped. The percentage recovery of cardiac output was similar to that of aortic flow. However, the difference was not statistically significant between 0 and 2.5 mmol/l. The percentage recovery of aortic pressure was lowest in the hearts receiving 10 mmol/l. Similar recovery profiles were observed with the first derivative of the rise in aortic pressure (Fig. 4) , stroke volume and stroke work, and there were no differences among all groups.
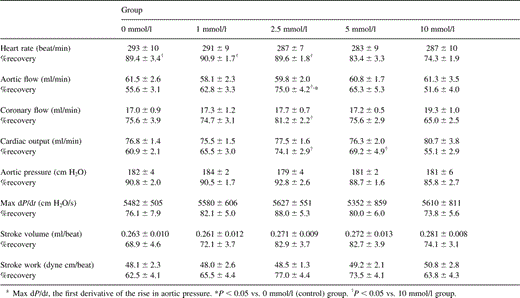
Preischemic function and postischemic recovery (%) of cardiac function in hearts receiving cardioplegic solution containing various concentrations of landiolol hydrochloridea
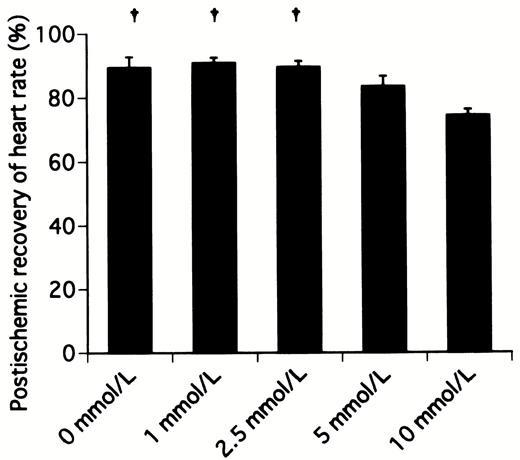
Postischemic recovery of heart rate after 20 min of working mode in hearts receiving cardioplegic solution containing various concentrations of landiolol hydrochloride. †P≪0.05 versus 10 mmol/l group.
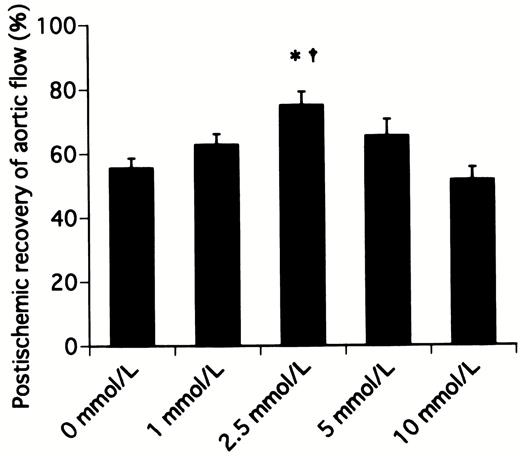
Postischemic recovery of aortic flow after 20 min of working mode in hearts receiving cardioplegic solution containing various concentrations of landiolol hydrochloride. *P≪0.05 versus 0 mmol/l (control) group, †P≪0.05 versus 10 mmol/l group.
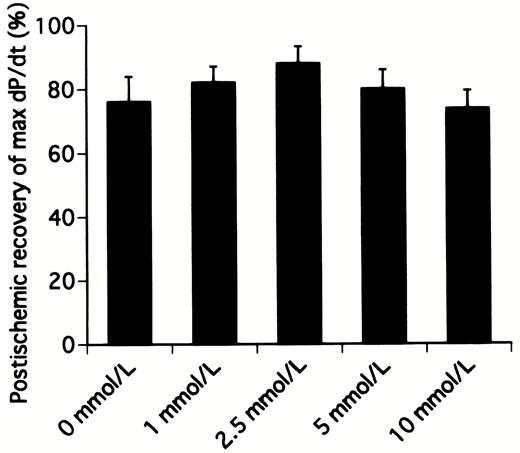
Postischemic recovery of the first derivative of the rise in aortic pressure (max dP/dt) after 20 min of working mode in hearts receiving cardioplegic solution containing various concentrations of landiolol hydrochloride.
The total amounts of the coronary effluent during 3 min of cardioplegic infusion in hearts receiving 0, 1, 2.5, 5, and 10 mmol/l landiolol hydrochloride were 30.0±1.2 ml (P≪0.05 vs. 10 mmol/l), 30.3±1.4 ml (P≪0.05 vs. 10 mmol/l), 29.3±1.2 ml (P≪0.05 vs. 10 mmol/l), 27.5±2.7 ml, and 20.7±2.8 ml, respectively (Fig. 5) . The total amount of the coronary effluent in the hearts receiving 10 mmol/l was much lower than those of other groups.
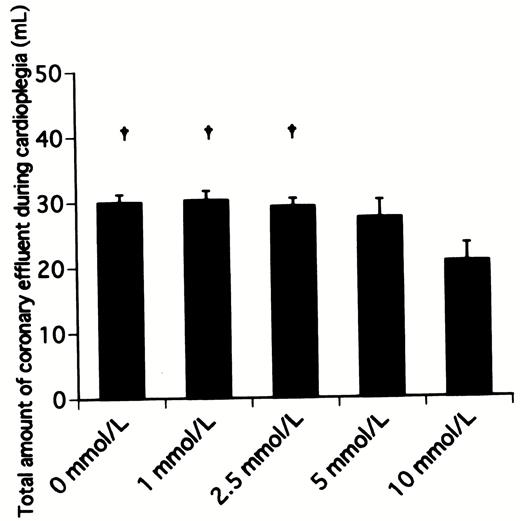
Total amount of coronary effluent during 3 min of infusion of cardioplegic solution containing various concentrations of landiolol hydrochloride. †P≪0.05 versus 10 mmol/l group.
4 Discussion
The present study demonstrated that ultra-short-acting cardioselective beta-blockade, landiolol hydrochloride, had the potential to enhance the postischemic cardiac function after warm cardioplegic arrest, when added to a commonly used cardioplegic solution, and that the optimal landiolol hydrochloride concentration for maximum postischemic functional recovery was 2.5 mmol/l. The percentage recoveries of aortic flow and heart rate decreased in hearts receiving 5 mmol/l or more, and the total amounts of coronary effluent during 3 min of cardioplegic infusion were low in similar concentrations. These results suggest that postischemic functional recovery was attenuated with excessively large doses of beta-blockade because of probable negative inotropism.
In in vitro experiments in isolated rat hearts, three phases of ischemia-induced release of NE have been recognized [4]. During the first phase of ischemia (0–10 min) accumulation of catecholamine in the extracellular space is prevented by the highly efficient uptake-1 mechanism and by presynaptic inhibition by adenosine. The latter is important in the rat, because adenosine concentrations are considerably higher than those in other species [12]. During the second phase of ischemia (10–40 min) the release of NE becomes non-exocytotic and is thought to involve the uptake-1 mechanism in the carrier-mediated efflux of NE in reverse of its normal transport direction. For example, NE overflow is increased from 3.7±0.6 to 481.2±56.2 pmol/g after 30 min of ischemia [4]. During the third phase of ischemia (≫40 min) the release of NE is no longer attenuated by uptake-1 inhibitors, which is explained by the occurrence of structural changes in the neuronal membrane of the myocardial neurons. In the present study, concentrations of NE can not be compared to those of above-mentioned ischemia, because hearts were electromechanically arrested by high-potassium crystalloid solution, St. Thomas' Hospital No. 2. However, it is highly possible that elevated concentrations of NE would have been observed in the hearts of the present study. We are planning to measure catecholamine levels in the myocardium in future investigations.
Cardiac beta-adrenergic receptors are chiefly the beta1-receptor subtype [13]. The beta-receptor is situated on the outer surface of the sarcolemma and is coupled to adenylyl cyclase by the stimulatory guanine nucleotide-binding protein. The major intracellular effects of beta-agonist are by formation of cyclic AMP, which increases the activity of the cyclic AMP-dependent protein kinase A. The latter phosphorylates various proteins including calcium channels [14].
Acute myocardial ischemia leads to a gradual increase in beta-adrenergic receptors. This increase occurs rapidly after the onset of global ischemia (15 min) and persists even after prolonged periods of global ischemia. In normal hearts, high catecholamines induced receptor desensitization with internalization of beta-adrenergic receptors. In acute myocardial ischemia, however, agonist-promoted internalization and functional uncoupling of beta-adrenergic receptors is abolished. Consequently, the balance of internalization and externalization of receptors is shifted towards an increase in functionally coupled receptors at the cell surface [15]. Above-mentioned events lead to intracellular accumulation of cyclic AMP and an increase of intracellular free calcium ion that may contribute to myocyte injury [16].
On the other hand, excess catecholamine affects calcium-transport mechanisms, primarily via oxidation reactions involving free radical-mediated damage. Therefore, pretreatment with antioxidant partly prevented the deleterious changes in cardiac membranes as well as beta-blockade [17]. It is a little difficult to decide to what extent catecholamine derived from nerve terminals in ischemic tissue can be associated with cardiotoxicity via oxidation reaction.
Landiolol hydrochloride is a highly cardioselective (beta1/beta2=255) beta-blockade when compared to esmolol (beta1/beta2=33) or propranolol (beta1/beta2=0.68). This agent is approximately nine times more potent in beta-blocking activity in vivo and eight times more cardioselective in vitro than esmolol. In addition, it has a short duration (half-life of 4 min) of activity, enabling rapid recovery after cessation of administration [10]. This ultra-short duration of landiolol hydrochloride is due to the rapid hydrolysis of its ester link, mediated primarily via an esterase. The esterase activity in man or dog is located in the red blood cell cytosol. However, in rat or guinea pig it is located in plasma, not in red blood cells [18]. In the present study, an isolated rat heart which received maximum cardioprotection was given approximately 140 mg/kg landiolol hydrochloride. The median lethal dose for acute toxicity of landiolol hydrochloride in mice was 290 mg/kg [10], and thus the dose given in the present study was markedly high. Adverse effects of administration of a high dose of landiolol hydrochloride upon various organs except the heart are unknown at this time. Large animal models under extracorporeal circulation should be tested for this purpose.
Several studies have suggested that esmolol could be used as effectively as potassium for inducing and maintaining cardiac arrest during normothermic cardiac operations [19,20]. It is expected that landiolol hydrochloride is as effective as esmolol for such clinical use. However, we selected preischemic administration of landiolol hydrochloride, added to a commonly used cardioplegic solution, followed by warm ischemia. The administration adopted in the present study is reasonable, because it protects the heart from marked accumulation of catecholamine caused by non-exocytotic release from the cardiac sympathetic nerve in 30 min ischemic tissue.
It has been previously reported that beta-blockade, propranolol, added to multidose potassium cardioplegia reduced metabolic activity during ischemia and improved ventricular compliance during reperfusion without depressing systolic function [21]. This beneficial effect may be related to an inhibition of the enzyme NADH oxidase by propranolol, the effect of which would be to slow mitochondrial respiration and thereby preserve adenosine triphosphate stores [22]. Recent studies have suggested that acute treatment of propranolol against ischemia-reperfusion injury may offer cardioprotection, and its mechanism responsible for the beneficial effect is as follows. The lipophilic interaction of propranolol with the cell membrane interrupts the carbon-centered free radical chain reaction generated within the sarcolemmal membrane, and propranolol consequently reduces lipid peroxidation [23]. It is unknown to what extent the cardioprotective effect of landiolol hydrochloride is due to antioxidation such as that with propranolol, and further investigation is needed.
Beta-agonist directly activates beta1- and beta2-receptors in coronary resistance vessels and increases coronary blood flow, and the vasodilation due to beta-agonist is dose-dependent and is attenuated by beta1- and beta2-antagonists [24]. Gross and Feigl [25] suggested that practocol, a selective beta1-receptor antagonist, blocked coronary vasodilation activated by isoproterenol only at a very high dose in the isolated potassium-arrested dog heart, because the beta-receptor on coronary smooth muscle is almost beta2, which is similar to those found in peripheral vessels.
The coronary flow rate after ischemia is well known to decrease because of ischemia-reperfusion injury [8]. In the present study, the percentage recovery of coronary flow in the group receiving 2.5 mmol/l landiolol hydrochloride was higher than that in the control group, whereas no significant differences were observed between two groups. The percentage recovery of coronary flow in the 10 mmol/l group was significantly lower than that in the 2.5 mmol/l group. This may be caused by a decreased heart rate in the 10 mmol/l group.
Many additives in relation to warm blood cardioplegia have been developed. However, it has not been reported that ultra-short-acting cardioselective beta-blockade enhances postischemic functional recovery. Although the effect of blood cardioplegia with landiolol was not investigated in the present study, it may be possible to elongate the safe duration of interruption during intermittent warm blood cardioplegia.
In conclusion, the present study demonstrated that ultra-short-acting cardioselective beta-blockade, landiolol hydrochloride, had the potential to enhance the postischemic cardiac function after warm cardioplegic arrest, when added to a commonly used St. Thomas' Hospital No. 2 cardioplegic solution. The mechanism by which landiolol hydrochloride achieves this effect may be through the adrenergic receptor-mediated action. Its other properties, including antioxidation such as that with propranolol, will be clarified in future investigations.