-
PDF
- Split View
-
Views
-
Cite
Cite
Jonathan Buber, Christopher Valle, Anne Marie Valente, Common congenital heart problems in acute and intensive care, European Heart Journal. Acute Cardiovascular Care, Volume 12, Issue 4, April 2023, Pages 267–279, https://doi.org/10.1093/ehjacc/zuad030
- Share Icon Share
Abstract
Over the past five decades, there have been multiple advances in the treatment of congenital heart defects, resulting in an increasing population of adults living with congenital heart disease (CHD). Despite improved survival, CHD patients often have residual haemodynamic sequelae and limited physiologic reserve and are at increased risk for acute decompensation with occurrence of arrhythmias, heart failure, and other medical conditions. Comorbidities occur more frequently and at an earlier age in CHD patients than in the general population. The management of the critically ill CHD patient requires an understanding of the unique aspects of congenital cardiac physiology as well as the recognition of other organ systems that may be involved. Certain patients may be candidates for mechanical circulatory support, and goals of care should be established with advanced care planning.
Introduction
Tremendous advances in the diagnosis and treatment of congenital heart disease (CHD) have resulted in improved survival and an increasing number of adult congenital heart disease (ACHD) patients. This increased longevity poses new challenges to healthcare systems, including increased resource utilization for patients with ACHD, particularly in acute care settings. Comorbidities occur more frequently and at an earlier age in CHD patients than in the general population.1 In a retrospective cohort study of 104 ACHD patients (median age 30 years) with 138 intensive care unit (ICU) admissions, the most common indications for admission were arrhythmias in 44%, followed by haemorrhage, heart failure (HF), and pulmonary conditions. The number of ICU admissions increased over the most recent time period (Figure 1).2 In this study, 48% of admissions were for patients with non-complex forms of CHD, emphasizing that even patients with anatomically milder forms of CHD are at risk for critical illness. This review will focus on the special anatomic and physiologic considerations in critically ill ACHD patients, with a focus on how their care may differ from those of patients with structurally normal hearts.
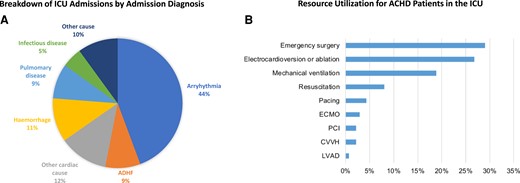
Non-elective intensive care unit admissions of adult patients with congenital heart disease. Figure recreated from data presented in Ramlakhan KP et al., Heart 2022. (A) Relative proportion of primary diagnosis for unplanned ICU admission to a single ICU from 2000 to 2015. (B) ICU resource utilization in patients with ACHD. Abbreviations: ICU, intensive care unit; ACHD, adult congenital heart disease; ADHF, acute decompensated heart failure; ASD, atrial septal defect; VSD, ventricular septal defect; CVVH, continuous venovenous haemofiltration; ECMO, extracorporeal membrane oxygenation; ICU, intensive care unit; LVAD, left ventricle assist device implantation; PCI, percutaneous coronary intervention.
Approach to the adult congenital heart disease patient presenting to the intensive care unit
The heterogeneity and complexity of the ACHD population makes it challenging to create uniform recommendations for critical care. Rather, the approach to a critically ill ACHD patient should be guided by first principles informed by the physiologic consequences of the underlying anatomy and prior repairs (Central Illustration). A detailed yet expedient review of the medical record to ascertain the baseline anatomy, prior interventional history, as well as recent diagnostic testing results is essential to providing timely and effective care. If this information is not readily available, then the clinician must rely on a careful clinical assessment and imaging studies to assist in early stabilization. At the core of this evaluation is the physical examination which may provide clues to the procedures performed in the distant past (i.e. lateral thoracotomy scar suggests history of coarctation repair and/or aortopulmonary shunt placement) as well as information about the current cardiovascular status. The chest radiograph can provide valuable information about cardiac position, cardiac size, pulmonary mechanics (such as an elevated hemidiaphragm due to phrenic nerve injury), and prior device lead placement. Finally, an electrocardiogram (ECG) must be performed as part of any initial ICU survey and reviewed for arrhythmia or ischaemia. It is necessary to recognize that some ACHD patients may have limited reserve at baseline and may have multiorgan involvement (Figure 2). Understanding these comorbidities is essential in formulating a treatment plan, as many ACHD patients live with subclinical organ dysfunction. In a single-centre study of 4977 ACHD patients, 28% had hepatorenal dysfunction, of which 9% was classified as severe (MELD XI score >15), which correlated with worsened survival.3 In treating adults with Fontan physiology, one should be aware that almost all have some degree of Fontan-associated liver disease that may not be detected with routine liver function tests.
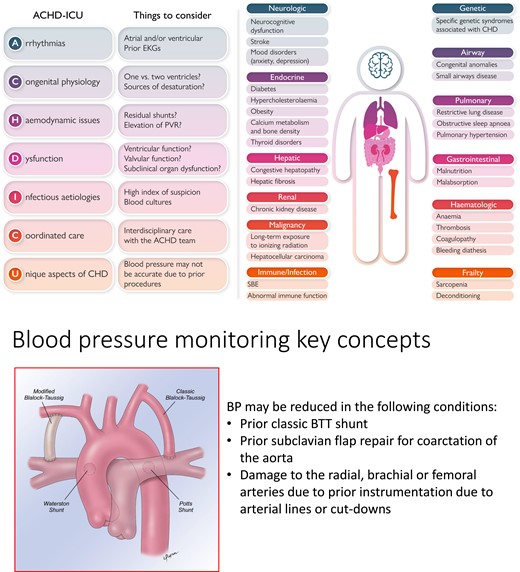
Key considerations in the management of adult congenital heart disease patients in the intensive care unit. Left, the ACHD-ICU acronym outlining the main points to be considered in the care of the ACHD patient. Right, non-cardiac problems impacting critical care. Bottom, unique aetiologies of low blood pressure in ACHD patients. Abbreviations: CHD, congenital heart disease; EKG, electrocardiogram; PVR, pulmonary vascular resistance; ACHD, adult congenital heart disease; SBE, subacute bacterial endocarditis; BP, blood pressure; BTT, Blalock–Taussig–Thomas.
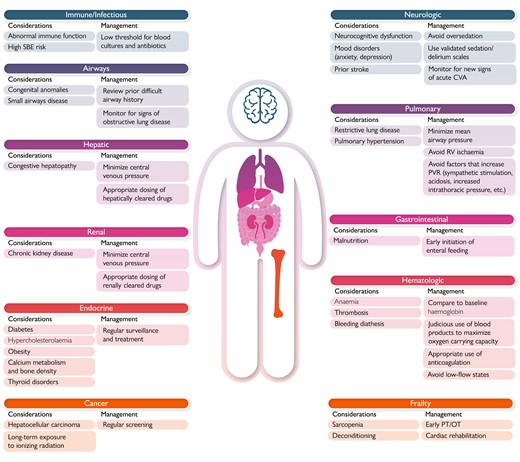
Non-cardiac complications in adults with congenital heart disease.
Armed with this initial survey, the intensivist can formulate a clinical plan informed by the patient’s current cardiac status. As congenital cardiologists, we have found the use of a mental ‘checklist’ helpful in the physiologic categorizations of patients (Table 1). While this list is by no means exhaustive, it can empower the intensivist to identify the key physiologic drivers in the most complex patients. Use of this checklist can aid the intensivist in categorizing patients into broad physiologic categories to help in decisions around important clinical factors such as inotrope and vasopressor selection, fluid management, use of microfilters on intravenous lines for right to left shunt physiology and strategies for optimal ventilation. Included in this checklist is an understanding of the patient’s baseline oxygen saturation, which is critically important for appropriate ventilation and oxygenation goals. For example, patients following Fontan completion for single ventricle physiology should have normal oxygen saturations; however, there are multiple sources of desaturation that may be present (Table 2). Figure 3 illustrates some unique haemodynamic considerations and key monitoring factors that arise with specific congenital conditions.
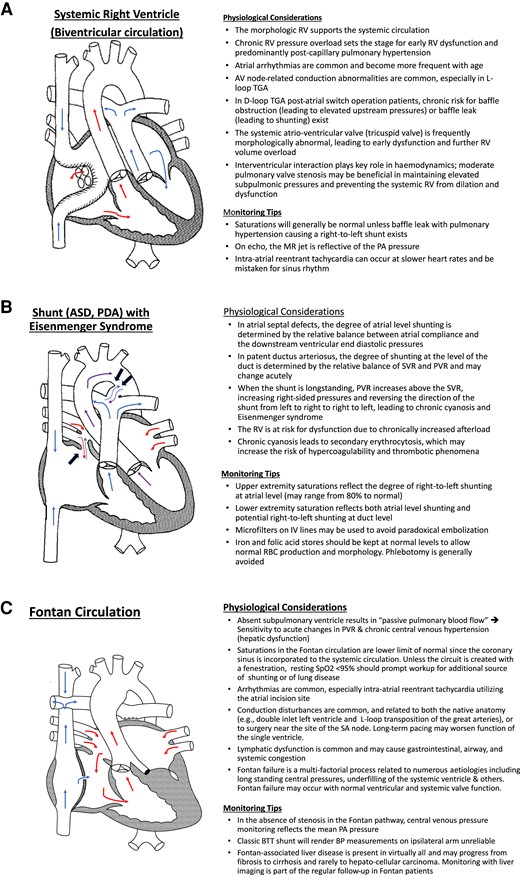
Key physiologic and monitoring considerations in selected congenital heart disease conditions. (A) In the example shown, the patient’s native anatomy was that of {S, D, D} transposition of the great arteries. Atrial-level switch procedures (‘Mustard’ and ‘Senning’) utilize intracardiac rerouting via a ‘baffle’ to redirect blood flow. The SVC and the IVC transport deoxygenated blood and direct it through the mitral valve to the left ventricle and out the PA. Surgical removal of the atrial septum allows pulmonary venous return to traverse from posterior left atrium through the space in the systemic venous baffle through the tricuspid valve to the right ventricle and out the aorta. RV, right ventricle; TGA, transposition of the great arteries; MR, mitral regurgitation; PA, pulmonary artery. (B) The presence of an unrestricted left-to-right shunt through an atrial septal defect (large arrow) or through a patent ductus arteriosus (two large arrows) leads to increased pulmonary blood flow, gradual pressure build-up, and vessel remodelling in the pulmonary vasculature. If left untreated, an increase in the PVR and shunt reversal (blue arrow at the atrial septal defect and purple arrow at the level of the patent ductus arteriosus) follows. ASD, atrial septal defect; PDA, patent ductus arteriosus; SVR, systemic vascular resistance, PVR, pulmonary vascular resistance; IV, intravenous. (C) In the example shown, the patient’s native anatomy was that of {S, D, S} mitral atresia, aortic atresia, and aortic arch hypoplasia resulting in hypoplastic left heart syndrome. The patient’s prior surgical palliation consisted of a Stage 1 or Norwood operation (atrial septectomy, Damus–Kaye–Stansel anastomosis of ascending aorta to main pulmonary artery with ligation and oversewing of distal main pulmonary artery, and aortic arch reconstruction) followed by a Glenn (superocavopulmonary) anastomosis and finally completion of a fenestrated, lateral tunnel Fontan circulation (inferocavopulmonary anastomosis). BTT, Blalock–Taussig–Thomas.
Obtain and review prior surgical records to define current anatomy. |
Obtain data on baseline vital signs (particularly outpatient saturations and blood pressure). |
Review recent imaging, electrophysiological and catheterization data to identify current physiology as below: |
ȃSingle or biventricular circulation? |
ȃMorphology of systemic ventricle? |
ȃSystolic and/or diastolic dysfunction? |
ȃPresence of shunt? Direction of shunting? Baseline saturations? |
ȃAtrioventricular valve disease? |
ȃSemilunar valve disease? Systemic outflow obstruction? |
ȃPresence of intracardiac baffle? Evidence of baffle obstruction or leakage? |
ȃPresence of extracardiac baffle/conduit? Evidence of obstruction and/or regurgitation? |
ȃPresence of pulmonary hypertension? |
Identify and document current medications. |
Perform and document complete physical examination (peripheral pulses, location of surgical scars, etc.). |
Assess pulmonary function (prior chest radiograph and pulmonary function tests). |
Assess renal function (creatinine clearance and cystatin C). |
Assess hepatic function (coagulation studies, albumin, and transaminases). |
Assess haematologic parameters (haemoglobin, iron stores, and transferrin saturation). |
Identify additional psychosocial issues (history of chronic pain and healthcare proxy/advanced directives). |
Obtain and review prior surgical records to define current anatomy. |
Obtain data on baseline vital signs (particularly outpatient saturations and blood pressure). |
Review recent imaging, electrophysiological and catheterization data to identify current physiology as below: |
ȃSingle or biventricular circulation? |
ȃMorphology of systemic ventricle? |
ȃSystolic and/or diastolic dysfunction? |
ȃPresence of shunt? Direction of shunting? Baseline saturations? |
ȃAtrioventricular valve disease? |
ȃSemilunar valve disease? Systemic outflow obstruction? |
ȃPresence of intracardiac baffle? Evidence of baffle obstruction or leakage? |
ȃPresence of extracardiac baffle/conduit? Evidence of obstruction and/or regurgitation? |
ȃPresence of pulmonary hypertension? |
Identify and document current medications. |
Perform and document complete physical examination (peripheral pulses, location of surgical scars, etc.). |
Assess pulmonary function (prior chest radiograph and pulmonary function tests). |
Assess renal function (creatinine clearance and cystatin C). |
Assess hepatic function (coagulation studies, albumin, and transaminases). |
Assess haematologic parameters (haemoglobin, iron stores, and transferrin saturation). |
Identify additional psychosocial issues (history of chronic pain and healthcare proxy/advanced directives). |
Obtain and review prior surgical records to define current anatomy. |
Obtain data on baseline vital signs (particularly outpatient saturations and blood pressure). |
Review recent imaging, electrophysiological and catheterization data to identify current physiology as below: |
ȃSingle or biventricular circulation? |
ȃMorphology of systemic ventricle? |
ȃSystolic and/or diastolic dysfunction? |
ȃPresence of shunt? Direction of shunting? Baseline saturations? |
ȃAtrioventricular valve disease? |
ȃSemilunar valve disease? Systemic outflow obstruction? |
ȃPresence of intracardiac baffle? Evidence of baffle obstruction or leakage? |
ȃPresence of extracardiac baffle/conduit? Evidence of obstruction and/or regurgitation? |
ȃPresence of pulmonary hypertension? |
Identify and document current medications. |
Perform and document complete physical examination (peripheral pulses, location of surgical scars, etc.). |
Assess pulmonary function (prior chest radiograph and pulmonary function tests). |
Assess renal function (creatinine clearance and cystatin C). |
Assess hepatic function (coagulation studies, albumin, and transaminases). |
Assess haematologic parameters (haemoglobin, iron stores, and transferrin saturation). |
Identify additional psychosocial issues (history of chronic pain and healthcare proxy/advanced directives). |
Obtain and review prior surgical records to define current anatomy. |
Obtain data on baseline vital signs (particularly outpatient saturations and blood pressure). |
Review recent imaging, electrophysiological and catheterization data to identify current physiology as below: |
ȃSingle or biventricular circulation? |
ȃMorphology of systemic ventricle? |
ȃSystolic and/or diastolic dysfunction? |
ȃPresence of shunt? Direction of shunting? Baseline saturations? |
ȃAtrioventricular valve disease? |
ȃSemilunar valve disease? Systemic outflow obstruction? |
ȃPresence of intracardiac baffle? Evidence of baffle obstruction or leakage? |
ȃPresence of extracardiac baffle/conduit? Evidence of obstruction and/or regurgitation? |
ȃPresence of pulmonary hypertension? |
Identify and document current medications. |
Perform and document complete physical examination (peripheral pulses, location of surgical scars, etc.). |
Assess pulmonary function (prior chest radiograph and pulmonary function tests). |
Assess renal function (creatinine clearance and cystatin C). |
Assess hepatic function (coagulation studies, albumin, and transaminases). |
Assess haematologic parameters (haemoglobin, iron stores, and transferrin saturation). |
Identify additional psychosocial issues (history of chronic pain and healthcare proxy/advanced directives). |
Mode of desaturation . | Mechanism . | Notes . |
---|---|---|
Mixed venous desaturation | Reduced oxygen delivery (DO2): causes of cardiogenic shock (arrhythmia, ischaemia, and tamponade) Increased oxygen extraction (VO2): fever and raised sympathetic drive (anxiety and pain) Anaemia (decreased oxygen carrying capacity) | Only contributing if there is a source of shunting (i.e. patent fenestration, baffle leak, or intrapulmonary shunting) |
Pulmonary venous desaturation | Pulmonary oedema Atelectasis Pleural effusion Pneumothorax Pneumonia/respiratory infection | Typical causes of hypoxaemia in non-congenital patients |
Venovenous collaterals | Collateral vessels from systemic veins to pulmonary veins can form secondary to long-standing systemic venous hypertension | |
Fenestration in the Fontan circulation | Typically created at the time of the Fontan completion with the aim of reducing the systemic venous pressures | |
Pulmonary arteriovenous malformations | Can develop in the setting of:
| |
Impaired pulmonary blood flow | Thrombus in pulmonary artery or Fontan pathway Acidosis Haemolysis (via free radical scavenging) Hypercarbic respiratory failure Dehydration | Elevated PVR leads to reduction in pulmonary blood flow relative to systemic blood flow |
Hepatic veins not incorporated into Fontan | Source of right-to-left shunting at atrial level | |
Drainage of coronary sinus into pulmonary venous atrium |
| |
Drainage of embryologic structures (levo-atrial cardinal vein) into coronary sinus draining into pulmonary venous atrium | Long-standing systemic venous hypertension can lead to ‘re-opening’ of embryologic venous structures | |
Patency of a previously unrecognized severely hypoplastic AV valve in atrio-pulmonary Fontan | Not applicable to patients with extracardiac or lateral tunnel style Fontan palliations |
Mode of desaturation . | Mechanism . | Notes . |
---|---|---|
Mixed venous desaturation | Reduced oxygen delivery (DO2): causes of cardiogenic shock (arrhythmia, ischaemia, and tamponade) Increased oxygen extraction (VO2): fever and raised sympathetic drive (anxiety and pain) Anaemia (decreased oxygen carrying capacity) | Only contributing if there is a source of shunting (i.e. patent fenestration, baffle leak, or intrapulmonary shunting) |
Pulmonary venous desaturation | Pulmonary oedema Atelectasis Pleural effusion Pneumothorax Pneumonia/respiratory infection | Typical causes of hypoxaemia in non-congenital patients |
Venovenous collaterals | Collateral vessels from systemic veins to pulmonary veins can form secondary to long-standing systemic venous hypertension | |
Fenestration in the Fontan circulation | Typically created at the time of the Fontan completion with the aim of reducing the systemic venous pressures | |
Pulmonary arteriovenous malformations | Can develop in the setting of:
| |
Impaired pulmonary blood flow | Thrombus in pulmonary artery or Fontan pathway Acidosis Haemolysis (via free radical scavenging) Hypercarbic respiratory failure Dehydration | Elevated PVR leads to reduction in pulmonary blood flow relative to systemic blood flow |
Hepatic veins not incorporated into Fontan | Source of right-to-left shunting at atrial level | |
Drainage of coronary sinus into pulmonary venous atrium |
| |
Drainage of embryologic structures (levo-atrial cardinal vein) into coronary sinus draining into pulmonary venous atrium | Long-standing systemic venous hypertension can lead to ‘re-opening’ of embryologic venous structures | |
Patency of a previously unrecognized severely hypoplastic AV valve in atrio-pulmonary Fontan | Not applicable to patients with extracardiac or lateral tunnel style Fontan palliations |
Mode of desaturation . | Mechanism . | Notes . |
---|---|---|
Mixed venous desaturation | Reduced oxygen delivery (DO2): causes of cardiogenic shock (arrhythmia, ischaemia, and tamponade) Increased oxygen extraction (VO2): fever and raised sympathetic drive (anxiety and pain) Anaemia (decreased oxygen carrying capacity) | Only contributing if there is a source of shunting (i.e. patent fenestration, baffle leak, or intrapulmonary shunting) |
Pulmonary venous desaturation | Pulmonary oedema Atelectasis Pleural effusion Pneumothorax Pneumonia/respiratory infection | Typical causes of hypoxaemia in non-congenital patients |
Venovenous collaterals | Collateral vessels from systemic veins to pulmonary veins can form secondary to long-standing systemic venous hypertension | |
Fenestration in the Fontan circulation | Typically created at the time of the Fontan completion with the aim of reducing the systemic venous pressures | |
Pulmonary arteriovenous malformations | Can develop in the setting of:
| |
Impaired pulmonary blood flow | Thrombus in pulmonary artery or Fontan pathway Acidosis Haemolysis (via free radical scavenging) Hypercarbic respiratory failure Dehydration | Elevated PVR leads to reduction in pulmonary blood flow relative to systemic blood flow |
Hepatic veins not incorporated into Fontan | Source of right-to-left shunting at atrial level | |
Drainage of coronary sinus into pulmonary venous atrium |
| |
Drainage of embryologic structures (levo-atrial cardinal vein) into coronary sinus draining into pulmonary venous atrium | Long-standing systemic venous hypertension can lead to ‘re-opening’ of embryologic venous structures | |
Patency of a previously unrecognized severely hypoplastic AV valve in atrio-pulmonary Fontan | Not applicable to patients with extracardiac or lateral tunnel style Fontan palliations |
Mode of desaturation . | Mechanism . | Notes . |
---|---|---|
Mixed venous desaturation | Reduced oxygen delivery (DO2): causes of cardiogenic shock (arrhythmia, ischaemia, and tamponade) Increased oxygen extraction (VO2): fever and raised sympathetic drive (anxiety and pain) Anaemia (decreased oxygen carrying capacity) | Only contributing if there is a source of shunting (i.e. patent fenestration, baffle leak, or intrapulmonary shunting) |
Pulmonary venous desaturation | Pulmonary oedema Atelectasis Pleural effusion Pneumothorax Pneumonia/respiratory infection | Typical causes of hypoxaemia in non-congenital patients |
Venovenous collaterals | Collateral vessels from systemic veins to pulmonary veins can form secondary to long-standing systemic venous hypertension | |
Fenestration in the Fontan circulation | Typically created at the time of the Fontan completion with the aim of reducing the systemic venous pressures | |
Pulmonary arteriovenous malformations | Can develop in the setting of:
| |
Impaired pulmonary blood flow | Thrombus in pulmonary artery or Fontan pathway Acidosis Haemolysis (via free radical scavenging) Hypercarbic respiratory failure Dehydration | Elevated PVR leads to reduction in pulmonary blood flow relative to systemic blood flow |
Hepatic veins not incorporated into Fontan | Source of right-to-left shunting at atrial level | |
Drainage of coronary sinus into pulmonary venous atrium |
| |
Drainage of embryologic structures (levo-atrial cardinal vein) into coronary sinus draining into pulmonary venous atrium | Long-standing systemic venous hypertension can lead to ‘re-opening’ of embryologic venous structures | |
Patency of a previously unrecognized severely hypoplastic AV valve in atrio-pulmonary Fontan | Not applicable to patients with extracardiac or lateral tunnel style Fontan palliations |
Clinical scenarios in the intensive care unit
Heart failure decompensation and cardiogenic shock
Heart failure decompensation and cardiogenic shock (CS) can occur in ACHD patients with either a morphological right or left ventricle supporting the systemic circulation or a single systemic ventricle in patients with a Fontan circulation or unrepaired univentricular heart defects. Certain groups of ACHD patients are at a higher risk of developing HF exacerbation, including those with moderate or severe lesion complexity, those with prior history of HF, atrial arrhythmias, and pulmonary hypertension.4
Despite the obvious anatomical considerations, there are currently no universally accepted definitions for HF exacerbation or CS for ACHD patients. The Society for Cardiovascular Angiography and Interventions defines CS as a sustained episode of systolic blood pressure (SBP) <90 mm Hg for at least 30 min, use of vasoactive agents to maintain SBP, and cardiac index <2.2 L/min/m2 in the absence of hypovolaemia, each determined to be secondary to cardiac dysfunction or requiring use of advanced mechanical support.5 Some of these criteria, such as the low SBP and low cardiac index, may be present in chronically—yet not critically ill—ACHD patients, and thus attention should be given to understanding the underlying physiology and baseline condition of the patient.
HF exacerbation is more broadly defined as rapid worsening in HF symptoms and worsening congestion.6 Several key precipitating factors for HF decompensation are common to ACHD and non-ACHD patients, including acute coronary syndrome, uncontrolled hypertension, infections, arrhythmias, sodium retention, medication non-adherence, and high output states such as anaemia and hyperthyroidism. Adult congenital heart disease patients may develop HF that is driven by extracardiac factors that directly impair myocardial performance which would not typically lead to decompensation in those with structurally normal hearts. These factors should be actively sought for and promptly addressed, as neglecting to acknowledge them may hinder the effectiveness of otherwise potent HF therapies.
In patients with a Fontan circulation, a rapid increase in the pulmonary impedance secondary to acute processes such as a pneumonia, pneumothorax, or PE may result in acute decrease in the pulmonary blood flow and further underfilling of the systemic ventricle, leading to HF decompensation and even to CS.7,8 In the setting of long-standing liver disease with elevated central venous pressure (CVP) as present in virtually all Fontan patients9 and in many patients with a dysfunctional subpulmonic ventricle or atrioventricular (AV) valve, the liver parenchyma is more susceptible to acute injury from various metabolic, toxin-related, and haemodynamic insults. Acute decline in the cardiac output during a HF exacerbation may thus lead to further impairment of flow in the portal circulation and acute-on-chronic liver injury, which may manifest as a sharp drop in the systemic vascular resistance (SVR) and contribute a distributive component to shock pathophysiology.10 Large systemic arterial to pulmonary fistulas and shunts that are naturally formed or created to augment pulmonary blood flow may pose a risk of overcirculation and volume overload to the systemic ventricle. The feasibility of closure of these shunts should be assessed in the setting of HF exacerbation.
Myocardial ischaemia may occur at a younger age than in the general population and can be secondary to classical atherosclerotic risk factors11 that are more common in lesions such as coarctation of the aorta,12 due to the higher lifetime prevalence of hypertension with superimposed vasculopathy. Other aetiologies include anomalous coronary origin or course predisposing to dynamic myocardial ischaemia, coronary compressions by a dilated pulmonary artery (PA) in severe pulmonary hypertension, or compression by a prosthetic pulmonary valve, especially if the native coronary anatomy is anomalous.13 Notably, ischaemic ECG changes may differ from the ‘classic’ ischaemic changes in the general population, depending on the underlying anatomy. Comparison with prior ECG tracings is helpful for assessment of acute changes.
Haemodynamic goals for the treatment of HF exacerbation and CS should be individualized and based on the underlying anatomy and physiology. In order to allow adequate forward flow to the pulmonary circulation, CVP goals should generally be higher in patients with a Fontan circulation or poorly compliant right ventricle such as in pulmonary atresia with intact ventricular septum or tetralogy of Fallot with restrictive right ventricular physiology. Similar considerations are present for those requiring mechanical ventilatory support, as lesions that depend on venous preload are particularly sensitive to changes in intrathoracic pressures. Adjustments to minimize positive intrathoracic pressure can be made using smaller tidal volumes, minimal positive end-expiratory pressure, pressure-regulated ventilatory modes, or spontaneous ventilation with minimal pressure support (Figure 4). It is also critical to recognize factors that will alter the balance of flow in any patient with a shunt lesion, as the direction and magnitude of the shunt are dictated by the delicate balance between the pulmonary and the systemic resistances. There are multiple factors which will increase pulmonary blood flow by either decreasing the pulmonary vascular resistance (PVR) or increasing the SVR (Figure 5). Additionally, the selection of vasopressors and inotropes should be based on their haemodynamic profiles in both the systemic and pulmonary vasculature, as summarized in Table 3.
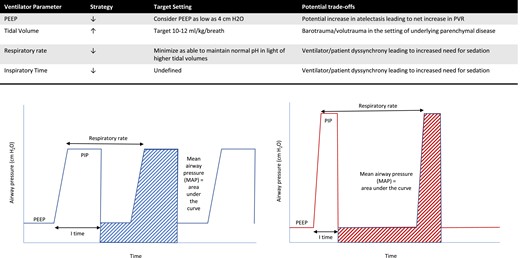
Select strategies to reduce pulmonary vascular resistance in mechanically ventilated patients with congenital heart disease. (Left) Lower inspiratory pressures leading to lower airways pressures. (Right) Lower respiratory rates with higher inspiratory pressures allowing the maintenance of normal pH and preventing atelectasis.
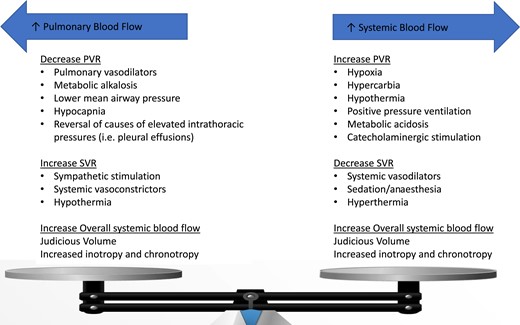
Factors affecting pulmonary (left) and systemic (right) blood flow. In ACHD patients with post-tricuspid shunt lesions, the direction and magnitude of the shunt is dictated by the delicate balance between the pulmonary and the systemic resistances. In patients with the Fontan circulation and pulmonary hypertension, sharp increase in the pulmonary vascular resistance may lead to acute haemodynamic derangement and even cardiogenic shock.
Inotropes, vasopressors, and pulmonary vasodilators’ effects on haemodynamics in adult congenital heart disease patients
Drug . | Cardiac output . | PVR . | SVR . | Tachycardia/arrhythmia . |
---|---|---|---|---|
Inodilators | ||||
ȃDobutamine | ||||
ȃ <5 mcg/kg/min | ↑ | ↘ | →/↘ | ++ |
ȃ5–15 mcg/kg/min | ↑↑ | → | ↘ | +++ |
ȃMilrinone | ↑↑ | → | ↘↘ | ++ |
Inopressors | ||||
ȃEpinephrine | ↑↑ | →/↘ | ↑↑ | +++ |
ȃDopamine | ↑ | → | ↑↑ | +++ |
ȃ <2 mcg/kg/min | ↑ | → | ↑ | ++ |
ȃ5–10 mcg/kg/min | ↑↑ | → | ↑ | +++ |
ȃ10–20 mcg/kg/min | ↑ | → | ↑↑ | ++++ |
ȃNorepinephrine | ↑ | →/↑ | ↑↑ | + |
Vasopressors | ||||
ȃVasopressin | →/↑ | →/↘ | ↑↑ | — |
ȃPhenylephrine | →/↑ | →/↑ | ↑↑ | — |
Pulmonary vasodilators | ||||
ȃInhaled nitric oxide | →/↑ | ↘↘ | ↘ | — |
ȃEpoprostenol | →/↑ | ↘↘ | ↘ | — |
Drug . | Cardiac output . | PVR . | SVR . | Tachycardia/arrhythmia . |
---|---|---|---|---|
Inodilators | ||||
ȃDobutamine | ||||
ȃ <5 mcg/kg/min | ↑ | ↘ | →/↘ | ++ |
ȃ5–15 mcg/kg/min | ↑↑ | → | ↘ | +++ |
ȃMilrinone | ↑↑ | → | ↘↘ | ++ |
Inopressors | ||||
ȃEpinephrine | ↑↑ | →/↘ | ↑↑ | +++ |
ȃDopamine | ↑ | → | ↑↑ | +++ |
ȃ <2 mcg/kg/min | ↑ | → | ↑ | ++ |
ȃ5–10 mcg/kg/min | ↑↑ | → | ↑ | +++ |
ȃ10–20 mcg/kg/min | ↑ | → | ↑↑ | ++++ |
ȃNorepinephrine | ↑ | →/↑ | ↑↑ | + |
Vasopressors | ||||
ȃVasopressin | →/↑ | →/↘ | ↑↑ | — |
ȃPhenylephrine | →/↑ | →/↑ | ↑↑ | — |
Pulmonary vasodilators | ||||
ȃInhaled nitric oxide | →/↑ | ↘↘ | ↘ | — |
ȃEpoprostenol | →/↑ | ↘↘ | ↘ | — |
Modified from: Contemporary management of cardiogenic shock: a scientific statement from the American Heart Association. 2017. Circulation.
Acute Cardiovascular Care Association position statement for the diagnosis and treatment of patients with acute myocardial infarction complicated by cardiogenic shock: a document of the Acute Cardiovascular Care Association of the European Society of Cardiology. European Heart Journal: Acute Cardiovascular Care. 2022.
Inotropes, vasopressors, and pulmonary vasodilators’ effects on haemodynamics in adult congenital heart disease patients
Drug . | Cardiac output . | PVR . | SVR . | Tachycardia/arrhythmia . |
---|---|---|---|---|
Inodilators | ||||
ȃDobutamine | ||||
ȃ <5 mcg/kg/min | ↑ | ↘ | →/↘ | ++ |
ȃ5–15 mcg/kg/min | ↑↑ | → | ↘ | +++ |
ȃMilrinone | ↑↑ | → | ↘↘ | ++ |
Inopressors | ||||
ȃEpinephrine | ↑↑ | →/↘ | ↑↑ | +++ |
ȃDopamine | ↑ | → | ↑↑ | +++ |
ȃ <2 mcg/kg/min | ↑ | → | ↑ | ++ |
ȃ5–10 mcg/kg/min | ↑↑ | → | ↑ | +++ |
ȃ10–20 mcg/kg/min | ↑ | → | ↑↑ | ++++ |
ȃNorepinephrine | ↑ | →/↑ | ↑↑ | + |
Vasopressors | ||||
ȃVasopressin | →/↑ | →/↘ | ↑↑ | — |
ȃPhenylephrine | →/↑ | →/↑ | ↑↑ | — |
Pulmonary vasodilators | ||||
ȃInhaled nitric oxide | →/↑ | ↘↘ | ↘ | — |
ȃEpoprostenol | →/↑ | ↘↘ | ↘ | — |
Drug . | Cardiac output . | PVR . | SVR . | Tachycardia/arrhythmia . |
---|---|---|---|---|
Inodilators | ||||
ȃDobutamine | ||||
ȃ <5 mcg/kg/min | ↑ | ↘ | →/↘ | ++ |
ȃ5–15 mcg/kg/min | ↑↑ | → | ↘ | +++ |
ȃMilrinone | ↑↑ | → | ↘↘ | ++ |
Inopressors | ||||
ȃEpinephrine | ↑↑ | →/↘ | ↑↑ | +++ |
ȃDopamine | ↑ | → | ↑↑ | +++ |
ȃ <2 mcg/kg/min | ↑ | → | ↑ | ++ |
ȃ5–10 mcg/kg/min | ↑↑ | → | ↑ | +++ |
ȃ10–20 mcg/kg/min | ↑ | → | ↑↑ | ++++ |
ȃNorepinephrine | ↑ | →/↑ | ↑↑ | + |
Vasopressors | ||||
ȃVasopressin | →/↑ | →/↘ | ↑↑ | — |
ȃPhenylephrine | →/↑ | →/↑ | ↑↑ | — |
Pulmonary vasodilators | ||||
ȃInhaled nitric oxide | →/↑ | ↘↘ | ↘ | — |
ȃEpoprostenol | →/↑ | ↘↘ | ↘ | — |
Modified from: Contemporary management of cardiogenic shock: a scientific statement from the American Heart Association. 2017. Circulation.
Acute Cardiovascular Care Association position statement for the diagnosis and treatment of patients with acute myocardial infarction complicated by cardiogenic shock: a document of the Acute Cardiovascular Care Association of the European Society of Cardiology. European Heart Journal: Acute Cardiovascular Care. 2022.
Arrhythmias in the intensive care unit
Cardiac arrhythmias are common in ACHD patients, and the entire arrhythmic spectrum can be encountered in the acute care setting. In many cases, arrhythmias are related to the underlying congenital anatomy and the type and timing of repair. Common arrhythmias such as atrial fibrillation also frequently occur, yet these tend to present at an earlier age presumably due to early onset of structural remodeling.14 Young patients with Ebstein anomaly may present with AV reentrant tachycardia due to Wolfe–Parkinson–White syndrome, and patients with congenially corrected transposition of the great arteries may present with high degree AV block. Right atrial incisions, together with cardiac remodelling secondary to haemodynamic overload, contribute to the high prevalence of atrial tachycardias in ACHD patients. The most frequently encountered type is intraatrial reentrant tachycardia (IART), especially cavotricuspid isthmus-dependent atrial flutter. A high degree of suspicion for atrial flutter should be used in ACHD patients, as the ECG may not resemble typical flutter. In certain lesions such as transposition of the great artery status post-atrial switch palliation (Mustard or Senning operations), atrial rates between 150 and 250 beats per minute may lead to rapid AV conduction and acute haemodynamic compromise (Figure 6).14 Ventricular arrhythmias are less common and related to the underlying substrate. Monomorphic ventricular tachycardia (VT) is often secondary to macro-reentry circuits located within anatomically well-defined isthmuses bordered by surgical scars and patch material.15 This is particularly common in patients with prior surgical repairs for tetralogy of Fallot who have undergone ventricular incisions and patch material closure of the ventricular septal defect, with or without augmentation of the right ventricular outflow tract.16 Less organized and faster polymorphic VT or ventricular fibrillation may be encountered in patients with progressive failure of either the systemic or sub-pulmonary ventricle.17
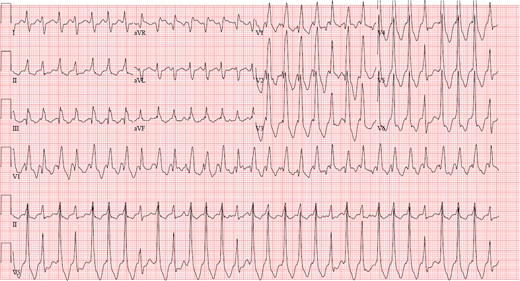
Electrocardiogram demonstrating intra-atrial reentrant tachycardia in a patient with D-loop transposition of the great arteries s/p atrial switch operation.
Similar to non-ACHD patients, evaluation for reversible causes of arrhythmia such as ischaemia, electrolyte abnormalities, acid-base derangements, and inflammatory process must be sought and corrected. Due to the close relationship between the haemodynamic and the electrical states in many CHD lesions, new or worsening residual haemodynamic abnormality such as residual shunt or haemodynamically significant valve lesion should be suspected and pursued. Arrhythmias causing haemodynamic instability require immediate cardioversion irrespective of the duration and anticoagulation state.14 Certain types of congenital defects such as transposition of the great arteries status post-atrial switch palliation and the Fontan palliation involve the risk of both IART and sinus node dysfunction. In those patients, post-conversion bradycardia and sinus node dysfunction may occur and back-up pacing capability needs to be available.17 In the case that transvenous pacemaker insertion is required, close familiarity with the underlying anatomy is essential. In patients with a superior vena cava (SVC) to PA (Glenn) anastomosis, the SVC will connect directly to the pulmonary arterial system and take a very different course then in the general population. Similar issues exist in patients with a Fontan circulation or interrupted inferior vena cava if femoral access is utilized. When feasible, cross-sectional imaging or angiography prior to the transvenous pacemaker insertion is recommended.
Rhythm control is generally preferred over rate control in ACHD patients due to the haemodynamic contributions of chamber synchrony and the atrial kick. When performed by experienced CHD electrophysiologists, ablative procedures have good long-term outcomes and are preferred over antiarrhythmic medical therapy alone. Similarly, bradyarrhythmias are often poorly tolerated due to the significant contribution of the heart rate to the cardiac output, and reversible causes should be addressed. When the bradyarrhythmia persists, permanent pacing should be performed by experienced CHD electrophysiologists.
Acute haemorrhagic and thromboembolic concerns
Individuals with CHD are uniquely predisposed to thromboembolic events at a young age due to a multitude of factors, including their underlying anatomy, sluggish blood flow in the venous system, residual shunts that allow for paradoxical embolism, prosthetic intracardiac material, the predisposition to arrhythmia and the presence of altered procoagulant and anticoagulant factors. In a longitudinal, registry-based observational study of patients with ACHD, 1 in 11 men and 1 in 15 women experienced a stroke between ages 18 and 64 years, with an incidence considerably higher than in the general population, especially in younger patients.18 In addition to the risk of cerebrovascular accidents (CVA), ACHD patients carry higher risk for venous thromboembolism and pulmonary embolism (PE) than the non-congenital population.19
There are a few unique populations for whom the development of acute thrombus is more likely to lead to admission to the ICU. In patients with single ventricle heart disease palliated to a Fontan, development of a thrombus along the pathway of venous return can lead to an acute increase in PVR with resultant low cardiac output, cyanosis, and the potential development of CS. Moreover, these patients often have residual shunts which can allow for paradoxical systemic embolism. Fontan patients appear particularly prone to the development of thrombus as they have decreased levels of protein C, protein S, and antithrombin III, impaired fibrinolytic potential, increased platelet reactivity, impaired endothelial function; subclinical liver dysfunction, and a high prevalence of atrial arrhythmias.20,21 While there is a growing evidence for the safety and efficacy of anticoagulation in the chronic management of thrombosis in the Fontan population,22 no studies have specifically assessed the relative efficacy of the various, available anticoagulants in this population in the acute setting, leaving clinicians to turn toward general guidelines.23 When considering the potential benefit of catheter-directed therapies in this population, providers must consider that a thrombus may not be able to be reached either due to the palliated anatomy or chronic vascular occlusions from repeated catheterizations.
The population of patients with chronic cyanosis is also uniquely prone to thrombotic events, especially the smaller subset with Eisenmenger syndrome (ES). Proposed mechanisms to account for this include local pulmonary vascular injury from elevated PA pressures, hypercoagulability secondary to changes in coagulation factors and platelet dysfunction resulting from long-standing cyanosis, and sluggish PA flow leading to red cell aggregation.24 Furthermore, the chronic cyanosis leads to compensatory erythrocytosis, as the body aims to maintain oxygen carrying capacity. However, the routine use of phlebotomy in the absence of true, life-threatening hyperviscosity syndrome should be avoided as it has been associated with worse outcomes, specifically increased risk of stroke in this population.
Critical care providers must constantly balance the risk of thrombotic events with that of bleeding, as the ACHD population is also prone to haemorrhage. In a single-centre, retrospective study of ACHD patients admitted to the ICU, haemorrhage was one of the more common primary indications for admission, occurring in 11% of the population.2 The reasons for haemorrhage in this population are numerous and often mirror the risk factors for thrombosis. The congestive hepatopathy in patients with single ventricles or failing subpulmonic ventricle may lead to both alterations in clotting factors leading to a bleeding diathesis as well as portal hypertension in severe cases with attendant varices and portal hypertensive gastropathy. Patients with cyanotic heart disease may have an associated thrombocytopenia that may be a result of reduced platelet lifespan or may be related to higher amount of megakaryocytes crossing through the right to left shunt, resulting in larger platelets that are not identified by automated cell counters. Patients with the Fontan circulation may also be at risk for significant bleeding from lower extremity varicosities, especially if they are anticoagulated at baseline. Other subpopulations are at elevated risk for life-threatening haemoptysis, including those with CHD-associated severe pulmonary hypertension, particularly those with ES.25,26 When approaching the ACHD patient with acute, life-threatening haemorrhage, the intensivist must rapidly act to reverse the effects of any anteceding anticoagulant use while monitoring closely for any untoward effects of blood product transfusion with respect to volume overload.
Sepsis and distributive shock
ACHD patients may develop distributive shock, most commonly as a manifestation of sepsis, which has been reported as the most common non-cardiac cause of death in a large, single-centre, retrospective study of >2500 subjects.27 Patients with ACHD may be predisposed to sepsis for a variety of reasons, including a higher baseline incidence of endocarditis28 as well as immunodeficiencies related to underlying genetic syndromes (e.g. 22q11 microdeletion syndromes and asplenia in heterotaxy syndrome).
The first principles of critical care for the septic ACHD patient should be extrapolated from the updated Surviving Sepsis Campaign.29 Management is pre-dictated by the rapid recognition of signs and symptoms of end-organ dysfunction from sepsis, early administration of appropriate antibiotics, and judicious use of fluid resuscitation and vasoactive support to improve end-organ perfusion and oxygen delivery. In ACHD patients, the intensivist must be aware of any physiologic considerations that may complicate standard management, such as the baseline low SVR present in many patients palliated with Fontan circulation.30 Providers must take into consideration the relative effects on the SVR and PVR of the various vasoactive agents (Table 3) when approaching patients with a circulation that is unusually sensitive to acute changes in PVR, such as Fontan circulation and pulmonary hypertension, or patients with acute and/or chronic failure of the subpulmonic ventricle. Moreover, clinicians must be thoughtful in the provision of initial fluid resuscitation, monitoring closely for evidence of volume overload in patients that may have baseline systolic and/or diastolic ventricular dysfunction. Finally, intensivists must recognize the baseline renal and hepatic dysfunction in patients with chronic systemic venous hypertension from right-sided HF or single ventricle heart disease.
Additional considerations
Mechanical circulatory support considerations for adult congenital heart disease patients
Mechanical circulatory support (MCS) options in ACHD patients include extracorporeal membrane oxygenation (ECMO), total artificial heart (TAH), and ventricular assist devices (VAD). Regardless of the purpose for which MCS is being considered, involvement of an ACHD expert from a very early stage in the decision-making process is imperative.
In several studies that evaluated outcomes of MCS, ACHD patients had similar improvement in functional status and quality of life compared with non-ACHD patients.31 Survival rates of ACHD patients bridged to heart transplant with a VAD were comparable to those transplanted directly, despite having more comorbidities and worse baseline functional status.32 Conversely, Dolgner et al33 reported on outcomes of ACHD patients from the Extracorporeal Life Support Organization (ELSO) registry from 1994 to 2016. They identified 368 ACHD patients who received MCS after cardiac surgery with an overall mortality of 61%. Pre-cannulation risk factors for mortality included Fontan physiology, weight >100 kg, female gender, delayed cannulation, and use of neuromuscular blockade.
In the largest, retrospective series from the ELSO database, in 230 patients with Fontan circulation supported with ECMO from 1987 to 2009, the overall survival to discharge was only 35%, and in the most recent 2 years of data (30 patients), only 36% survived despite advances in the practice of ECMO.34
Common indications for MCS utilization in ACHD patients are generally similar to the general population and can include:
Stabilization of patients who present with CS, low cardiac output state, or cardiomyopathies due to acute coronary injury
Rescue therapy in patients unable to wean from cardiopulmonary bypass or those who need peri-procedural support
In the presence of reversible causes of ventricular dysfunction and often serves as a bridge to decision or as a bridge to transplant35
Early during cardiopulmonary resuscitation in complex CHD patients36
In patients awaiting heart transplantation and have prohibitive end-organ damage
The first and most important step in considering MCS for ACHD patients is establishment of a thorough understanding of the underlying anatomy and the prior surgical and catheter-based interventions. Identifying the patency of blood vessels that can serve for cannulation is key, as many ACHD patients have undergone multiple prior cardiac procedures placing them at a high risk of mediastinal adhesions and vascular complications. Updated imaging studies including ultrasound imaging of the neck and the femoral vessels as well as cross-sectional imaging of the chest are essential to evaluate for vessel patency and relationship of vascular structures, such as a dilated aorta, to the anterior chest wall.
For patients with a Fontan circulation, MCS planning requires differentiating between the need to improve antegrade Fontan flow in cases of low cardiac output vs. unloading the systemic ventricle in cases of severely elevated end-diastolic pressures. Ventricular assist device utilization in the Fontan circulation increases the mean systemic pressure and cardiac output. It also increases pulmonary blood flow and systemic venous return, thus ultimately reducing CVP. A VAD is less likely to be helpful the setting of low end-diastolic pressure (<10–12 mmHg) of the systemic ventricle. In such cases, when medium- or longer-term support is required, the SynCardia TAH (SynCardia, Tucson, Arizona, USA) can be considered. TAH is a fully implantable pulsatile VAD that replaces both the ventricles after cardiectomy and is attached to atrial cuffs. Anecdotal reports of TAH use for patients with a Fontan circulation suggest short term favourable results.37,38
Venoarterial (VA)-ECMO use requires adequate capture of venous drainage to help pump output to the systemic circulation. In patients with a Fontan circulation, there is typically no right atrial reservoir, and a dual upper and lower peripheral venous cannulation may be necessary if peripheral VA cannulation is being used.39 Ascending aortic cannulation can also be challenging after Stage 1 Norwood or Damus–Kaye–Stansel-type operations (performed for hypoplastic left heart) due to multiple suture lines.
For patients with an RV supporting the systemic circulation and in need of a VAD, the triangular geometry and the hypertrophy of the morphological RV frequently necessitate trabecular resection and/or alternate positioning of the inflow cannula, often on the diaphragmatic surface or free wall (Figure 7). Pre-operative imaging and in some cases three-dimensional printing may be helpful to delineate cardiac anatomy. In patients with transposition of the great arteries palliated with an atrial switch operation (Senning or Mustard), the atria are unlikely to accommodate venous drainage cannulas. The baffles in this anatomic constellation may require higher CVP due to the inherent diastolic dysfunction from baffle stiffness.
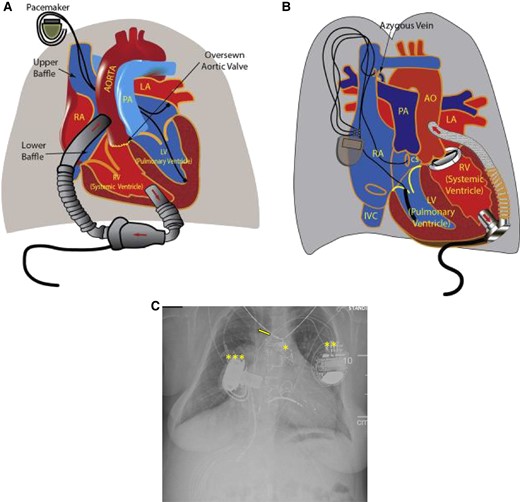
Illustration detailing the orientation of ventricular assist device (VAD) placement and the direction of blood flow using an axial flow HeartMate II (Abbott Laboratories, Chicago, Illinois) in a patient with d-transposition of the great arteries (TGA) with atrial switch (A) and a centrifugal flow HVAD (Medtronic Inc., Minnesota, Minnesota) in an l-TGA (B). Reprinted with permission from Menachem et al. AO, aorta; CS, coronary sinus; IVC, inferior vena cava; LA, left atrium; LV, left ventricle; PA, pulmonary artery; RA right atrium. (C) Chest radiograph from an adult with pulmonary atresia with intact ventricular septum s/p transcatheter pulmonary valve implantation (*), CRT-D implantation (**), and right ventricular HeartWare VAD implantation (***). A right central line present (arrow).
Other general considerations for ECMO support in ACHD patients include need for closure of residual shunts to avoid systemic embolization and circular shunting, as well as repair of AV valves.
Blood flow physiology and baseline saturations are important considerations for venovenous (VV) ECMO use, when used to treat respiratory failure complicated by severe desaturations. Baseline venous and arterial saturations may be different in ACHD patients secondary to congenital or acquired intracardiac shunts and/or systemic and pulmonary circulations that are partially or completely parallel rather than in series.
Health equity and access
There is increasing focus and recognition of the numerous health disparities that exist for patients living with CHD.40 While there are very limited data on healthcare disparities experienced by ACHD patients in the ICU setting, the field is rapidly evolving. With regard to health outcomes for ACHD patients, geography may be as important as more traditional risk factors for adverse health disparities, including race, sex, and socioeconomic status. One study found that gaps in outpatient care were common for ACHD patients both during the transition from paediatric to adult cardiology care and later in life with clinic location significantly predicting the likelihood of a patient experiencing a gap in care.41 A more recent study similarly found that ACHD patients living in the most disadvantaged areas (as assessed by the area deprivation index) are more likely to be younger, Hispanic, of non-white race, female, reliant upon Medicaid insurance and have severe CHD as compared to those living in the most advantaged areas.42 Moreover, patients from the most disadvantaged areas were significantly more likely to require cardiac care in the emergency or inpatient setting. While this study did not specifically assess critical care admissions, the same trends in disparities among care utilization may extend to the ICU setting. Future work will need to identify and target health inequities in ICU outcomes for ACHD patients.
Palliative and end-of-life care
Despite impressive advances in care for patients with CHD, the median age of death remains less than 50 years.43 Patients with CHD have a lifetime of medical interactions and, as a result, may have different concerns and perspectives from other patients with serious illness complications later in life. There has been an increasing awareness of the importance of recognizing ACHD patients’ experiences nearing end of life and their views on topics such as palliative care and advance care planning.44 A retrospective mortality study of 390 ACHD patients from an administrative and clinical database in Belgium revealed that 87% of patients were hospitalized, 78% of patients had an emergency room visit, and 19% of patients had an ICU admission in their final year of life.45 Steiner et al. reported that approximately 40% of ACHD patients experienced inpatient and ICU hospitalizations in the last 30 days of life and 64% died in the hospital. Compared to patients with cancer, ACHD patients were more likely to have inpatient [adjusted risk ratio 1.57; 95% confidence interval (CI) 1.12–2.18] and ICU admissions in the last 30 days of life (adjusted risk ratio 2.56; 95% CI 1.83–3.61), and more likely to die in the hospital (adjusted risk ratio 1.75; 95% CI 1.43–2.13). Importantly, ACHD patients were more likely to have documentation of advance care planning (adjusted risk ratio 1.46; 95% CI 1.09–1.96) than those with malignancies.46 In a recent American Heart Association scientific statement, inclusion of specialty-trained palliative care clinicians embedded in teams caring for patients and families with CHD was recommended.47
Conclusions
The management of critically ill adults with CHD requires an understanding of the unique aspects of the congenital cardiac physiology as well as the recognition of other organ systems that may be involved. Adult congenital heart disease patients most commonly present to the ICU for management of arrhythmias, acute decompensated HF, shock, and bleeding/thrombosis. Intensivists must adapt and apply their expertise with standard ICU therapies (i.e. mechanical ventilation, vasoactive support, MCS, etc.) through the lens of a congenital cardiologist more familiar with unusual underlying anatomy and physiology. While certain patients may be candidates for MCS, it is extremely important to establish goals of care with advanced care planning given the high rate of resource utilization at end of life in this complex population.
References
Author notes
Conflict of interest: A.M.V. is supported by the Sarah M. Liamos Fund for Adult Congenital Heart Disease Research.
- cardiac arrhythmia
- eisenmenger complex
- fontan procedure
- hemodynamics
- care of intensive care unit patient
- cardiac support procedures
- heart diseases
- congenital heart defects
- congenital heart disease
- heart failure
- right ventricle
- adult
- comorbidity
- critical illness
- intensive care
- intensive care unit
- heart
- physiology
- decompensation
- advance care planning
- care goals
Comments