-
PDF
- Split View
-
Views
-
Cite
Cite
Martin Reindl, Ivan Lechner, Magdalena Holzknecht, Christina Tiller, Priscilla Fink, Fritz Oberhollenzer, Agnes Mayr, Felix Troger, Mathias Pamminger, Benjamin Henninger, Markus Theurl, Gert Klug, Christoph Brenner, Axel Bauer, Bernhard Metzler, Sebastian J Reinstadler, Improved detection of echocardiographically occult left ventricular thrombi following ST-elevation myocardial infarction, European Heart Journal. Acute Cardiovascular Care, Volume 12, Issue 10, October 2023, Pages 703–710, https://doi.org/10.1093/ehjacc/zuad069
- Share Icon Share
Abstract
The aim of this study was to investigate predictors of transthoracic echocardiography (TTE)-occult left ventricular (LV) thrombi (LVT) and to propose a clinical model for improved detection of TTE-occult LVT post–ST-elevation myocardial infarction (STEMI). Patients with acute STEMI are at significant risk for developing LVT. However, this complication often (up to 65%) remains undetected by using TTE, referred to as TTE-occult LVT.
In total, 870 STEMI patients underwent TTE and cardiac magnetic resonance (CMR), the reference method for LVT detection, 3 days after infarction. Clinical (body mass index, peak cardiac troponin T) and echocardiographic [ejection fraction, apical wall motion scores (AWMSs)] predictors were analysed. Primary endpoint was the presence of TTE-occult LVT identified by CMR imaging. From the overall cohort, 37 patients (4%) showed an LVT by CMR. Of these thrombi, 25 (68%) were not identified by TTE. Transthoracic echocardiography-occult thrombi did not significantly differ in volume (1.4 vs. 2.74 cm3), diameter (19.0 vs. 23.3 mm), and number of fragments or shape compared with TTE-apparent LVT (all P > 0.05). For predicting these TTE-occult LVT, the 16-segment AWMS (AWMS16Seg) showed highest validity {area under the curve: 0.91 [95% confidence interval (CI): 0.89–0.93]; P < 0.001}, with an association independent of ejection fraction and 17-segment AWMS (AWMS17Seg) [odds ratio: 1.68 (95% CI: 1.43–1.97); P < 0.001] and clinical (body mass index, peak troponin) and angiographic (culprit lesion, post-interventional thrombolysis in myocardial infarction flow) associates of TTE-occult LVT (all P < 0.05). Dichotomization at AWMS16Seg ≥ 8 (n = 260, 30%) allowed for a detection of all TTE-occult LVT (sensitivity: 100%), with a corresponding specificity of 77%.
After acute STEMI, AWMS16Seg served as a simple and very robust predictor of TTE-occult LVT. An AWMS16Seg-based algorithm to identify patients for additional CMR imaging offers great potential to optimize detection of TTE-occult LVT following STEMI.
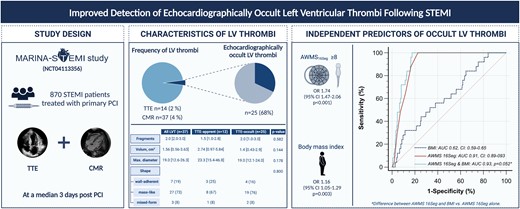
This study is based on data from the Magnetic Resonance Imaging In Acute ST-Elevation Myocardial Infarction study, which evaluated 870 ST-elevation myocardial infarction patients treated by primary percutaneous coronary intervention and who underwent transthoracic echocardiography (TTE) and cardiac magnetic resonance (CMR) after a median time of 3 days after infarction, for the evaluation of left ventricular thrombi (LVT). Of the total study cohort (left pie chart), 4% (n = 37) of patients presented with a LVT, detected by CMR (dark area). From those LVT, a total of 14 LVT (including two false positives) were detected by TTE. Accordingly, 25 LVT (68%) were classified as TTE-occult LVT, represented by the hatched line of the right pie chart. Although TTE-occult thrombi had smaller diameter and volume, these differences were not statistically significant. In multivariable logistic regression analysis, the 16-segment apical wall motion score (AWMS16Seg) and body mass index emerged as independent predictors of TTE-occult LVT. The optimal cut-off value of AWMS16Seg (≥8) yielded an area under the curve (AUC) of 0.91 for the detection of TTE-occult LVT. Although the combination of AWMS16Seg and body mass index resulted in an even higher AUC (0.93), this combination was not significantly better as compared with AWMS16Seg alone. CI, confidence interval; OR: odds ratio. ‘Created with Biorender’.
Introduction
In patients with ST-elevation myocardial infarction (STEMI) treated with primary percutaneous coronary intervention (PCI), the occurrence of left ventricular (LV) thrombus (LVT) is still common with an incidence of ∼4–6% in the early phase after infarction.1,2 The presence of LVT following STEMI is independently associated with adverse clinical outcomes.2,3 In particular, LVT formation may result in stroke and systemic thromboembolism (∼five-fold increased risk), which can potentially be prevented by early LVT detection and appropriate treatment (reported risk reduction of 85%).4–6
Current clinical practice guidelines recommend routine transthoracic echocardiography (TTE) after PCI for STEMI to identify or exclude early LVT.7,8 However, published data showed that the value of TTE for detecting LVT is very limited. In fact, a previous analysis reported a sensitivity of 35% for non-contrast-TTE and 64% for contrast-TTE; therefore, a relevant number of LVT remain TTE-occult.9 Cardiac magnetic resonance (CMR) imaging, on the other hand, is the current gold standard modality for detecting LVT (sensitivity: 87%, specificity: 99%).5,10 However, CMR is not part of routine care after STEMI. As TTE-occult LVT detected only by CMR are associated with a similar embolic risk as those detected by both TTE and CMR,11 improved detection of TTE-occult LVT is warranted to further improve clinical outcomes post-STEMI.
The present study, therefore, aimed to (i) determine the incidence of TTE-occult LVT in a large PCI-treated STEMI cohort undergoing CMR imaging and (ii) identify clinical and echocardiographic parameters for improved detection of TTE-occult LVT.
Methods
Patient population and endpoint definition
For the present analysis, 870 STEMI patients enrolled in the prospective ‘Magnetic Resonance Imaging In Acute ST-Elevation Myocardial Infarction’ trial12,13 (NCT04113356) between 2011 and 2022 were analysed. Inclusion criteria were defined as first-ever STEMI diagnosed according to the European Society of Cardiology/American College of Cardiology/American Heart Association committee criteria7,8 and re-vascularized by primary PCI within 24 h. Exclusion criteria were defined as inability or unwillingness to sign written informed consent, an age <18 years, any history of a previous myocardial infarction or coronary intervention, Killip class ≥3 at time of CMR scan, an estimated glomerular filtration rate <30 mL/min per 1.73 m2, and any other specific contraindication to CMR (pacemaker, severe claustrophobia, orbital foreign body, cerebral aneurysm clip, and known or suggested contrast agent allergy to gadolinium).
For biomarker analysis, serial measurements of high-sensitivity cardiac troponin T were performed using an enzyme immunoassay (high-sensitivity cardiac troponin T; E 170, Roche Diagnostics, Vienna, Austria).
Prior to study inclusion, written informed consent was given by all participants. The study was designed and conducted in compliance with the Declaration of Helsinki and received approval by the research Ethics Committee of the Medical University of Innsbruck.
Primary study endpoint was the presence of TTE-occult thrombus, defined as LVT detected by CMR but not by TTE.
Cardiac magnetic resonance imaging
All CMR scans were conducted on a 1.5 Tesla Magnetom AVANTO-scanner (Siemens® Healthineers AG, Erlangen, Germany) within the first week after treatment with primary PCI. The standardized imaging protocol of our research group was published in detail previously.14,15 Cine images were acquired by using breath-hold, retrospective electrocardiogram-triggered true-FISP bright-blood sequences. Electrocardiogram-triggered, phase-sensitive inversion recovery sequences were used to obtain late gadolinium enhancement (LGE) images 15–20 min after application of a 0.2 mmol/kg bolus of contrast agent (Gadovist®, Bayer, Leverkusen, Germany).
Left ventricular thrombi were diagnosed as an intracavitary mass with low-intensity tissue characteristics on LGE images, delineated by high-signal intensity structures (e.g. infarcted myocardium and blood pool) irrespective of location or morphology. By using a PACS integrated locating function, short- and long-axis cine images were precisely screened for a corresponding morphological correlate of a mural or protruding LV lesion, typically adherent to regions with wall motion abnormality. Left ventricular thrombi were carefully distinguished from microvascular obstruction, papillary muscles, trabeculae, chordal structures, tangential views of the LV wall, and technical artefacts.2 If an exact differentiation between microvascular obstruction and wall-adherent thrombus was doubtful, LGE sequence was repeated using a ‘long-inversion’ time of ∼550 ms. If diagnosis still remained unclear, the LGE sequence was repeated serially to detect microvascular obstruction by gradually filling it with contrast medium. This was continued until the differentiation between these entities was finally clarified. The presence or absence of LVT on CMR was evaluated by two experienced examiners, blinded to each other and to the clinical data.
Transthoracic echocardiography
Transthoracic echocardiography scans were obtained within the first week after PCI at the Echocardiography Laboratory of the Heart Centre Innsbruck using Philips EPIQ Elite, PureWave and CVx devices. Post-processing was conducted using Image Arena (TOMTEC Imaging Systems, Unterschleissheim, Germany). Left ventricular ejection fraction (LVEF) was determined according to the biplane Simpson method. Left ventricular thrombi were defined as independent LV intracavitary mass distinguishable from papillary muscles, trabeculae, chordal structures, tangential views of the LV wall, and technical artefacts.9,16 The use of contrast agent was at the discretion of the examiner. Apical wall motion was assessed by two different scoring systems, the 17-segment apical wall motion score (AWMS17Seg) and the 16-segment AWMS (AWMS16Seg). The AWMS17Seg was determined according to the 17-segment model,17 for which segmental contraction was graded in the following way: 0 = normal, 1 = mild hypokinesis, 2 = moderate hypokinesis, 3 = severe hypokinesis, 4 = akinesis, and 5 = dyskinesis.9 For the AWMS16Seg, the 16-segment model with a four-grade scoring system for segmental contraction (1 = normal, 2 = hypokinesis, 3 = akinesis, and 4 = dyskinesis) was used.18 Echocardiographic analyses were performed by two experienced cardiologists with a TTE EACVI certification, blinded to clinical and CMR data.
Statistical analyses
Continuous variables were expressed as median with interquartile range (IQR) and categorical variables as numbers with corresponding percentages. Differences in continuous and categorical variables between two groups were tested by Mann–Whitney U-test and χ2 test, respectively. Univariable and multivariable logistic regression analyses were performed to disclose predictors of TTE-occult LVT. All variables in Table 1 showing a P-value of <0.10 in univariable testing for TTE-occult thrombus were included into multivariable logistic regression analysis. To ensure statistical robustness, three multivariable models were created Table 2. Receiver operating curve (ROC) analysis was performed to assess the discriminative power of echocardiographic parameters for detection of TTE-occult LVT. According to Rice and Harris, the area under the curve (AUC) values were interpreted as negligible (≤0.55), small (0.56–0.63), moderate (0.64–0.70), and strong (≥0.71).19 Dichotomization of echocardiographic parameters for the detection of TTE-occult LVT was performed by using the Youden index and, to establish an optimized screening tool, the cut-off with highest sensitivity. All tests were two-tailed, and a P-value of <0.05 was considered statistically significant. SPSS Statistics 28.0.1 (IBM, Armonk, NY, USA) and MedCalc v 22.0.22 (Ostend, Belgium) were used for statistical analyses.
. | Total population (n = 870) . | No LVT/TTE-apparent LVT (n = 845, 97%) . | TTE-occult LVT (n = 25, 3%) . | P-value . |
---|---|---|---|---|
Age, years | 57 (51–66) | 58 (51–66) | 55 (52–63) | 0.567 |
Female, n (%) | 146 (17) | 144 (17) | 2 (8) | 0.465 |
Body mass index, kg/m2 | 26.2 (24.4–28.7) | 26.1 (24.4–28.7) | 27.8 (25.0–31.7) | 0.038 |
Hypertension, n (%) | 419 (48) | 412 (49) | 9 (36) | 0.206 |
Current smoker, n (%) | 467 (54) | 454 (54) | 13 (52) | 0.859 |
Hyperlipidaemia, n (%) | 490 (56) | 479 (57) | 11 (44) | 0.205 |
Diabetes mellitus, n (%) | 74 (9) | 70 (8) | 4 (16) | 0.174 |
Total ischaemia time, min | 186 (120–312) | 185 (120–311) | 213 (147–374) | 0.357 |
Door-to-balloon time, min | 25 (7–53) | 25 (7–54) | 30 (11–44) | 0.849 |
Peak cTnT, ng/L | 5005 (2455–8551) | 4890 (2385–8245) | 8638 (5102–15 050) | <0.001 |
Culprit lesion, n (%) | <0.001 | |||
RCA | 338 (39) | 336 (40) | 2 (8) | |
LAD | 403 (46) | 380 (45) | 23 (92) | |
LCX | 122 (14) | 122 (14) | 0 (0) | |
LM | 1 (<1) | 1 (<1) | 0 (0) | |
RI | 6 (1) | 6 (1) | 0 (0) | |
Infarct location (%) | <0.001 | |||
Anterior | 403 (46) | 380 (45) | 23 (92) | |
Non-anterior | 467 (54) | 465 (55) | 2 (8) | |
Number of affected vessels, n (%) | 0.137 | |||
1 | 501 (58) | 482 (57) | 19 (76) | |
2 | 244 (28) | 241 (29) | 3 (12) | |
3 | 125 (14) | 122 (14) | 3 (12) | |
TIMI flow pre-PCI, n (%) | 0.572 | |||
0 | 566 (65) | 547 (64) | 19 (76) | |
1 | 136 (16) | 133 (16) | 3 (12) | |
2 | 128 (15) | 125 (15) | 3 (12) | |
3 | 40 (4) | 40 (5) | 0 (0) | |
TIMI flow post-PCI, n (%) | 0.128 | |||
0 | 11 (1) | 10 (1) | 1 (4) | |
1 | 8 (1) | 7 (1) | 1 (4) | |
2 | 84 (10) | 80 (9) | 4 (16) | |
3 | 767 (88) | 748 (89) | 19 (76) | |
Echocardiographic parameters | ||||
LVEF, % | 50 (43–57) | 50 (44–57) | 39 (35–41) | <0.001 |
AWMS17Seg | 3 (0–14) | 3 (0–13) | 17 (16–20) | <0.001 |
AWMS16Seg | 5 (4–8) | 5 (4–8) | 11 (10–13) | <0.001 |
cMRI-apparent LV thrombus | 37 (4) | 12 (1) | 25 (100) | <0.001 |
TTE-apparent LV thrombus | 14 (2) | 14 (2) | 0 (0) | 0.516 |
Time to TTE, days | 3 (2–4) | 3 (2–4) | 2 (2–4) | 0.329 |
Time to MRI, days | 3 (2–5) | 3 (2–5) | 4 (2–6) | 0.236 |
Antithrombotic therapy | ||||
Aspirin, n (%) | 862 (99) | 838 (99) | 24 (96) | 0.102 |
P2Y12 inhibitors | 870 (100) | 845 (100) | 25 (100) | — |
Anticoagulant, n (%) | 59 (7) | 34 (4) | 25 (100) | <0.001 |
NOAC | 37 (4) | 24 (3) | 13 (52) | |
Vitamin K antagonist | 22 (3) | 10 (1) | 12 (48) |
. | Total population (n = 870) . | No LVT/TTE-apparent LVT (n = 845, 97%) . | TTE-occult LVT (n = 25, 3%) . | P-value . |
---|---|---|---|---|
Age, years | 57 (51–66) | 58 (51–66) | 55 (52–63) | 0.567 |
Female, n (%) | 146 (17) | 144 (17) | 2 (8) | 0.465 |
Body mass index, kg/m2 | 26.2 (24.4–28.7) | 26.1 (24.4–28.7) | 27.8 (25.0–31.7) | 0.038 |
Hypertension, n (%) | 419 (48) | 412 (49) | 9 (36) | 0.206 |
Current smoker, n (%) | 467 (54) | 454 (54) | 13 (52) | 0.859 |
Hyperlipidaemia, n (%) | 490 (56) | 479 (57) | 11 (44) | 0.205 |
Diabetes mellitus, n (%) | 74 (9) | 70 (8) | 4 (16) | 0.174 |
Total ischaemia time, min | 186 (120–312) | 185 (120–311) | 213 (147–374) | 0.357 |
Door-to-balloon time, min | 25 (7–53) | 25 (7–54) | 30 (11–44) | 0.849 |
Peak cTnT, ng/L | 5005 (2455–8551) | 4890 (2385–8245) | 8638 (5102–15 050) | <0.001 |
Culprit lesion, n (%) | <0.001 | |||
RCA | 338 (39) | 336 (40) | 2 (8) | |
LAD | 403 (46) | 380 (45) | 23 (92) | |
LCX | 122 (14) | 122 (14) | 0 (0) | |
LM | 1 (<1) | 1 (<1) | 0 (0) | |
RI | 6 (1) | 6 (1) | 0 (0) | |
Infarct location (%) | <0.001 | |||
Anterior | 403 (46) | 380 (45) | 23 (92) | |
Non-anterior | 467 (54) | 465 (55) | 2 (8) | |
Number of affected vessels, n (%) | 0.137 | |||
1 | 501 (58) | 482 (57) | 19 (76) | |
2 | 244 (28) | 241 (29) | 3 (12) | |
3 | 125 (14) | 122 (14) | 3 (12) | |
TIMI flow pre-PCI, n (%) | 0.572 | |||
0 | 566 (65) | 547 (64) | 19 (76) | |
1 | 136 (16) | 133 (16) | 3 (12) | |
2 | 128 (15) | 125 (15) | 3 (12) | |
3 | 40 (4) | 40 (5) | 0 (0) | |
TIMI flow post-PCI, n (%) | 0.128 | |||
0 | 11 (1) | 10 (1) | 1 (4) | |
1 | 8 (1) | 7 (1) | 1 (4) | |
2 | 84 (10) | 80 (9) | 4 (16) | |
3 | 767 (88) | 748 (89) | 19 (76) | |
Echocardiographic parameters | ||||
LVEF, % | 50 (43–57) | 50 (44–57) | 39 (35–41) | <0.001 |
AWMS17Seg | 3 (0–14) | 3 (0–13) | 17 (16–20) | <0.001 |
AWMS16Seg | 5 (4–8) | 5 (4–8) | 11 (10–13) | <0.001 |
cMRI-apparent LV thrombus | 37 (4) | 12 (1) | 25 (100) | <0.001 |
TTE-apparent LV thrombus | 14 (2) | 14 (2) | 0 (0) | 0.516 |
Time to TTE, days | 3 (2–4) | 3 (2–4) | 2 (2–4) | 0.329 |
Time to MRI, days | 3 (2–5) | 3 (2–5) | 4 (2–6) | 0.236 |
Antithrombotic therapy | ||||
Aspirin, n (%) | 862 (99) | 838 (99) | 24 (96) | 0.102 |
P2Y12 inhibitors | 870 (100) | 845 (100) | 25 (100) | — |
Anticoagulant, n (%) | 59 (7) | 34 (4) | 25 (100) | <0.001 |
NOAC | 37 (4) | 24 (3) | 13 (52) | |
Vitamin K antagonist | 22 (3) | 10 (1) | 12 (48) |
AWMS, apical wall motion score; cTnT, cardiac troponin T; LAD, left anterior descending artery; LCX, left circumflex artery; LVEF, left ventricular ejection fraction; NOAC, non-vitamin K antagonist oral anticoagulant; PCI, percutaneous coronary intervention; RCA, right coronary artery; RI, ramus intermedius; TIMI, thrombolysis in myocardial infarction. Statistically significant differences, defined as P-value <0.05, are highlighted in bold.
. | Total population (n = 870) . | No LVT/TTE-apparent LVT (n = 845, 97%) . | TTE-occult LVT (n = 25, 3%) . | P-value . |
---|---|---|---|---|
Age, years | 57 (51–66) | 58 (51–66) | 55 (52–63) | 0.567 |
Female, n (%) | 146 (17) | 144 (17) | 2 (8) | 0.465 |
Body mass index, kg/m2 | 26.2 (24.4–28.7) | 26.1 (24.4–28.7) | 27.8 (25.0–31.7) | 0.038 |
Hypertension, n (%) | 419 (48) | 412 (49) | 9 (36) | 0.206 |
Current smoker, n (%) | 467 (54) | 454 (54) | 13 (52) | 0.859 |
Hyperlipidaemia, n (%) | 490 (56) | 479 (57) | 11 (44) | 0.205 |
Diabetes mellitus, n (%) | 74 (9) | 70 (8) | 4 (16) | 0.174 |
Total ischaemia time, min | 186 (120–312) | 185 (120–311) | 213 (147–374) | 0.357 |
Door-to-balloon time, min | 25 (7–53) | 25 (7–54) | 30 (11–44) | 0.849 |
Peak cTnT, ng/L | 5005 (2455–8551) | 4890 (2385–8245) | 8638 (5102–15 050) | <0.001 |
Culprit lesion, n (%) | <0.001 | |||
RCA | 338 (39) | 336 (40) | 2 (8) | |
LAD | 403 (46) | 380 (45) | 23 (92) | |
LCX | 122 (14) | 122 (14) | 0 (0) | |
LM | 1 (<1) | 1 (<1) | 0 (0) | |
RI | 6 (1) | 6 (1) | 0 (0) | |
Infarct location (%) | <0.001 | |||
Anterior | 403 (46) | 380 (45) | 23 (92) | |
Non-anterior | 467 (54) | 465 (55) | 2 (8) | |
Number of affected vessels, n (%) | 0.137 | |||
1 | 501 (58) | 482 (57) | 19 (76) | |
2 | 244 (28) | 241 (29) | 3 (12) | |
3 | 125 (14) | 122 (14) | 3 (12) | |
TIMI flow pre-PCI, n (%) | 0.572 | |||
0 | 566 (65) | 547 (64) | 19 (76) | |
1 | 136 (16) | 133 (16) | 3 (12) | |
2 | 128 (15) | 125 (15) | 3 (12) | |
3 | 40 (4) | 40 (5) | 0 (0) | |
TIMI flow post-PCI, n (%) | 0.128 | |||
0 | 11 (1) | 10 (1) | 1 (4) | |
1 | 8 (1) | 7 (1) | 1 (4) | |
2 | 84 (10) | 80 (9) | 4 (16) | |
3 | 767 (88) | 748 (89) | 19 (76) | |
Echocardiographic parameters | ||||
LVEF, % | 50 (43–57) | 50 (44–57) | 39 (35–41) | <0.001 |
AWMS17Seg | 3 (0–14) | 3 (0–13) | 17 (16–20) | <0.001 |
AWMS16Seg | 5 (4–8) | 5 (4–8) | 11 (10–13) | <0.001 |
cMRI-apparent LV thrombus | 37 (4) | 12 (1) | 25 (100) | <0.001 |
TTE-apparent LV thrombus | 14 (2) | 14 (2) | 0 (0) | 0.516 |
Time to TTE, days | 3 (2–4) | 3 (2–4) | 2 (2–4) | 0.329 |
Time to MRI, days | 3 (2–5) | 3 (2–5) | 4 (2–6) | 0.236 |
Antithrombotic therapy | ||||
Aspirin, n (%) | 862 (99) | 838 (99) | 24 (96) | 0.102 |
P2Y12 inhibitors | 870 (100) | 845 (100) | 25 (100) | — |
Anticoagulant, n (%) | 59 (7) | 34 (4) | 25 (100) | <0.001 |
NOAC | 37 (4) | 24 (3) | 13 (52) | |
Vitamin K antagonist | 22 (3) | 10 (1) | 12 (48) |
. | Total population (n = 870) . | No LVT/TTE-apparent LVT (n = 845, 97%) . | TTE-occult LVT (n = 25, 3%) . | P-value . |
---|---|---|---|---|
Age, years | 57 (51–66) | 58 (51–66) | 55 (52–63) | 0.567 |
Female, n (%) | 146 (17) | 144 (17) | 2 (8) | 0.465 |
Body mass index, kg/m2 | 26.2 (24.4–28.7) | 26.1 (24.4–28.7) | 27.8 (25.0–31.7) | 0.038 |
Hypertension, n (%) | 419 (48) | 412 (49) | 9 (36) | 0.206 |
Current smoker, n (%) | 467 (54) | 454 (54) | 13 (52) | 0.859 |
Hyperlipidaemia, n (%) | 490 (56) | 479 (57) | 11 (44) | 0.205 |
Diabetes mellitus, n (%) | 74 (9) | 70 (8) | 4 (16) | 0.174 |
Total ischaemia time, min | 186 (120–312) | 185 (120–311) | 213 (147–374) | 0.357 |
Door-to-balloon time, min | 25 (7–53) | 25 (7–54) | 30 (11–44) | 0.849 |
Peak cTnT, ng/L | 5005 (2455–8551) | 4890 (2385–8245) | 8638 (5102–15 050) | <0.001 |
Culprit lesion, n (%) | <0.001 | |||
RCA | 338 (39) | 336 (40) | 2 (8) | |
LAD | 403 (46) | 380 (45) | 23 (92) | |
LCX | 122 (14) | 122 (14) | 0 (0) | |
LM | 1 (<1) | 1 (<1) | 0 (0) | |
RI | 6 (1) | 6 (1) | 0 (0) | |
Infarct location (%) | <0.001 | |||
Anterior | 403 (46) | 380 (45) | 23 (92) | |
Non-anterior | 467 (54) | 465 (55) | 2 (8) | |
Number of affected vessels, n (%) | 0.137 | |||
1 | 501 (58) | 482 (57) | 19 (76) | |
2 | 244 (28) | 241 (29) | 3 (12) | |
3 | 125 (14) | 122 (14) | 3 (12) | |
TIMI flow pre-PCI, n (%) | 0.572 | |||
0 | 566 (65) | 547 (64) | 19 (76) | |
1 | 136 (16) | 133 (16) | 3 (12) | |
2 | 128 (15) | 125 (15) | 3 (12) | |
3 | 40 (4) | 40 (5) | 0 (0) | |
TIMI flow post-PCI, n (%) | 0.128 | |||
0 | 11 (1) | 10 (1) | 1 (4) | |
1 | 8 (1) | 7 (1) | 1 (4) | |
2 | 84 (10) | 80 (9) | 4 (16) | |
3 | 767 (88) | 748 (89) | 19 (76) | |
Echocardiographic parameters | ||||
LVEF, % | 50 (43–57) | 50 (44–57) | 39 (35–41) | <0.001 |
AWMS17Seg | 3 (0–14) | 3 (0–13) | 17 (16–20) | <0.001 |
AWMS16Seg | 5 (4–8) | 5 (4–8) | 11 (10–13) | <0.001 |
cMRI-apparent LV thrombus | 37 (4) | 12 (1) | 25 (100) | <0.001 |
TTE-apparent LV thrombus | 14 (2) | 14 (2) | 0 (0) | 0.516 |
Time to TTE, days | 3 (2–4) | 3 (2–4) | 2 (2–4) | 0.329 |
Time to MRI, days | 3 (2–5) | 3 (2–5) | 4 (2–6) | 0.236 |
Antithrombotic therapy | ||||
Aspirin, n (%) | 862 (99) | 838 (99) | 24 (96) | 0.102 |
P2Y12 inhibitors | 870 (100) | 845 (100) | 25 (100) | — |
Anticoagulant, n (%) | 59 (7) | 34 (4) | 25 (100) | <0.001 |
NOAC | 37 (4) | 24 (3) | 13 (52) | |
Vitamin K antagonist | 22 (3) | 10 (1) | 12 (48) |
AWMS, apical wall motion score; cTnT, cardiac troponin T; LAD, left anterior descending artery; LCX, left circumflex artery; LVEF, left ventricular ejection fraction; NOAC, non-vitamin K antagonist oral anticoagulant; PCI, percutaneous coronary intervention; RCA, right coronary artery; RI, ramus intermedius; TIMI, thrombolysis in myocardial infarction. Statistically significant differences, defined as P-value <0.05, are highlighted in bold.
Results
Baseline patient characteristics
Median age of the overall cohort (n = 870) was 57 (IQR: 51–66) years, and 17% (n = 146) were female. All patients underwent primary PCI after a median total ischaemic time of 186 min (IQR: 120–312). The baseline patient characteristics are summarized in Table 1. A detailed flow chart of the study is provided in the Supplementary section.
Left ventricle thrombus formation after ST-elevation myocardial infarction
Cardiac magnetic resonance examinations for the assessment of LVT were performed 3 (IQR: 2–5) days after infarction. From the overall cohort, 37 (4%) patients showed an LVT by CMR. All LVT were apically located. In the vast majority (n = 35, 95%) of patients with LVT, the left anterior descending artery was the culprit lesion. In two patients, LVT developed due to occlusion of the right coronary artery, which was a large vessel that supplied a major part of the apex.
Echocardiograms were conducted 3 (IQR: 2–4) days after infarction (median time difference between TTE and CMR: 15 h).
Of the 37 (4%) thrombi detected by CMR, 25 (68%) were not identified by TTE (23 non-contrast and 2 contrast-enhanced echocardiograms), thus referred to as TTE-occult LVT (3% of the overall cohort). In two patients, an LVT was reported by TTE, although no thrombus was identified by CMR. Accordingly, TTE provided a sensitivity of 32.4% [95% confidence interval (CI): 18.0–49.8] and a specificity of 99.8% (95% CI: 99.1–100) for LVT detection. There were no significant differences in thrombus volume, size, number of fragments, and shape between TTE-apparent and TTE-occult LVT (all P > 0.05, Table 3).
All differences in baseline characteristics according to TTE-apparent and TTE-occult LVT are summarized in Table 1. Briefly, patients with TTE-occult LVT had higher peak troponin concentrations (median 8638 vs. 4890 ng/L, P < 0.001) and were more commonly affected by anterior infarct location (92 vs. 45%, P < 0.001). Furthermore, patients with TTE-occult LVT presented with a higher body mass index as compared with those with TTE-apparent thrombi (27.8 vs. 26.1 kg/m2, P = 0.038).
Determinants of transthoracic echocardiography–occult left ventricular thrombus
The median LVEF of the overall cohort was 50% (IQR: 43–57). AWMS17Seg and AWMS16Seg were three (IQR: 0–14) and five (IQR: 4–8) points, respectively. Patients with TTE-occult LVT showed a significantly lower LVEF (median 39 vs. 50%, P < 0.001) and higher AWMS17Seg (median 17 vs. 3 points, P < 0.001) and AWMS16Seg (median 11 vs. 5 points, P < 0.001; Table 1).
The univariable and multivariable logistic regression analyses for the detection of TTE-occult LVT are presented in detail in Table 2. In the multivariable echocardiographic model including LVEF, AWMS17Seg, and AWMS16Seg, only AWMS16Seg emerged as an independent predictor of TTE-occult LVT [odds ratio (OR): 1.68 (95% CI: 1.43–1.97), P < 0.001]. In the clinical model, AWMS16Seg and body mass index emerged as independent predictors of TTE-occult LVT after adjustment for univariable associates [OR: 1.74 (95% CI: 1.47–2.06), P < 0.001 and OR: 1.16 (95% CI: 1.05–1.29), P = 0.003, respectively]. The association between AWMS16Seg and TTE-occult LVT remained significant also after adjustment for infarct location and post-interventional thrombolysis in myocardial infarction (TIMI) flow [OR: 1.68 (95% CI: 1.43–1.97), P < 0.001; angiographic model of Table 2]. These findings are further illustrated in the Graphical abstract.
Logistic regression analysis for the detection of a transthoracic echocardiography-occult left ventricular thrombus
. | Univariable . | Multivariable . | ||
---|---|---|---|---|
. | OR (95% CI) . | P-value . | OR (95% CI) . | P-value . |
Echocardiographic model | ||||
AWMS16Seg | 1.68 (1.43–1.97) | <0.001 | 1.68 (1.43–1.97) | <0.001 |
AWMS17Seg | 1.36 (1.19–1.54) | <0.001 | — | — |
LVEF, % | 0.89 (0.85–0.93) | <0.001 | — | — |
Clinical model | ||||
AWMS16Seg | 1.68 (1.43–1.97) | <0.001 | 1.74 (1.47–2.06) | <0.001 |
Body mass index | 1.09 (1.00–1.19) | 0.040 | 1.16 (1.05–1.29) | 0.003 |
Peak cTnT | 1.00 (1.00–1.00) | 0.003 | — | — |
Angiographic model | ||||
AWMS16Seg | 1.68 (1.43–1.97) | <0.001 | 1.68 (1.43–1.97) | <0.001 |
Infarct location | 14.07 (3.30–60.07) | <0.001 | — | — |
TIMI flow post-PCI | 0.57 (0.33–0.96) | 0.035 | — | — |
. | Univariable . | Multivariable . | ||
---|---|---|---|---|
. | OR (95% CI) . | P-value . | OR (95% CI) . | P-value . |
Echocardiographic model | ||||
AWMS16Seg | 1.68 (1.43–1.97) | <0.001 | 1.68 (1.43–1.97) | <0.001 |
AWMS17Seg | 1.36 (1.19–1.54) | <0.001 | — | — |
LVEF, % | 0.89 (0.85–0.93) | <0.001 | — | — |
Clinical model | ||||
AWMS16Seg | 1.68 (1.43–1.97) | <0.001 | 1.74 (1.47–2.06) | <0.001 |
Body mass index | 1.09 (1.00–1.19) | 0.040 | 1.16 (1.05–1.29) | 0.003 |
Peak cTnT | 1.00 (1.00–1.00) | 0.003 | — | — |
Angiographic model | ||||
AWMS16Seg | 1.68 (1.43–1.97) | <0.001 | 1.68 (1.43–1.97) | <0.001 |
Infarct location | 14.07 (3.30–60.07) | <0.001 | — | — |
TIMI flow post-PCI | 0.57 (0.33–0.96) | 0.035 | — | — |
AWMS, apical wall motion score; CI, confidence interval; cTnT, cardiac troponin T; LVEF, left ventricular ejection fraction; OR, odds ratio; PCI, percutaneous coronary intervention; TIMI, thrombolysis in myocardial infarction. Statistically significant differences, defined as P-value <0.05, are highlighted in bold.
Logistic regression analysis for the detection of a transthoracic echocardiography-occult left ventricular thrombus
. | Univariable . | Multivariable . | ||
---|---|---|---|---|
. | OR (95% CI) . | P-value . | OR (95% CI) . | P-value . |
Echocardiographic model | ||||
AWMS16Seg | 1.68 (1.43–1.97) | <0.001 | 1.68 (1.43–1.97) | <0.001 |
AWMS17Seg | 1.36 (1.19–1.54) | <0.001 | — | — |
LVEF, % | 0.89 (0.85–0.93) | <0.001 | — | — |
Clinical model | ||||
AWMS16Seg | 1.68 (1.43–1.97) | <0.001 | 1.74 (1.47–2.06) | <0.001 |
Body mass index | 1.09 (1.00–1.19) | 0.040 | 1.16 (1.05–1.29) | 0.003 |
Peak cTnT | 1.00 (1.00–1.00) | 0.003 | — | — |
Angiographic model | ||||
AWMS16Seg | 1.68 (1.43–1.97) | <0.001 | 1.68 (1.43–1.97) | <0.001 |
Infarct location | 14.07 (3.30–60.07) | <0.001 | — | — |
TIMI flow post-PCI | 0.57 (0.33–0.96) | 0.035 | — | — |
. | Univariable . | Multivariable . | ||
---|---|---|---|---|
. | OR (95% CI) . | P-value . | OR (95% CI) . | P-value . |
Echocardiographic model | ||||
AWMS16Seg | 1.68 (1.43–1.97) | <0.001 | 1.68 (1.43–1.97) | <0.001 |
AWMS17Seg | 1.36 (1.19–1.54) | <0.001 | — | — |
LVEF, % | 0.89 (0.85–0.93) | <0.001 | — | — |
Clinical model | ||||
AWMS16Seg | 1.68 (1.43–1.97) | <0.001 | 1.74 (1.47–2.06) | <0.001 |
Body mass index | 1.09 (1.00–1.19) | 0.040 | 1.16 (1.05–1.29) | 0.003 |
Peak cTnT | 1.00 (1.00–1.00) | 0.003 | — | — |
Angiographic model | ||||
AWMS16Seg | 1.68 (1.43–1.97) | <0.001 | 1.68 (1.43–1.97) | <0.001 |
Infarct location | 14.07 (3.30–60.07) | <0.001 | — | — |
TIMI flow post-PCI | 0.57 (0.33–0.96) | 0.035 | — | — |
AWMS, apical wall motion score; CI, confidence interval; cTnT, cardiac troponin T; LVEF, left ventricular ejection fraction; OR, odds ratio; PCI, percutaneous coronary intervention; TIMI, thrombolysis in myocardial infarction. Statistically significant differences, defined as P-value <0.05, are highlighted in bold.
. | All LV thrombi (n = 37) . | TTE-apparent LVT (n = 12) . | TTE-occult LVT (n = 25) . | P-value . |
---|---|---|---|---|
Fragments | 2.0 (2.0–3.0) | 1.5 (1.0–2.8) | 2.0 (1.0–3.0) | 0.582 |
Volume, cm3 | 1.56 (0.56–3.63) | 2.74 (0.97–5.84) | 1.4 (0.43–2.9) | 0.144 |
Max. diameter, mm | 19.0 (12.6–26.3) | 23.3 (15.4–46.8) | 19.0 (12.1–24.0) | 0.178 |
Shape | 0.800 | |||
Wall-adherent | 7 (19) | 3 (25) | 4 (16) | |
Mass-like | 27 (73) | 8 (67) | 19 (76) | |
Mixed-form | 3 (8) | 1 (8) | 2 (8) |
. | All LV thrombi (n = 37) . | TTE-apparent LVT (n = 12) . | TTE-occult LVT (n = 25) . | P-value . |
---|---|---|---|---|
Fragments | 2.0 (2.0–3.0) | 1.5 (1.0–2.8) | 2.0 (1.0–3.0) | 0.582 |
Volume, cm3 | 1.56 (0.56–3.63) | 2.74 (0.97–5.84) | 1.4 (0.43–2.9) | 0.144 |
Max. diameter, mm | 19.0 (12.6–26.3) | 23.3 (15.4–46.8) | 19.0 (12.1–24.0) | 0.178 |
Shape | 0.800 | |||
Wall-adherent | 7 (19) | 3 (25) | 4 (16) | |
Mass-like | 27 (73) | 8 (67) | 19 (76) | |
Mixed-form | 3 (8) | 1 (8) | 2 (8) |
LV, left ventricular; LVT, left ventricular thrombus; TTE, transthoracic echocardiography.
. | All LV thrombi (n = 37) . | TTE-apparent LVT (n = 12) . | TTE-occult LVT (n = 25) . | P-value . |
---|---|---|---|---|
Fragments | 2.0 (2.0–3.0) | 1.5 (1.0–2.8) | 2.0 (1.0–3.0) | 0.582 |
Volume, cm3 | 1.56 (0.56–3.63) | 2.74 (0.97–5.84) | 1.4 (0.43–2.9) | 0.144 |
Max. diameter, mm | 19.0 (12.6–26.3) | 23.3 (15.4–46.8) | 19.0 (12.1–24.0) | 0.178 |
Shape | 0.800 | |||
Wall-adherent | 7 (19) | 3 (25) | 4 (16) | |
Mass-like | 27 (73) | 8 (67) | 19 (76) | |
Mixed-form | 3 (8) | 1 (8) | 2 (8) |
. | All LV thrombi (n = 37) . | TTE-apparent LVT (n = 12) . | TTE-occult LVT (n = 25) . | P-value . |
---|---|---|---|---|
Fragments | 2.0 (2.0–3.0) | 1.5 (1.0–2.8) | 2.0 (1.0–3.0) | 0.582 |
Volume, cm3 | 1.56 (0.56–3.63) | 2.74 (0.97–5.84) | 1.4 (0.43–2.9) | 0.144 |
Max. diameter, mm | 19.0 (12.6–26.3) | 23.3 (15.4–46.8) | 19.0 (12.1–24.0) | 0.178 |
Shape | 0.800 | |||
Wall-adherent | 7 (19) | 3 (25) | 4 (16) | |
Mass-like | 27 (73) | 8 (67) | 19 (76) | |
Mixed-form | 3 (8) | 1 (8) | 2 (8) |
LV, left ventricular; LVT, left ventricular thrombus; TTE, transthoracic echocardiography.
As revealed by ROC analysis, AWMS16Seg was the strongest predictor for TTE-occult LVT [AUC 0.91 (95% CI: 0.89–0.93), P < 0.001, Graphical abstract] as compared with AWMS17Seg [AUC 0.89 (95% CI: 0.86–0.91), P < 0.001] and LVEF [AUC 0.84 (95% CI: 0.81–0.86), P < 0.001].
The combination of AWMS17Seg and AWMS16Seg [AUC 0.91 (95% CI: 0.88–0.93)] and LVEF and AWMS16Seg [0.91 (95% CI: 0.89–0.94), as well as body mass index and AWMS16Seg [AUC 0.93 (95% CI: 0.90–0.95)], did not lead to a significantly higher AUC as compared with AWMS16Seg alone (P-values for difference: 0.532, 0.540, and 0.052, respectively).
Dichotomization of LVEF at a cut-off value of 43% (Youden index) yielded a sensitivity of 88% and a specificity of 76% for TTE-occult LVT. The 17-segment AWMS dichotomized at a threshold of 14 points (Youden index) had a sensitivity and specificity of 96% and 75%, respectively. The 17-segment AWMS ≥ 11 points provided a sensitivity of 100% with a specificity of 70%. The 16-segment AWMS ≥ 8 points was the optimal cut-off by Youden with a sensitivity of 100% and a specificity of 77%. Accordingly, using AWMS16Seg ≥ 8 (n = 260, 30%), all TTE-occult LVT could be detected. The incidence of TTE-occult LVT in the group with AWMS16Seg ≥ 8 was 10% (25 of 260 patients), resulting in a number needed to scan of 10 to detect one TTE-occult LVT in this high-risk group. These results are further illustrated in the suggested screening algorithm (Figure 1).
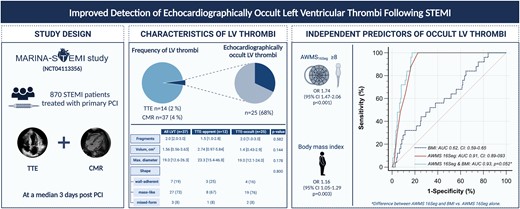
Suggested screening algorithm. Suggested screening algorithm based on the optimal cut-off for 16-segment apical wall motion score (AWMS16seg) <8 (blue, low risk) and ≥8 (red, high risk) yielding a sensitivity of 100% and a specificity of 77% for the detection of transthoracic echocardiography (TTE)-occult left ventricular thrombus (LVT). After dichotomization of the study population, 70% (n = 610) of ST-elevation myocardial infarction (STEMI) patients belong to this low-risk group, defined as AWMS16Seg < 8 (blue). In this group, no occult LVT was identified. Thus, this group has a negative predictive value of 100%. Therefore, further testing by cardiac magnetic resonance imaging is not required. Thirty percent (n = 260) of the study population belong to the high-risk group, defined as AWMS16Seg ≥ 8 (red) with a positive predictive value of 12. All TTE-occult LVT (n = 25) of the study cohort were found in this group. In this group, 10% of patients presented with a TTE-occult LVT, represented by the hatched line in the pie chart. ‘Created with Biorender’.
Discussion
This is the largest study thus far to comprehensively investigate the incidence, characteristics, and clinical determinants of TTE-occult LVT in patients following acute STEMI. All patients underwent a TTE and CMR at a standardized time point after PCI for STEMI (in median 3 days). The main findings were as follows: the number of total LVT detected was significant (n = 37, 4%), with a substantial proportion of TTE-occult thrombi (n = 25, 68%). Transthoracic echocardiography–occult thrombi showed no morphological differences (similar size, number of fragments, and shape). The AWMS16Seg emerged as the most valid predictor of both TTE-apparent and TTE-occult LVT. For specific prediction of TTE-occult LVT, AWMS16Seg and body mass index were the only parameters that showed an independent prognostic value. The AUC of AWMS16Seg was 0.91 and did not significantly improve when combined with body mass index.
Consequently, this data highlights the excellent predictive validity of AWMS16Seg for LVT in the clinical setting of STEMI treated with primary PCI. Given the imaging limitations of TTE for direct visualization of LVT, AWMS16Seg holds great potential as a simple and fast clinical tool to identify patients at high risk for occult LVT who would particularly benefit from further CMR imaging. As body mass index was specifically associated with TTE-occult but not with TTE-apparent LVT, obesity may be considered as a co-factor when selecting patients for additional CMR scans to optimize LVT detection. The added predictive value of body mass index over AVMS16Seg is, however, very small.
Detection of left ventricle thrombus following acute ST-elevation myocardial infarction
There is a considerable difference in the reported incidence of LVT after STEMI.1 Several factors may explain this variation including the timing and frequency of imaging, the method of imaging used for LVT detection, and substantial changes in STEMI treatment over the last years.5
CMR is the current modality of choice for LVT detection and has therefore been used for the present study. We performed CMR examinations during hospitalization at a median of 3 days after STEMI and observed a LVT incidence of 4%. While the incidence is comparable to previous large STEMI trials using CMR,2 the comparatively early CMR examination could potentially have left some LVT undetected.20 On the other hand, performing a relatively late scan can also have adverse consequences due to a delayed initiation of therapy. Therefore, the optimal timing for LVT detection after STEMI is still unknown and warrants further research.6 Although CMR is the preferred imaging technique with the highest diagnostic accuracy for LVT,5 the use of this costly method is limited. Therefore, TTE is still widely used to diagnose LVT in everyday clinical practice. However, previous studies consistently reported substantial limitations in the diagnostic accuracy of TTE. Particularly, the sensitivity was demonstrated to be low, ranging from 29% to 35%.1,9 This sensitivity can be increased by the use of contrast medium, but only up to ∼60% in dedicated research settings.9 Comparable with this earlier data, in the present analysis, in which only a minority of patients were evaluated by contrast-TTE, a sensitivity of TTE of 32% was observed. Based on this data, it can be assumed that the majority of LVT post-STEMI remain undetected in everyday clinical routine. Even large LVT can be missed by TTE, which was highlighted by our finding that the volume in LVT did not differ significantly between echocardiographically occult and visible thrombi. It is also important to emphasize that patients with TTE-occult LVT have a similar rate of embolism as those with TTE-apparent thrombus.11 In light of the high morbidity associated with TTE-occult LVT,11 new clinical approaches are necessary to improve the detection of this feared but treatable complication in STEMI patients.
Predictors of transthoracic echocardiography–occult left ventricle thrombi
As visualization of LVT per se is limited by TTE, different echocardiographic indicators and predictors of LVT formation have been described.21 It has long been known that global LV dysfunction is associated with an increased risk of LVT. Indeed, LVEF has repeatedly been demonstrated to predict LVT formation in STEMI patients,2,5 which has been confirmed by our analysis. Increased stasis due to decreased contractility and more pronounced sub-endocardial injury are considered the main underlying pathomechanisms explaining the relation between LVEF and development of LVT.5 Since LVT after STEMI almost exclusively occur apically, it has been hypothesized that injury and dysfunction of only apical segments might be more relevant than global LV changes.9 A study by Weinsaft et al. specifically addressed this question by investigating the validity of AWMS in comparison to LVEF. In 201 STEMI patients undergoing CMR to define presence of LVT, they demonstrated that although both parameters strongly predicted LVT, the validity of AWMS, concretely AWMS17Seg, was superior to that of LVEF.9 These findings highlight the decisive role of regional wall motion abnormalities of the apex for LVT formation. Since echocardiographic predictors of LVT that are visible by TTE anyway are less interesting from a clinical point of view, we focused specifically on the challenging scenario of TTE-occult LVT. In our significantly larger cohort of 870 STEMI patients, AWMS16Seg emerged as an independent determinant of TTE-occult LVT with the highest predictive value. Accordingly, our data suggest AWMS16Seg as the preferred clinical tool over AWMS17Seg and LVEF for identifying patients at high risk for TTE-occult LVT. This is in line with the latest EACVI/ASE guidelines, which recommend the easier AWMS16Seg over the relatively complex AWMS17Seg that was evaluated by Weinsaft and colleagues.9,18 The rationale for this simpler AWMS16Seg is based on the fact that endocardial excursion and thickening of the tip of the apex (segment 17) are imperceptible via TTE.18 Accordingly, exclusion of segment 17 was proposed to provide a more reliable picture of apical contractility. Furthermore, sub-grading of hypokinesis as required for AWMS17Seg is relatively difficult and increases the risk for observer variance.18 Together, our data support the arguments and recommendation of the EACVI/ASE for the AWMS16Seg also with regard to the detection of TTE-occult LVT following STEMI.
Besides AWMS16Seg, we could reveal an independent association between body mass index and TTE-occult LVT. Because body mass index was specifically related to TTE-occult LVT and not to TTE-apparent thrombi, obesity does not appear to be a pathophysiological factor for thrombus generation but does affect TTE image quality. Hence, our findings highlight that obesity increases the risk of overlooking LVT by TTE in daily clinical routine.
Clinical implications
Evaluating patients with STEMI for LVT is challenging in clinical routine. A central problem is the low sensitivity of TTE for LVT detection even when focusing on this specific question.9 At the same time, routine use of CMR imaging as an initial tool for LVT screening would need immense efforts in the clinical setting and incur significant costs. In this regard, the current data suggests AWMS16Seg as a fast and robust tool to identify the sub-group of STEMI patients at high risk for TTE-occult LVT in whom additional LVT assessment by CMR is desirable. Indeed, an AWMS16Seg-based algorithm using a score value of ≥8 would have enabled all patients with thrombus (100% sensitivity) to be correctly referred for additional imaging with CMR, while preventing unneeded CMR imaging in the majority of patients. While body mass index was independently associated with TTE-occult LVT, suggesting that obesity may be a co-factor in the decision to perform additional CMR scans to improve LVT detection, it is important to acknowledge that the incremental predictive value provided by body mass index was quite small. In clinical settings where CMR is not available, the systematic use of AWMS16Seg could help identify high-risk patients who would benefit from transfer to facilities equipped with CMR, potentially improving the overall standard of patient care. Computed tomography holds promise as a future imaging modality in such scenarios. However, its utility for LVT detection remains to be validated.
Limitations
Although the present CMR study analysed a large STEMI cohort and the results regarding LVT incidence and validity of thrombus predictors are comparable with previous data,1 external validation of our findings is desirable to underscore the generalizability of the present data. Furthermore, only clinically stable STEMI patients (Killip class < 3) were included to ensure reliable CMR imaging. Thus, our data may not be applicable to the small sub-group of unstable STEMI patients.22 Study-specific analysis of echocardiographic markers was performed by experienced observers blinded to clinical data. However, image acquisition was performed as part of clinical routine, which explains the absence of contrast-TTE in the majority of patients. Another limitation of our study arises from specific inclusion/selection criteria, which could generate variability in patient cohort size and incidence rates. Finally, the median delay for CMR was 3 days (IQR: 2–5), suggesting that some LVT may not have been detected. Importantly, the optimal time to achieve the highest detection rate of LVT without delayed initiation of therapy remains unclear.6
Conclusions
In STEMI patients treated with primary PCI, AWMS16Seg as assessed by TTE emerged as an excellent and independent predictor of TTE-occult LVT. Integration of this simple parameter as a stratification tool to determine the need for further CMR imaging may improve detection of TTE-occult LVT in clinical care of STEMI patients.
Supplementary material
Supplementary material is available at European Heart Journal: Acute Cardiovascular Care
Funding
This work was supported by grants from the Austrian Science Fund (FWF): KLI 772-B (B.M.), the Tiroler Wissenschaftsfonds (M.R.), and the Austrian Society of Cardiology (S.J.R.).
Data availability
The data underlying this article will be shared on reasonable request to the corresponding author.
References
Abbreviations
- AWMS
apical wall motion score
- AUC
area under the curve
- CMR
cardiac magnetic resonance
- CI
confidence interval
- IQR
interquartile range
- LGE
late gadolinium enhancement
- LV
left ventricular
- LVEF
LV ejection fraction
- OR
odds ratio
- PCI
primary percutaneous coronary intervention
- ROC
receiver operating curve
- STEMI
ST-elevation myocardial infarction
- TTE
transthoracic echocardiography
Author notes
Martin Reindl and Ivan Lechner contributed equally to the study.
Conflict of interest: None.
Comments