-
PDF
- Split View
-
Views
-
Cite
Cite
David W. Onstad, Bruce E. Hibbard, Thomas L. Clark, David W. Crowder, Kris G. Carter, Analysis of Density-Dependent Survival of Diabrotica (Coleoptera: Chrysomelidae) in Cornfields, Environmental Entomology, Volume 35, Issue 5, 1 October 2006, Pages 1272–1278, https://doi.org/10.1093/ee/35.5.1272
- Share Icon Share
Abstract
We analyzed published field data concerning western corn rootworm (Diabrotica virgifera virgifera LeConte) and northern corn rootworm (Diabrotica barberi Smith and Lawrence) survival from egg to adult to create general relationships between density and survival. A series of equations were generated that best fit the data, and these equations may be used to help understand density-dependent survival in future studies. In general, the datasets showed a decline in the proportion surviving to adulthood as egg density increased. The survival of northern corn rootworm was lower than that of western corn rootworm. Data for both natural and mechanical infestations of soil with eggs show decreasing proportion surviving as density increases. Several nonlinear equations fit the data for both species very well. Density-dependent survival can account for differences in observations of insecticide survival at different initial western corn rootworm densities.
Western corn rootworm, Diabrotica virgifera virgifera LeConte, and northern corn rootworm, Diabrotica barberi Smith and Lawrence, are the corn rootworm species of economic concern in the primary corn (Zea mays L.) growing regions of the United States. Western corn rootworm is generally the more problematic species, but northern corn rootworm predominates in some areas (Levine and Oloumi-Sadeghi 1991). Both species have developed resistance to 2-yr corn rotations in some areas (Levine et al. 2002). Both are being targeted for control by transgenic insecticidal corn.
In agricultural landscapes, rootworm populations likely depend upon corn roots as the primary food source, although the western corn rootworm can survive on almost all grass species for 10 d and develop to at least the third instar on most grass species (Clark and Hibbard 2004, Oyediran et al. 2004, Wilson and Hibbard 2004). Because the larvae inhabit the soil, studies of their behavior and survival are difficult to perform. Studies of survival depend on knowing the density of either eggs or larvae and number of emerging adults.
Density-dependent survival is the change in the proportion surviving as the density changes. Toepfer and Kuhlmann (2005) recently published a review of the literature on western corn rootworm survival. Onstad et al. (2001) and Mitchell and Riedell (2001) showed density dependence in the proportion of western corn rootworm eggs surviving to adulthood in analyses of published data. Storer (2003) assumed that "most density-dependence occurs after the larvae have become established but before they have reached adulthood." Data from Hibbard et al. (2004) supported the assumption of Storer (2003) that density-dependent mortality is negligible during establishment because the percent of larvae recovered did not vary consistently with infestation density. Density-dependent survival is observed when eggs and/or larvae have lower survival at higher densities when, for example, predation, parasitism, or competition for food increases as rootworm density increases. Because this relationship of immature survival to density can have significant influence on model results (Onstad et al. 2003, Crowder et al. 2005a,b), on estimates of toxin mortality (Onstad et al. 2001), and therefore, on integrated pest management (IPM) and insect resistance management, we performed a more thorough study. The purpose of this paper is to define the general relationship between survival and egg or larval density more clearly and accurately for both rootworm species.
Materials and Methods
The field data concerning rootworm survival from egg to adult were obtained from published literature for western and northern corn rootworm [Branson et al. (1980), n = 5; Branson and Sutter (1985), n = 4; Fisher (1985), n = 3; Gray and Tollefson (1987,1988a), n = 12; Spike and Tollefson (1988), n = 4; Elliott et al. (1989), n = 4]. All studies measured survival of western corn rootworm, but only Fisher (1985) and Gray and Tollefson (1987,1988a) measured northern corn rootworm survival. Published mean values were used, not the raw data. Gray and Tollefson (1987,1988a,b) measured natural infestations of the soil with eggs, but all the other studies are based on mechanical infestations of the soil with eggs. We studied two measures of rootworm egg densities because models typically calculate values as number per hectare, wheras field studies often evaluate number per fraction of a corn row. We converted the numbers per hectare observed by Gray and Tollefson (1987,1988a,b) to the number of eggs per 0.305 m of row by accounting for 10,000 m2/ha and 0.232 m2/area per 0.305 m of row when row spacing is 0.76 m. The other egg data were transformed from numbers per unit of row to number per hectare by knowing or assuming the row spacing and the unit length. If the row spacing was not indicated in the publication, we assumed that it was 0.76 m.
We used SAS regression software (PROC NLIN) to fit equations relating proportion surviving to adulthood (proportion emerging) to the density of eggs (SAS Institute 2002). For northern corn rootworm (n = 15) and western corn rootworm (n = 32), we fit functions, respectively, to the observations with density expressed as number of eggs per 0.3 m of row, N and W, or as millions of eggs per hectare, EGGN and EGGW. For the purposes of consistency, regression equations were based on functions previously published (Onstad et al. 2001,2003, Crowder and Onstad 2005). One kind of equation has the form Proportion Surviving = Aexp(B × Density), whereas the other two have the form Proportion Surviving = 1/(A + B × Density ×C), where C is an exponent of density. In one of the latter cases, we set A = 1.0 to match the assumption that when density is very low (e.g., only one larva per plant), survival is close to 100% (same approach as Onstad et al. 2001). In the other case, we determined the lowest positive intercept (value of A) that provided a fit similar to the case with A = 1.0.
We visually compared the resulting regression equations for western corn rootworm to independent data collected by Sutter et al. (1991) in insecticide studies. To evaluate three previously published equations for western corn rootworm (Onstad et al. 2001,2003, Crowder and Onstad 2005), we regressed observed versus predicted values using SigmaPlot regression software (Systat Software 2004).
To determine the effect of density-dependent survival on the carrying capacity of western corn rootworm in cornfields (i.e., the number of beetles produced per hectare), we simulated the model of Crowder and Onstad (2005) for 30 yr to find the maximum density of adults predicted by the model. The simulated landscape consisted of 100% continuous, nontransgenic corn without soil insecticide use. Thus, the only mortality factors were overwintering mortality of 50% and one of the three equations fit to the data. Fecundity was 220 viable eggs per adult, which is standard for the model. To compare model results to reality, we surveyed the literature for data demonstrating high densities of adults (or eggs) produced on a per hectare basis over an entire season.
Results
The six equations fit to the 15 observations of northern corn rootworm are presented in Table 1. With r2 > 0.88, all six equations fit the data well (Table 1). Equations 2, 3, 5, and 6 with intercepts from 0.1133 to 1.0 all had approximately the same r2 = 0.90, although the function with an intercept of 1.0 actually had the highest r2 when expanded to more than two digits. For equations 3 and 6 (Table 1), we set the y-intercept equal to 1.0 to match the assumption that a single rootworm larva would experience almost no density-independent mortality and would survive to adulthood.
Regression equations of the proportion of inunature stage of northern corn rootworni population surviving to adult based on 15 observations

In all equations, the regression algorithm selected only two coefficients; for equations 2, 3, 5, and 6, we chose the intercept and made it a constant in the regression.
N is the no. of eggs per 0.3 m of row and EGGN is millions of eggs per hectare. The two coefficients of each equation are shown starting from left (Coefficient 1) to right (Coefficient 2).
df = 2,13.
Regression equations of the proportion of inunature stage of northern corn rootworni population surviving to adult based on 15 observations

In all equations, the regression algorithm selected only two coefficients; for equations 2, 3, 5, and 6, we chose the intercept and made it a constant in the regression.
N is the no. of eggs per 0.3 m of row and EGGN is millions of eggs per hectare. The two coefficients of each equation are shown starting from left (Coefficient 1) to right (Coefficient 2).
df = 2,13.
Equations 1-3 and the observations are shown in Fig. 1. The data from Fisher (1985) are different from the data collected over the 3 yr of the Gray and Tollefson (1987,1988a,b) study. Whether the differences are caused by environment, method (mechanical [Fisher] versus natural infestations [Gray and Tollefson]), or the large variability in egg sampling is uncertain.
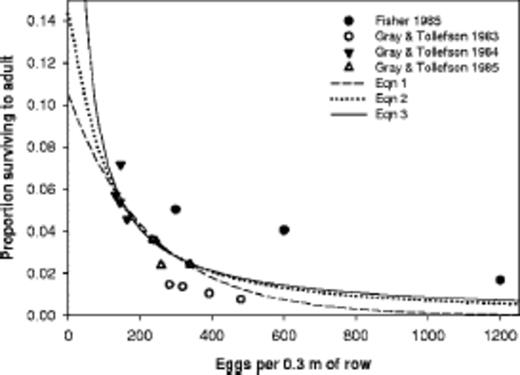
Observations of Fisher (1985) and Gray and Tollefson (1987, 1988a,b) and three regression equations fit to the data for northern corn rootworm survival in cornfields.
The six equations fit to the 32 observations of western corn rootworm are presented in Table 2. With r2 > 0.88, all six equations fit well (Table 2). Equations 8, 9, 11, and 12 with intercepts from 0.2994 to 1.0 all had approximately the same r2 = 0.93, although the function with an intercept of 1.0 actually had the highest r2 when expanded to more than two digits. Equations 7-9 and the observations are displayed in Fig. 2. Note that Gray and Tollefson (1987,1988a,b) made most of the observations at low densities. Their results are much different from those of Branson et al. (1980).
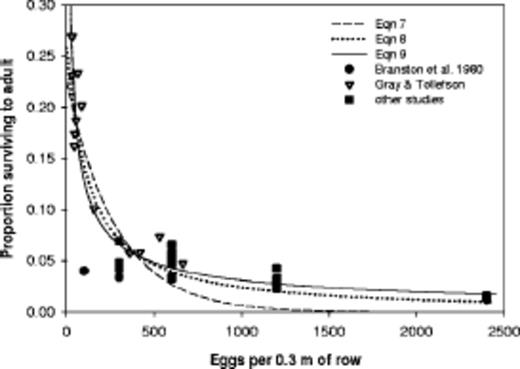
Observations and three regression equations fit to the data for western corn rootworm survival in cornfields. All observations of mechanical infestations except for the Gray and Tollefson (1987, 1988a,b) data for natural infestations.
Regression equations of the proportion of inunature stage of western corn rootworm population surviving to adult based on 32 observations

In all equations, the regression algorithm selected only two coefficients; for equations 8, 9, 11, and 12, we chose the intercept and made it a constant in the regression.
W is the no. of eggs per 0.3m of row and EGGw is millions of eggs per hectare. The two coefficients of each equation are shown starting from left (Coefficient 1) to right (Coefficient 2).
df = 2,30.
Regression equations of the proportion of inunature stage of western corn rootworm population surviving to adult based on 32 observations

In all equations, the regression algorithm selected only two coefficients; for equations 8, 9, 11, and 12, we chose the intercept and made it a constant in the regression.
W is the no. of eggs per 0.3m of row and EGGw is millions of eggs per hectare. The two coefficients of each equation are shown starting from left (Coefficient 1) to right (Coefficient 2).
df = 2,30.
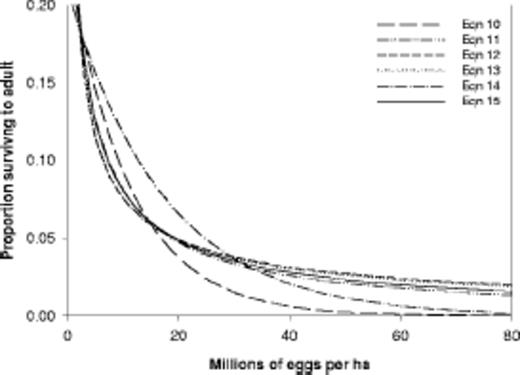
Sutter et al. (1991) measured the survival from egg to adulthood at four densities of mechanically-infested eggs of the western corn rootworm in plots treated with soil insecticides at planting time. These data are the averages for all seven insecticides. Figure 4 shows that, even though insecticide mortality may have occurred in each of the 3 yr, the observed proportion surviving to adulthood decreased as egg density increased. Sutter et al. (1991) state that "Our results revealed that inherent toxicity of insecticides had little influence on survival of immatures." Their statistical analysis indicated that in only 1 of the 3 yr (1982) was mean survival in the untreated check plots significantly higher than in treated plots (Sutter et al. 1991). The survival values for 1982 are much lower than the other values in Fig. 4, showing the effect of the chemical insecticides.
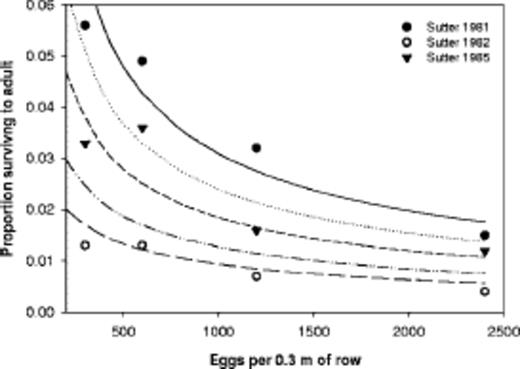
Observations of Sutter et al. (1991) for western rootworm survival in cornfields. corn rootworm in insecticide-treated plots and curves based on a range of hypothetical insecticide survival rates (dis played next to curves) considered along with density-dependent survival function (equation 9).
To determine the influence of the two kinds of survival, we accounted for various values of insecticide survival, Si, as well as subsequent density-dependent survival by reducing density based on insecticide survival in equation 9: Proportion Surviving = Si/[1 + 0.3282 × (Si × W)0.6592]. The resulting curves displayed in Fig. 4 show two points. First, a density-independent insecticide survival rate of 0.05 (5% of neonates surviving) along with equation 9 could account for the observations in 1982. Second, survival observed over a range of densities should flatten out as density-independent survival decreases even though density-dependent survival remains in effect. Furthermore, it is possible, according to the curves in Fig. 4, that insecticides were killing 50-75% of the neonates in 1985, but that sampling was not sufficient to detect this statistically. Thus, knowledge of density-dependent survival and of its influence over our observations may help scientists perform better field experiments.
The simulations of the generational time-step model of Crowder and Onstad (2005) indicated that the carrying capacity of the cornfields would be 1, 1.1, and 2.3 million adults produced/ha, respectively, for equations 10-12. Onstad et al. (2001) calculated a carrying capacity of 0.7 million adults produced/ha with a daily time-step model, which had other factors that could have reduced oviposition and egg viability.
Table 3 contains results of our literature survey for very high densities of adult rootworms produced over an entire season. Generally, the densities of northern corn rootworm are lower than those of western corn rootworm. The densities of western corn rootworm exceeded four million per hectare in one report in Table 3. These values may be above a carrying capacity and not sustainable over several generations. The results for the simulations with equations 10-12 match the range of the rest of the data presented in Table 3 (0.5-3.7 million adults/ha).
Highest adult densities of western and northern corn rootworm found in a survey of literature
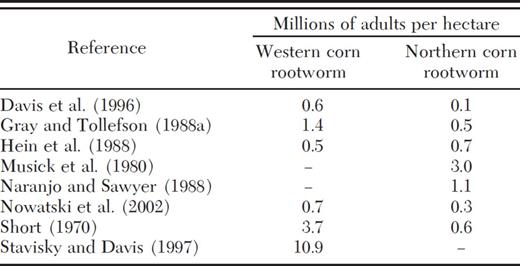
Highest adult densities of western and northern corn rootworm found in a survey of literature
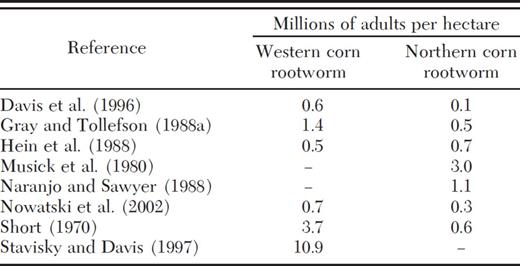
With regard to egg densities, several entomologists have observed >10 million eggs/ha in natural infestations. In a 3-yr study of fall densities, Hein and Tollefson (1985) observed >10 million eggs/ha in 2 yr for northern corn rootworm (maximum was 35 million) and in all 3 yr for western corn rootworm (maximum was 66 million). In a 3-yr study of spring densities, Gray and Tollefson (1987) observed >10 million eggs/ha in 2 yr for northern corn rootworm (maximum was 21 million) but in only 1 yr for the western corn rootworm (maximum was 29 million). Pierce and Gray (2006) observed 68-100 million eggs/ha in the fall over 2 yr at two locations. Thus, equations 4-6 and 10-15 must be able to accurately calculate spring density-dependent survival over a range from 1 to 35 million eggs/ha for northern corn rootworm and 1-100 million/ha for western corn rootworm.
Discussion
Almost all the separate datasets and all the regression equations fit to the data indicate that immature survival of two pest Diabrotica species in cornfields is density dependent. In other words, the proportion of the egg and larval population surviving to adulthood declines as density of immature stages increases. This is clear for both northern and western corn rootworm populations in cornfields.
A comparison of Figs. 1 and 2 indicates that the survival of northern corn rootworm is lower than survival of western corn rootworm. For instance, at an egg density of 500 per 0.3 m of row, northern corn rootworm has <2% survival, whereas the survival for western corn rootworm is ~5%. Similarly, at egg densities around 100 per 0.3 m of row, northern corn rootworms have 6% survival, whereas it is >15% for most of the natural infestation data in Fig. 2 for western corn rootworm. This supports the conclusion of Woodson (1994) that northern corn rootworm has lower larval survival than western corn rootworm.
Based on simple logic, Diabrotica survival in cornfields should be influenced by plant density at least over the lower end of the range of densities. In reality, however, commercial cornfields in most of northcentral United States almost always have >60,000 plants/ha (Rankin 2004). Spike and Tollefson (1988) measured western corn rootworm survival at corn densities of 39,000, 63,000, and 87,000 plants/ha. They found no significant difference in survival from egg to adulthood at the two higher plant densities. Proportion surviving to adulthood was significantly lower for 39,000 plants/ha in 1 yr.
The equations presented in this paper can be used to estimate how much density-independent mortality occurs in studies of insecticides and insecticidal transgenic corn. The data required to make the appropriate calculations include the proportion of viable eggs, the number of eggs infested per unit of space, and the number of adults emerging over the same unit of space. These data must be collected for treated plots and untreated control plots. The selected equation can be rearranged to calculate the number of larvae alive before the population incurred density-dependent survival. This number of larvae is divided by the number of viable eggs to calculate the proportion surviving any intoxication assumed to occur in the neonate stage. A similar procedure was described by Onstad et al. (2003).
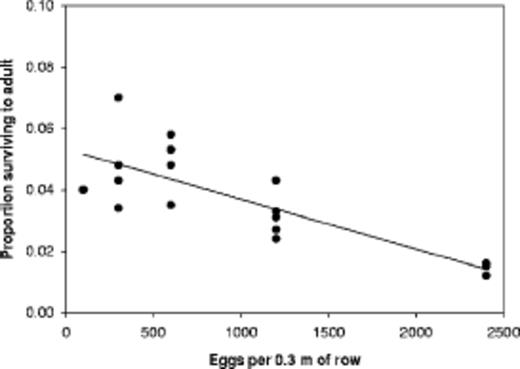
Subset of observations of mechanical infestations of western corn rootworm eggs and survival in cornfields. The line based on the regression, Y = 0.0533 - W × 1.63 × 10-5, has an r2 = 0.63(n = 18), and both coefficients are significantly different from 0(P < 0.0001).
In population ecology, survival is often modeled as a series or set of independent factors that when multiplied produce the fraction of the life stage or population that survives. The key points are that ecologists work with survival (not mortality) and they attempt to find factors that are independent of each other (one not incorporated into the other) so that multiplication is logical and valid. For example, we often think of insecticide toxicity affecting survival independently of intraspecific competition or of predation. Thus, for models, we must decide whether the functions described in this paper are independent of some factors or incorporate them. Density-independent survival factors can include weather, soil quality, and toxic roots or insecticides. Equations 3, 6, 9, 12, and 13 all have intercepts of 1.0, indicating either that no density-independent mortality occurred during those field studies or that we need more data to confirm the extrapolation at low egg densities. The other equations have, perhaps, more reasonable intercepts that indicate that density-independent survival ranged from 20 to 40% during those field studies. For all equations, it is safe to assume that the survival rates are independent of survival to toxins because none of the studies used insecticides or insecticidal transgenic plants.
Which equation is best for modeling purposes? Equations 11, 12, 13, and 15 are indistinguishable at moderate to high egg densities (Fig. 3). Equation 12 and, because of its similar coefficients, equation 13 permit high carrying capacities that may better describe the observations in Table 3. Equation 13 has been used more often in modeling manuscripts either as the standard function for immature survival or in sensitivity analyses, including the work of Storer (2003), but the intercept of 1.0 may be unrealistic over the long run. It may be better to continue evaluating the influence of density-dependent survival in sensitivity analyses than to set in stone one equation as the only true description of real survival.
There are a number of differences between field trials with natural and mechanical infestations. First, it is easy to control exactly how many eggs are present in any given location with mechanical infestations. Natural infestations have a much more clumped distribution. Unfortunately, eggs are typically infested at one soil depth in mechanical infestations. Because soil temperature varies with soil depth, mechanically infested eggs have a more synchronous hatch than naturally infested fields, for which oviposition and tilling distributes eggs at various depths. With natural infestations, if egg density must be known, eggs must be washed from the soil to create an estimate of egg densities. Egg washing is an extremely tedious process (Ruesink 1986) and has a wide margin of error at any egg density. Because naturally infested eggs have a clumped distribution (Branson 1986), estimates for egg densities have an extremely wide margin of error when egg densities are low. The clumped distribution is somewhat alleviated because larvae can move from crowded to less damaged roots nearby (Hibbard et al. 2003,2004,2005).
As Fig. 2 indicates for the western corn rootworm, few observations of survival have been made for egg densities <100 per 0.3 m of row. Only some of the natural infestations studied by Gray and Tollefson (1987,1988a,b) had very low egg densities. Plant damage can barely be detected at these low infestation rates (Branson et al. 1980, Hibbard et al. 2004); thus, food scarcity and starvation may not be the cause of any real density-dependent mortality at infestation rates <100 eggs per 0.3 m of row. However, predation and parasitism may be important factors at these low densities. Obviously, if a toxin can kill 99% of a population, as few as 6-12 larvae would exist in 0.3 m of row after a mechanical infestation of 600-1,200 eggs. Thus, very small infestations are very relevant to IPM and insect resistance management. To confirm our conclusions based partially on the observations by Gray and Tollefson (1987,1988a,b), we are currently performing an experiment in Missouri cornfields to measure larval survival at densities as low as 25 eggs per 0.3 m of row using mechanical infestations of eggs of the western corn rootworm.
We thank P. Mitchell and N. Storer for advice concerning the manuscript. This work was supported by a grant to Onstad from the USDA Biotechnology Risk Assessment program and Onstad's cooperative agreement with the USDA-ARS facilitating modeling work of interest to the USEPA.
References
Author notes
The ideas expressed in this paper may not represent those of the USDA or USEPA.