-
PDF
- Split View
-
Views
-
Cite
Cite
Marie Perkins, Lisa Ferguson, Richard B. Lanctot, Iain J. Stenhouse, Steve Kendall, Stephen Brown, H. River Gates, Jeffery O. Hall, Kevin Regan, David C. Evers, Mercury exposure and risk in breeding and staging Alaskan shorebirds, The Condor: Ornithological Applications, Volume 118, Issue 3, 1 August 2016, Pages 571–582, https://doi.org/10.1650/CONDOR-16-36.1
- Share Icon Share
Abstract
Mercury contamination has become a major concern in the Arctic, where elevated mercury deposition has led to large increases in mercury exposure for some Arctic wildlife over the past century. Chronic mercury exposure in birds is known to reduce reproductive success, which may ultimately result in population declines. Many species of Arctic-breeding shorebirds are declining, and exposure to environmental contaminants, such as mercury, may be an important factor. We quantified mercury exposure in 10 shorebird species breeding and staging in Alaska. We analyzed 229 blood and 73 feather samples collected in 2008–2009 for total mercury concentrations. Mercury in blood represents local exposure, whereas mercury in feathers reflects exposure during feather development. Concentrations of mercury ranged from 0.03 to 2.20 μg g−1 in shorebird blood and from 0.16 to 3.66 μg g−1 in shorebird feathers. Most shorebirds sampled during staging had relatively low blood mercury, but some breeding species had sufficiently high concentrations for potential adverse effects. Overall, blood mercury concentrations of breeding shorebirds differed by moisture content of their predominant foraging habitat, with the highest concentrations found in species using wet to aquatic habitats. We also found variation in mercury concentrations by age class and sex for some species, with females showing lower concentrations than males, but we found no relationship between the amount of mercury in feathers and in blood. The degree of mercury exposure seen in Arctic-breeding shorebirds may be of particular concern when combined with other ecological stressors, such as habitat loss, predation, disturbance, and climate change.
Resumen
La contaminación con mercurio se ha vuelto una preocupación importante en el Ártico. Los elevados depósitos de mercurio han llevado a grandes incrementos en la exposición al mercurio en algunos animales silvestres del Ártico durante el siglo pasado. Se sabe que la exposición crónica al mercurio en aves reduce el éxito reproductivo, lo que podría resultar en declives poblacionales. Las poblaciones de muchas especies de aves playeras que se reproducen en el Ártico están disminuyendo; la exposición a contaminantes ambientales, como el mercurio, podría ser un factor importante. Cuantificamos la exposición al mercurio en 10 especies de aves playeras que se reproducen y hacen paradas migratorias en Alaska. Analizamos la concentración total de mercurio en 229 muestras de sangre y 73 plumas recolectadas entre 2008 y 2009. El mercurio en la sangre representa la exposición local, mientras que el mercurio en las plumas refleja la exposición durante el desarrollo de las mismas. Las concentraciones de mercurio variaron entre 0.03 y 2.20 μg g−1 en la sangre de las aves playeras, y entre 0.16 y 3.66 μg g−1 en las plumas. La mayoría de las aves playeras muestreadas durante la parada migratoria tenían relativamente poco mercurio en la sangre, pero algunas especies en reproducción tenían concentraciones lo suficientemente altas como para desencadenar potenciales efectos adversos. En general, las concentraciones de mercurio en la sangre de las aves playeras en reproducción fueron diferentes dependiendo de la humedad de los hábitats en los que se alimentan predominantemente, con mayores concentraciones encontradas en especies que usan hábitats húmedos o acuáticos. También encontramos variación en las concentraciones de mercurio entre clases de edad y sexo en algunas especies (las hembras tienen menores concentraciones que los machos), pero no encontramos relación entre la cantidad de mercurio en las plumas y en la sangre. El grado de la exposición al mercurio observado en aves playeras que se reproducen en el Ártico podría ser particularmente preocupante cuando se combina con otras causas de estrés ecológico, como la pérdida de hábitat, la depredación, el disturbio y el cambio climático.
Palabras clave: mercurio, aves costeras, Ártico, Alaska, sangre, plumas
Introduction
Mercury contamination has increased globally from anthropogenic inputs and has become a major concern in the Arctic (AMAP 2011). Long-range transport of anthropogenically produced atmospheric mercury combined with the unique features of the Arctic environment has resulted in greater mercury deposition and availability than in more temperate environments (Poissant et al. 2008, AMAP 2011). For example, the Yukon River Basin, which drains most of central interior Alaska and the Yukon Territory, had higher annual mercury concentrations compared to 8 other North American river basins (Schuster et al. 2011). Continued increases of mercury contamination in the Arctic are likely. Sunderland et al. (2009) demonstrated that at current atmospheric deposition rates, mercury concentrations in the eastern North Pacific Ocean will double by 2050. Mercury emissions from Asia, the principle source of long-range mercury pollution deposited across the Arctic, are on the rise and predicted to increase further (Durnford et al. 2010, AMAP 2011, UNEP 2013). The Arctic may also be at risk of increasing mercury concentrations due to climate change. Permafrost is an important repository for mercury deposited in the region over past centuries (Schuster et al. 2011, Stern et al. 2011), and as Arctic temperatures increase and permafrost melts, sequestered mercury representing thousands of years of deposition has the potential to be rapidly released into the environment (Schuster et al. 2011).
Over the past century, elevated mercury deposition has led to large increases in mercury exposure for some Arctic wildlife (Dietz et al. 2009, AMAP 2011). Once deposited on the landscape, mercury can be converted into methylmercury by bacterial methylation under anaerobic conditions, particularly in wetland and aquatic environments (Driscoll et al. 2007). Methylmercury is the most bioavailable and toxic form of mercury (Wiener et al. 2003); consequently, many studies investigating mercury exposure in birds have focused on fish-eating species that obtain their food from wetland or aquatic environments (Wolfe et al. 2007, Scheuhammer et al. 2007, 2011). Bioaccumulation of mercury also occurs in terrestrial habitats, however, and adverse effects of mercury exposure on nonpiscivorous species are increasingly apparent (Evers et al. 2005, Edmonds et al. 2010, 2012, Hallinger and Cristol 2011, Jackson et al. 2011a, 2011b, 2015, Lane et al. 2011).
Mercury can have detrimental effects on wildlife (Wolfe et al. 1998, 2007, Scheuhammer et al. 2007, 2011). Even at sublethal concentrations, chronic exposure in birds can impair physiology, behavior, and reproductive success, which can ultimately have population level effects; such negative effects have been documented in piscivores, such as the Common Loon (Gavia immer; Burgess and Meyer 2008, Evers et al. 2008, 2011). Although less is known about the effects of mercury in avian invertivores, dosing studies indicate that this foraging guild is at greater risk of exposure than avian piscivores (Heinz et al. 2009). Reduced reproductive success and suppressed immune function has been related to mercury exposure in the Tree Swallow (Tachycineta bicolor), an invertivore songbird (Brasso and Cristol 2008, Hawley et al. 2009, Hallinger and Cristol 2011). Additionally, one of the more insightful field-based studies on an avian invertivore was conducted on the Carolina Wren (Thryothorus ludovicianus) at 2 contaminated river floodplains in Virginia, where adult reproductive impairment was quantified with known mercury body burdens (Jackson et al. 2011a).
Shorebirds may be particularly vulnerable to mercury exposure because they forage primarily on invertebrates in coastal and estuarine wetlands where mercury is likely to be more bioavailable (Driscoll et al. 2007, Colwell 2010). In addition, they are long-distance migrants that visit many countries with poor pollution control practices, increasing their risk of contaminant exposure (Kunisue et al. 2002). Areas of Central and South America are of particular concern because mercury contamination is significantly increasing due to artisanal small-scale gold mining (UNEP 2013). Braune and Noble (2009) found liver mercury concentrations to be generally lower in 11 shorebird species sampled at noncontaminated sub-Arctic sites across Canada relative to those sampled in contaminated temperate wetlands (Eagles-Smith et al. 2008). By contrast, however, Hargreaves et al. (2010, 2011) found blood mercury concentrations in some shorebird species breeding in the eastern Canadian Arctic to be as high as those found in shorebirds sampled in mercury contaminated wetlands in San Francisco Bay. Among the few studies to assess the effects of mercury exposure on shorebirds, Hargreaves et al. (2010, 2011) showed that high mercury exposure in shorebirds breeding in Nunavut, Canada, may have reduced reproductive success. These findings suggest that mercury exposure may play an important role in recent shorebird declines (Morrison et al. 2006, Bart et al. 2007).
Mercury concentrations in bird blood provide a measure of recent dietary exposure (Wolfe et al. 1998, Evers et al. 2005) and therefore represent local exposures. Feather mercury concentrations reflect exposure at the time of feather development and remobilized mercury from body burdens (Wolfe et al. 1998, Evers et al. 2005). The majority of adult shorebirds molt feathers on the wintering grounds (Holmes 1966, 1971, Pyle 2008); therefore, feather mercury concentrations reflect both exposure to mercury on the wintering areas and mercury bioaccumulated over time, especially for individuals highly exposed to mercury where not all mercury can be eliminated from the body via natural processes (depurated).
In this study, we investigated mercury exposure in shorebirds breeding and staging in Alaska. We determined baseline blood and feather mercury concentrations of adult (after-hatch year, AHY) shorebirds sampled during the breeding season and AHY and hatch year (HY) shorebirds sampled at staging sites during the post-breeding season. Specifically, we examined how blood mercury concentrations varied by species or subspecies, age, and sex.
We predicted shorebirds breeding farther north would have greater mercury concentrations because mercury deposition in Arctic habitats is predicted to be higher than in sub-Arctic regions (AMAP 2011). We also expected blood mercury concentrations to vary among species, given their different ecological traits such as prey selection and habitat use. Because upland areas tend to have less bioavailable mercury than wetland areas (Driscoll et al. 2007), we predicted species that primarily forage in upland areas to have lower mercury exposure. We predicted HY shorebirds to have lower blood mercury concentrations than AHY because HY birds are able to rapidly depurate mercury during juvenile feather growth (Fournier et al. 2002). We expected breeding AHY females to have lower blood mercury concentrations than breeding AHY males because female birds are able to depurate mercury into the eggs they produce (Lewis and Furness 1993). Finally, we predicted there would not be a relationship between blood and feather mercury concentrations in AHY shorebirds because most of the mercury in feathers is reflective of the conditions where the feathers were grown, which is typically away from the breeding grounds.
Methods
Study Species and Sites
Nine species of shorebirds were sampled for blood and/or feathers at their nest sites during the breeding season at Big Slough and Manokinak on the Yukon Delta National Wildlife Refuge (NWR) in western Alaska and near the village of Barrow in northern Alaska (hereafter Barrow study site) during 2008 and 2009 (Figure 1). Both sexes were sampled for most species, but because of nest attendance behavior, only male phalarope and female Pectoral Sandpiper (Calidris melanotos) could be sampled. Nests were found using systematic area searches and behavioral cues of adults and rope-dragging in suitable tundra areas (Naves et al. 2008). Adults were captured on their nests using bownets; all individuals were at least 1 year old (i.e. AHY birds hatched the prior summer or earlier). Sex of each individual was determined using plumage and morphometric measurements (see Birds of North America species accounts, Poole 2005, Gates et al. 2013) or molecular analysis (Gates et al. 2013).
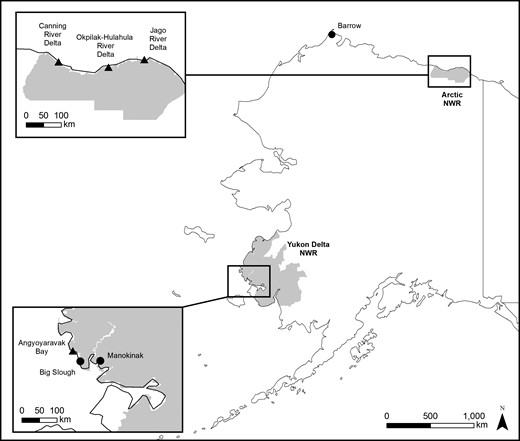
Locations of shorebird sampling to determine mercury exposure during the 2008 and 2009 breeding seasons (circles) and 2008 post-breeding staging (triangles) in Alaska, USA.
In 2008, we also sampled 5 species of shorebirds for blood and/or feathers while they were staging at coastal areas prior to their southbound migration; sample sites included Angyoyaravak Bay in the Yukon Delta NWR and the Canning, Jago, and Okpilak-Hulahula river deltas in the Arctic NWR (Figure 1). Shorebirds were captured with mist nets at foraging and roosting habitats. We determined age by plumage characteristics (Poole 2005), although sex could not be determined during this period.
Sample Collection
We collected blood and feather samples using standard protocols (Evers 2008). Approximately 50–100 μL of blood (no more than 1% of the bird's body weight) was collected in heparinized capillary tubes via puncture of the brachial vein using a small gauge needle. Blood samples were placed on ice packs in a cooler during field collection and stored frozen until shipping. At sites with electric freezers, capillary tubes were sealed and placed in a labeled 10 cc plastic vacutainer to prevent breakage. At remote field sites, blood from the capillary tubes was transferred to a microtainer tube after returning to base camp and stored in a dry-nitrogen–filled cryoshipper that maintained samples at −40°C. In 2008, we also collected the 2nd secondary flight feather at Barrow and the 9th or 10th secondary at the Arctic NWR study sites for mercury analysis. All feather samples were placed in a clean plastic bag or paper envelope and stored at room temperature.
Sample Analysis
We analyzed whole blood and feather samples collected in 2008 for total mercury concentrations at the Utah State Veterinary Diagnostic Laboratory (Logan, UT, USA) using validated protocols with an ELAN 6000 inductively coupled plasma mass spectrometer (ICP-MS, Perkin Elmer, Shelton, CT, USA). To quantify mercury content, whole blood and feather analyses were performed using nitric acid digested samples. Standard curves and quality control (QC) samples were analyzed every 5 samples. National Institute of Standards and Technology standards were also analyzed to verify accuracy of the analytical results. QC analyses were considered acceptable if within ±10% of the known mercury concentration but were typically less than ±5%.
We analyzed whole blood samples collected in 2009 for total mercury concentrations at the Biodiversity Research Institute (Portland, MN, USA). Samples were placed in nickel sample boats, weighed, and analyzed with a Milestone DMA-80 direct mercury analyzer (Milestone Inc., Shelton, CN, USA) using the U.S. Environmental Protection Agency's certified method (USEPA 2007). Before and after every set of 30 samples, 1 sample each of 2 standard reference materials (Dorm-3 and Dolt-4), 2 method blanks, and 1 sample blank were included. A duplicate shorebird blood sample was analyzed after every set of 20 samples. QC analyses were also considered acceptable if within ±10% of the known mercury concentration. We report total mercury concentrations in micrograms per gram (μg g−1) wet weight (ww) for blood and μg g−1 fresh weight (fw) for feathers.
Statistical Analysis
We report the mean, standard deviation, and range of mercury concentrations for blood and feathers from shorebird species sampled during each year at each location; because of small sample sizes, study sites within the Arctic NWR were combined. Results were analyzed by age and sex where possible. We log-transformed values of mercury concentrations when not normally distributed prior to analysis. Data were analyzed using paired and unpaired t-tests, and analysis of variance (ANOVA) tests followed by Tukey's HSD post hoc tests, as appropriate. For the ANOVA, we investigated how mercury concentrations were related to several variables and included interaction terms when relevant (discussed later). We present back-transformed least squared means with lower and upper 95% confidence limits (LCL and UCL, respectively) of blood and feather mercury concentrations. Backward elimination was used to remove interaction terms with P > 0.10. All statistical analyses were conducted in JMP 9 (SAS Institute 2010). Because of sample size limitations, comparisons between species, years, sex, and age were restricted to particular situations described below.
Sampling location
We compared concentrations of blood mercury in 2 subspecies of Dunlin (Calidris alpina): C. a. arcticola sampled at Barrow, and C. a. pacifica sampled at the Yukon Delta NWR. Sex and the interaction between sex and subspecies were included in the model. We combined samples from both years in Barrow for the analysis.
Habitat use
We also investigated differences in blood mercury concentrations among species for (1) HY individuals captured while staging at the Arctic NWR and (2) AHY individuals breeding at Barrow. We did not consider the effect of year or sex in this analysis because most (4 of 6) species were only sampled in 1 year, and the sex that was sampled varied with species. We further investigated the differences in blood mercury concentrations among breeding shorebirds grouped by the moisture content of their predominant foraging habitat. Foraging habitat groups consisted of (1) upland (American Golden-Plover [Pluvialis dominica]); (2) intermediate or mixed habitats (Dunlin, Semipalmated Sandpiper [Calidris pusilla], and Pectoral Sandpiper); and (3) wet to aquatic (Red Phalarope [Phalaropus fulicarius] and Long-billed Dowitcher [Limnodromus scolopaceus]). Field observations suggest these are the primary foraging areas for these bird species on the breeding and staging grounds (Poole 2005, R. B. Lanctot personal observation).
Transfer to feathers and eggs
Using separate t-tests, we compared blood mercury concentrations between AHY and HY age classes for Dunlin (C. a. pacifica) and Rock Sandpiper (Calidris ptilocnemis) captured during staging at the Yukon Delta NWR. We tested for differences in blood mercury concentrations between sexes for mated individuals of Dunlin using a paired t-test; we combined data from both sites and years for this analysis. We were unable to sample mated pairs of other species, but we also compared blood mercury concentrations between sexes for unmated male and female Semipalmated Sandpiper breeding at Barrow.
Variation among years
To examine annual differences, we compared blood mercury concentrations of Dunlin and Red Phalarope sampled from Barrow in 2008 and 2009. Sex and the interaction between sex and year were included in the model for Dunlin, whereas only year was included in the Red Phalarope analysis because only males were sampled.
Blood and feather mercury relationship
We also compared blood and feather mercury concentrations within the same species and age class using linear correlation for AHY Red Phalarope and for HY and AHY Semipalmated Sandpiper.
Results
We analyzed blood and/or feather samples for mercury concentrations from 84 staging shorebirds sampled at the Arctic NWR and Yukon Delta NWR from July 25 to September 9, 2008, and from 166 breeding shorebirds sampled at Barrow and the Yukon Delta NWR from June 11 to July 11, 2008, and again from June 9 to July 22, 2009 (Table 1 and 2).
Number of samples (n), mean (x), standard deviation (SD), and the range of blood mercury concentrations (μg g−1, fw) by species, age (AHY = adult, HY = hatch year), and sampling location (status: B = breeding, S = post-breeding staging).
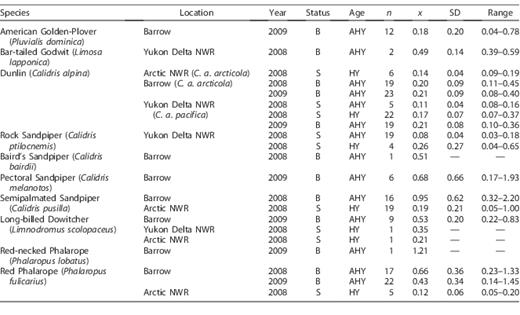
Number of samples (n), mean (x), standard deviation (SD), and the range of blood mercury concentrations (μg g−1, fw) by species, age (AHY = adult, HY = hatch year), and sampling location (status: B = breeding, S = post-breeding staging).
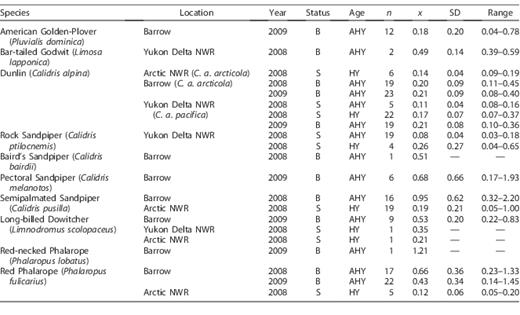
Number of samples (n), mean (x), standard deviation (SD), and the range of feather mercury concentrations (μg g−1, ww) by species, age (AHY = adult, HY = hatch year), and sampling location (status: B = breeding, S = post-breeding staging).
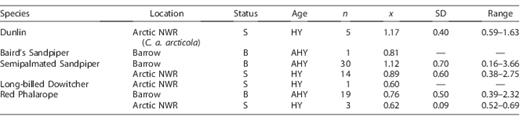
Number of samples (n), mean (x), standard deviation (SD), and the range of feather mercury concentrations (μg g−1, ww) by species, age (AHY = adult, HY = hatch year), and sampling location (status: B = breeding, S = post-breeding staging).
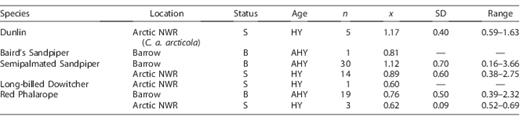
Blood mercury concentrations in individual Alaskan shorebirds ranged from a low of 0.03 μg g−1 in a staging Rock Sandpiper to a high of 2.20 μg g−1 in a breeding Semipalmated Sandpiper (Table 1). Mean blood mercury concentrations among 9 breeding shorebird species were less extreme, ranging from 0.18 μg g−1 in American Golden-Plover to 0.95 μg g−1 for Semipalmated Sandpiper; both of these averages were from the Barrow study site (Table 1). AHY birds sampled during staging generally had low blood mercury concentrations, with averages <0.11 μg g−1 (Table 1). As predicted, staging HY shorebirds also had low mean blood mercury concentrations, ranging from 0.12 μg g−1 in Red Phalarope to 0.25 μg g−1 in Rock Sandpiper (Table 1).
Feather mercury concentrations in individual shorebirds were generally higher than blood concentrations, ranging between 0.16 and 3.66 μg g−1; samples at both ends of this range were from AHY Semipalmated Sandpiper breeding at Barrow (Table 2). Across species, mean feather mercury concentrations ranged from 0.60 μg g−1 in the single HY Long-billed Dowitcher to 1.17 μg g−1 in HY Dunlin.
Sampling location
We found no significant difference in blood mercury concentrations between breeding Dunlin subspecies, C. a. arcticola sampled at Barrow and C. a. pacifica sampled at the Yukon Delta NWR (Table 3).
Statistical analyses for blood mercury concentrations (μg g−1, ww) and feather mercury concentrations (μg g−1, fw, correlation analyses only) for shorebird species sampled in different geographic locations and age classes (AHY = adult, HY = hatch year) in Alaska, USA, in 2008 and 2009.

Statistical analyses for blood mercury concentrations (μg g−1, ww) and feather mercury concentrations (μg g−1, fw, correlation analyses only) for shorebird species sampled in different geographic locations and age classes (AHY = adult, HY = hatch year) in Alaska, USA, in 2008 and 2009.

Habitat use
A comparison of blood mercury concentrations among 6 breeding species of shorebirds at Barrow indicated that species had significantly different concentrations (2008 and 2009 combined; Table 3), with the mean concentration for Semipalmated Sandpiper >4.5 times higher than that for American Golden-Plover (Figure 2). As predicted, we also found that blood mercury concentrations of shorebirds breeding at Barrow differed significantly by the moisture content of their predominant foraging habitat (Table 3). The upland habitat foraging group had the lowest blood mercury concentrations, with higher concentrations found in birds sampled in the intermediate or mixed habitat group, and even higher concentrations in the wet to aquatic habitat group.
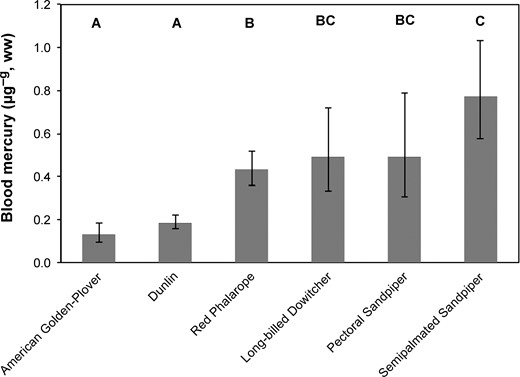
Back-transformed mean ±95% confidence intervals of blood mercury concentrations in shorebirds breeding at Barrow, Alaska, during 2008 and 2009. Sexes combined where applicable. Species sharing a letter do not differ (P > 0.05). Sample size: American Golden-Plover (n = 12), Dunlin (n = 42), Red Phalarope (n = 39), Long-billed Dowitcher (n = 9), Pectoral Sandpiper (n = 6), and Semipalmated Sandpiper (n = 16).
Transfer to feathers and eggs
For Dunlin staging at the same location, blood mercury concentrations of HY individuals were significantly greater than AHY (Table 3). As predicted, an analysis of mated breeding Dunlin sampled at both Barrow and Yukon Delta NWR found females (0.14 μg g−1; LCL = 0.12 and UCL = 0.17 μg g−1) to have significantly lower mercury concentrations than males (0.23 μg g−1; LCL = 0.20 and UCL = 0.27 μg g−1; Table 3).
Variation among years
The blood mercury concentrations of male Red Phalarope breeding at Barrow differed between years (Table 3). No year effect was found in breeding Dunlin at Barrow (Table 3).
Blood and feather mercury relationship
We found no relationship between blood and feather mercury concentrations for AHY Red Phalarope and AHY Semipalmated Sandpiper sampled during the breeding season or HY Semipalmated Sandpiper sampled while staging (Table 3).
Discussion
We found elevated blood mercury concentrations in breeding shorebirds sampled around Barrow, particularly in Semipalmated Sandpiper, Red Phalarope, Long-billed Dowitcher, and Pectoral Sandpiper. Blood mercury concentrations within our study are comparable, although higher for some species, to those measured by Hargreaves et al. (2011) for 6 species of breeding shorebirds in the eastern Canadian Arctic. In our study, Semipalmated Sandpiper, Pectoral Sandpiper, and Red Phalarope (sampled in 2008) all had greater mean blood mercury concentrations than the highest species concentration (Ruddy Turnstone, 0.59 μg g−1) in Hargreaves et al. (2011). Blood mercury concentrations for all breeding shorebird species sampled in this study, with the exception of Dunlin and American Golden-Plover, were also similar to those found in breeding Black-necked Stilt (Himantopus mexicanus; range: 0.47–1.65 μg g−1) and American Avocet (Recurvirostra americana; range: 0.30–0.68 μg g−1) sampled in San Francisco Bay wetlands with known point-source mercury pollution (Eagles-Smith et al. 2009). Overall, our results suggest that shorebirds breeding at Barrow are being exposed to elevated mercury concentrations. Lower blood mercury concentrations were found in breeding shorebirds at the Yukon Delta NWR and during the post-breeding staging at the Arctic and Yukon Delta NWRs.
The feather mercury concentrations in this study are similar to those found in body feathers of AHY Red Knot (Calidris canutus) and Sanderling (Calidris alba) sampled at a staging location in Delaware Bay (Burger et al. 1993, 2015). Feather mercury concentrations for AHY Semipalmated Sandpiper sampled in Delaware Bay, however, were lower than those measured in this study (Burger et al. 2015). By contrast, our feather mercury concentrations for HY shorebirds were generally low compared to feather mercury concentrations from recently hatched Black-necked Stilt and American Avocet sampled in mercury contaminated wetlands in San Francisco Bay (Ackerman et al. 2008).
Sampling location
As predicted, we found that shorebirds sampled at our northern sampling sites (Barrow and Arctic NWR) had greater blood mercury concentrations than those sampled at a sub-Arctic site (Yukon Delta NWR); however, only 2 species were sampled on the Yukon Delta NWR during the breeding season. One of 2 Bar-tailed Godwit (Limosa lapponica) sampled at the Yukon Delta NWR had a relatively high blood mercury concentration of 0.59 μg g−1, suggesting that more individuals should be sampled to evaluate the mercury risk for this species. We found no difference in blood mercury concentrations between Dunlin subspecies breeding at Barrow and the Yukon Delta NWR. Because these 2 subspecies winter in different regions of the world (East Asia and the Pacific Coast of North America), sampling feathers grown on the wintering grounds to assess differences in mercury exposure would be insightful. The 2 Alaskan subspecies of Dunlin also had similarly low blood mercury concentrations to a third subspecies, C. a. hudsonia, breeding in Nunavut, which had a mean concentration of 0.19 μg g−1 (Hargreaves et al. 2011). The low blood mercury concentrations found in Dunlin compared to the relatively high blood mercury concentrations of other species suggest that life history traits, such as foraging habitat and diet, may play a critical role in mercury exposure.
The majority of the shorebirds sampled during staging at both the Arctic NWR and Yukon Delta NWR had low blood mercury concentrations. In particular, AHY Rock Sandpiper sampled at the Yukon Delta NWR had the lowest mean blood mercury of all the sampled shorebird species. All shorebirds sampled at the Arctic NWR and many of those sampled at the Yukon Delta NWR were HY birds, which generally had low blood mercury concentrations. A few individual HY birds did have relatively high concentrations, however. Most noteworthy, a HY Semipalmated Sandpiper sampled at the Arctic NWR had a blood mercury concentration of 1.0 μg g−1. Unfortunately, mercury concentrations for staging shorebirds are difficult to interpret because we do not know their breeding site or how long they were at the staging area prior to sampling.
Habitat use
We found distinct differences in blood mercury concentrations among breeding shorebird species sampled at the Barrow study site, suggesting differential mercury exposure for shorebird species breeding in the same locality, possibly because of differences in foraging location. Mercury methylation occurs predominantly in wetland and aquatic environments, and thus individuals foraging in upland areas would be expected to have lower mercury exposure. As expected, we found that blood mercury concentrations of shorebirds differed among shorebird species grouped by primary foraging habitat, with the lowest mean blood mercury concentrations found in the upland foraging species. In this limited study, Arctic shorebirds that primarily forage in the wettest habitat had 2.5 times greater mercury exposure than their upland foraging counterparts.
By contrast, we found little variation in blood mercury concentrations among different HY shorebird species sampled in the Arctic NWR during staging. The AHY individuals of the same species breeding at Barrow (i.e. Semipalmated Sandpiper, Dunlin, and Red Phalarope) did have significantly different blood mercury values, however, possibly indicating that the young of these species could also differ.
Transfer to feathers and eggs
We examined the blood mercury concentrations between AHY and HY Rock Sandpipers and Dunlin sampled during staging at Yukon Delta NWR. We found no difference in blood mercury exposure by age for Rock Sandpiper but found HY Dunlin to have significantly greater blood mercury concentrations than AHY. These results contrast with our expectations, given that HY birds are able to eliminate mercury through feather growth (Fournier et al. 2002). The AHY birds were possibly also eliminating mercury through feather molt, however, because both species begin a body molt in July, with Dunlin also molting their flight feathers (Warnock and Gill 1996, Gill et al. 2002). In addition, because the breeding sites for these staging birds are unknown, the age classes could represent different geographic areas of mercury risk. A more definitive examination of how age classes compare would require sampling juveniles and adults from the same breeding area prior to molt.
We found female Dunlin to have significantly lower blood mercury concentrations than males attending the same nest, supporting the notion that females may transfer mercury into their eggs (Lewis and Furness 1993). Although Ackerman et al. (2007) also found female American Avocet and Black-necked Stilt had lower blood mercury concentrations than males, we did not find a significant difference between unmated male and female Semipalmated Sandpiper. The among-individual variation was likely sufficiently high to supersede sex-based differences in mercury concentrations.
Variation among years
Mercury exposure rates in Arctic wildlife could potentially increase with global climate change (Schuster et al. 2011, Stern et al. 2011). Previous research found mercury concentrations in the eggs of some Arctic-breeding seabirds significantly increased from 1975 to 2003 (Braune 2007). Increased egg mercury concentrations were also found for 4 species of Arctic-breeding shorebirds sampled at the same location in Nunavut between 2008 and 2011 (Hargreaves et al. 2010, 2011, McCloskey et al. 2013). In this study, we found that Dunlin had similarly low blood mercury concentrations in 2008 and 2009, whereas Red Phalarope were found to have significantly higher blood mercury concentrations in 2008 compared to 2009. These patterns are likely not meaningful given the short duration of this study but provide baseline data for later studies.
Blood and feather mercury relationship
As predicted, there was no relationship between blood and feather mercury concentrations in AHY Semipalmated Sandpiper and Red Phalarope. These AHY shorebirds likely grew their sampled secondary feathers on the wintering grounds where they would have received differing mercury inputs than from the breeding areas. We would expect a positive relationship between blood and feather mercury concentrations for individuals that grew their feathers at the location where blood was sampled, such as HY shorebirds sampled at their breeding grounds, but we did not have the samples to make such a comparison because all our HY birds were sampled while staging. The lack of a relationship between blood and feather mercury concentrations for staging HY Semipalmated Sandpiper suggests their exposure to mercury inputs when their flight feathers were grown on the breeding grounds differed from when they were sampled.
Adverse effects
Chronic exposure to mercury is known to reduce reproductive success in birds; however, the threshold at which reproductive success is reduced (the adverse effect level) can vary among species (Evers et al. 2008, Heinz et al. 2009, Jackson et al. 2011a). For the Common Loon, adverse effect levels for mercury are well established; a 10% reduction in fledged young was observed in adults with blood mercury concentrations at 2.0 μg g−1 (Burgess and Meyer 2008, Evers et al. 2008, 2011). Recent studies on avian invertivores, however, have shown adverse effects of mercury exposure on reproductive success at even lower mercury concentrations than those established for the Common Loon (Hallinger and Cristol 2011, Jackson et al. 2011a). Research on the Carolina Wren determined that mercury concentrations of 0.70 and 3.0 μg g−1 for blood and tail feathers, respectively, were associated with a 10% reduction in nest success (Jackson et al. 2011a), but assessments of adverse effects have not been fully developed for shorebirds. Heinz et al. (2009), in the only laboratory study that attempted to assess mercury impacts on shorebirds, did not determine median lethal concentrations for methylmercury-injected American Avocet and Black-necked Stilt embryos. Additionally, although Hargreaves et al. (2010) showed that paternal feather mercury concentrations were correlated with reduced hatching success for shorebirds breeding in Nunavut, they did not determine an adverse effect level related to reduced reproductive success. Therefore, the adverse effect levels determined for the Carolina Wren (Jackson et al. 2011a) are currently the best available estimates for reproductive effects of mercury on small-bodied invertivores. Using the earlier concentrations as benchmarks for shorebirds, our study suggests that shorebirds breeding around Barrow are at risk of reduced reproductive success from mercury exposure. The mean blood mercury concentration for Semipalmated Sandpiper exceeded this threshold, as did individual blood mercury concentrations for breeding Long-billed Dowitcher, Red Phalarope, Red-necked Phalarope (Phalaropus lobatus), Pectoral Sandpiper, and American Golden-Plover. The only birds sampled with feather mercury concentrations >3.0 μg g−1 were Semipalmated Sandpiper breeding at Barrow. We were unable to evaluate the relationship between mercury concentrations and reproductive success in this study because nesting success data were not available (Yukon Delta NWR) or were likely artificially enhanced by a predator removal program (Barrow).
Conclusions
This study indicates that multiple shorebird species breeding in Alaska have elevated blood and feather mercury concentrations. Some species had greater blood mercury concentrations than others, likely related to breeding location, habitat association, age, and sex. In some species, mercury exposure was sufficiently high to produce potential adverse reproductive effects. Our results, combined with those of Hargreaves et al. (2010, 2011), indicate that mercury exposure in Arctic-breeding shorebirds may be widespread and potentially detrimental. This mercury exposure may be particularly concerning when combined with other ecological stressors, such as habitat loss, predation, disturbance, and climate change. Future research is needed to determine whether these exposure levels are typical, why birds breeding at Barrow have elevated exposure, and how elevated mercury exposure might impact individual productivity. Given the high degree of conservation concern for many Arctic-breeding shorebird species, we recommend further research to develop a mercury exposure profile for multiple shorebird species breeding across the North American Arctic. This type of research would determine the extent of mercury contamination in Arctic shorebirds, identify species at greatest risk from environmental mercury loads, and determine where biological mercury hotspots (such as Barrow) might exist. Further studies using stable isotopes of carbon, nitrogen, and mercury are needed to better understand the routes of exposure to methylmercury and the mechanisms of food web transfer. In addition, marking individuals with known mercury body burdens would facilitate the tracking of mercury effects on reproductive performance of shorebirds.
Acknowledgments
We thank the many field technicians, but especially Brooke Hill, Dan Ruthrauff, Roy Churchwell, and Matt Sexson (U.S. Geological Survey, Alaska Science Center) that collected samples in remote regions of Alaska. We thank the Kaktovik Inupiat Corporation for allowing us to conduct research on their lands.
Funding statement: Funding for the collection of shorebird samples and mercury analysis was provided by the Biodiversity Research Institute, Manomet Center for Conservation Sciences, and the U.S. Fish and Wildlife Service. Individuals from each of the funding organizations are authors on the manuscript, but none of the funders had influence on the content of the manuscript or required approval of the final manuscript. The conclusions in this article are solely those of the authors and do not necessarily represent the views of the U.S. government.
Ethics statement: Capture, handling, and sampling of animals took place under applicable state and federal permits for wildlife research under Biodiversity Research Institute and the U.S. Geological Survey and followed protocols approved by the Institutional Animal Care and Use Committees at the U.S. Fish and Wildlife Service and University of Alaska Fairbanks.
Author contributions: D.C.E., I.J.S., and R.B.L. conceived the idea, design, and experiment. R.B.L., S.K., S.B., J.O.H., K.R., and H.R.G. performed the experiments. M.P., L.F., R.B.L., D.C.E., and I.J.S. wrote the paper. D.C.E., I.J.S., R.B.L., M.P., and L.F. developed or designed methods. M.P. and L.F. analyzed the data. R.B.L., S.K., S.B., J.O.H., D.C.E., and I.J.S. contributed substantial materials, resources, or funding.
Literature Cited
Author notes
Current address: Department of Natural Resource Sciences, McGill University, Ste Anne de Bellevue, Quebec, Canada
Current address: The Wetlands Institute, Stone Harbor, New Jersey, USA
Current address: U.S. Fish and Wildlife Service, Hakalau Forest National Wildlife Refuge, Hilo, Hawaii, USA