-
PDF
- Split View
-
Views
-
Cite
Cite
Jason M. Hill, Jennifer Walsh, Adrienne I. Kovach, Chris S. Elphick, Male-Skewed Sex Ratio in Saltmarsh Sparrow Nestlings: Proporción de Sexos Sesgada hacia los Machos en Pichones de Ammodramus caudacutus, The Condor: Ornithological Applications, Volume 115, Issue 2, 1 May 2013, Pages 411–420, https://doi.org/10.1525/cond.2013.120116
- Share Icon Share
Abstract.
Sex ratios of adult birds are frequently skewed, but in few species is the sex ratio of offspring also skewed. Adult females, however, should modify their offspring's sex ratio and invest more in the sex with the greatest future reproductive potential. We investigated the relationship between nestlings' morphology, timing of reproduction, and sex ratios in broods of the Saltmarsh Sparrow (Ammodramus caudacutus), a species with extreme levels of multiple paternity: males provide no parental care and sex ratios of adults may be highly skewed. By molecular methods, we sexed 157 nestlings from 52 Saltmarsh Sparrow nests in Connecticut over 2 years. Their sex ratio was significantly skewed toward males (59%) and did not vary with brood size, but this imbalance is insufficient to fully explain the reported skew in adults' sex ratio. Just prior to fledging, male nestlings were larger than female nestlings, which may suggest that they are more costly to produce. Survivorship is greater in nests initiated immediately after high spring tides, but we found no relationship between sex-ratio bias and the risk of tidal flooding. Female Saltmarsh Sparrows consistently produce male-skewed broods, regardless of their nests' risk of flooding, suggesting that male nestlings are a consistently better investment. The Saltmarsh Sparrow appears to be an exception to the pattern typical of birds, in which the population of adults is skewed toward males but the sex ratio of offspring is balanced. These results may help to refine future age-specific population projections for this species of high conservation concern.
Resumen.
La proporción de sexos en las aves adultas está frecuentemente sesgada, pero en pocas especies también está sesgada en la descendencia. Las hembras adultas, sin embargo, deberían modificar la proporción de sexos de sus descendientes e invertir más en el sexo con el mayor potencial reproductive a futuro. Investigamos la relación entre la morfología de los pichones, la sincronización de la reproducción y la proporción de sexos en las nidadas de Ammodramus caudacutus, una especie con niveles extremos de paternidad múltiple: los machos no brindan cuidado parental y la proporción de sexos de los adultos puede estar muy sesgada. Por medio de métodos moleculares, determinamos el sexo de 157 pichones provenientes de 52 nidos de A. caudacutus en Connecticut a lo largo de dos años. Su proporción de sexos estuvo significativamente sesgada hacia los machos (59%) y no varió con el tamaño de la nidada, pero esta desproporción es insuficiente para explicar acabadamente el sesgo reportado en la proporción de sexos de los adultos. Justo antes del emplumamiento, los pichones macho fueron más grandes que los pichones hembra, lo que puede sugerir que son más costos de producir. La supervivencia es mayor en los nidos iniciados inmediatamente después de las mareas altas de primavera, pero no encontramos una relación entre el sesgo en la proporción de sexos y el riesgo de inundación por las mareas. Las hembras de A. caudacutus produjeron sistemáticamente nidadas sesgadas hacia los machos, independientemente del riesgo de inundación de sus nidos, sugiriendo que los pichones macho son sistemáticamente una mejor inversión. A. caudacutus parece ser una excepeión del patrón típico de las aves, en el cual la población de adultos está sesgada hacia los machos pero la proporción de sexos de la descendencia está balanceada. Estos resultados pueden ayudar a ajustar futuras proyecciones poblacionales específicas de la edad para esta especie con alto valor de conservación.
Introduction
In many bird species, the adults' sex ratio (ASR) is skewed towards one sex (Donald 2007). Of the species reviewed by Donald (2007), 65% had ASR significantly skewed, most often toward males (85%). In birds, skewed ASRs are generally explained as originating from two sources of differential mortality: the cost of reproductive care and the cost of mate acquisition (Liker and Székely 2005). In relatively few bird species (∼10%) is parental care by a single sex (Cockburn 2006), but females generally provide disproportionately more care than males (Schwagmeyer et al. 1999). Female birds' mortality rates are generally higher than those of males (Searcy and Yasukawa 1981, Liker and Székely 2005), perhaps stemming (at least in some species) from their increased parental investment (Trivers 1972, Bennett and Owens 2002). The costs associated with mate acquisition, however, are incurred largely by males (Liker and Székely 2005). Males may face higher energetic costs and mortality than females as result of their brighter plumage coloration (Promislow et al. 1992), ornamentation (Tufvesson et al. 1999), territorial behavior (Ydenberg and Krebs 1987), or male—male fighting (Payne 1984).
In contrast to those among adults, skewed sex ratios among offspring are uncommon, most studies reporting balanced ratios (Donald 2007). These findings support the Darwin-Fisher hypothesis (Darwin 1871, Fisher 1930) that a balanced ratio of offspring is adaptive when both sexes are equally costly to produce. Trivers and Willard (1973), however, proposed that females should favor the production of the sex that has the greater future reproductive value. This primary sex-ratio manipulation could occur through such mechanisms as hormonal control of yolk development in follicles that give rise to male and female gametes (Pike and Petrie 2003) or disproportionate production of male or female oocytes (Badyaev 2009). Subsequent experimental studies have skewed the sex ratio of offspring by manipulating maternal condition, food availability, and territory quality (Komdeur et al. 1997, Robertson et al. 2006), and meta-analyses have found evidence for facultative sex-ratio adjustment in response to mate quality, breeding system, environmental predictability, the sequence in which eggs are laid, territory quality, and time within the breeding season (West and Sheldon 2002, Cassey et al. 2006). In addition, in studies of individual species, the sex ratio of offspring has been reported to vary over the course of a breeding season (Blank and Nolan 1983, Graham et al. 2011). It can fluctuate dramatically from year to year, perhaps favoring the sex rarer in the previous year (Byholm et al. 2002). Variation also has been linked to a large array of other factors including climatic variation (Weatherhead 2005), food supply (Wiebe and Bortolotti 1992, Dzus et al. 1996), and the presence of brood parasites (Zanette et al. 2005). The possibility that some of these patterns are simply artificats of sampling also remains (Cassey et al. 2006).
Like most birds, the Saltmarsh Sparrow (Ammodramus caudacutus) has been reported to have a male-skewed ASR (reviewed in Greenlaw and Rising 1994). In some cases, the apparent imbalance is considerable, frequently exceeding two males for every female (Greenlaw and Rising 1994). For example, in the populations we studied in Connecticut, we have found a ratio of 2.7:1 during standardized mist netting (Gjerdrum et al. 2008a). Survival has not been analyzed with mark—recapture studies, but return rates suggest that this apparent sex-ratio bias is not caused by differential survival of adults and rather may be due to differences between the sexes in home-range size (Post and Greenlaw 1982). Recent telemetry-based estimates of home-range size (Shriver et al. 2010), however, indicate that differences in space use alone may be insufficient to account for the ASR bias.
The Saltmarsh Sparrow also has an unusual mating system, with one of the highest levels of multiple paternity of any bird species measured. Hill et al. (2010) found that in one-third of nests every nestling was sired by a different male and that in all 57 completely sampled nests paternity was multiple (Hill et al. 2010). Females provide all parental care, and the nonterritorial males compete overtly for access to females via scramble competition (Woolfenden 1956, Greenlaw and Post 2012). Skewed ASRs have been linked to the evolution of mating systems and sex roles (Donald 2007, Kokko and Jennions 2008), so understanding the origins of the Saltmarsh Sparrow's unusual mating system would benefit from a clearer understanding of sex-ratio bias in the species. Given that current knowledge of mortality rates and space use appear insufficient to explain the bias, we investigated the sex ratio of offspring. Although in birds the sex ratio of offspring rarely diverges from 0.5 (Donald 2007), we investigated whether the Saltmarsh Sparrow's male-skewed ASR could be explained by a male-skewed sex ratio of the offspring.
Many aspects of Saltmarsh Sparrow reproduction are linked to the tidal cycle, so we also investigated whether this has an influence on sex allocation. Saltmarsh Sparrow nests are constructed near ground level, and nest initiation is often highly synchronized with the lunar cycle because of the related tidal flooding (Shriver et al. 2007). Nest loss from flooding can exceed 50% (DeRagon 1988, Bayard and Elphick 2011), and nests are more likely to succeed if they are initiated shortly after high spring tides, which occur roughly every 28 days (Gjerdrum et al. 2005, Shriver et al. 2007). At our study sites, most Saltmarsh Sparrow nests are flooded repeatedly during the nesting cycle (Bayard and Elphick 2011). Nests, however, are typically underwater for only about 90 min during the tidal peak, and both eggs and older nestlings are capable of surviving complete inundation of the nest (Gjerdrum et al. 2008b). Chicks achieve this feat by climbing out of the nest and up through the vegetation to stay above the water level (J. Hill and J. Walsh, pers. obs.). Adult male Saltmarsh Sparrows average approximately 10% larger than females (Woolfenden 1956), and this pattern may apply to nestlings also. Although the hypothesis is untested, larger nestlings may survive floods better because their greater mass offers more protection against hypothermia and their larger size makes them more adept climbers. If females can manipulate the sex of their offspring then it could be advantageous to produce more chicks of the sex with the greater ability to survive floods. Larger chicks could be especially advantageous in nests initiated later in the lunar cycle because these nests are most likely to suffer flooding. Alternatively, if females cannot assess tidal intervals, they may favor the larger sex without regard to the risk of flooding.
Measurements of nestlings' morphology can shed light on the cost of producing male and female offspring (Slagsvold et al. 1986). Larger or heavier offspring are generally more costly to produce (Teather and Weatherhead 1988) but have higher rates of post-fledgling survival (Naef-Daenzer et al. 2001, Potti et al. 2002). This higher rate of survival may stem in part from the ability of larger nestlings to outcompete their smaller siblings for access to food (Råberg et al. 2005).
We used a combination of molecular and morphological measurements to test our hypotheses regarding sex-specific production of offspring in the Saltmarsh Sparrow. We collected blood samples from nestlings to test whether nestlings' overall sex ratio was male-skewed and assessed the ability of standard morphological measurements to predict nestlings' sex with principal components analysis (PCA). We predicted that the overall sex ratio should be male-skewed. Since adult males are larger and heavier than adult females (Woolfenden 1956), we predicted that male nestlings should be larger and heavier than female nestlings. We used generalized estimating equations (GEE, Liang and Zeger 1986) to examine variation in the production and body mass of male nestlings over the course of the nesting season and in relation to high spring tides.
Methods
During the summers of 2006 and 2007, we searched for Saltmarsh Sparrow nests throughout ten salt marshes along the Connecticut coast of Long Island Sound (Fig. 1). We found nests by flushing females during systematic weekly searches of each marsh or by observing female sparrows carrying food. We monitored nests approximately every 4 days until the young fledged or the nest failed, and found 78% of 52 nests prior to hatching. We aged nestlings by observing hatching (day = 0) or by the descriptions of Woolfenden (1956). To calculate clutchinitiation dates we assumed a 12-day incubation period beginning with the laying of the penultimate egg (Greenlaw and Rising 1994). We numbered each day of the nesting season sequentially, starting with 28 May in both years (our earliest clutch-initiation date) and assigned nests a corresponding initiation date. With our monitoring methods, we could not determine the eggs' order of hatching, but in this species all eggs usually hatch within 24 to 36 hr of each other (Woolfenden 1956).
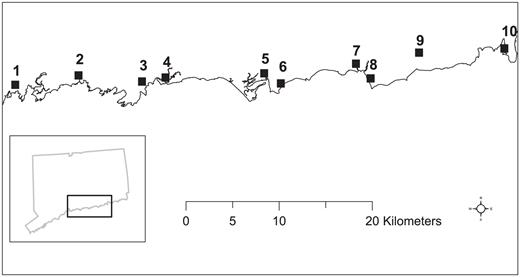
Salt marshes along the Connecticut coast of Long Island Sound where Saltmarsh Sparrow nests were monitored during the summers of 2006 and 2007: 1, Farm River (91 ha); 2, Pine Orchard (50 ha); 3, West River (131 ha); 4, East River (337 ha); 5, Indian River (33 ha); 6, Hammock River (123 ha); 7, Hager Creek (28 ha); 8, Plum Bank (158 ha); 9, Lieutenant River (417 ha); 10, Watt's Island (67 ha).
Although spring tides coincide with both the full and new moons, the higher spring tides that cause flooding typically occur only every other cycle. This cycling is not exact, however, and there is annual variation in whether the highest spring tides coincide with the full moon or the new moon. In our study area, spring tides associated with the full moon were consistently higher than new-moon tides in 2006, lower in 2007 (data from National Oceanic and Atmospheric Administration, station 8465705). Following Shriver et al. (2007) we used the clutch-initiation dates to determine how quickly a nest was initiated following the most recent high spring tides.
When nestlings were 6 to 8 days old they were banded and a 30-µL blood sample was taken via brachial-vein puncture and collected with capillary tubes. Blood samples were temporarily kept in a cooler on ice in the field, then stored in Eppendorf tubes filled with a buffer solution (Longmire et al. 1988) consisting of 100 mM Tris HCl at pH 8.0, 100 mM EDTA, 10 mM NaCl, and 0.5% sodium dodecyl sulfate. We strove to visit nests for blood sampling when nestlings were exactly 7 days old (2–3 days prior to fledgling), but our estimates of hatching dates were likely imperfect for some nests (±1 day). During these nest visits we also took standard morphological measurements: body mass (g), wing chord (mm), bill width and length (mm), and tarsus and culmen length (mm). To reduce handling times on cool days or during floods, some measurements were not taken on nestlings from seven nests. We attempted to capture and color-band the attending female of each nest and had success with 32 of 49. Comparing the times and locations of nests with banded and unhanded females suggested that all of the unhanded females were likely different individuals. Three banded females each produced two nests. Except in the GEE models as subsequently described, we randomly excluded one nest from each of these females from our analyses. We excluded from morphological analyses adults and nestlings for which any measurement was missing.
We extracted DNA from blood samples with a DNeasy Blood Kit (Qiagen, Valencia, CA) and identified the sex by amplifying the CHD1 gene on the avian sex chromosomes. Becuase of a difference in the size of an intron on the CHD1-W and CHD1-Z genes, this method results in two fragments of different sizes in females and one fragment in males. For PCR amplification we used the primers 2550F and 2718R (Fridolfsson and Ellegren 1999), following the methods of Quintana et al. (2008). Amplified products were resolved on a 1% agarose gel, and primers were validated with nine samples of adult Saltmarsh Sparrows of known sex (5 males and 4 females). In all nine tests, the genetic identification corresponded with field identification via the presence of a brood patch or cloacal protuberance in females and males, respectively (Pyle 1997).
Statistical Analyses
For analyses we used the R statistical software. We first used an ANOVA to examine variation in sex ratios across all sizes of broods because a large disparity among brood sizes could unduly influence our test for a disparity in the nestlings' overall sex ratio. On the basis of these results we included nestlings from all brood sizes in subsequent analyses. To examine differences in morphology by sex and temporal variation in sex-specific production of offspring, we used GEEs with a logit-link transformation and nestling sex (1 = male, 0 = female) as the response variable (Poisbleau et al. 2012). We used the Geepack package (Halekoh et al. 2006) in R with an exchangeable working correlation structure, also known as a compound symmetry covariance structure (Zuur et al. 2009), clustered on maternal identity to account for the likely correlation among siblings' measurements. This approach accounts for the lack of independence between siblings and correspondingly adjusts the test statistics and standard errors of parameter estimates, resulting in a more conservative test of the main effects (Liang and Zeger 1986). We used plots of binned residuals to assess model fit (Gelman and Hill 2006). We examined possible deviation from a 1:1 ratio by using an intercept-only GEE model, akin to the random-intercept modeling approach of Graham et al. (2011). To examine changes in nestlings' sex ratio over the breeding season we used the same basic model with clutchinitiation date included as an explanatory variable. We also used the basic model with the number of days between nest initiation and the previous high spring tide to determine if nestlings' sex was related to the tidal cycle. Prior to blood collection, some nests lost eggs and nestlings to drowning or partial nest predation. Because we were unable to determine the sex of lost eggs and nestlings, we estimated the potential effect of these missing offspring on the nestlings' overall sex ratio with a binomial test that assumed a near-even sex ratio in the missing individuals. Unlike the GEE models, however, the binomial test does not account for the lack of independence among nestlings. All sex ratios are presented as the proportion of male nestlings in our sample.
To determine if we could distinguish nestlings' sex by their morphology, we first fit binomial GEE models, as previously described, with a single morphological character. We did not examine multivariate models since preliminary analysis revealed that all morphological variables were strongly (rs ≥ 0.43) and significantly (P < 0.001) correlated with each other. Instead, we applied PCA to the morphological data. We first standardized the variables with the Vegan package (Oksanen et al. 2011). To test the ability of the principal components to predict a nestling's sex, we used a binomial generalized linear model that included the predicted scores for any principal component that explained >10% of the variation in the morphological data. Results are presented as coefficients ± SE for models and means ± SE for morphologic data.
Results
We sampled 157 nestlings from 52 nests and were able to ascertain the sex of all nestlings genetically. Ninety-three male (59%) and 64 female (41%) nestlings were sampled during the two years. The proportion of males in our sample was significantly greater than 0.5 (intercept ± SE = 0.38 ± 0.16, Wald χ2 = 6.0, P = 0.02). The odds of a nestling being male were 1.46 (= e0.378) times higher than of its being female (approximate 95% CI = 1.08, 1.98). While under observation, 15 nests (28.8%) lost a total of 17 eggs or nestlings that could not be sexed. Even if the sex ratio in these 17 eggs and nestlings was nearly even (8 males, 9 females), we would likely have still detected a skewed ratio (binomial test with 101 males out of 174 offspring, P = 0.04; = 0.58, 95% CI: 0.503, 0.655). There was no evidence that the sex ratio varied by brood size, and point estimates of the mean ratio for each brood size were all above 0.5 (Fig. 2).
We obtained complete morphological measurements from 134 (85%) of the 157 nestlings. Male nestlings were produced at a steady rate through the nesting season (coefficient ± SE = -0.01 ± 0.01, Wald χ2 = 0.5, P = 0.48) and without regard to the timing of high spring tides (Fig. 3; 0.02 ± 0.01, χ2 = 1.8, P = 0.18). Nestlings' body mass did not vary through the nesting season (0.016 ± 0.02, χ2 = 0.5, P = 0.47) and was unrelated to the timing of high spring tides (-0.002 ± 0.04, χ2 = 0.01, P = 0.96). Linear measurements of the sexes were similar (Table 1), but male nestlings averaged 31% heavier than females (Fig. 4; 95% CI: 1.15, 1.49). The first two principal components of our PCA showed some ability to separate male from female nestlings and explained 65% and 11% of the variation, respectively; all other components individually explained <10% of the variation in the morphological data (Fig. 5). The loadings of the eigenvalue equations of the first two principal components were Z1 = -0.45 wing length - 0.38 body mass 0.40 tarsus length - 0.41 culmen - 0.43 bill length - 0.37 bill width and Z2 = -0.03 wing length + 0.68 body mass + 0.34 tarsus length - 0.48 culmen - 0.04 bill length - 0.44 bill width. Of the first two principal components, scores of only the second were predictive of nestlings' sex (-0.01 ± 0.10, z = -0.1, P = 0.96 and 0.87 ± 0.28, z = 3.1, P = 0.002, respectively).
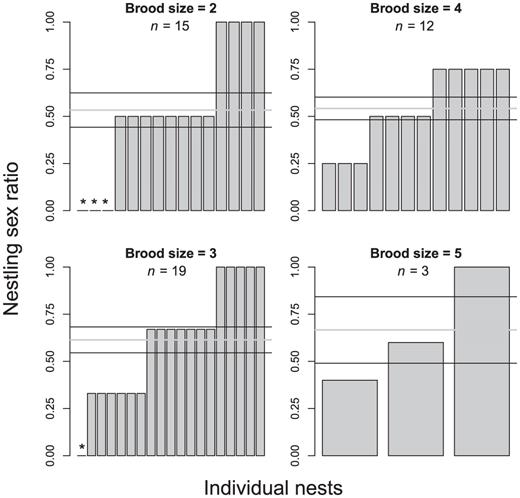
Sex ratio of offspring in 49 Saltmarsh Sparrow nests by brood size in salt marshes along Long Island Sound, 2006–2007. Gray lines, means; black lines, ±SE (black lines). One nest of each of the three females that contributed data from two nests was excluded. Nests without male offspring are denoted with “*”. There was no evidence that the sex ratio varied by brood size (F 3,45 = 0.4, P = 0.79).
Results of the univariate generalized estimating equation comparing morphological traits of male (n = 81) and female (n = 53) Saltmarsh Sparrow nestlings in 2006 and 2007 along ∼60 km of Long Island Sound, Connecticut. Each nestling was measured once, approximately 2 or 3 days prior to fledging. Coefficients are presented on the logit scale. Means of morphological measurements are rounded to the nearest integer.
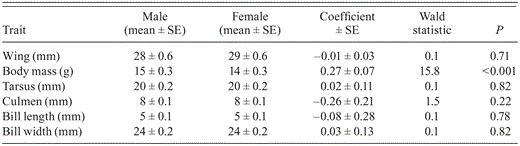
Results of the univariate generalized estimating equation comparing morphological traits of male (n = 81) and female (n = 53) Saltmarsh Sparrow nestlings in 2006 and 2007 along ∼60 km of Long Island Sound, Connecticut. Each nestling was measured once, approximately 2 or 3 days prior to fledging. Coefficients are presented on the logit scale. Means of morphological measurements are rounded to the nearest integer.
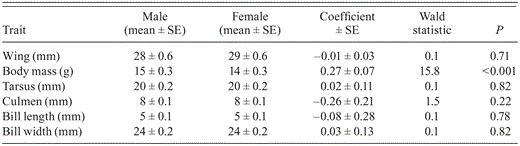
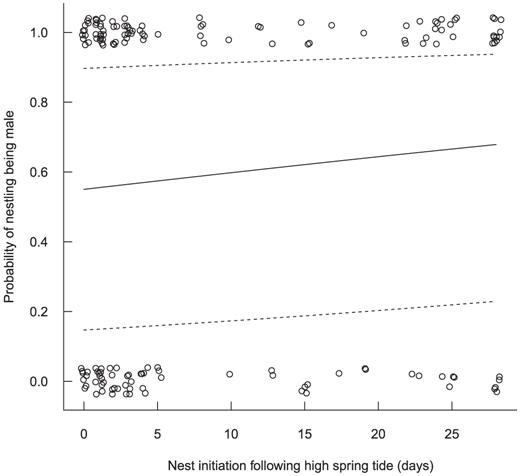
The probability, with 95% confidence intervals, of a Saltmarsh Sparrow nestling being male given the number of days a nest was initiated after the most recent high spring tide (day 0). Male nestlings were produced at a similar rate irrespective of timing (coefficient ± SE = 0.02 ± 0.01, Wald statistic = 1.8, P = 0.18), as assessed via generalized estimating equations accounting for the potential non-independence among siblings.
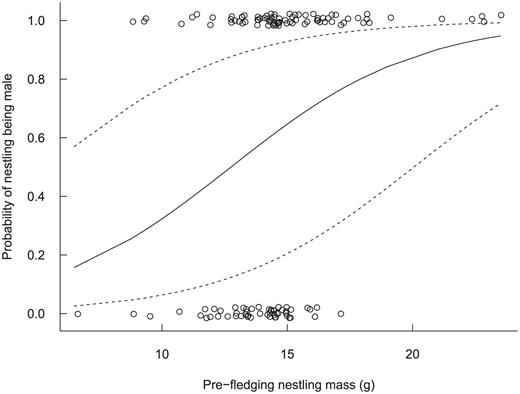
The probability, with 95% confidence intervals, that a Saltmarsh Sparrow nestling was molecularly sexed as male, given its body mass. Male nestlings averaged 31% (95% CI: 1.15, 1.49) larger than female nestlings (0.27 ± 0.07, P < 0.001), as assessed via generalized estimating equations accounting for the potential non-independence among siblings.
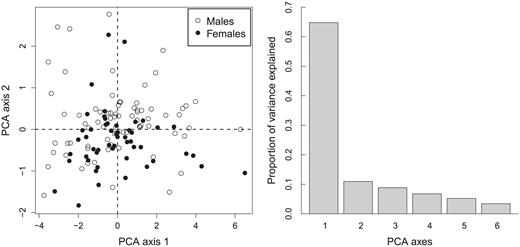
Plot of the first and second principal component axes (left panel) resulting from a principal component analysis of six morphometric measurements taken from Saltmarsh Sparrow nestlings 2–3 days prior to fledging (n = 127). The predicted second principal component scores predicted nestlings' sex, while the predicted first principal component scores did not. The first two axes cumulatively explained 76% of the variation in the dataset, and the proportion of variance explained in the PCA by each principal component axis is given in the right panel.
Discussion
Our findings demonstrate a skewed sex ratio in Saltmarsh Sparrow offspring, but it is insufficient to explain the ASR observed in this species. Adult females produced significantly more male offspring than female offspring over the two years of our study. These results differ from those of most bird studies, which have documented few differences in the frequency at which male and female offspring are produced (Donald 2007). Patterns of manipulation in the sex ratio of offspring can vary even among closely related species like the Australian miners (Manorina spp.), in which offspring of three species have been reported to have a male-skewed, a balanced, and a female-skewed sex ratios (Arnold et al. 2001, Ewen et al. 2001, Clarke et al. 2002). Likewise, in contrast to our results with the Saltmarsh Sparrow, Postma et al. (2011) reported a balanced ratio in the offspring from 699 Song Sparrow (Melospiza melodia) broods. We can only speculate about the different evolutionary forces operating on the sex ratios of these two sparrows, but one striking difference between them is their mating systems. Song Sparrows are socially monogamous, territorial, and both sexes provide parental care to their offspring (Hill et al. 2011). Saltmarsh Sparrows, on the other hand, have one of the highest levels of multiple paternity of any avian species (Hill et al. 2010), and males provide no parental care, do not form pair bonds, and are nonterritorial (Woolfenden 1956).
We estimated the offspring sex ratio as 1.45:1, males to females. Our estimate could be affected by the 17 individuals that died prior to sampling if the sexes are unequal in probability of hatching (Pike and Petrie 2003, Alonso-Alvarez 2006) or probability of surviving the first few days after hatching (Székely et al. 2006). Other studies have documented maleskewed mortality of nestlings and eggs (Teather and Weatherhead 1989, Whittingham and Dunn 2000). We found no relationship, however, between brood size and a nest's sex ratio (Fig. 1) and no evidence that an even sex ratio among the unsexed birds would have led to a different conclusion.
The sex ratio of offspring may change steadily through the breeding season (Krebs et al. 2002, Graham et al. 2011), perhaps in relation to local food availability (Dzus et al. 1996, Komdeur 1996). We did not, however, detect a change in the sex ratio of Saltmarsh Sparrow nestlings over the course of the nesting season. Insect communities within salt marshes fluctuate seasonally (Davis and Gray 1966), but Saltmarsh Sparrows appear to target seasonally abundant species, switching their prey (Post and Greenlaw 2005), and we found no evidence for changes in nestlings' body mass across the season. Adult females can maintain their body mass through the season (Post and Greenlaw 1982; J. Hill, unpubl. data), which may enable them to consistently produce more of the larger (i.e., male) offspring. Our results match the pattern found in a wide range of avian taxa including the Tree Swallow (Tachycineta bicolor, Whittingham and Dunn 2000), Boreal Owl (Aegolius funereus; Hipkiss and Hornfeldt 2004), and White Stork (Ciconia ciconia; Tryjanowski et al. 2011) in which the brood's sex ratio was unrelated to date of laying within a season.
We also found no relationship between production of males or body mass and the timing of the high spring tides that are related to a nest's probability of success (Shriver et al. 2007). This result suggests that female Saltmarsh Sparrows are either incapable of controlling the timing of their differential production or that there is no benefit to reducing production of males or increasing nestlings' mass (e.g., by more frequent feeding) later in the tidal cycle. The offsprings' consistently male-skewed sex ratio suggests that, despite their larger mass and potentially greater cost to produce, males are more valuable (Trivers and Willard 1973). Lifetime reproductive success of either male or female Saltmarsh Sparrows has not been estimated, however, and would be necessary to test this hypothesis directly. Male Saltmarsh Sparrow nestlings may be a better investment if they are more likely to survive floods (because of being larger than female nestlings) and if males' reproductive success is greater than than females' because of advantage in scramble competition or greater longevity (as suggested by the male-skewed ASR).
We found support through our PCA and subsequent modeling that male nestlings tend to be heavier than females, with longer tarsi, and smaller bills. These findings mirror the sexual dimorphism in adult Saltmarsh Sparrows (Woolfenden 1956). In dimorphic species in which the male is larger, male nestlings can be more costly for parents to produce (Teather and Weatherhead 1988). For instance, in the Common Murre (Uria aalge), in which male offspring are only slightly larger than their female siblings, parents rearing male chicks lost twice the mass they did when rearing female nestlings (Cameron-MacMillan et al. 2007).
Our findings suggest that although the male-skewed sex ratio in the Saltmarsh Sparrow is present from fledging, the sex ratio of offspring alone is insufficient to explain the observed male-skewed ASR. Five attempts have been made to quantify the ASR of the Saltmarsh Sparrow through banding data, and all have concluded it to be highly skewed, between 1.9 and 2.7 adult males per adult female (reviewed in Greenlaw and Rising 1994, Gjerdrum et al. 2008a). Some combination of differences between the sexes in home-range size, probability of capture, and post-fledging mortality must therefore also play a role. A number of factors could lead to a sex ratio increasingly skewed from egg to adult (Clarke et al. 2002). As in many species of waterfowl (Anatidae), predators discovering a nest may kill the attending female disproportionately (Sargeant et al. 1984). Alternatively, behavioral differences between the sexes may make females more vulnerable to predation or other sources of mortality away from the nest (Kenward et al. 1981). Since female birds generally disperse farther than males (Clarke et al. 1997), they may also face increased risks during this period (Steifetten and Dale 2006). Post and Greenlaw (1982) and Di-Quinzio et al. (2001) found no sexual differences in apparent return rates of Saltmarsh Sparrows, but return rates are troublesome proxies of survivorship since they do not account for site fidelity, emigration, or probability of detection (Lebreton et al. 1992, Martin et al. 1995).
Recently, concern has been raised about the effects of a skewed sex ratio on species of conservation concern. Donald (2007) found that the ASR in populations of globally threatened bird species was significantly more skewed than in nonthreatened species. This may be of particular concern for the Saltmarsh Sparrow, a species of concern (American Bird Conservancy 2007, BirdLife International 2009), if a male-skewed ratio of nestlings exacerbates the male-skewed ASR. Sex ratios skewed toward adult males can inhibit population growth by lowering the effective population size (Nomura 2002, Lenz et al. 2007, but see Ewen et al. 2011) and can result in higher mortality and lower fecundity of females through more frequent aggressive encounters with males (Le Galliard et al. 2005). Unmated males may aggressively compete for access to females at their nests, resulting in egg loss and nestling mortality (Taylor et al. 2001, Anderson et al. 2004). This risk is especially plausible in the Saltmarsh Sparrow, in which copulation may be forced upon females and is often associated with fights involving multiple males (Woolfenden 1956, Greenlaw and Post 2012). These antagonistic encounters likely increase as the population becomes more male-skewed. Future studies should incorporate information about sex ratios of offspring and adults into population projections and evaluate the effect of a male-skewed population on the species' risk of extinction and turnover within a confined area (Possingham et al. 1993, Steifetten and Dale 2006, Ferrer et al. 2009). Comparing sex ratios at different life stages and better survival estimates could help identify especially hazardous periods and improve age-specific population estimates, especially if the probability of detection differs by sex (Newson et al. 2005), and determining a species' population trend and status throughout its range is a priority (Greenlaw and Rising 1994, Elphick 2006).
Acknowledgments
We thank the Office of Long Island Sound Programs, Association of Field Ornithologists (E. Alexander Bergstrom Memorial Research Award), Wilson Ornithological Society, Eastern Bird Banding Association, and the Department of Ecology and Evolutionary Biology at the University of Connecticut for providing financial support. We offer thanks to T. Bayard, S. Meiman, J. Junda, C. Field, C. Kellogg, and E. Dario for their assistance in the field, to the Guilford and Clinton Connecticut Land Conservation Trusts and all of the many private property and business owners who granted us permission to access their properties, and to D. Diefenbach and T. Wagner for comments on our statistical analysis.
Literature Cited