-
PDF
- Split View
-
Views
-
Cite
Cite
Theresa L. Pope, Tara J. Conkling, Kathryn N. Smith, Melanie R. Colon, Michael L. Morrison, R. Neal Wilkins, Effects of Adult Behavior and Nest-Site Characteristics on Black-Capped Vireo Nest Survival, The Condor: Ornithological Applications, Volume 115, Issue 1, 1 February 2013, Pages 155–162, https://doi.org/10.1525/cond.2012.110141
- Share Icon Share
Abstract.
Predation is the leading cause of nest failure for many bird species. Most studies associate risk of predation with vegetation characteristics of the nest site. Alternatively, the Skutch hypothesis suggests that adults' activity at the nest can attract the attention of predators and lead to an increased risk of predation. We investigated whether vegetation characteristics surrounding nest sites affected nest attentiveness and visitation and if these behaviors affected nest survival. We used 1-hr nest observations and 8-hr video observations at Black-capped Vireo (Vireo atricapilla) nests in three regions of Texas to quantify nest attentiveness and visitation during incubation and the nestling stage. Females spent 80% more time on nests during incubation and 250% more time on nests during the nestling stage than did males, but visitation was similar for each sex. In general, nest behavior did not appear to be affected by vegetation characteristics, except males' attentiveness during incubation increased as average cover from 0 to 2 m increased. Adults' activity did not appear to increase the risk of nest predation. Despite visitation being more frequent during the nestling stage than during incubation, visitation during either stage did not affect nest survival. Overall, nest survival improved with attentiveness during incubation.
Resumen.
La depredación es la causa principal del fracaso del nido para muchas especies de aves. La mayoría de los estudios asocian el riesgo de depredación con las características de la vegetación del sitio de nidificación. Alternativamente, la hipótesis de Skutch sugiere que la actividad de los adultos en el nido puede atraer la atención de los depredadores y conducir a un aumento en el riesgo de depredación. Investigamos si las características de la vegetación que rodea a los nidos afectaron la atención y las visitas a los nidos y si estos comportamientos afectaron la supervivencia del nido. Empleamos observaciones directas del nido de 1 hr y videos de 8 hr en nidos de Vireo atricapilla en tres regiones de Texas para cuantificar la atención y las visitas al nido durante los estadios de incubacion y pichón. Las hembras gastaron 80% más de tiempo en el nido durante la incubación y 250% más de tiempo en el nido durante el estadio de pichón que los machos, pero las visitas fueron similares para ambos sexos. En general, el comportamiento del nido no parece estar afectado por las características de la vegetación, a excepción de la atención de los machos durante la incubación, que aumentó a medida que la cobertura media incrementó de 0 a 2 m. La actividad de los adultos no pareció aumentar el riesgo de depredación del nido. A pesar de que las visitas son más frecuentes durante el estadio de pichón que durante la incubación, las visitas durante cada estadio no afectaron la supervivencia del nido. En general, la supervivencia del nido mejoró con la atención durante la incubación.
Introduction
Parental care is a reproductive strategy used by many taxa, including fish, birds, and mammals. Over 90% of bird species provide some form of parental care (Kendeigh 1952). Parental care can be separated into distinct categories including nest building, incubation, and feeding young. In many species, males participate mainly in feeding young, though in some they help build nests and bring food to incubating females (Erhlich et al. 1988, Barg et al. 2006). In the family Vireonidae, males participate in incubation, sharing duties with the female during the day (Erhlich et al. 1988, Grzybowski 2001). Many studies investigating avian parental care have related variables such as ambient temperature (Martin and Ghalambor 1999, Conway and Martin 2000, Londono et al. 2008), food availability (Eikenaar et al. 2003, Londono et al. 2008), and predation risk (Martin and Ghalambor 1999, Ghalambor and Martin 2002) to rates of incubation and feeding young, though most have focused on species in which the female alone incubates.
Skutch (1949) proposed that nest predation increases with activity at the nest. His hypothesis assumes that predation occurs during the day or that predators remember the location of nests and return to depredate them at night. As a means of defense against predators, adults may adjust the amount of time spent on and off of the nest during incubation (Conway and Martin 2000, Martin et al. 2000, Fontaine and Martin 2006). Adults may also adjust the rates at which they feed (i.e., the frequency with which males feed incubating females or both adults feed nestlings; Ghalambor and Martin 2002, Fontaine and Martin 2006) to avoid attracting attention when predators are present (Mullin and Cooper 1998, Conway and Martin 2000, Martin et al. 2000, Ghalambor and Martin 2002, Fontaine and Martin 2006, Eggers et al. 2008). Adults may also increase their rates of predator-defense behaviors to compensate for poor nest location (Cresswell 1997, Komdeur and Kats 1999, Weidinger 2002, Remes 2005, Eggers et al. 2008), depending on the species (Weidinger 2002).
The Black-capped Vireo (Vireo atricapilla) listed as endangered by the U.S. Fish and Wildlife Service, is currently known to breed mostly in central and southwestern Texas (Graber 1961, Ratzlaff 1987, Grzybowski 1995, Wilkins et al. 2006). Other studies have shown snakes to be major predators of Black-capped Vireo nests (Stake and Cimprich 2003; see also Conkling et al. 2012), and snakes may be using the adult vireos' activity to locate nests (Weatherhead and Blouin-Demers 2004, Stake et al. 2005). Similarly, more exposed nests (e.g., poorly concealed or near edges) may also attract the attention of visual predators (Martin 1993, Benson et al. 2010). Therefore, we predicted that vireos should adjust their behaviors at the nest to minimize predation risk corresponding to the nest's level of exposure. Specifically, we predicted that attentiveness to the nest (minutes on nest per hour) should increase and that visitation (trips to nest per hour) should decrease as cover around the nest decreased. We also predicted that increases in attentiveness and decreases in visitation should be good predictors of survival of Black-capped Vireo nests.
Methods
Study Area
Our sampling frame encompassed three study regions from central to southwestern Texas. In central Texas, study areas were on private properties in Coryell County (2008–2009) and at Balcones Canyonlands National Wildlife Refuge in Burnet, Williamson, and Travis counties (2010). The study area in south-central Texas was at Kerr Wildlife Management Area and nearby private lands (2008–2010) in Kerr County. Devils River State Natural Area and Dolan Falls Preserve in Val Verde County were the study areas in southwestern Texas (2009–2010).
The study regions' topography and vegetation varied, from desert scrub in southwestern Texas to rocky limestone hillsides and mesas in central Texas. Common vegetation across all regions includes oaks (e.g., Quercus sinuata, Q. fusiformis, and Q. buckleyi), Ashe juniper (Juniperus ashei), deciduous trees other than oaks (e.g., Ulmus crassifolia, Diospyros texana, Sophora secundiflora), prickly pear (Opuntia spp.), and greenbrier (Smilax spp.). Management varied by study area but included prescribed burning, cattle grazing, hunting of native and exotic animals, wildlife viewing, other human recreation, and Brown-headed Cowbird (Molothrus ater) trapping.
Data Collection
Nest monitoring. Beginning in late March, we located Black-capped Vireo territories by surveying study areas for singing males, visiting each territory every 3–4 days. We used a GPS unit (Garmin Ltd., Olathe, KS) to mark 3–6 Black-capped Vireo locations (e.g., singing perches) per visit to a territory until we marked at least 15 locations for each territorial male, enough to provide a good representation of each territory (International Bird Census Committee 1970). From early April to mid-July, we searched each of the monitored territories for nests every 3–5 days, spending no longer than 1 hr in a territory per visit as stipulated in our federal permit. In general, we used behavioral cues from adults to locate nests, but we also located nests opportunistically. Once we located a nest, we checked its status every 2–4 days until the nest failed or fledged young. Although we placed cameras at nests, we often could not determine nest contents from the camera view. Therefore, we also used a nest mirror, binoculars, or direct observation to determine the contents of the nest, using the method that caused the least disturbance to the nest and nearby vegetation. We addled any Brown-headed Cowbird eggs in the nest to prevent hatching and removed Brown-headed Cowbird nestlings found in the nest.
Nest-camera observations. We used a continuously recording video-camera system to observe adults' behavior, identify predators, and confirm nest fate. We placed cameras only on nests at which incubation had been initiated (i.e., once the penultimate egg was laid) to avoid nest abandonment, as required by our federal permit. Because we wanted to be able to record as much behavior at the nest as possible, when placing cameras we preferentially chose nests earlier in the nesting cycle (e.g., day 2 of incubation vs. day 12).
We used weatherproof bullet cameras with a 3.6-mm lens and infrared lighting (Rainbow, Costa Mesa, CA) to record activity at the nest 24 hr a day. We placed video cameras near enough to nests to capture all activities but far enough away so as not disturb the birds (approximately 1–2 m). We connected camera units to digital video recorders (Detection Dynamics, Austin, TX) and 12—V, 26 A-hr batteries (Batteries Plus, Hartland, WI) with 15-m cables. In 2009 and 2010, we supplemented battery power with 20-watt solar panels (Suntech, San Francisco, CA). We used 4-GB (2008) or 8-GB (2009–2010) SD memory cards and a time-lapsed recording of 5 frames per second to maximize data storage. We checked the camera systems every 3–4 days to replace SD cards and batteries as needed and left the cameras in place until the young fledged or the nest failed.
If we observed a loss of nest contents (i.e., eggs or nestlings) between consecutive nest checks, we viewed all nest video footage recorded during that interval to confirm nest fate and identify predators (when possible). Parasitism by cowbirds (23% of nests; n = 172) occurred before cameras were placed, and we did not consider parasitism to be an instance of predation. In 2008, we made 24 hr of behavioral observations for every third day of footage recorded to determine the number of trips to the nest and number of minutes spent on the nest per day. Initial analyses indicated that two 4-hr sampling periods best duplicated activity recorded during daylight hours. We used this sampling strategy in 2009 and 2010, beginning with the first full day of video recorded, then every fourth day until the nest fledged young or failed. We randomly selected start times for each of the sampling periods with a random-number table of possible hours of daylight. We did not record activity at night because the female generally incubates or broods overnight. If the random number selected for the second sampling period that day would cause the sampling periods to overlap, we selected the next random number that yielded no overlap. Therefore, we observed 8 hr of daytime activity at the nest for each day sampled, resulting in 1–7 days of footage per nest. Because we used different methods in 2008, in this paper we present only behavioral observations from 2009 and 2010.
For each video segment, we determined nest attentiveness by calculating the min hr-1 an adult was present during the observation period and determined visitation by calculating the total trips hr-1, with a trip being each time an adult arrived at or exited from the nest (i.e., each visit comprises two trips). We used trips as a metric instead of visits because sometimes adults were already at a nest at the beginning of observation or remained on the nest at the end of an observation. We also used trips because we assumed that each trip to and from the nest might attract the attention of predators. We did not calculate nest attentiveness and visits by sex because we could not always distinguish individual adults on the video. Because we sampled nest activities in two 4-hr segments, we calculated the mean of the segments to determine the value of nest attentiveness and visits for each day of video observed. We then grouped observations by nest stage, taking the average for all of the days watched at a nest during each stage.
Direct nest observations. Because of specific research objectives, nest observations were limited to study areas in Kerr County. Each week, we randomly selected nests for observation from all of the active nests being monitored. Observing between sunrise and 13:00 CDT, we recorded the stage of the nest (incubation or nestling) for each observation, then stood or sat ≥10 m from the nest, hidden by vegetation so as not to disturb the adults, but still able to view the nest. We used binoculars or a spotting scope to observe the focal pair at the nest for 1 hr, recording all visits to the nest.
For each observation, we determined nest attentiveness for each sex by calculating min hr-1 the male or female was at the nest during the observation period, as well as the total min hr-1 either adult was present. We also determined visits to the nest by calculating trips hr-1 for each adult by sex and total trips hr-1. We grouped observations by incubation or nestling stage. To reduce the effect of pseudoreplication (Hurlbert 1984), we calculated the mean for nest attentiveness and visitation if we observed a nest more than once during a stage.
Vegetation measurements. We measured vegetation at all nests at which we made observations or placed video cameras. Most Black-capped Vireo nests are placed at heights of 0.5 to 2.0 m in the vegetation (Grzybowski 1995), so we assessed percent cover at the nests with a 2-m coverboard. We stood approximately 7 m from the nest in each cardinal direction to estimate the percent cover at each height class (0.1-m intervals) between 0 and 2 m (Guthery et al. 1981). We averaged percent cover from each direction separately, then combined averages from all directions to estimate average cover at the nest from 0 to 2 m (average cover).
To calculate the percent cover within a 25-m radius of each nest (percent cover) we created a buffer around each nest point in ArcGIS 9.3 (Environmental System Research Institute, Redlands, CA). Using National Agriculture Imagery Program orthoimagery (resolution 1 m) encompassing the study area (Seamless Data Warehouse, U.S. Geological Survey), we categorized each cell as cover or no cover, then divided the number of cells classified as cover by the total number of cells in the buffer area.
Statistical Analyses
Nest-video observations. Using SPSS 15.0 (SPSS, Inc., Chicago, IL), we performed multiple regression (Zar 1999:419– 421) to determine if study region, year, average cover, and percent cover affected nest attentiveness and visitation. We used dummy coding for categorical variables with >2 levels (e.g., study region). We did not use a repeated-measures design because we did not observe all nests during both stages. We analyzed each nest stage separately to maintain independence for each nest with observations during both stages.
We ran nest-survival analyses in program MARK (White and Burnham 1999) to determine if adults' behavior influenced nest survival. We analyzed nest survival during the incubation and nestling stages separately because some nests lacked information for both stages (e.g., nest failure during incubation, footage lost because of camera malfunction). We tested a set of candidate models that included various combinations of covariates describing nest attentiveness, visitation, clutch size, year, study region, and cover, in addition to the constant-survival model. Initially, each model included the intercept and one covariate as a main effect. Other candidate models included each behavior covariate plus clutch size. If the main-effect models had values of Akaike's information criterion corrected for small sample sizes (AICc) larger than the intercept-only model, we concluded that the covariate did not influence nest survival and removed it from further consideration. If any of the main-effect models had a value of AICc smaller than that of the intercept-only model, we included the covariate in additional candidate models that included the intercept and all possible combinations of the selected covariates, then evaluated support for each model with AICc. We averaged models and examined coefficients and 95% confidence intervals for significance of effects. We did not include a goodness-of-fit test because our intent was to evaluate variables of interest for possible effects on nest survival, not to predict Black-capped Vireo nest survival on the basis of our best-supported models.
Mean nest attentiveness and visitation ( ± SD) during the incubation and nestling stages recorded from video recorded at Black-capped Vireo nests in Burnet, Coryell, Kerr, Travis, Val Verde, and Williamson counties, Texas, 2009–2010, and during direct observations at nests in Kerr County, 2008–2010.
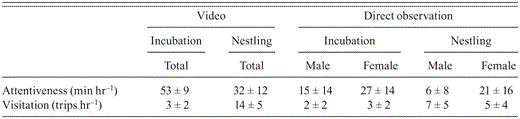
Mean nest attentiveness and visitation ( ± SD) during the incubation and nestling stages recorded from video recorded at Black-capped Vireo nests in Burnet, Coryell, Kerr, Travis, Val Verde, and Williamson counties, Texas, 2009–2010, and during direct observations at nests in Kerr County, 2008–2010.
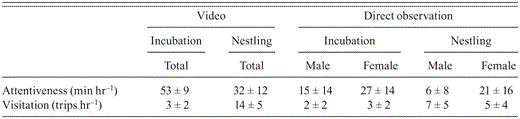
Direct nest observations. We analyzed data from direct and video observations of nests separately because we wanted to be able to investigate differences in parental effort by sex. We used the same methods as above to determine if nest attentiveness or visitation affected nest survival, and to determine factors affecting nest attentiveness and visitation, except we separated behavior covariates by sex and did not include study region in the analyses.
Results of multiple regression analysis evaluating effects of cover, study region, and year on Black-capped Vireo behavior from video recorded in Burnet, Coryell, Kerr, Travis, Val Verde, and Williamson counties, Texas, 2009–2010.
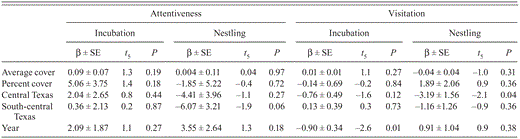
Results of multiple regression analysis evaluating effects of cover, study region, and year on Black-capped Vireo behavior from video recorded in Burnet, Coryell, Kerr, Travis, Val Verde, and Williamson counties, Texas, 2009–2010.
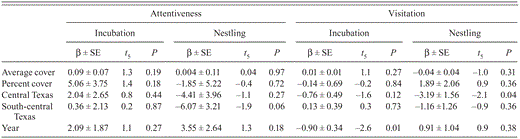
Results
Nest Video Observations
We recorded 63 cases of predation from video recorded at 172 nests, 2008–2010. Avian predators (37%, n = 23) and snakes (37%, n = 23) were the most common predators observed. The Brown-headed Cowbird (n = 11), Western Scrub-Jay (Aphelocoma californica, n = 7), and Greater Roadrunner (Geococcyx californianus, n = 3) were the most common avian predators. We observed all three of these species removing nestlings from nests (see also Stake and Cavanagh 2001). Other predators were ants (n = 5), hawks (n = 2), gray fox (Urocyon einereoargenteus, n = 2), eastern fox squirrel (Sciurus niger, n = 2), coyote (Canis latrans, n = 1), ringtail (Bassariscus astutus, n = 1), bobcat (Lynx rufus, n = 1), northern raccoon (Procyon lotor, n = 1), and greater arid-land katydid (Neobarrettia spinosa, n = 1; see Smith et al. 2012 for details of this event).
Video recorded at 122 nests in 2009 and 2010 yielded 410 days of behavioral observations (Table 1). Average cover, percent cover, year, and study region did not affect nest attentiveness during either nest stage (Table 2). Average cover, percent cover, and study region did not affect visitation during incubation, but visitation was less in 2009 than in 2010 (Table 2). Average cover, percent cover, and year did not affect visitation during the nestling stage, though visitation was less in central than in southwestern Texas (Table 2).
The best-supported models for nest survival based on behavior during each stage included nest attentiveness as a covariate (Table 3), which suggests that nest attentiveness during the incubation and nestling stages appears to affect survival of Black-capped Vireo nests. Nest survival increased as nest attentiveness during incubation increased; in contrast, nest survival decreased as nest attentiveness during the nestling stage increased (Table 4; Fig. 1). Inclusion of year as a covariate did not significantly improve models' support over that of models that included nest attentiveness during the nestling stage as a covariate (ΔAICc ≤ 2), even though these models had more support than the intercept-only model (Table 3).
Model-selection results from analyses of survival of Black-capped Vireo nests from video recorded in Burnet, Coryell, Kerr, Travis, Val Verde, and Williamson counties, Texas, 2009–2010. Models are ranked from most supported (ΔAICc = 0) to least supported; K is the number of parameters in each model. The Akaike weight (wi) is the weight of the evidence for model i, given the data. The model likelihood indicates the support of the model, given the data.
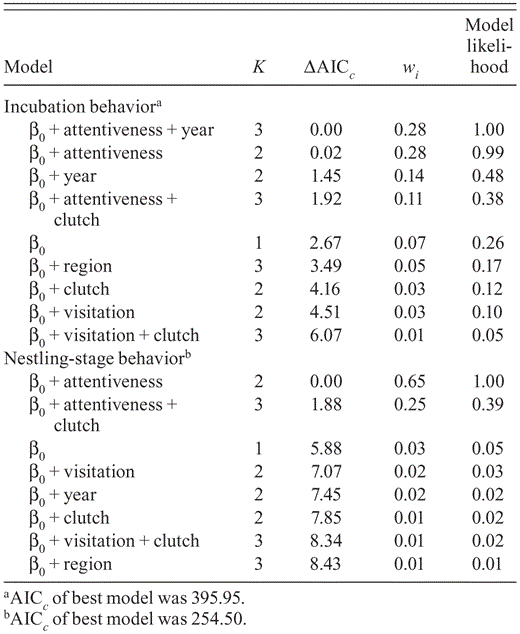
Model-selection results from analyses of survival of Black-capped Vireo nests from video recorded in Burnet, Coryell, Kerr, Travis, Val Verde, and Williamson counties, Texas, 2009–2010. Models are ranked from most supported (ΔAICc = 0) to least supported; K is the number of parameters in each model. The Akaike weight (wi) is the weight of the evidence for model i, given the data. The model likelihood indicates the support of the model, given the data.
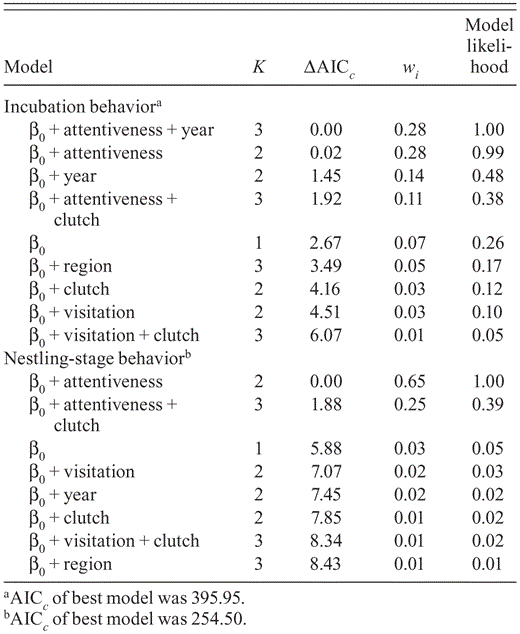
Direct Nest Observations
We made 37 observations at 19 nests in 2008,53 observations at 36 nests in 2009, and 30 observations at 22 nests in 2010. We observed 67 nests during incubation and 53 during the nestling stage. During incubation, females spent 80% more time on nests than did males, and during the nestling stage they spent 250% more time on nests than did males, but visitation by both sexes was similar (Table 1).
None of the variables we examined affected females' nest attentiveness during either nest stage (Table 5). Males' attentiveness during either nest stage was unaffected by year or percent cover (Table 5). Although males' attentiveness during the nestling stage was not affected by average cover, during incubation it increased as average cover increased (Table 5). None of the variables affected males' or females' visitation during either stage (Table 5).
Model-averaged parameter estimates, standard error, and 95% confidence intervals from top models (ΔAICc ≤ 2) from analyses of survival of Black-capped Vireo nests based on video recorded in Burnet, Coryell, Kerr, Travis, Val Verde, and Williamson counties, Texas, 2009–2010 and direct observations at nests in Kerr County, Texas, 2008–2010.
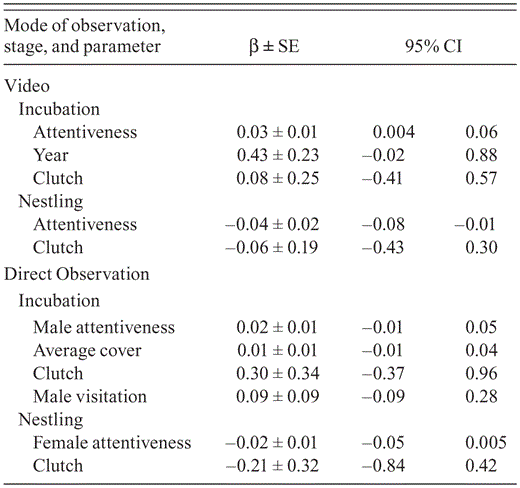
Model-averaged parameter estimates, standard error, and 95% confidence intervals from top models (ΔAICc ≤ 2) from analyses of survival of Black-capped Vireo nests based on video recorded in Burnet, Coryell, Kerr, Travis, Val Verde, and Williamson counties, Texas, 2009–2010 and direct observations at nests in Kerr County, Texas, 2008–2010.
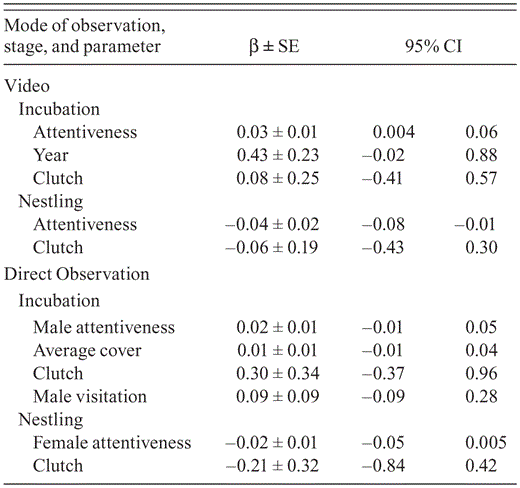
Nest attentiveness during the incubation and nestling stages may affect survival of Black-capped Vireo nests because the best-supported models for nest survival based on behavior during each stage included nest attentiveness as a covariate (Table 6). Although the trends were for nest survival to decrease as the female's attentiveness during the nestling stage increased and nest survival to increase as the male's attentiveness during incubation increased (Table 4), these trends were not significant because the 95% confidence intervals overlapped zero. Models that included average cover as a covariate did not have more support than the intercept-only model even though males' attentiveness during incubation increased with average cover (Table 6).
Discussion
The Skutch hypothesis (1949) suggests the risk of nest predation should increase as adults' activity at the nest increases. Our results do not support the Skutch hypothesis because visitation did not have a negative relationship with survival of Black-capped Vireo nests, despite visitation being higher during the nestling stage than during incubation. Support for the Skutch hypothesis has been ambiguous, with some studies finding that the adults' activity increases the risk of nest predation (Martin et al. 2000, Muchai and du Plessis 2005), others finding no relationship (Roper and Goldstein 1997, Weidinger 2002, Schaefer et al. 2005, Fontaine et al. 2007, Chalfoun and Martin 2010).
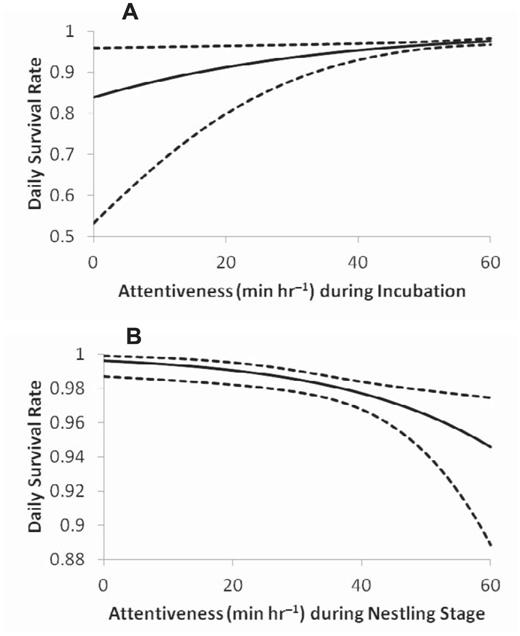
Predicted daily survival rate in relation to nest attentiveness with 95% confidence intervals, based on parameter estimates from model “Α0 + attentiveness” for both the (A) incubation and (B) nestling stages from video observations at Black-capped Vireo nests in Burnet, Coryell, Kerr, Travis, Val Verde, and Williamson counties, Texas, 2009–2010.
Results of multiple-regression analysis evaluating effects of cover, study region, and year on Black-capped Vireo behavior from direct observations at nests in Kerr County, Texas, 2008–2010.
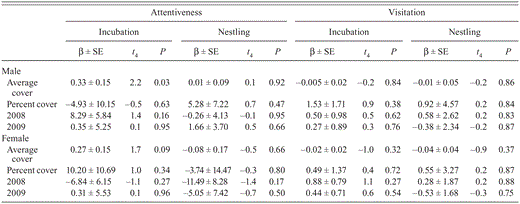
Results of multiple-regression analysis evaluating effects of cover, study region, and year on Black-capped Vireo behavior from direct observations at nests in Kerr County, Texas, 2008–2010.
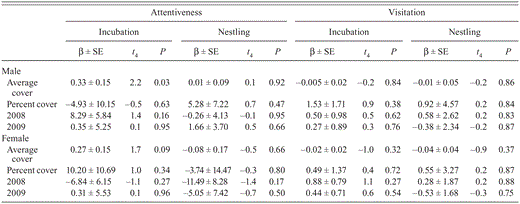
The Skutch hypothesis assumes that activity at the nest attracts the attention of predators. Skutch developed this hypothesis in the neotropics, where diurnal snakes are a major nest predator (Skutch 1985, Libsch et al. 2008), and assuming diurnal predators use visual cues to locate prey. We found that the major predators of Black-capped Vireo nests were birds and snakes, followed by mammals. All cases of predation by birds occurred during daylight, whereas most of those by snakes and mammals occurred during twilight or at night when the vireos provide no active behavioral cues to assist predators in locating nests. Yet snakes may identify nest locations during daylight and return to depredate nests at night when adults are sleeping or not present. Given the diversity of predators of Black-capped Vireo nests, it is likely that vireos are not using vegetation characteristics (e.g., cover) to select nest sites as a means of predator defense because nest sites that provide cover from all categories of predator are difficult to locate (Halupka and Greenley 2009).
In general, Black-capped Vireos' behavior at their nests was likely unaffected by the vegetation characteristics we considered. Males' nest attentiveness during incubation increased, however, as average cover increased. From results of previous studies (Remes 2005, Eggers et al. 2008), we expected longer incubation shifts at more exposed nests where adults may be trying to avoid detection by visual predators. If that were the case, then visitation should be lower at exposed nests as adults would not switch incubation duties as often, which we did not observe. Adults visited nests less often during the nestling stage in central than in southwestern Texas. Most visits to nests are to carry food to nestlings, so regional differences in visitation may result from differences in clutch size or food availability. However, clutch sizes did not differ by study region, and we can only speculate about the nature of food availability, as we did not measure food resources during our study.
Model-selection results from analyses of survival of Black-capped Vireo nests for direct observations at nests in Kerr County, Texas, 2008–2010. Models are ranked from most supported (ΔAICc. = 0) to least supported; K is the number of parameters in each model. The Akaike weight (wi.) is the weight of the evidence for model i, given the data. The model likelihood indicates the support of the model, given the data.
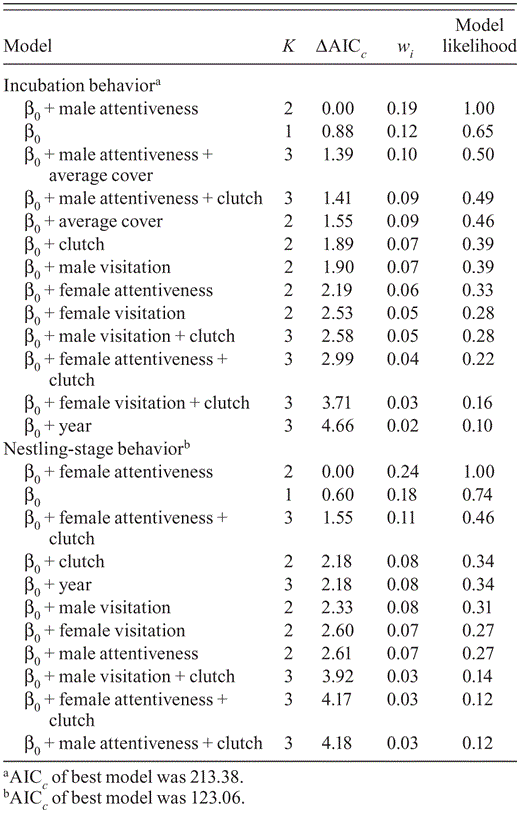
Model-selection results from analyses of survival of Black-capped Vireo nests for direct observations at nests in Kerr County, Texas, 2008–2010. Models are ranked from most supported (ΔAICc. = 0) to least supported; K is the number of parameters in each model. The Akaike weight (wi.) is the weight of the evidence for model i, given the data. The model likelihood indicates the support of the model, given the data.
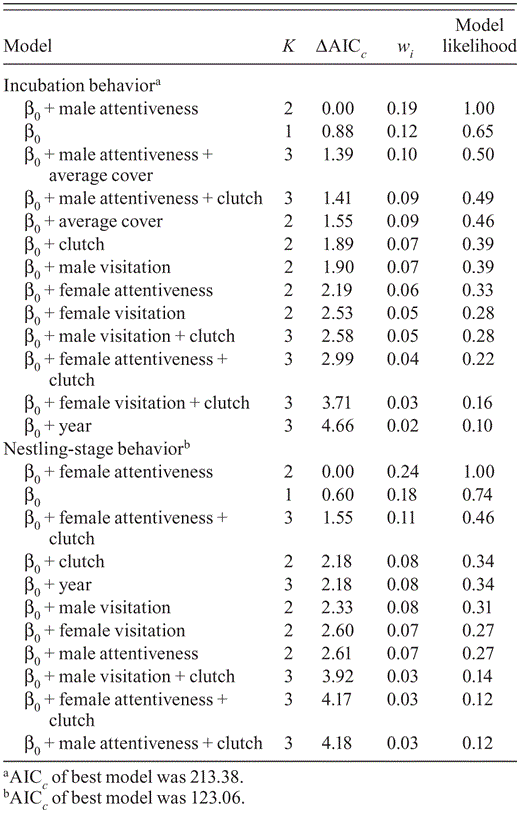
Although vegetation characteristics or potential risk of nest predation did not appear to influence adults' activity at Black-capped Vireo nests, adults' behavior may influence nest survival. Nest attentiveness during the nestling stage appearing to affect nest survival negatively may be the result of observations made only during the early nestling period. Because we averaged females' and total nest attentiveness for all observations during the nestling stage, attentiveness at nests failing early in the nestling stage would be greater because the only observations were made while females were still brooding young nestlings. Nest attentiveness during incubation also affected nest survival, with increased attentiveness improving survival. Nest attentiveness can be a form of nest defense, by hiding eggs or nestlings, camouflaging nests, or discouraging predators (Montgomerie and Weatherhead 1988, Martin 1992, Weidinger 2002, Schaefer et al. 2005), leading to improved survival. Nest survival may improve if males are more attentive during incubation because sharing a larger proportion of incubation duties and bringing food to the incubating female likely improved the female's body condition. As adults in good condition can invest more energy into reproduction (Ricklefs 1977), these results emphasize the importance of the male's participation in determining the outcome of nests of species with biparental care.
For many bird species, predation is the leading cause of nest failure (Nice 1957, Ricklefs 1969). Strategies for protecting nests from predation include placing nests where least exposed to discovery by predators, actively defending nests, and adjusting behavior at nests (Weidinger 2002, Remes 2005, Eggers et al. 2008). For species inhabiting areas with a diverse suite of nest predators, managing for vegetation characteristics at potential nest sites that limit exposure to all types of predators will be difficult; therefore, future research should focus on evaluating what vegetation characteristics provide the best resources for foraging and provisioning young. As habitat loss continues to affect many species, it will be important to protect and maintain habitat that provides these resources to ensure successful reproduction, a component necessary for population persistence.
Acknowledgments
We thank the Texas Parks and Wildlife Department, The Nature Conservancy, U.S. Fish and Wildlife Service, and all of the private landowners who allowed access to their properties for providing housing and/or access to field sites. We thank the Institute of Renewable Natural Resources for logistical support, the numerous technicians that provided assistance in the field, and all of the graduate students and employees of the Institute of Renewable Natural Resources that watched hours of video. F. Thompson, A. Cox, A. Rodewald, and L. Kearns provided technical support and advice regarding nest cameras. B. Collier provided advice for statistical analyses. A. Aispuro translated the abstract into Spanish. The U.S. Army Integrated Training Area Management Program, Office of the Secretary of Defense, Department of Defense, Texas Department of Transportation, Texas Parks & Wildlife Department, and a Tom Slick Senior Graduate Fellowship provided funding for our research.
Literature Cited