-
PDF
- Split View
-
Views
-
Cite
Cite
Mark Williamson, Joseph B. Williams, Erica Nol, Laboratory Metabolism of Incubating Semipalmated Plovers, The Condor: Ornithological Applications, Volume 108, Issue 4, 1 November 2006, Pages 966–970, https://doi.org/10.1093/condor/108.4.966
- Share Icon Share
Abstract
The Semipalmated Plover (Charadriussemipalmatus), anarctic-nesting migratory shorebird, regularlyencounters low temperatures during the breedingseason. We measured the basal metabolism of adultsduring incubation at Churchill, Manitoba, Canada todetermine basal metabolic rate (BMR),lower critical temperature(Tlc), total evaporative waterloss (TEWL), and dry thermal conductance(Cm). BMR and Tlcwere 47.4 kJ day−1and 23.3°C, respectively, TEWL was2.5 mL H2O−d,and Cm was1.13 mW g−1 °C−1.Measured BMR and Tlc were consistentwith high values found for other shorebird speciesbreeding in the Arctic, while Cm was18% higher than predicted from allometricequations. These metabolic data suggest thatSemipalmated Plovers are adapted to balance therequirements of incubation against energetic andthermoregulatory demands in the Arctic, especiallyin harsh early breeding season conditions.
Metabolismo de Incubación de Charadrius semipalmatus en Condiciones de Laboratorio
Charadrius semipalmatus es un ave playera migratoria que anida en el ártico y está regularmente expuesta a bajas temperaturas durante le época reproductiva. Medimos el metabolismo basal de individuos adultos en Churchill, Manitoba, Canadá, para determinar el índice de metabolismo basal (IMB), la temperatura crítica baja (Tlc), la pérdida total de agua por evaporación (PTAE) y la conducción térmica seca (Cm). El IMB fue 47.4 kJ día−1, la Tlc fue 23.3°C, la PTAE fue 2.5 mL H2O−d y la Cm fue 1.13 mW g−1 °C−1. Los valores medidos de IMB y Tlc fueron consistentes con los altos valores registrados para otras especies de aves playeras que se reproducen en el ártico, mientras que la Cm fue 18% más alta que la predicha por ecuaciones alométricas. Esta información metabólica sugiere que C. semipalmatus está adaptado a balancear los requerimientos de incubación en función de las demandas energéticas y de termo-regulación en el ártico, especialmente durante condiciones adversas al inicio de la época reproductiva.
Shorebirds (Charadriiformes) migrate long distances each spring to breed in the Arctic, an environment of low ambient temperatures, strong winds, and high relative humidity, which in concert lead to high thermoregulatory demands. Several studies support the hypothesis that shorebirds (mostly Scolopacidae) have evolved physiological adaptations to cope with an energetically demanding way of life, including relatively high field metabolic rates and basal metabolic rates (BMR) relative to their body size (Kersten and Piersma 1987, Castro et al. 1992, Piersma and Morrison 1994, Lindström 1997, Piersma and Baker 2000, Kvist and Lindström 2001) and seasonal hypertrophy of metabolically active organs such as the liver, heart, and intestines (Piersma et al. 1991, Weber and Piersma 1996). However, relatively little is known about the metabolism of adult Charadridae, especially during the breeding season (Piersma et al. 2003).
Semipalmated Plovers (Charadrius semipalmatus) breed in the Canadian Arctic at latitudes up to 73°N, and begin nesting while snow remains on the ground, typically encountering high winds and low temperatures while incubating on exposed nests (Nol and Blanken 1999). Thus, thermoregulatory demands are high during incubation. To increase our understanding of the energetics of Semipalmated Plovers during the breeding season, we measured their laboratory metabolism and total evaporative water loss during incubation. We also calculated their dry thermal conductance to gain understanding of their nonevaporative heat loss during a time when energy balance may be critical. We predicted that, overall, Semipalmated Plovers would exhibit a metabolic pattern indicative of adaptation to the rigors of incubation in a cold climate.
Methods
Using walk-in traps, we captured eight incubating Semipalmated Plovers in early June near Churchill, Manitoba (58°44′N, 94°07′W) and transported them to our laboratory at the Churchill Northern Studies Centre. To measure oxygen consumption (VO2) and total evaporative water loss (TEWL, the sum of respiratory and cutaneous water loss), we constructed a stainless steel, water-jacketed metabolic chamber with a volume of 5 L, painted with a flat black interior to reduce reflected radiation (Porter 1969). Temperature within the chamber was controlled (±0.1°C) by a Neslab (model RTE-140, Thermo Neslab, Inc., Newington, New Hampshire) water bath and measured with a 36-gauge copper-constantan thermocouple. A rubber gasket rendered the lid airtight. During measurements, birds were placed in the chamber on wire mesh above mineral oil to prevent feces from contributing to evaporative water.
An air compressor pushed air through drying columns, filled with Drierite® (CaSO4, W. A. Hammond Drierite Co. Ltd., Xenia, Ohio) and Ascarite (NaOH-vermiculite), to remove atmospheric water vapor. Air was then directed through a mass flow controller (3 L min−1 maximum; Tylan model FC-260, Entegris, Inc., Minneapolis, Minnesota), calibrated against a 5 L bubblemeter (Levy 1964), and finally into the metabolic chamber. We maintained flow rate at 1093 ml min−1, so that oxygen concentration in the chamber did not fall below 20.5%. Excurrent air passed through Teflon tubing to a dewpoint hygrometer (General Eastern model Hygro M4, General Electric Corp., Fairfield, Connecticut), calibrated against a National Institute of Standards and Technology benchmark, allowing measurement of TEWL. Air was then subsampled for analysis of oxygen concentration and consumption by birds using an Applied Electrochemistry (Pittsburgh, Pennsylvania, model S-3AII) O2 analyzer, calibrated with dry outside air free of CO2. Voltage and thermocouple outputs were monitored continuously with a Campbell data logger (model CX-10, Campbell Scientific, Inc., Logan, Utah).
Beginning around 19:00, we maintained a subject bird for 2 hr at 30°C to allow it to become accustomed to the chamber. During the third hour we recorded oxygen consumption. Traces of O2 consumption were nearly flat in the third hour of measurement, but we took the lowest 15-min average during the hour as the bird's basal metabolic rate (BMR). Thereafter, we decreased chamber temperature in 5°C increments, from 30°C down to −10°C, and remeasured O2 consumption after the bird had experienced each temperature for 1 hr. One of the birds remained active during our measurement of BMR, as indicated by variation in the trace of oxygen concentration, and was subsequently released. Thus, we measured BMR and the response to decreasing temperature of seven postabsorptive, incubating individuals (five males, two females). All birds were weighed prior to placing them into the chamber.
At the conclusion of the trials all birds were reweighed and released in the immediate vicinity of their nests. In all but one case the remaining mate was in attendance at the nest site when the captured bird was returned.
Statistical Analysis and Calculations






Results
Predicted and measured BMR, TEWL, Tlc, and Cm are based on a mean body mass of 42.0 ± 2.1 g for the seven individuals used in this study. The average mass-specific basal metabolic rate (BMR) for Semipalmated Plovers was 47.4 kJ day−1. This value is within 5% of Kersten and Piersma's (1987) predicted value for similar-sized shorebirds at temperatures below Tlc (49.8 kJ day−1). TEWL for Semipalmated Plovers was 2.5 ml H20 day−1, within 11% of the value of 2.8 ml H20 day−1 predicted by Williams (1996). Losses from body wastes were not included in the equation for TEWL and are relatively insignificant, especially in smaller birds (Dawson 1982, Williams 1996).
Tlc was calculated as 23.3°C. Metabolic rate increased linearly below Tlc as ambient temperature (Ta) decreased (r2 = 0.67, P = 0.05). A comparison of our results with the allometrically derived equation of Root (1988) for estimating Tlc from body mass yielded a predicted value of 19.9°C, 15% less than the value we measured. All seven individuals that we tested responded similarly to the drop in temperature with no apparent outliers to this pattern (Fig. 1). Dry thermal conductance (Cm) was measured at 1.13 mW g−1 °C−1, 18% greater than the allometric prediction (0.93 mW g−1 °C−1) given by Aschoff (1981).
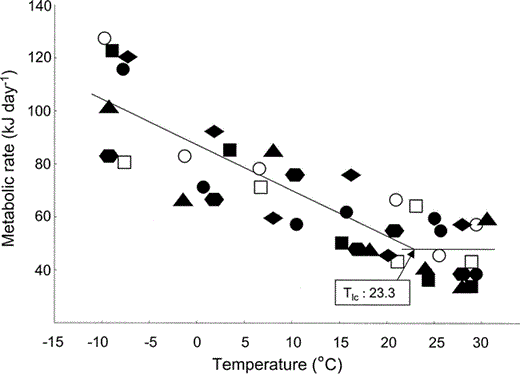
Scholander model indicating relationship of ambient temperature to metabolic rate for seven incubating Semipalmated Plovers under postabsorptive, resting conditions.
Males: filled symbols, females: unfilled symbols. Metabolic rate measured as kJ day−1. Tlc = lower critical temperature.
Discussion
Our measurements of Semipalmated Plover metabolism reveal a pattern of elevated BMR, which appears characteristic for arctic-nesting migratory shorebirds (Kersten and Piersma 1987, Piersma et al. 1991, 1995, Castro et al. 1992). For example, reported values of BMR for members of the Charadridae and Scolopacidae range from 24% (European Oystercatcher [Haematophus ostralegus]) to 50% (Grey Plover [Pluvialis squatarola]) higher than allometric predictions (Kersten and Piersma 1987). BMR values of Semiplamated Plovers are consistent with those obtained for other migratory shorebirds during incubation, i.e., a BMR significantly elevated above what would be expected from allometric predictions for other nonpasserines and in comparative agreement with shorebird-specific predictions.
Williams' (1996) phylogenetic survey of TEWL accurately predicts the reduced importance of evaporative cooling in arctic-nesting species such as Semipalmated Plovers. Although heat loss attributable to TEWL is a significant factor in arid environments, in cold climates such as the Canadian Arctic, evaporative water loss is largely recoverable through condensation of water from expired air in cold nasal passages and metabolic water production, and is therefore not considered a driving metabolic factor (Gill 1995, Williams 1996).
Estimates of lower critical temperature on the basis of BMR or body mass alone (Root 1988) did not accurately predict the elevated Tlc values found in Semipalmated Plovers, inviting further investigation. For example, are relatively high lower critical temperatures of arctic-nesting shorebirds an adaptive consequence of elevated basal metabolic rates, or merely evidence of origin in a mesic environment? Other migratory species with lower critical temperatures similar to those of shorebirds may breed 15–30° (up to 3000 km) farther south and typically experience much smaller Tlc–Ta gradients (and therefore less hypothermal stress below the lower critical temperature) than are typically encountered by birds breeding in the Arctic (Yarborough 1971, Root 1988).
We expected that feather insulation would be high in arctic-breeding birds and therefore that dry thermal conductance would be lower than allometric predictions for Semipalmated Plovers, however we found the reverse to be true. Relative measures such as feather density and body fat percentage were not recorded, but elevated Tlc values do not suggest a high degree of insulation for this species during breeding. To date, no studies of daily energy expenditure for free-living Semipalmated Plovers have been completed. Piersma et al. (2003) recorded high daily energy expenditure levels in other shorebird species, and it would be of interest to investigate whether Semipalmated Plovers use increased levels of metabolic heat production to overcome their relatively high thermal conductance.
There is strong evidence that BMR is plastic and can be modified seasonally by shorebirds when dealing with changing energetic requirements. Considerable work has been done on seasonal and latitudinal variation in the BMR of Red Knots (Calidris canutus) and other shorebirds (Piersma et al. 1991, 1995, Weber and Piersma 1996, Kersten et al. 1998, Kvist and Lindstrom 2001, Piersma 2002, Lindström and Klaassen 2003). Other metabolic efficiencies can be gained through seasonal hypertrophy or atrophy of muscles and organs to improve assimilative or thermogenic capacities (Piersma et al. 1996, Piersma 2002) and there is considerable interest in determining the limits to upper metabolic activity and the constraints it places upon species (summarized by Piersma 2002).
Overall, the temperature difference that a bird can maintain between body (Tb) and ambient (Ta) temperature is a function of thermogenesis, conductance, and TEWL (Calder and King 1974), and can provide the metabolic margin for a successful breeding season or even survival in harsh conditions. Elevated BMR and Tlc in Semipalmated Plovers, in combination with low TEWL and an ability to balance Cm with metabolic heat production, provide a plausible mechanism for the tolerance of this species to the physiological costs of steep and prolonged Tb–Ta gradients during incubation in the Arctic.
Acknowledgments
We thank the Churchill Northern Studies Centre for use of their facilities in Churchill, Manitoba, Canada. This study was carried out under permits from Environment Canada and the Canadian Wildlife Service, and supported by Northern Scientific Training Program grants from Trent University awarded to MW, a National Sciences and Engineering Research Council (Canada) operating grant to EN, and National Science Foundation grants awarded to R. E. Ricklefs and JBW. The authors also wish to thank Theunis Piersma, Magda Havas, and an anonymous reviewer for their constructive comments on the manuscript.
Literature Cited
Author notes
E-mail: [email protected]