-
PDF
- Split View
-
Views
-
Cite
Cite
Brian A. Cooper, Martin G. Raphael, M. Zachariah Peery, Trends in Radar-Based Counts of Marbled Murrelets on the Olympic Peninsula, Washington, 1996–2004, The Condor: Ornithological Applications, Volume 108, Issue 4, 1 November 2006, Pages 936–947, https://doi.org/10.1093/condor/108.4.936
- Share Icon Share
Abstract
The Marbled Murrelet (Brachyramphusmarmoratus) is a highprofile, federally threatened seabird, but noreliable estimates of population trends at inlandbreeding areas exist for this species. We conductedland-based radar studies of Marbled Murrelets at3–7 sites on the Olympic Peninsula,Washington, in 1996–2002 and 2004 to estimatepopulation changes and to examine relationshipsbetween our counts and oceanographic conditions,murrelet productivity, and regional at-sea countsof murrelets. Morning radar counts of murreletsvaried significantly among and within sites but didnot decline from 1996–2004, suggesting thatthe inland breeding population of murrelets isstable in this area. A retrospective power analysisindicated that we had a 25% and 56%chance of detecting 2% and 4% annualdeclines, respectively. Thus, if relatively smallannual declines did occur during our study period,there is a high probability that they would havegone undetected, even though they could add up to abiologically important decline over time. It isunlikely that murrelets on the Olympic Peninsuladeclined by ≥6% annually, however,because retrospective analyses indicated that powerto detect such declines was >88%. Therewas no significant relationship between radarcounts and at-sea counts or productivity ofmurrelets in the nearby San Juan Islands during thestudy period. We also did not detect a relationshipbetween radar counts and mean sea-surfacetemperatures or the Northern Oscillation Index,suggesting that variation in oceanographicconditions (e.g., the strong 1998 ElNiño event) was not associated withvariation in morning radar counts of MarbledMurrelets. A prospective power analysis indicatedthat small (2%–4%)annual declines could be detected with reasonablyhigh power (≥80%) with thecurrent radar sampling design by extending thestudy to 11–15 years.
Tendencias en los Conteos por Radar deBrachyramphusMarmoratus en Olympic Peninsula,Washington, Entre 1996 Y 2004
Brachyramphusmarmoratus es un ave marinadestacada que se encuentra amenazada a nivelfederal. A pesar de esto, no existen estimacionesconfiables sobre las tendencias poblacionales deesta especie en las áreas reproductivascontinentales. Realizamos estudios deB.marmoratus con radares ubicadosen el continente en 3 a 7 sitios de OlympicPeninsula, Washington, entre 1996 y 2002, y en2004, para estimar los cambios poblacionales y paraexaminar las relaciones entre nuestros conteos ylas condiciones oceanográficas, laproductividad de B.marmoratus y los conteos marinosregionales de esta especie. Los conteos por radarmatutinos variaron significativamente entre ydentro de los sitios, pero no disminuyeron entre1996 y 2004, sugiriendo que las poblaciónreproductiva continental de B.marmoratus permanece estable enesta área. Un análisis retrospectivode poder indicó que tuvimos una probabilidaddel 25% y del 56% de detectardisminuciones anuales del 2% y 4%,respectivamente. De este modo, si se presentarondisminuciones anuales relativamente menores durantenuestro periodo de estudio, existe una altaprobabilidad de que no fueran detectadas, inclusoaunque su efecto se hubiera acumulado hasta tenerconsecuencias biológicas importantes. Sinembargo, es poco probable que B.marmoratus haya disminuidoanualmente en un 6% o más en OlympicPeninsula, debido a que los análisisretrospectivos indicaron que el poder para detectarestas disminuciones fue mayor al 88%. Nohubo una relación significativa de losconteos por radar con los conteos en el mar o conla productividad de B.marmoratus en las vecinas Islasde San Juan durante el periodo de estudio. Tampocodetectamos una relación entre los conteospor radar con la temperatura media superficial delmar, ni con el Índice de Oscilacióndel Norte, sugiriendo que la variación enlas condiciones oceanográficas (e.g.,el fuerte evento de El Niño de 1998) noestuvo asociada con la variación en losconteos. Un análisis retrospectivo de poderindicó que pequeñas disminucionesanuales (2%–4%)podrían ser detectadas con un poderrazonablemente alto (≥80%) conel diseño actual de muestreo por radar,mediante la extensión del estudio por 11 a15 años más.
Introduction
The Marbled Murrelet (Brachyramphus marmoratus) is protected as a threatened species in both the United States and Canada. There is ongoing interest in the status of the species, as exemplified by the recent five-year status review requested by the U.S. Fish and Wildlife Service (McShane et al. 2004, U.S. Fish and Wildlife Service 2004, Huff et al. 2006). The primary reasons for conservation concern are: 1) most Marbled Murrelets nest on limbs of large trees, most often in larger patches of old-growth forest, and the amount of this habitat has declined due to logging and development; 2) the species is detrimentally affected by forest fragmentation, which can lead to increased nest predation; 3) Marbled Murrelets have a low recruitment rate, so populations remain stable only when adult survival rates are high; 4) Marbled Murrelets forage in nearshore marine habitat that is close to human development and prone to oil spills and other marine pollution; 5) there is the potential for bycatch of these murrelets in nearshore net-based fisheries; and 6) overfishing may be affecting the quality and quantity of prey available to murrelets (U.S. Fish and Wildlife Service 1997, Raphael, Evans Mack et al. 2002, Raphael et al. 2006, Becker and Beissinger 2006). Demographic modeling suggests that the Washington population of Marbled Murrelets is declining at ~3% per year (Beissinger and Nur 1997, McShane et al. 2004).
To test predictions from these modeling efforts and to estimate population trend and size, researchers began coordinated vessel-based surveys in 2000 throughout the range of the murrelet in Washington, Oregon, and California (Miller et al. 2006). Although these data are valuable, they do not provide trend information for specific inland breeding areas, which are often far from foraging areas (Whitworth et al. 2000). The main reason there is so little information available on trends or population sizes in specific inland breeding areas is that murrelets have very cryptic inland behavior (e.g., nesting in large trees and visiting nests primarily during periods of low ambient light levels; Nelson 1997) that severely hinders visual observations of birds at inland locations. Further, audiovisual surveys (Evans Mack et al. 2003) were not designed to provide an index of abundance and the high variation in audiovisual counts would require a massive survey effort to detect population trends (Jodice et al. 2001, Smith and Harke 2001, Bigger et al. 2006). Recent studies have shown, however, that radar can be used successfully and with less effort to obtain information on inland flight behavior and the abundance of Marbled Murrelets (Hamer et al. 1995, Burger 1997, 2001, Cooper et al. 2001, Cooper and Blaha 2002, Raphael et al. 2002, Burger et al. 2004). In addition, radar methods are being proposed to monitor regional murrelet population trends in British Columbia, Canada. This sort of effort requires information on within and among site and year variation so that sampling designs can be effective. In this study, we used radar to monitor local populations of murrelets on the Olympic Peninsula, Washington, during 1996–2004. We also examined sources and extent of variability in radar counts and used these data to estimate required survey effort to detect varying levels of population decline.
Recent evidence suggests that changes in ocean conditions, such as those that occur as the result of the El Niño–Southern Oscillation (ENSO) and the Pacific Decadal Oscillation (PDO), affect the distribution, abundance, and reproduction of seabirds (Ainley et al. 1994, Oedekoven et al. 2001). Strong ENSO events (such as the 1998 event) result in a reversal of the flow of the California Current System, the presence of a surface layer of warm, nutrient-depleted water, and the replacement of coastal upwelling with downwelling (Hunt 1995). A consequence of these events is a marked reduction in primary production, followed by a reduction in the abundance of some fishes and zooplankton that are an important food source for seabirds. Because productivity in areas like Puget Sound and the Strait of Juan de Fuca (Fig. 1) is primarily driven by tides, there is less expectation for ENSO, PDO, and related events to have a similar impact (Hunt 1995). However, the oceanic variation associated with ENSO events has been linked to changes in diet, productivity, survival, and distribution of Marbled Murrelets along the Pacific coast (Ainley et al. 1995, Becker 2001, Becker and Beissinger 2003, Peery et al. 2006; Becker et al., in press) and has been associated with widespread reproductive failure in several species of seabirds in the northeastern Pacific (Hodder and Graybill 1985, Ainley and Boekelheide 1990, Wilson 1991). It is possible that fewer Marbled Murrelets fly inland when there is widespread nesting failure in a particular year, especially if the failure occurs before the nestling period in July. Further, there is evidence indicating that nonbreeding murrelets in central California rarely fly inland during the breeding season. This suggests that lower radar-based counts should occur during years of poor breeding effort and that they are essentially indices of the breeding effort in that area (Peery et al. 2004, Bigger et al. 2006). In this paper, we examine relationships among our counts, oceanographic conditions, murrelet productivity, and regional at-sea counts of Marbled Murrelets to determine whether radar counts were driven by regional population size or breeding effort mediated by oceanographic conditions.
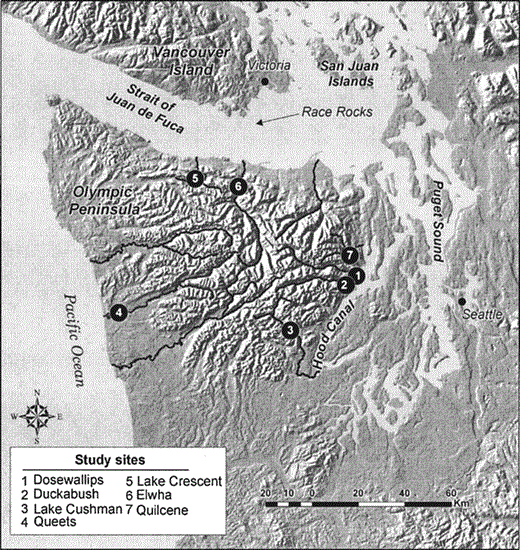
Locations of sampling sites used for radar observations of Marbled Murrelets on the Olympic Peninsula, Washington, 1996–2004.
Methods
Study Area
Our surveys were conducted in seven of the major river valleys of the Olympic Peninsula, Washington, near large (4–40 km2) blocks of low-elevation, old-growth coniferous forest (Fig. 1). The area we studied is fairly unusual in that most (93%) of the habitat inland from the radar sites is conserved in wilderness areas or Olympic National Park, and thus did not have any large habitat changes due to timber management over the course of our study (Raphael et al. 2006). All but one of the drainages sampled (Queets) were deeply incised glacial valleys surrounded by peaks 1000–2000 m asl. In contrast, the Queets site was in a low valley between rolling hills of the coastal plain.
Data Collection
We used radar to survey Marbled Murrelets entering river drainages 1–4 times per year, usually on consecutive days, from late June through late July. Three of the seven drainages (Quilcene, Dosewallips, and Duckabush) were sampled in eight years (1996–2002 and 2004), and the remaining four drainages (Cushman, Queets, Crescent, and Elwha) were sampled in six years (1998–2002 and 2004). Radar sampling occurred from ~105 min before to ~75 min after sunrise, based on previous studies that identified peak flights into forests near sunrise (Cooper et al. 2001). Sampling was not conducted if rain interfered with radar transmission or when headwinds or tailwinds exceeded 25 km hr−1. Radar site selection, equipment, and sampling methods are detailed in Cooper et al. (2001). In summary, we used a 10–12 kW marine radar transmitting at 9.410 GHz (X-band), mounted on a truck or van. The radar was operated at the 1.5 km range, with the pulse length set at 0.07 µsec and the forward edge of the antenna elevated ~10–15°. The effective radar beam sampling area for murrelets (Fig. 2) was measured by conducting field tests with similarly sized (~200 g) Rock Pigeons (Columba livia) suspended from weather balloons at various heights and distances up to 1.5 km away.
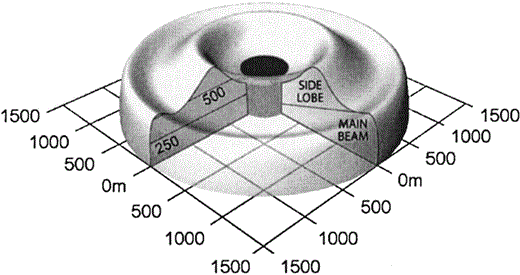
Approximate murrelet-sampling airspace for the Furuno FR–1510 marine radar at the 1.5 km range setting.
Note that the configuration of the radar beam within 250 m of the origin (i.e., the darkened area) was not determined.
During each session, we videotaped the radar screen, and the operator stood outside and recorded visual observations of Marbled Murrelets and other species whose flight characteristics on radar could be confused with those of murrelets. Weather conditions were recorded at the beginning of each session and as they changed during a session. Based on previous studies that compared flight speeds of Marbled Murrelets and other species (Cooper et al. 2001 and references therein), only radar targets flying at >64 km hr−1 were counted as murrelets, excluding those that visually or auditorily were identified as other species.
Statistical Analyses
For all analyses, we classified targets as flying “landward” or “seaward” if they were flying within 60° of the valley's long axis in a landward (i.e., inbound) or seaward (outbound) direction, respectively, and classified targets that were not flying in a landward or seaward direction as “other.” We used the mean number of landward-flying radar targets as our murrelet count each year (Cooper et al. 2001).
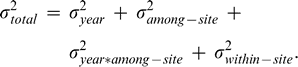
Following Franklin et al. (2004), we used a two-stage approach when analyzing radar counts. First, we used restricted maximum likelihood techniques with a model that included all fixed effects (linear year, SST, NOI, productivity, and density) to estimate random effects. This full fixed-effects model was executed with two competing covariance structures, compound symmetric and first-order autoregressive (Littell et al. 1996), to assess the correlation of radar counts within sites across years. The most appropriate covariance structure was selected with Akaike weights (Burnham and Anderson 2002), where only covariance parameters were used in the calculation of second-order Akaike's information criterion (AICc) because restricted maximum likelihood ignores fixed effects (Littell et al. 1996). Once the best covariance structure was selected, the second stage estimated and tested for the significance of the fixed effects using full maximum likelihood and F-tests. We used a one-sided F-test to test the null hypothesis that the population was not declining (Ho: b ≥ 0; Ha: b < 0) because: (1) the question of interest from a management perspective is whether the population declined, and (2) power to detect a decline is greater for one- than two-sided tests for a given critical value.
We estimated two coefficients of variation for the variance component associated with each random effect as described by Bigger et al. (2006). The first coefficient of variation (CVpop) was estimated as , where
was the mean natural logarithm of radar counts and provided an estimate of the intrinsic variability in counts at that level. CVest was estimated as SE(σ ^2)/σ ^2, where SE(σ ^2) was the standard error of the variance component (σ ^2) and provided a measure of how well we estimated the variance component given our sampling design and sample size. We tested the null hypothesis that each variance component was equal to zero with Z-tests (Zar 1999).
For comparisons of radar counts and sea-surface temperatures (SSTs), we used temperatures recorded at Race Rocks, located south of Victoria, British Columbia, in the Strait of Juan de Fuca (Fig. 1). A strong correlation has been found between SSTs at Race Rocks and SSTs along the outer coast of Washington (Wilson 2005). Monthly mean SSTs at Race Rocks were obtained from the Ocean Science and Productivity Division of Fisheries and Oceans Canada (available at 〈http://www-sci.pac.dfo-mpo.gc.ca/osap/data/lighthouse/raceroct.txt). We also compared our radar counts with the Northern Oscillation Index (NOI; available at 〈www.pfeg.noaa.gov). The NOI is an index of oceanographic variation that is based on the difference in sea level pressure between the northeastern Pacific and Darwin, Australia (Schwing et al. 2002). The NOI is dominated by interannual variation associated with El Niño and La Niña events and usually has high negative values when there are El Niño conditions along the west coast of North America. The NOI is thought to be a better index of environmental variability in the northeastern Pacific than the Southern Oscillation Index (Schwing et al. 2002). Following Becker et al. (in press), we used the mean values of the first four months of each year to compute both SSTs and the NOI, as this time is the most important for the onset of reproduction in seabirds due to effects of upwelling on prey abundance (Ainley et al. 1996a, 1996b).
We also compared our radar counts with productivity and at-sea densities of Marbled Murrelets near the San Juan Islands during 1996–2004. The productivity index was calculated from juvenile:adult ratios adjusted for time of year of survey, following methods of Beissinger (1995) and Peery et al. (in press). Specifically, counts of juveniles were adjusted upward by the proportion of young that would have fledged by the end of the survey period, based on a cumulative frequency of fledging dates from Washington and British Columbia (Hamer and Nelson 1995; Desolation Sound Marbled Murrelet Study, Simon Fraser University, unpubl. data). We calculated a separate adjustment factor using regression for each of three time periods within the survey season (Evans Mack et al. 2004). Murrelet densities were obtained from at-sea surveys conducted near the San Juan Islands in July of each year (Evans Mack et al. 2004; MGR, unpubl. data).
Power to detect declines in radar counts was estimated using Monte Carlo simulations as outlined by Bigger et al. (2006). We first conducted a retrospective analysis, in which we estimated statistical power to detect annual declines ranging from 2% to 10% (in 2% increments) given sample size (number of sites and surveys per site) and the number of years in which surveys were conducted in this study. We then conducted a prospective analysis in which we estimated the number of years it would take to detect 2%–10% declines with 80% power. For all power analyses, we used one-sided tests of the null hypothesis that the population was unvarying over the study period with a critical value of 0.05. All summary statistics are reported as mean ± SE, unless noted otherwise.
Results
We conducted 148 radar counts from 1996 to 2004. Mean radar counts varied widely among sampling sites within each year (Fig. 3A). The mean of these mean annual site counts was fairly stable, however, when data were combined among sites each year, for both the sites with eight years of count data and the sites with six years of data (Fig. 3B).
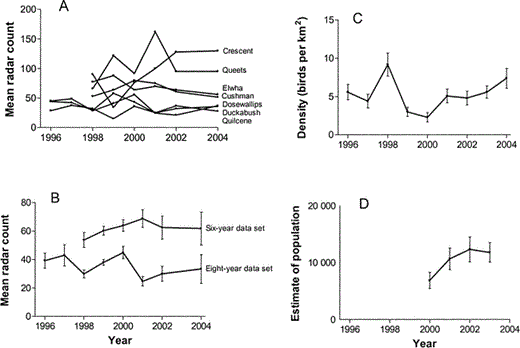
(A) We observed high among-site variation in mean annual radar counts of landward-flying targets in the Olympic Peninsula, Washington, but (B) low among-year variation in mean (± SE) radar counts of landward-flying targets.
The three drainages (Quilcene, Dosewallips, and Duckabush) sampled during all eight years of our study are the “eight-year data set.” These three sites, along with the four sites that were sampled during the last six years of the study (Cushman, Queets, Crescent, and Elwha sites) are the “six-year data set.” (C) Radar counts were not significantly related to annual densities (± SE) of Marbled Murrelets observed during at-sea surveys in the San Juan Islands during 1996–2004. (D) In contrast to the lack of year effects in radar counts, at-sea estimates of murrelet numbers (± SE) in the waters surrounding the Olympic Peninsula increased by ~71% between 2000 and 2003 (Miller et al. 2006).
Based on Akaike weights, the mixed model with autoregressive covariance structure fit the count data slightly better (1.1 times) than the model with compound symmetric covariance structure. Therefore, all tests of fixed and random effects were based on the autoregressive covariance structure. This autoregressive covariance structure indicated a significant correlation in radar counts within sites across years (covariance parameter = 988 ± 0.03; Z = 30.2, P < 0.01), and a decline in this correlation as counts were separated by an increasing number of years. Variance among sites (σ2site) accounted for most (56%) of the total variance in radar counts and σ2site was significantly greater than zero (Table 1). Variance within sites (σ2within-site) accounted for most of the remaining variation (37%) and σ2within-site was also significantly greater than zero. Little variation in counts occurred among years or due to the interaction between year and site (Table 1). Although significant variation in counts occurred among and within sites, the magnitude of this variation was modest (CVpop = 0.10–0.12). Within-site variance (σ2within-site) was well estimated (CVest = 0.14), but more uncertainty existed in our estimate of among-site variance (σ2site; CVest = 0.60), probably because only seven sites were sampled.
Variation among sites and within sites accounted for most of the variance (σ ^2) in the number of Marbled Murrelets detected during radar surveys from 1996 to 2004 on the Olympic Peninsula, Washington. 95% confidence limits are in parentheses following variance component estimates. CVpop is the coefficient of variation describing the intrinsic variability in murrelet counts due to a specific source of variation and CVest describes how well the variance component was estimated in this study.
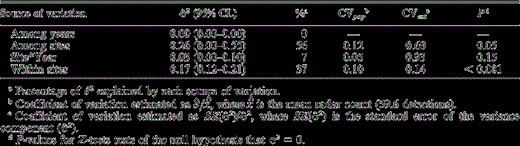
Variation among sites and within sites accounted for most of the variance (σ ^2) in the number of Marbled Murrelets detected during radar surveys from 1996 to 2004 on the Olympic Peninsula, Washington. 95% confidence limits are in parentheses following variance component estimates. CVpop is the coefficient of variation describing the intrinsic variability in murrelet counts due to a specific source of variation and CVest describes how well the variance component was estimated in this study.
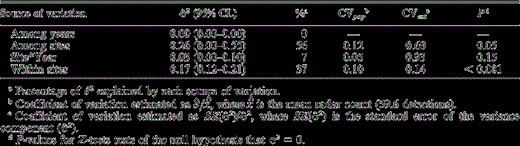
The slope associated with the linear year term (b = −0.03 ± 0.04) was not significantly less than zero (F1,99 = 0.6, P = 0.44), indicating that radar counts did not decline over the study period (Fig. 3A, 3B). Radar counts also were not significantly related to SST (F1,99 = 0.3, P = 0.57), the NOI (F1,99 = 1.0, P = 0.32), murrelet productivity (F1,99 = 0.2, P = 0.69), or at-sea density of murrelets (F1,99 = 0.1, P = 0.81; Fig. 3C).
We qualitatively compared our radar counts to estimates of murrelet populations in all waters surrounding the Olympic Peninsula (i.e., in Puget Sound, the Strait of Juan de Fuca, and along the outer Washington coast) for all years these data were available (i.e., 2000–2003; Miller et al. 2006). In contrast to the lack of year effects in radar counts and in densities of murrelets in the San Juan Islands, at-sea estimates of murrelets in the waters surrounding the Olympic Peninsula increased by ~71% between 2000 and 2003 (Fig. 3D). Most of this increase in at-sea counts was due to increases along the outer Washington coast; counts in Puget Sound and the Strait of Juan de Fuca were more constant over this period.
A retrospective power analysis indicated that power to detect 2% and 4% annual declines in our radar data was low (25% and 56%, respectively), but power to detect ≥6% annual declines was ≥88% (Table 2). The prospective power analysis indicated that ≥80% power to detect 2% and 4% declines could be achieved by extending the study to 15 and 11 years, respectively.
Estimates of statistical power and years required to detect population declines using radar counts of Marbled Murrelets on the Olympic Peninsula, Washington. Both parameters were estimated with a critical value of 0.05 and assuming the sample size (number of sites and surveys per site) used in this study. Power to detect declines in counts was estimated assuming an eight-year study period, as in this study (i.e., a retrospective analysis). Years to detect declines in counts was the number of years a study would need to be implemented to detect a decline with 80% power (i.e., a prospective analysis).
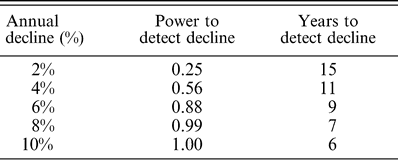
Estimates of statistical power and years required to detect population declines using radar counts of Marbled Murrelets on the Olympic Peninsula, Washington. Both parameters were estimated with a critical value of 0.05 and assuming the sample size (number of sites and surveys per site) used in this study. Power to detect declines in counts was estimated assuming an eight-year study period, as in this study (i.e., a retrospective analysis). Years to detect declines in counts was the number of years a study would need to be implemented to detect a decline with 80% power (i.e., a prospective analysis).
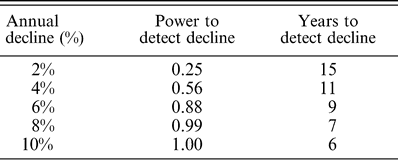
Discussion
Trends in Radar and At-sea Counts
We did not detect any significant differences among years in our radar counts on the Olympic Peninsula during 1996–2004. At-sea counts from the San Juan Islands during the same period also did not indicate a population change. Although not statistically significant, at-sea population estimates from the outer coast of Washington suggested a possible increase in murrelet numbers from 2000 to 2003 (Miller et al. 2006). Marine densities of birds in the San Juan Islands also increased during 2000 through 2003, even though there was no overall change in density between 1996 and 2004 (Evans Mack et al. 2004; MGR, unpubl. data). Thus, the short-term increase in the outer coast population, if real, could be an artifact of annual variation (i.e., an unusually low count in 2000, supported by the low counts in the San Juan Islands in 2000) and not indicative of a true increase in murrelet populations.
It is possible that changes in inland populations of Marbled Murrelets are occurring on the Olympic Peninsula, but that radar sampling was not conducted over a sufficient number of years or with sufficient intensity within years to detect these changes. For example, our retrospective analysis indicated that we had only a 25% and 56% chance of detecting 2% and 4% annual declines, respectively. Demographic models reported by McShane et al. (2004) projected annual declines of ~3% over the next ten years. If this level of annual decline did occur during our study period, there is a high probability that these changes would have gone undetected, even though they could add up to a biologically important decline over time.
There are other reasons why declining trends in our data might be unexpected. For example, there was not a dramatic change in the extent of suitable nesting habitat in our study area because ~93% of nesting habitat inland from our radar sampling stations was protected from cutting and essentially no loss from harvest or fires occurred during the years of our study. In total, habitat loss over the entire study area (including unprotected areas) was only 1.3% over the past ten years. In addition to the low loss of murrelet habitat, there were no major oil spills in the area during our study period. In the past, however, Marbled Murrelets have been killed in several large oil spills in the area, including the 1988 Nestucca spill off northern Washington and Vancouver Island (>145 murrelets killed; Burger 1993) and the 1991 Tenyo Maru spill at the entrance of the Strait of Juan de Fuca (200–400 murrelets killed; Carter and Kuletz 1995). Finally, the limited available data suggest that loss of murrelets to local fisheries bycatch has probably also been low (Carter et al. 1995).
Our work does not support a continuation of major Marbled Murrelet declines as reported previously in Washington, Oregon, and California (Ralph et al. 1995, U.S. Fish and Wildlife Service 1997). One of the few historical data sets available from the vicinity of our study area prior to our study is from at-sea surveys made in Clayoquot Sound in southwestern Vancouver Island in 1982 (Sealy and Carter 1984) that were repeated using similar methods in 1992 and 1993 (Kelson et al. 1995). The latter dataset indicated that there had been a 40% decline in counts in this area from 1982 to 1993, which the authors attributed to loss of old-growth nesting habitat. Old-growth forests have been reduced by ~80% in western Oregon and Washington (U.S. Fish and Wildlife Service 1997). However, Burger (2000) suggested that the lower counts near Vancouver Island could be linked to warm sea surface temperatures, which were considerably higher in the 1990s than in 1982 and which are associated with less suitable foraging conditions for murrelets in that area.
Relationship Between Radar Counts, Oceanographic Conditions, and Murrelet Productivity
Changing ocean temperatures have a profound influence on marine ecosystems of the eastern Pacific Ocean (McGowan et al. 1998). Recent El Niño–Southern Oscillation (ENSO) events have been associated with widespread reproductive failure in several species of seabirds (Vermeer et al. 1979, Hodder and Graybill 1985, Wilson 1991, Ainley et al. 1994) and with changes in the abundance of seabirds (Veit et al. 1996, Oedekoven et al. 2001). However, little is known about ENSO effects on Marbled Murrelets (Burger 2000), except that the availability of murrelet prey and murrelet diets probably are affected by ocean temperatures (Ainley et al. 1995; Becker et al., in press) and murrelet productivity is lower in warm-water years when prey is reduced (Becker et al., in press).
Despite a strong ENSO event in 1998 that produced warm SSTs in the eastern Pacific, we did not detect a relationship between radar counts on the Olympic Peninsula and either SSTs or the NOI. This apparent lack of a relationship between radar counts and SSTs, the NOI, or murrelet productivity suggests that numbers of Marbled Murrelets flying inland on the Olympic Peninsula were not affected by the ENSO events themselves, or by any changes in murrelet productivity that may have been associated with these events. Similarly, Burger (2000) did not find a statistically significant relationship between mean counts of landward-flying radar targets and SSTs on Vancouver Island during 1995–1998, although he noted that sample sizes were too small for rigorous statistical testing.
Our data and data for California (Peery et al. 2004, Bigger et al. 2006) suggest that the effect of oceanographic conditions on radar counts may be greater in California than on the Olympic Peninsula. One explanation for this geographical difference is that ENSO effects tend to diminish farther north (Wooster and Fluharty 1985). Alternatively, ENSO effects in areas like Puget Sound and the Strait of Juan de Fuca might be weaker because foraging opportunities in these areas tend to be driven by tidally generated upwellings, rather than by the current- and wind-driven productivity of open coastlines (Hunt 1995). The upwelling of cold, nutrient-rich waters, which is inhibited by ENSO conditions, is more likely to be an important physical feature determining murrelet foraging opportunities along open coasts than in Puget Sound (Hunt 1995). Tidal processes such as the generation of tidal fronts (which are not affected by ENSO events) are probably more important determinants of foraging opportunities for murrelets in inland waters of sounds and channels (Hunt 1995, Day and Nigro 2000). In support of this explanation, our only site located along the open, outer coast of Washington (Queets) had large interannual variation in counts of murrelets relative to most of the other sites that were located adjacent to protected waters, with the lowest mean count at Queets occurring during 1998 (mean radar count [95% CI] = 66.0 [43.3–88.7] targets in 1998 compared to 113.3 [90.0–136.5] targets in all other years). Similarly, Wilson and Manuwal (1986) suggested that the inner Strait of Juan de Fuca is somewhat protected from oceanic climatic events based on their study of Rhinoceros Auklets (Cerorhinca monocerata). They speculated that El Niño conditions in 1983 were responsible for Rhinoceros Auklets nesting along the outer coast of Washington switching their diet to Pacific Saury (Colobis saira), while auklets nesting in the Strait of Juan de Fuca maintained their normal diet of sandlance (Ammodytes hexapterus).
There are compelling reasons to continue to study and understand the responses of Marbled Murrelets to variation in ocean conditions. In the short term, the effects of ocean temperatures on at-sea- or radar-based counts need to be considered to help us interpret trends in these counts. There are also conservation implications for understanding the effects of ocean warming on Marbled Murrelet populations, given recent evidence for long-term increases in ocean temperatures (Roemmich and McGowan 1995).
Comparison of Radar and At-sea Counts
To date, the most widely used method for the long-term monitoring of Marbled Murrelet populations in the U.S. has been at-sea counts (Miller et al. 2006). We did not find a significant relationship between radar counts and at-sea densities of murrelets, but neither data set had a consistent trend over the short period that we examined. Thus, more years of data are needed to determine if there is a relationship between annual radar counts and at-sea counts. In the meantime, we believe that radar methods provide an independent index of abundance that can be used to help assess population trends detected by at-sea surveys. Further, radar counts can be used to assess trends for particular inland areas, unlike at-sea counts, which are more difficult to link to specific inland areas.
Power to Detect Trends in Radar Counts
We had comparatively good power to detect trends in our radar counts given our sampling design and survey effort. For example, we calculated a reasonable probability (≥80%) of detecting a 2% annual decline in 15 years with ~3 surveys per year at our seven sites. By comparison, Bigger et al. (2006) estimated that four radar surveys at 22 sites per year were needed to detect a 2.5% annual decline in 10 years with 80% power in northern California. This difference is likely due to the lower among-year variation in our radar counts compared to that of Bigger et al. (2006). Although variance components were estimated in exactly the same way in the two studies, we detected no annual variation in radar counts on the Olympic Peninsula, whereas Bigger et al. (2006) attributed ~17% of the total variance in radar counts to annual variation. Given the low among-year variation we observed, we speculate that the higher power to detect trends in this study could have been related to the greater temporal stability of the largely tidally driven productivity in Puget Sound. This highlights the need to consider location-specific oceanographic conditions on the foraging, and thus breeding, opportunities of Marbled Murrelet populations across their range.
Acknowledgments
The USDA Forest Service Pacific Northwest Research Station and the Olympic Natural Resources Center provided funds for this study under the auspices of the Northwest Forest Plan. The study was administered by D. Evans Mack of the USDA Forest Service and J. Calhoun of the Olympic Natural Resources Center. Thanks to S. Speckman for her comments on annual variation in regional oceanographic conditions and murrelets. J. Baldwin provided invaluable statistical advice. We also thank R. J. Blaha, A. A. Stickney, T. J. Mabee, and the many other biologists who have participated in fieldwork since 1996. J. H. Plissner led the field trials for measuring the effective sampling area of the radar. R. J. Blaha assisted with preparation of figures and B. Galleher assisted with habitat map analyses. A previous version of this manuscript was improved by the comments of R. H. Day, P. G. R. Jodice, T. J. Mabee, and an anonymous reviewer.
Literature Cited
Author notes
E-mail: [email protected]