-
PDF
- Split View
-
Views
-
Cite
Cite
Guillermo E. Pérez, Keith A. Hobson, Isotopic Evaluation of Interrupted Molt in Northern Breeding Populations of the Loggerhead Shrike, The Condor: Ornithological Applications, Volume 108, Issue 4, 1 November 2006, Pages 877–886, https://doi.org/10.1093/condor/108.4.877
- Share Icon Share
Abstract
The Loggerhead Shrike (Laniusludovicianus) breedsthroughout North America and various populationsapparently exhibit diverse molt strategies.However, molt in this species and how it may varygeographically is generally poorly known. Weinvestigated molt sequence in 27 breedingLoggerhead Shrikes using stable hydrogen(δD) isotope analysis of flightfeathers. Because feather δD varies with thelatitude at which feathers are grown in NorthAmerica, it is relatively straightforward toidentify those feathers grown south of anindividual's breeding location. We sampled 11feathers per individual to evaluate locations offeather molt between breeding and wintering groundsin North America. Sampling took place in centralSaskatchewan, Canada (n = 18 individuals) andin the southern region of the Saskatchewan-Manitobaborder (n = 9). We found evidencethat shrikes initiated flight feather molt on theirbreeding grounds (P1 and P3) but thenlargely suspended molt until reaching theirwintering areas. The isotopic evidence suggeststhat the first primary (P1, most depleted indeuterium, mean = −103‰) provides information onshrike breeding latitudes while the innermosttertial (S9, most enriched in deuterium, mean = −49‰)provides information on shrike wintering orsouthernmost molting latitudes.
Evaluaciόn Isotopica de la Muda Interrumpida de Poblaciones Norteñas Reproductivas de Lanius ludovicianus
Laniusludovicianus se reproduceextensamente en Norte América y al parecervarias poblaciones exhiben diversas extrategias demuda. Sin embargo, de la muda de esta especie ycomo puede variar geográficamente,generalmente poco se sabe. Investigamos lasecuencia de muda de las plumas de vuelo en 27Laniusludovicianus reproductivosusando análisis de isótopo estable dehidrógeno (δD). Porque δDen plumas varía con la latitud a la cual lasplumas crecen en Norte América, esrelativamente sencillo identificar las plumas de unindividuo que crecieron al sur del sitio dereproducción. Muestreamos 11 tipos de plumaspor individuo para evaluar las ubicaciones de mudade plumas entre los sitios de reproducción einvernales en Norte América. El muestreoocurrió en Saskatchewan central,Canadá, (n = 18 individuos) y enla región sureña de la frontera entreSaskatchewan y Manitoba (n = 9). Encontramosevidencia que Laniusludovicianus iniciaron la mudade plumas de vuelo en sus sitios dereproducción (P1 y P3) perodespués en gran parte la muda es suspendidahasta alcanzar sus sitios invernales. La evidenciaisotópica sugiere que la primera primaria(P1, más agotada en deuterio, promedio = −103‰)nos proporciona información de la latitudede reproducción de Laniusludovicianus, mientras que lapluma terciara más próxima (S9,la más enriquecida en deuterio, promedio = −49‰)nos proporciona información de la latidud delos sitios invernales o el lugar mássureño de muda del Laniusludovicianus.
Introduction
Molt, the seasonal replacement of feathers, is one of the most energy-demanding processes in the annual life cycle of birds (Young 1991, Marks 1993, Howell et al. 1999). For birds that migrate between breeding and wintering grounds, the timing and extent of molt represents an evolutionary trade-off between competing demands for successful reproduction and preparation for migratory flight (Howell et al. 1999, 2003, Leu and Thompson 2002). However, despite its importance as a life history trait, molt receives comparatively little attention (Young 1991, Thompson and Leu 1994, Leu and Thompson 2002). This paucity of information is partly due to the fact that the establishment of molting patterns relies heavily upon museum specimens (Howell et al. 1999) and is inherently biased to those areas where birds are accessible for capture and study, typically on the breeding grounds or at migration stopover sites in Holarctic areas (Thompson and Leu 1994). As a result, information on molt is often incomplete with respect to extent, timing, and location of molt among age and sex classes, and across populations (Young 1991, Leu and Thompson 2002, Siikamäki et al. 1994). Molt is influenced by food availability, age, and sex and can differ within species (Thompson and Leu 1994, Hemborg 1999, Leu and Thompson 2002). In North America, the first and adult prebasic molt for most passerines occurs after the breeding season (whether they breed or not) and usually precedes autumn migration, but may also take place during migration or on the wintering grounds (Pyle 1997, Leu and Thompson 2002). Some species begin molting shortly after the breeding season, suspend or interrupt molt during fall migration, and complete it on the wintering grounds (Pyle 1997, Leu and Thompson 2002).
Shrikes (Laniidae) are a predominantly Old World family that includes species and populations exhibiting diverse molt strategies (Lefranc and Worfolk 1997). Sedentary shrikes tend to undertake a complete molt soon after breeding, while some migratory populations undergo a postbreeding molt in the vicinity of their breeding grounds prior to fall migration (Miller 1928, Yosef 1996). Others may not start molt until after migration (Yosef 1996) and those that begin their molt on the breeding grounds may either continue it during fall migration or suspend molt during migration and resume molting again at their wintering areas (Palmer 1898, Miller 1931, Lefranc and Worfolk 1997). In North America, the Loggerhead Shrike (Lanius ludovicianus) comprises 11 subspecies and is widespread, being found roughly from coast to coast and from southern Canada to the Isthmus of Tehuantepec in Mexico (Miller 1931). Northernmost populations are migratory and winter in the southern U.S. and Mexico (Fig. 1). Information on the molt of this species, especially for these migratory populations, is relatively poor and several possible strategies have been reported or assumed (Yosef 1996).
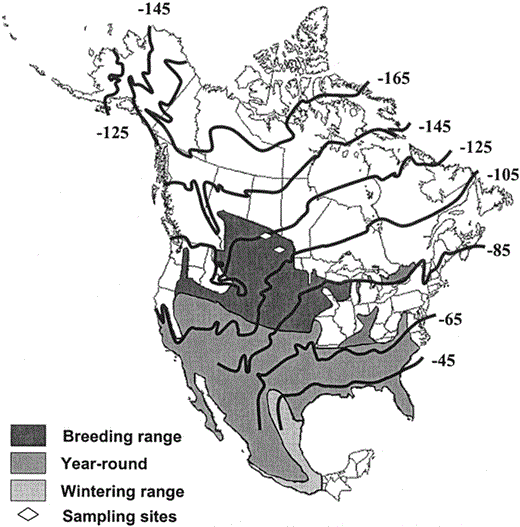
Breeding, wintering, and year-round distribution of the Loggerhead Shrike in North America, and deuterium (δD) contour lines based on a geographical information system-based model of δD values of feathers for the average growing season (Meehan et al. 2004).
We used an isotopic discrimination factor between δD of precipitation (δDp) and δD of feathers (δDf) of −25‰. Diamonds represent the sampling locations in central Saskatchewan and in southern regions of the Saskatchewan-Manitoba border.
Previously, Hobson and Wassenaar (2001) investigated migratory connectivity between breeding and wintering grounds of Loggerhead Shrikes using stable hydrogen isotope analysis of feathers. This approach is based on the fact that a robust latitudinal gradient in growing-season averaged deuterium exists in precipitation in North America and this pattern is reflected in local foodwebs across the continent (Hobson and Wassenaar 1997). Thus, feathers reflect this spatial pattern in deuterium concentrations, making it possible to infer latitudinal locations of molt from isotopic analysis of feathers. This approach has significant advantages over mark-recapture methods, since it does not depend on initial capture and marking of individuals. Rather, identification of molt location is possible for each bird captured (Hobson 1999). Using this technique, Hobson and Wassenaar (2001) were able to identify the relative use of wintering grounds in the southern United States and northern Mexico by populations of northern migratory shrikes. A key assumption of that study was that the outer tail (rectrix) feather that they analyzed was indeed grown on or close to the breeding grounds prior to fall migration. That assumption was based on reports by Miller (1928), but it was not clear to what extent this was true of northern populations. Northern birds may be expected to differ in molt strategy from more southerly birds (Yosef 1996, Pyle 1997). In this study, we investigated molt in northernmost migratory Loggerhead Shrikes by isotopically examining feathers along a sequence of primary, secondary, tertial, and tail feathers. We reasoned that δD values of feathers could be used to assign approximate latitude, making it possible to identify which feathers were grown on the breeding grounds, during fall migration, and on the wintering grounds (Norris et al. 2004).
Methods
Feather Collection
We obtained feather samples from the northernmost population of Loggerhead Shrikes by opportunistically detecting and capturing individuals along highways and grid roads in central and southeastern Saskatchewan and southwestern Manitoba. Birds were captured using a walk-in trap baited with a (protected) live mouse. Sampling took place from 24 June to 6 July 2004. All birds were sexed according to presence or absence of a brood patch and cloacal protuberance. We attempted to age birds to second year (SY) and after-second-year (ASY) based on criteria outlined in Pyle (1997) for this species. However, these techniques were abandoned, since we did not feel we could confidently classify birds in our northern population using these methods. Indeed, as noted by Pyle (1997), during some months within our study period Loggerhead Shrikes can be aged with only 5%–25% accuracy. All birds were marked with a U.S. Fish and Wildlife Service aluminum band. We clipped approximately 1.0 × 0.5 cm sections of the proximal web from remiges and rectrices to minimize effects on flight (these sections overlapped adjacent feathers during flight). We sampled primaries P1, P3, P6, and P9, secondaries S1, S3, and S6, tertial S9, and rectrices R1, R3, and R6. Each feather piece was placed in a separate, labeled paper envelope.
We sampled 27 adult birds and tried to obtain all 11 feather samples from each individual. However, not all eleven samples were always taken from each bird, because occasionally feathers were missing or being replaced. However, in all other cases, the previous year's feather was sampled. Eighteen birds were captured in central Saskatchewan and nine within the putative hybrid zone of SE Saskatchewan and SW Manitoba.
Stable-isotope Analyses
Feather samples were cleaned in 2:1 chloroform:methanol solution overnight, drained, and air dried under a fumehood for at least 3 hr. Feather vane subsamples were then cut and 350 µg ± 10 µg weighed into 4.0 × 3.2 mm silver capsules for online hydrogen isotope analysis by continuous-flow isotope-ratio mass spectrometry (CFIRMS). Stable hydrogen isotope analytical measurements (δD) followed the “comparative equilibration” technique described by Wassenaar and Hobson (2003). Isotopic values were expressed in delta notation in parts per thousand (‰) as the nonexchangeable hydrogen portion of samples normalized on the Vienna Standard Mean Ocean Water–Standard Light Antarctic Precipitation (VSMOW-SLAP) standard scale. Feather samples were analyzed at the stable-isotope facility of the National Water Research Centre in Saskatoon, Canada.
Statistical Analyses
We depicted our feather isotope data graphically using frequency distributions to examine for potential bimodal patterns corresponding to feather growth on breeding and nonbreeding (i.e., en route and wintering) areas. By inspection of the feather δD contour map for North America (Fig. 1), we estimated a cutoff of −90‰ between breeding and nonbreeding areas. Since the original data was non-normal (Fig. 2), we opted to group feathers (P1s, P3s, etc.) and analyze them independently to examine whether molting differed between the sexes. For this we used chi-square and Fisher's exact tests (in 2 × 2 contingency tables, with the smallest expected frequency less than 5; Siegel 1956). We also used chi-square and Fisher's exact tests to test for differences in frequencies of molt within primaries, secondaries (including tertial S9), and rectrices. The Bonferroni correction (Sokal and Rohlf 1995) was used to reduce possible Type I error and alpha was subsequently set at 0.008 for primaries and secondaries and 0.016 for rectrices. Independent sample t-tests were used for parametric data to test whether molting patterns differed between sexes and to test differences in feather isotope values between sampling areas. We were interested in determining the degree to which P1 showed isotopic concordance with the breeding ground signature expected for feathers. Therefore, for our two collection sites, we compared our observed P1 δD values with expected feather deuterium values (δDf) derived from the growing-season, altitudinally corrected continental pattern of Meehan et al. (2004) and corrected for isotopic discrimination between feather and precipitation δD (δDp) by 25‰ (Wassenaar and Hobson 2000). Statistical analyses were performed using SPSS version 12.0 for windows (SPSS 2004). All results are expressed as means ± SD.
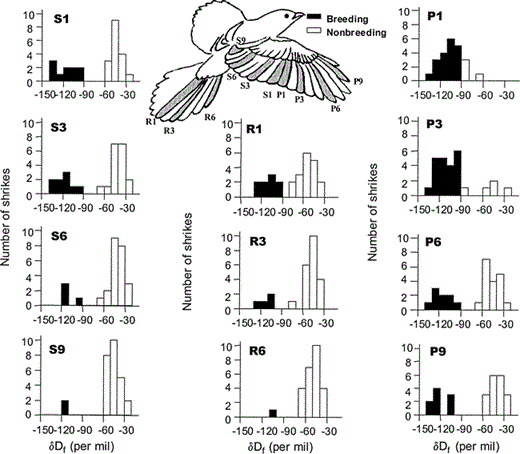
In general, deuterium values of primary (P1, P3, P6, P9), secondary (S1, S3, S6), tertial (S9), and rectrix (R1, R3, R6) feathers of summering Loggerhead Shrikes sampled in Saskatchewan and Manitoba, Canada, revealed a bimodal distribution, indicating that flight-feather molt occurs either on the breeding grounds or at nonbreeding areas.
Shrike sketch was based on Harris and Franklin (2000).
Results
Feathers from individuals showed considerable variation in isotopic structure (Table 1), revealing that they were not all grown on the breeding grounds (Fig. 1). After assigning feather origins based on their range of δDf values, we found no effect of sex on where feathers were grown (breeding or nonbreeding areas) across feathers (all P > 0.07), so we combined male and female feathers within type for all further analyses. Within primary feathers, we found no difference in distribution of assigned origin between P1 vs. P3 and P6 vs. P9 (Fisher's exact test, P = 0.71 and χ21 < 0.001, P > 0.99, respectively). Comparisons of origin for all other primary feather combinations were significant (all P < 0.001). Comparisons of origin for all secondary feather combinations were nonsignificant after the Bonferroni correction (all P > 0.02). Within rectrices, with the exception of R1 vs. R6 (Fisher's exact test, P = 0.01) all comparisons were nonsignificant (R1 vs. R3, χ21 = 2.1, P = 0.15; R3 vs. R6, Fisher's exact test, P > 0.19). There was difference in δDf between the two sampling areas (t21 = 3.7, P < 0.001). The expected average δDf value in central Saskatchewan was −125‰, whereas our mean observed average was −112‰ (95% CI = −119‰ to −103‰). In the SE Saskatchewan and SW Manitoba area, the expected average δDf value was −112‰, whereas our mean observed average was −91‰ (95% CI = −100‰ to −82‰; Table 1). Subsequent primaries showed increasing likelihood of being grown south of breeding sites.
Summary of deuterium (δD) values of flight feathers from Loggerhead Shrikes sampled in prairie Canada (Fig. 1). All sampled feathers of Loggerhead Shrikes from central Saskatchewan (A) were more depleted in deuterium than feathers of shrikes from SE Saskatchewan–SW Manitoba (B), indicating that, on average, shrikes from site A molted their feathers at more northerly latitudes than conspecifics from site B. P1 refers to the innermost primary feather, P9 is the outermost primary feather, S1 is the outermost secondary feather, S6 is the innermost secondary feather, S9 is the innermost tertial feather, R1 is the innermost rectrix, R6 is the outermost rectrix (Fig. 2), and n is the sample size.
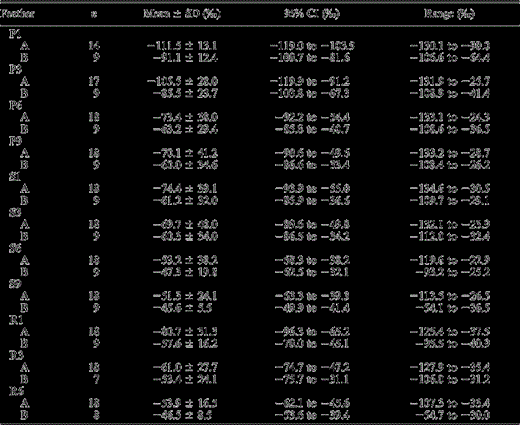
Summary of deuterium (δD) values of flight feathers from Loggerhead Shrikes sampled in prairie Canada (Fig. 1). All sampled feathers of Loggerhead Shrikes from central Saskatchewan (A) were more depleted in deuterium than feathers of shrikes from SE Saskatchewan–SW Manitoba (B), indicating that, on average, shrikes from site A molted their feathers at more northerly latitudes than conspecifics from site B. P1 refers to the innermost primary feather, P9 is the outermost primary feather, S1 is the outermost secondary feather, S6 is the innermost secondary feather, S9 is the innermost tertial feather, R1 is the innermost rectrix, R6 is the outermost rectrix (Fig. 2), and n is the sample size.
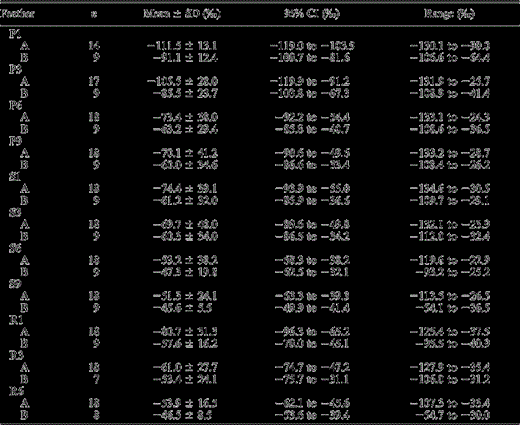
In general, feather groups (primaries, secondaries, tertial S9, and rectrices) showed a bimodal distribution, indicating they were grown either on the breeding grounds or on nonbreeding areas (Fig. 1, 2). Thus, in this population, molt appears to be initiated on the breeding grounds and then suspended pending arrival on the wintering (or more southern molting) grounds. The main segregation among individuals occurred with the degree of primary molt prior to migration (Table 2).
Distribution of feather molt for each individual Loggerhead Shrike sampled according to the isotopic criteria: breeding grounds (less than −90‰), or nonbreeding areas (greater than −90‰). Feathers P1, P3, P6, and P9 refer to primary feathers 1, 3, 6, and 9. Feathers S1, S3, and S6 refer to secondary feathers 1, 3, and 6. Feather S9 refers to the innermost tertial feather. Feathers R1, R3, and R6 refer to rectrices 1, 3, and 6 (Fig. 2). Adult Loggerhead Shrikes initiated their flight-feather molt with P1 on the breeding grounds, but molt was not completed prior to fall migration. Following some partial primary replacement, molt was completed on the wintering or more southerly molting grounds.
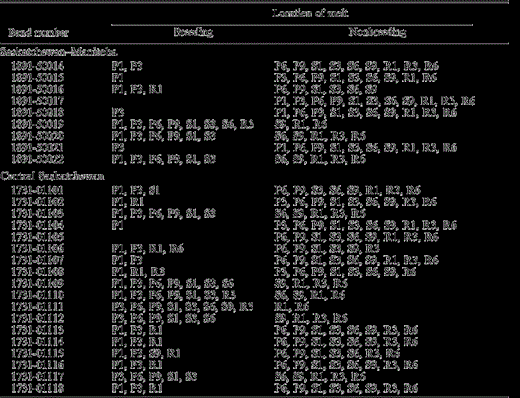
Distribution of feather molt for each individual Loggerhead Shrike sampled according to the isotopic criteria: breeding grounds (less than −90‰), or nonbreeding areas (greater than −90‰). Feathers P1, P3, P6, and P9 refer to primary feathers 1, 3, 6, and 9. Feathers S1, S3, and S6 refer to secondary feathers 1, 3, and 6. Feather S9 refers to the innermost tertial feather. Feathers R1, R3, and R6 refer to rectrices 1, 3, and 6 (Fig. 2). Adult Loggerhead Shrikes initiated their flight-feather molt with P1 on the breeding grounds, but molt was not completed prior to fall migration. Following some partial primary replacement, molt was completed on the wintering or more southerly molting grounds.
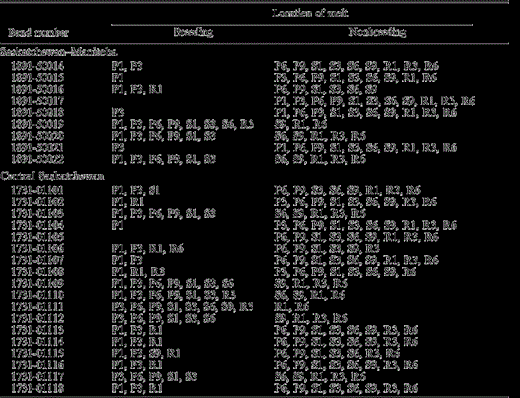
Discussion
Our isotopic analysis of feathers from northern summering Loggerhead Shrikes indicated that, in adults, flight-feather molt was initiated on the breeding grounds starting with P1, but was rarely completed prior to fall migration. Instead, following some partial primary replacement, molt appeared to be largely suspended until birds reached either their wintering grounds or southern regions that overlap with the known winter range of this species. On average, the most positive δDf values were found in feather S9. It is possible that S9 indicates a molting area prior to reaching the ultimate wintering grounds along the Gulf States of the U.S. and northern Mexico, as in, for example, Lazuli Buntings (Passerina amoena; Young 1991), and Baird's Sparrows (Ammodramus bairdii; Voelker 2004). Unfortunately, the lack of suitable precipitation δD contours for Mexico precluded accurately assigning birds to that region. The suspension of molt in our shrikes was clearly not total and some individuals continued replacing feathers during their autumn movements to the wintering grounds as evidenced by intermediate δDf values. However, most individuals replaced the majority of their flight feathers below the −65‰ δDf contour line.
Our isotopic evidence strongly suggests that the innermost primary flight feathers of migratory northern-latitude adult shrikes were consistently replaced before fall migration, commencing with P1 and continuing outwardly (Palmer 1898, Miller 1928, Yosef 1996). Miller (1928) reported that as the third primary is lost, the center rectrices drop and feather replacement continues outwardly, a pattern also supported by our data. Our analysis also supports the suggestion that molting of the inner tail feathers usually precedes secondary feather replacement and begins with R1, continuing outward (Miller 1928). However, rectrices can often be replaced adventitiously and therefore may not be the most reliable flight feather tract to use in molt studies. According to Miller (1928), as P4 is dropped secondary molt starts at one or two points simultaneously, with either tertial S8 or secondary S1, or both. Yosef (1996) also suggested that molting of secondary feathers starts at two points, but instead with S1 and S6. Our results show that the secondary molting succession began with outermost S1 and continued inward to S6. Both Palmer (1898) and Miller (1928) stated that secondary replacement culminates toward the center of the wing with either S5 or S6, which agrees with our results. Miller (1928) and Pyle (1997) suggested that S9, in both preformative and prebasic molt, is usually replaced prior to fall migration. Pyle (1997) stated that in ~93% of birds, the first prebasic (preformative) molt included 1–3 tertials. Interestingly, our data suggest that feather S9 was most consistently replaced last, at southernmost molting latitudes. Since this result is incongruous with previous molting accounts, we hypothesize that shrikes may adopt a previously undocumented prealternate molt, which may include tertials on the wintering grounds (P. Pyle, Point Reyes Bird Observatory, pers. comm.). Alternatively, the molt sequence of tertials could be S7–S8–S9 or S8–S7–S9, centering at S7 or S8, which could explain the replacement of S9 at southernmost molting latitudes during the prebasic molt (P. Pyle, pers. comm.).
The expected δD values for P1 from birds breeding at two sites in Saskatchewan and Manitoba were more depleted than those measured. There are several possible explanations for this. First, since we had no control over dispersal, our sample could have included birds that moved into our area having grown their feathers farther south the previous year (Hobson et al. 2004). This would result in feathers being more deuterium-enriched than expected. Secondly, there is evidence that raptorial birds have more positive discrimination factors between growing-season average precipitation δD values and feather δD values (Lott and Smith 2006). This would result in feathers having higher δD values than those expected from the altitude-corrected map of Meehan et al. (2004). In addition, since we sampled the base region of feather vanes, representing the last portion to be grown, this growth may have occurred during the initial stages of migration. Finally, any given feather-growing season can be displaced isotopically from the long-term average pattern previously found for North America (Hobson and Wassenaar 1997, Meehan et al. 2004, Hobson 2005). Currently, we have no way to evaluate these possibilities. Nonetheless, this phenomenon does not alter our interpretation of relative locations of molt sites for the birds examined, since the bimodal nature of the results clearly identifies two primary areas of molt that correspond to northern and southern latitudes.
Individual variation in molt may be related to contrasting time and energy budgets of individuals based on breeding success and general phenology (Norris et al. 2004). Competing energetic demands of molt, the need for premigratory conditioning (Marks 1993), and brood rearing will, in part, be influenced by food availability (Young 1991, Leu and Thompson 2002). It is also possible that there is a selection pressure on shrikes to reach their wintering grounds as soon as possible, since they are known to be territorial at wintering sites (Temple 1995) and birds returning earlier can presumably increase their chances of securing better winter territories. Should shrikes suspend molt to compensate for relatively short breeding seasons at northern latitudes in favor of reaching wintering sites earlier? We predict that more southerly breeding populations will show less tendency to interrupt molt following breeding. Hemborg et al. (2001) found that male Pied Flycatchers (Ficedula hypoleuca) from northern latitudes overlapped breeding and postnuptial molt to compensate for short breeding seasons. Howell et al. (1999) observed that in three different species of gulls, residents and short-distance migrants began their prebasic molt months earlier than their long-distance migratory counterparts. Perhaps previously unaccounted-for intraspecific differences among various populations of shrikes from different breeding latitudes may explain the overall variability encountered among descriptions of molt in this species (Miller 1931, Yosef 1996). These are important factors to consider, since populations from the same locality will tend to be more alike with respect to molt than birds from widely separate localities within the range of the species (Miller 1931).
Unquestionably, if accurate age information were available for our sample, a much more powerful interpretation of molting patterns in this species would be possible. Miller (1928) wrote the most thorough systematic review of molting patterns in Loggerhead Shrikes and similarly was only able to age birds to after-hatch-year (AHY) during the latter half of the breeding season. An alternative interpretation of our data is that the bimodal distribution of the δDf values is in fact an effect of a bimodal age distribution. Pyle (1997) stated that during the preformative molt, ~50% of shrikes show an eccentric molt pattern that can include the outer 4–6 primaries (i.e., containing P6 and P9) and the inner 3–5 secondaries (i.e., containing S6). Therefore, it could be argued that the bimodal distribution is the result of SY birds with retained juvenile inner primaries and outer secondaries (P1, P3, S1, S3), thus having a breeding ground isotopic signal, and outer primaries and inner secondaries representing those feathers subsequently grown on the wintering grounds. Although possible, our results emphatically show that most birds grew their feathers on both the breeding and wintering grounds and it is unlikely that our sample consisted of only SY birds.
It is clear that shrikes, including those from northern populations, begin their molt on the breeding grounds (Palmer 1898, Miller 1928, 1931, Yosef 1996, Pyle 1997), but the extension of molt following fall migration has not previously been documented. More studies are needed to examine how this strategy may change with latitude of the breeding population. We also encourage long-term isotopic studies on marked birds of known age and reproductive success. Identifying which feather(s) are most consistently replaced at specific latitudes during migration may in turn indicate the use of staging areas, which can have important conservation implications (Leu and Thompson 2002).
Our results have implications for the previous isotopic study of Hobson and Wassenaar (2001), which used outer rectrices to indicate the breeding area origins of shrikes on the wintering grounds. The reduced likelihood that rectrices are molted on the breeding grounds, at least in northern populations, suggests that these authors provided very conservative estimates of the proportion of northern birds in various southern wintering populations. Thus, future studies should use P1 as a good indicator of breeding location and S9 as an indicator of wintering location.
Acknowledgments
Several individuals assisted with sample collection, including Steve Van Wilgenburg, Alejandro Donatti, and Trevor Van Damme. Stable-isotope analyses were conducted by Leonard Wassenaar at the National Hydrology Research Institute, in Saskatoon. We thank Dan Mazerolle for suggestions on statistical analyses and Peter Pyle and anonymous reviewers for constructive comments and suggestions that improved our manuscript. Funding was provided by the Canadian Wildlife Service, Prairie and Northern Region, through their Endangered Species Recovery Program, and by direct funding to KAH. GEP is grateful for the personal support provided by the Saskatchewan Environment Resource Management Award, Orville Erickson Memorial Scholarship Fund, and the Dr. Malcolm Ramsay Memorial Student Award. Our research protocol was authorized by the Canadian Council on Animal Care administered through the University of Saskatchewan. Shrikes were banded under a Canadian Wildlife Service banding permit.
Literature Cited
Author notes
Corresponding author. E-mail: [email protected]