-
PDF
- Split View
-
Views
-
Cite
Cite
Ralph W. Schreiber, Elizabeth A. Schreiber, Ashley M. Peele, Edward H. Burtt, Pattern of Damage to Albino Great Frigatebird Flight Feathers Supports Hypothesis of Abrasion by Airborne Particles, The Condor: Ornithological Applications, Volume 108, Issue 3, 1 August 2006, Pages 736–741, https://doi.org/10.1093/condor/108.3.736
- Share Icon Share
Abstract
On 11 July 1985, an albino juvenile Great Frigatebird (Fregata minor) was collected by RWS and EAS on Christmas Island (2°N, 157°W) in the tropical Pacific. First observed on 13 July 1984 as a 55–60-day-old chick, the albino was seen flying about the colony on 4 November 1984 and again in February 1985. In July 1985 the juvenile was at its nest with such badly abraded plumage that it could not fly. Burtt (1986) proposed an aerodynamic model that predicts the distribution of melanic feathers in response to abrasion from airborne particles in a flying bird. The albino frigatebird provides a test of this model, since frigatebirds spend most of their time flying and rarely contact water, ground, or objects. Abrasion of the primaries and tail of this albino Great Frigatebird follows the pattern predicted by Burtt (1986). Furthermore, the pattern of damage to secondaries and tertiaries suggests that friction of feathers against each other is another important source of wear.
Resumen
El Patrón de Daño en el Plumaje de un Individuo Albino de Fregata Minor Apoya la Hipótesis de Abrasión por Partículas Aéreas
El 11 de julio de 1985 un juvenil albino de la especie Fregata minor fue colectado por RWS y EAS en la Isla de Navidad (2°N, 157°W), en el Pacífico tropical. Primero fue observado el 13 de julio de 1984 como un polluelo de 55–60 días de edad, el cual fue visto nuevamente el 4 de noviembre de 1984 volando sobre la colonia, y también en febrero de 1985. En julio de 1985 el juvenil fue encontrado en su nido con el plumaje tan severamente dañado que no podía volar. Burtt (1986) propuso un modelo aerodinámico que predecía la distribución de las plumas melánicas en un ave voladora en respuesta a la abrasión proveniente de partículas aéreas. Este individuo albino proporciona una prueba de este modelo, ya que las aves del género Fregata pasan gran parte de su tiempo volando y raramente se ponen en contacto con el agua, suelo u objetos. La abrasión de las primarias y la cola siguen el patrón predicho. Además, el patrón de daño en las secundarias y terciarias sugiere que la fricción de las plumas entre sí es otra razón importante del desgaste.
Bachman (1839) noted that birds changed color when the distal edges of their feathers wore away to reveal brightly colored proximal barbs and barbules. Averill (1923) observed that the white barbs of recently shed primaries of gulls were missing whereas the black barbs, although abraded, remained intact and functional. His observation was repeated by Test (1940) for Northern Flickers (Colaptes auratus) and experimentally replicated in Lesser Black-backed Gulls (Larus f. fuscus) by Bergmann (1982). Burtt (1986) showed that feathers with melanin resisted experimental abrasion by powdered silicon, a common airborne particulate, significantly better than feathers that lacked melanin. Burtt (1979, 1981) proposed that the almost universal dark coloration of the primaries of birds was the product of selection for durability that could withstand the turbulent flow of airborne particles around the wing tips. Furthermore, he suggested that turbulence and abrasion should be less on the inner primaries and secondaries than on those primaries that form the tip of the wing, and still less on areas of the wing and body subjected only to laminar airflow, for example the primary coverts. Burtt (1986) supported his hypothesis with comparative data from differently colored body regions of wood-warblers subjected to more or less turbulence during flight. Barrowclough and Sibley (1980) noted that the albinistic primaries on one wing of a Yellow-rumped Warbler (Dendroica coronata) showed substantially more wear than the dark primaries on the opposite wing. Nonetheless, the pattern of abrasion predicted by Burtt (1986) has not been measured, in part because dark, melanic coloration of the primaries is almost universal among birds. We report the pattern of abrasion in the primaries, secondaries, and tertiaries of the wing and in the tail feathers of an albino Great Frigatebird (Fregata minor) and compare the observed pattern of wear to that predicted by Burtt (1986).
On 13 July 1984 on Christmas Island (2°N, 157°W) in the central Pacific, RWS and EAS found an albino Great Frigatebird chick at 55–60 days of age. It was in the nest where it was fed by its typically colored parents, grew normally, fledged successfully, and was seen flying around the colony on 4 November 1984 and again in February 1985. Its flight, which appeared normal, was above the low-lying vegetation characteristic of the island. However, on 1 July 1985, RWS and EAS observed the same albino, now a juvenile, back at its nest. It had been rendered flightless by excessive damage to the wing and tail feathers. RWS and EAS collected the albino Great Frigatebird on 11 July 1985. In addition to its all white plumage, the juvenile had pale blue eyes and pink feet and bill. The specimen was deposited in the Los Angeles County Museum of Natural History (LACM #102773). The Museum loaned the albino and a typically pigmented juvenile, also from Christmas Island, to AMP and EHB for the purpose of comparing the pattern of feather damage in typical and albinistic plumage.
Methods
We measured the length of the rachis and the inner and outer vanes of the primaries, secondaries, and tertiaries of both wings and all tail feathers on both the albino and the typically pigmented juvenile. Measurements were made with a thin plastic ruler marked in half millimeters and inserted between feathers so that the end of the ruler was against the skin where the feather emerged. The end of the ruler was cut so that the scale began at the end in contact with the skin. For each feather, we measured the rachis from the point of contact with the skin and the emergent feather. We measured the inner and outer vanes from the first pennaceous barb near the base of the feather to the most distal barb that measured at least 5 mm from its base to its tip. Barb length was measured with a small, thin, plastic ruler marked in half millimeters and cut as described above. The ruler was inserted between barbs so that the scale began at the point where the barb branched from the rachis.
We focused our analysis on the wing and tail feathers because these are the areas discussed by previous authors and because we could unambiguously compare feathers from exactly the same position in the wing and tail of the albino and typical bird. To evaluate the damage to the albinistic feathers, we took the length of the vane of the albino feather and divided it by the length of vane of the typical feather from the same position in the wing or tail.
We were not able to analyze our results statistically because the exposure to turbulent air flow differs from feather to feather depending on its position in the wing or tail. Furthermore, as far as we know, this is the only juvenile albino frigatebird ever observed.
Results
At the time of its collection on 11 July 1985, the wing of the albino frigatebird measured 462 mm, ~80% of the mean length for a juvenile from Christmas Island (Schreiber and Schreiber 1988), and the tail measured 199 mm, 54% of mean length.
After receiving the albino specimen, EHB and AMP observed extensive damage to the wing and tail of the albino juvenile that closely matched the field notes and photographs of RWS and EAS (Fig. 1A, B). The pattern of damage (Fig. 1A, B) does not suggest that it was caused by contact with the nest or the ground. Damage from the nest would have occurred along the leading edge of the outermost primary where it and the wing rested on the edge of the nest. No damage was observed along the leading edge until close to the tip of the feather. Had the feathers been dragged along the ground, the ends of the primaries and tail feathers would have been worn flat. However, the barbs were broken from the rachis which protruded well beyond the vane in both wing and tail feathers (Fig. 1A, B), a pattern inconsistent with dragging the feather along the ground. Therefore, we conclude that the damage is the product of many collisions between airborne particles and the barbs and rachis of the feathers.
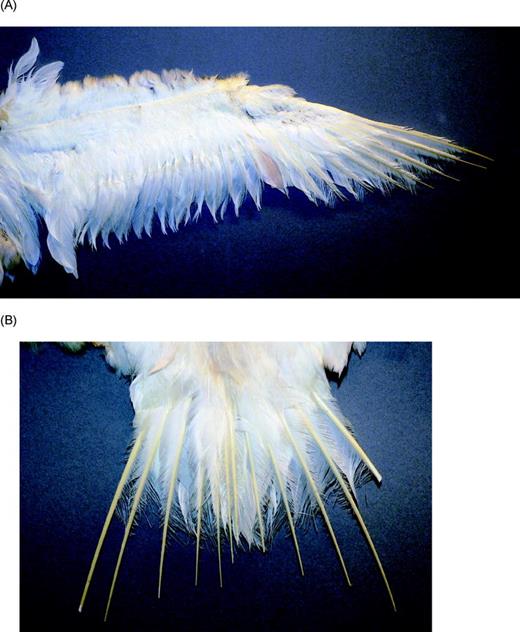
Figure 1. (A) Ventral surface of the left wing and (B) tail of the albino juvenile Great Frigatebird showing the destruction of the barbs and the exposed rachises
Damage to the feathers included barbs broken from the rachis, especially its outer portion, and barbules stripped from the barbs. The cortex of the rachis of many feathers had abraded to the point that the inner medullary layer was revealed. In addition, we noticed that the rachises and barbs were unusually brittle. Whereas a normal feather is resilient and springs back to its original shape when bent, the albinistic rachises and barbs were stiff and tended to break under pressure. We were not able to quantify the brittleness, but found that we had to be very careful with our measurements so as not to break the albinistic barbs or rachises. We did not have to take the same care with the black barbs and rachises.
As predicted by Burtt (1986), primaries 10, 9, and 8, which form the tip of the wing, show the lowest proportion of intact vane of all primaries (Fig. 2). The innermost primaries (P1) on each wing were not included in our analysis as both were emerging from protective sheaths, which suggests that the bird was molting. There is a gradual decline in the proportion of intact vane in secondaries 1 and 2 compared to the inner primaries (4, 3, 2), and secondaries 3, 5, and 6 are badly worn. The proportion of intact vane rises well above the proportion of the least damaged primaries in secondaries 8–15, after which the proportion of intact vane again drops, into the 30 percent range in secondaries 20 and 21, well below the level of all primaries and other secondaries. The tertiaries have a low proportion of intact vane close to the secondaries, the proportion then rises, falls, rises again, but falls below 30 percent next to the body. Although there is variation in the amount of intact vane among the different feathers, the pattern is one of seemingly gradual increase and decrease along the length of the wing. Most feathers show a pattern of wear that is similar to that of their immediate neighbors.
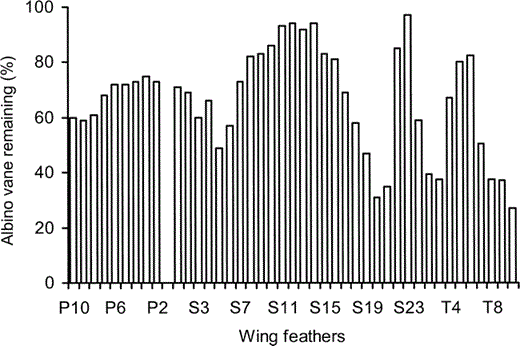
Figure 2. The proportion of intact vane in the wing feathers of the albino juvenile Great Frigatebird, plotted as a percentage of the length of the vane of a wing feather from the same position on a typically colored juvenile Great Frigatebird. Primary 10 (P10) is the outermost primary and primary 1 (P1) is nearest the wrist. P1 on both wings had just been molted and the measurement of the partially regrown replacement feather is not included in the figure. Secondary 1 (S1) is next to P1 and S23 is the secondary closest to the body and next to tertiary 1 (T1). Vane lengths from feathers in the same position on the left and right wings were averaged
The pattern of wear in the tail feathers is also orderly (Fig. 3). On each side, the outermost tail feather (LT6 and RT6) has the lowest proportion of intact vane and the innermost tail feather (LT1 and RT1) has proportionately less intact vane than its immediate lateral neighbor. In a typical frigatebird, the middle four tail feathers (LT2–RT2) have almost the same length (Fig. 4). The general pattern is an increasing proportion of intact vane from tail feather six, the outermost tail feather, to tail feather two on both sides, then a substantial drop in intact vane in the middle feather on both sides.
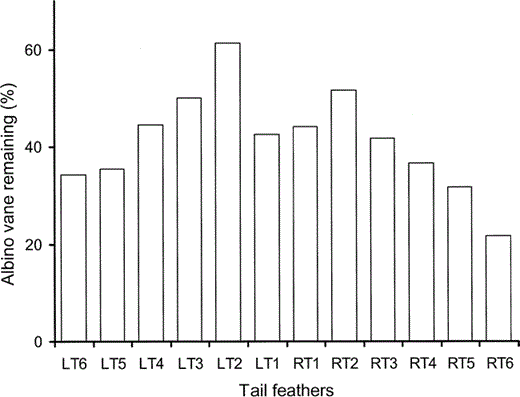
Figure 3. The proportion of intact vane in the tail feathers of the albino juvenile Great Frigatebird, plotted as a percentage of the length of the vane of a tail feather from the same position on a typically colored juvenile Great Frigatebird. Numbering of the tail feathers, of which there are 12, begins with the middle two tail feathers and proceeds innermost to outermost on the left (LT1–LT6) and right (RT1–RT6) sides
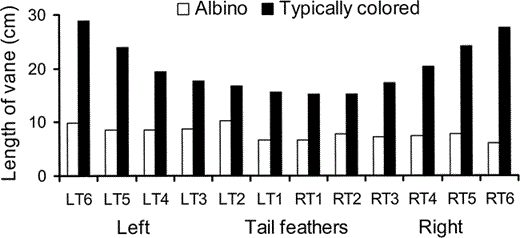
Figure 4. The length of the intact vane of the tail feathers of an albino (white columns) and a typical (dark columns) juvenile Great Frigatebird plotted as a function of position in the tail
The actual lengths of the tail feather vanes of the typically pigmented and albino frigatebirds are shown in Figure 4. What is striking about this comparison is that the length of intact vanes is much more similar (range: 10.3–6.0 cm) across the tail of the albino than the length of intact vanes across the tail of the typical juvenile (range: 15.1–28.8 cm). Furthermore, there is no clear pattern to the slight variation among the albino's tail feathers, whereas the normal bird shows the variation expected in a deeply forked tail, with long outer tail feathers and short central tail feathers.
Discussion
Much of the damage sustained by the albino frigatebird is consistent with abrasion by airborne particles hitting the barbules, barbs, and rachises of wing and tail feathers during flight (Burtt 1986). The tip of the wing, which is formed by primaries 10, 9, and 8, is subject to rapid and turbulent airflow and is the part of the wing with the most motion during flapping flight. This is the region where collisions between airborne particles and feathers will have the most kinetic energy and, therefore, the greatest potential for damage to the feather (Burtt 1986). That primaries 10, 9, and 8 show more damage (lower proportion of intact vane) than primaries farther from the wing tip supports the importance of airborne particles as an abrasive force on feathers as suggested by Burtt (1979, 1986). The data are particularly convincing because frigatebirds do not walk about with their wings dragging on the ground nor climb in vegetation where their feathers could be damaged by contact with leaves, stems, or branches. Neither do Frigatebirds dive into the ocean for food or rest on its surface. Thus, their feathers are not subject to the force of entering the water at high speed, the friction of swimming under or on the water, or the corrosive effect of salt water. Therefore, damage to the flight feathers of frigatebirds must come almost exclusively from abrasion by airborne particles. Because Christmas Island is in the middle of the tropical Pacific where airborne particles are few and small (Duce et al. 1980), abrasion from airborne particles will be less for frigatebirds than for continental birds that fly in air with larger, more abundant particles. Nonetheless, such minimal abrasion was enough to seriously damage the outermost primaries of a frigatebird that lacked the protective hardening that melanin provides to feathers (Bonser 1995, Goldstein et al. 2004).
There are worn feathers in the wing that are not at the wing tips. These are located at the wrist, elbow, and adjacent to the body. Apparently, these feathers are worn and broken from rubbing against other feathers during the flapping motion of the wing. There is also dramatic variation in the degree of wear of the tertiary feathers. This may be the result of the layering of the tertiaries, which are positioned such that some feathers are shielded beneath others. The upper, exposed tertiaries would experience more abrasion from airborne particulates, and thus have a lower proportion of intact vane than the lower, covered tertiaries. To our knowledge, no one has explored such patterns of wear or the color patterns that might result from placing melanic feathers near joints where movement of feathers against each other will increase wear.
The tail of the albino frigatebird also shows a pattern of wear that is consistent with abrasion by airborne particles. The tips of all feathers are frayed as would be expected from turbulent air rolling from relatively high pressure under the tail of a bird in flight into the lower pressure above the tail. What is particularly striking is that the tail feathers of the albino are all about the same length in contrast to the very different lengths in a typically pigmented frigatebird. When the tail is folded the middle feathers are uppermost and, since the tail is usually folded in flight, the middle tail feathers will receive a rapid but laminar flow of air from the back and a turbulent flow sweeping around the lateral edges and tips of the tail (the tip vortices). However, in frigatebirds, the longest tail feathers are the outermost feathers and these fold underneath all the others, the next longest are under all but the longest and so on, until the middle and shortest tail feathers. This means that particles swirling around the tips of the tail in the vortices will abrade the distal ends of the longest feathers (LT6 and RT6) first; then, as these feathers wear down to the length of the next longest and next outermost feathers (LT5 and RT5), these second-longest will begin to abrade away and so on, until all the feathers are more or less the length of the shortest and uppermost feathers (LT1 and RT1). If the flow of particulates we describe is correct, then all feathers that fold under the longest remaining feathers (in this case, LT2 and RT2), i.e., those that currently determine the length of the tail and therefore where the tip vortices and the most severe abrasion occur, must be as short as or shorter than the feathers lateral to them and longer than the feathers medial to them. This is the pattern shown by the worn tail feathers of the albino frigatebird (Fig. 4). Thus, the pattern of wear across the tail, as well as at the wing tips, is consistent with abrasion by airborne particles caught up in the flow of air around a flying bird (Burtt 1986).
In addition to providing increased resistance to abrasion, melanin hardens feathers against bacterial degradation (Goldstein et al. 2004), which could have been a factor in the damage to the feathers of the albino. However, birds that forage in the air, as frigatebirds do, have a very low incidence of feather-degrading bacteria (Burtt and Ichida 1999). Furthermore, the pattern of damage observed in the wings and tail is not predicted by bacterial degradation, which would be expected to affect the less robust body feathers more than the reinforced flight feathers. This was not observed.
We can only speculate why the white feathers of this albino frigatebird were so fragile when the white feathers of other species that share its oceanic habitat (e.g., Common White Tern [Gygis alba]) are not. The differences may be structural. For example, the cortex of melanic barbs is thicker than that of white barbs (Voitkevich 1966), and the cortex of albinistic barbs may be thinner than that of white barbs. Alternatively, the elliptical shape and reinforced ridges of normal barbs may differ in albinistic barbs. Another possibility is that the chemistry of albinistic barbs may differ from that of typical barbs. Melanin granules may promote disulfide bridges between molecules of β-keratin (Goldstein et al. 2004), which is the structural material of feathers. Such bonding may strengthen dark, melanic feathers more than white, nonmelanic feathers. If such bonding were reduced in albinistic feathers, this would weaken the structure even in comparison to typical white feathers. These are testable ideas that merit detailed study of the fine structure and chemistry of albinistic and normal white feathers.
Acknowledgments
We thank Kimball Garrett and the Los Angeles County Museum of Natural History for loaning us the albino Great Frigatebird and a normal juvenile, also collected on Christmas Island, and the Harvard Museum of Comparative Zoology where we measured additional, typically colored juvenile frigatebirds. We are grateful to the Wilson Ornithological Society, which provided a travel grant to AMP that enabled her to meet with EAS in Washington where they discussed the data and drafted the manuscript. Our thanks to Pam Y. Burtt for the digital photographs of the albino frigatebird. We also thank Carla Dove and an anonymous referee for comments on an earlier draft of the manuscript.
Literature Cited
Author notes
Corresponding author. [email protected]
Deceased.