-
PDF
- Split View
-
Views
-
Cite
Cite
Guillermo Fernández, David B. Lank, Sex, Age, and Body Size Distributions of Western Sandpipers During the Nonbreeding Season With Respect to Local Habitat, The Condor: Ornithological Applications, Volume 108, Issue 3, 1 August 2006, Pages 547–557, https://doi.org/10.1093/condor/108.3.547
- Share Icon Share
Abstract
We documented the local density and sex, age-class, and body size distributions of Western Sandpipers (Calidris mauri) among habitats at Bahía Santa María, northwestern Mexico, during the nonbreeding season. Three habitats were recognized: brackish flats, mangroves, and cattail marshes, which we ranked as richest to poorest in food resources and safest to most dangerous in predation danger. Western Sandpiper population structure differed among habitats. Bird densities were highest in brackish flats, the richest and safest habitat, and males and adults of both sexes were overrepresented. In cattail marshes, which appeared to be the poorest and most dangerous habitat, bird densities were lower, and the sex ratio and age ratios within each sex were more even. In mangroves, bird densities were similar to those in cattail marshes, but sex and age ratios were similar to those in brackish flats. Exposed culmen, an index of structural size, was not related to habitat use in either sex. Body mass of immature males was more variable than that of adults among habitats and immature males gained mass throughout the winter. Birds in brackish flats and mangroves were initially heavier, but tended to lose mass, whereas birds in cattail marshes were initially lighter, but tended to gain mass. Mass distributions thus converged in late winter. While the social and ecological causes and significance of differential sex and age-class distributions among habitats remain largely unquantified, evidence from this and previous studies suggests that nonbreeding population structure is a common phenomenon with important implications for migratory shorebirds.
Resumen
Distribución De Los Sexos, Clases De Edad Y Tamaño De Calidris Mauri Con Respecto Al Tipo De Hábitat Durante La Época No-reproductiva
Se determinó la densidad y distribución de los sexos, clases de edad y tamaño de Calidris mauri en diferentes hábitats de Bahía Santa María, noroeste de México, durante la época no-reproductiva. Se reconocieron tres hábitats: planicies lodosas, manglares y tulares, los cuales se clasificaron de mayor a menor con respecto a la densidad de invertebrados y de menor a mayor con respecto al riesgo de depredación. La estructura poblacional de C. mauri difirió entre hábitats. La densidad de aves fue alta en planicies lodosas, el hábitat con mayor densidad de invertebrados y menor riesgo de depredación, y los machos y los adultos de ambos sexos estuvieron sobre-representados en este tipo de hábitat. En tulares, el hábitat que pareció tener la menor densidad de invertebrados y el mayor riesgo de depredación, la densidad de aves fue más baja y la proporción de sexos y edades en cada sexo fue similar. En manglares, la densidad de aves fue similar a la observada en tulares, pero la proporción de sexos y edades fue similar a la observada en planicies lodosas. El culmen expuesto, un índice del tamaño estructural, no se relacionó con el uso del hábitat en ninguno de los sexos. El peso de los machos inmaduros fue más variable entre hábitats que el de los machos adultos, y los machos inmaduros aumentaron su peso durante el invierno. Las aves en planicies lodosas y manglares fueron inicialmente más pesadas pero tendieron a perder peso, mientras que las aves en tulares fueron inicialmente más ligeras pero ganaron peso. Por lo tanto, la distribución de los pesos corporales convergió entre hábitats a fines del invierno. Aunque el origen y el significado social y ecológico de la distribución diferencial de sexos y clases de edad permanecen en gran parte sin ser cuantificados, este y estudios previos muestran que la estructura de poblaciones no-reproductivas es un fenómeno común, el cual presenta implicaciones importantes para las poblaciones de aves playeras migratorias.
Introduction
Habitat choice by birds is a dynamic process affected by many factors. Individuals often choose among habitats that differ in foraging profitability, competition, and level of predation danger (Fretwell and Lucas 1970, Lima and Dill 1990, Sutherland 1996). If individuals or classes of individuals differ in their ability to exploit resources among habitats, for example through differences in foraging morphology, then specialization in different habitats may optimize foraging profitability for different individuals (Zharikov and Skilleter 2002). Different abilities can thus generate differential distributions within a population. Differential distributions would be further favored if asymmetric competition for food occurs, in which the competitive balance changes among habitats. Finally, if the level of predation danger also varies among habitats, individuals will not necessarily select habitats based solely on energetic return. Instead, those individuals with higher vulnerability or lower energetic demands may accept lower energetic returns in order to forage in habitats that are safer (Warnock 1990, Cresswell 1994, Dierschke 1998, Ydenberg et al. 2002, Whitfield 2003). Thus, differences in body size and body condition among competitors affect each individual's best habitat choice, by influencing its ability to acquire resources and its susceptibility to competition and predation (Sutherland 1996).
Many species of migratory shorebird exhibit differential habitat distribution patterns in which sex and age classes are spatially segregated, either latitudinally (Myers 1981, Shepherd et al. 2001, Nebel et al. 2002) or among habitats on a local scale (Cresswell 1994, Warnock 1994, Zharikov and Skilleter 2002, Whitfield 2003, Shepherd and Lank 2004). The underlying mechanisms responsible for such patterns, and their consequences, are poorly understood (Ruiz et al. 1989, Warnock 1994, Nebel et al. 2002, O'Hara et al. 2005). Although shorebirds can move among a variety of habitats, their ability to do so does not suggest a lessened dependence on any given one (Warnock and Takekawa 1995, Shepherd and Lank 2004).
We examined patterns of habitat distribution of Western Sandpipers (Calidris mauri) wintering in Bahía Santa María (“Santa María”), located in northwestern Mexico. On the nonbreeding grounds, Western Sandpipers are partially segregated by sex and age class, with males predominant in the north, and older birds predominant in the center of the range (Nebel et al. 2002). Local age-class segregation by habitat also occurs (Warnock and Takekawa 1995, Buenrostro et al. 1999). Like many other shorebirds, Western Sandpipers may defend feeding territories on the wintering grounds (Tripp and Collazo 1997), but in general their population structure consists of broadly overlapping home ranges (Warnock and Takekawa 1996). Relative to other calidrid sandpipers, Western Sandpipers are highly sexually dimorphic, with females about 10% heavier and 15% longer-billed than males (Cartar 1984). The Western Sandpiper is well suited for studying patterns of differential habitat distribution, because this sexual dimorphism in bill morphology and body size correlates with sex-related differences in foraging behavior and vulnerability to predation that may affect habitat use decisions (Burns and Ydenberg 2002, Ydenberg et al. 2002, Mathot and Elner 2004).
Over 350 000 Western Sandpipers, or 10% of the global population (Bishop et al. 2000), spend the winter at Santa María. Western Sandpipers are widely distributed among a mosaic of habitats (Engilis et al. 1998). The primary objectives of this study were to determine the density and the sex, age-class, and size distributions of Western Sandpipers within this mosaic, and to suggest potential explanations for the observed patterns. We recognized three habitats used by Western Sandpipers—brackish flats, mangroves, and cattail marshes—that we expected to differ with respect to prey density and level of predation danger. Prey density for estuarine shorebirds is often a function of nutrient input, hydrology, and sediment grain size, and has typically been sampled directly (Colwell and Landrum 1993, Zharikov and Skilleter 2002, Rodrigues et al. 2006). The level of predation danger includes both the abundance of predators and aspects of the structure of the habitat (Lank and Ydenberg 2003). For small shorebirds, feeding closer to cover entails a higher risk both of being attacked by an avian predator and of the attack being successful (Leger and Nelson 1982, Cresswell 1994, Whitfield 2003, Dekker and Ydenberg 2004). We therefore recorded predator encounter frequency and estimated distance to cover to rank the habitats with respect to relative level of predation danger.
Methods
Study Area
This research was conducted at Santa María (25°02′N, 108°18′W), about 90 km northwest of Culiacán City, northwestern Mexico. Santa María is the largest wetland on the Sinaloa coast, and is composed of 1350 km2 of a diverse habitat mosaic, which includes an outer bay, intertidal mudflats, mangroves, brackish flats, emergent brackish marshes, and freshwater marshes (Engilis et al. 1998). Study sites were located on the east side of the wetland, just south of the village of La Reforma, covering an area of approximately 180 km2 (15 km × 12 km). The distance between study sites ranged from 0.3 km to 13 km. Three habitats were recognized: brackish flats, mangrove–salt marsh flats, and cattail marshes. Brackish flats were large areas ranging from completely open unvegetated flats to sparsely vegetated areas with Scirpus spp. and Salicornia spp. The mangrove–salt marsh flats (“mangroves”) were smaller open flats broken up by patches of mangroves. Mangroves were dominated by black mangrove (Avicennia germinans) with some emergent vegetation, mainly Spartina spp. and Salicornia spp. The cattail marshes were small beaches in freshwater areas, adjacent to extensive stands of cattails (Typha spp.) with other secondary vegetation, such as Scirpus spp., Atriplex spp., and Chenopodium spp. Brackish flats and mangroves are not affected by daily tidal cycles, but may be flooded twice a month during spring tides, with the highest tides in December. In cattail marshes, the water level is affected by the amount of rain and agricultural runoff during summer and winter, respectively.
Data Collection
We trapped and observed Western Sandpipers at over 100 locations in the wetland during three winters: November to February of 1999–2000, and December to February of 2000–2001 and 2001–2002. We used mist nets accompanied by broadcasts of Western Sandpiper alarm calls to capture sandpipers during morning sessions (e.g., 07:00 to 11:00). At the time of capture, we measured body mass (±0.5 g) and bill (exposed culmen, ±0.1 mm). Each bird was sexed based on bill measurements (female ≥24.8 mm, male ≤24.2 mm; Page and Fearis 1971), and aged as an immature (<1 year old) or adult based on plumage coloration and wear of primary feathers (Page et al. 1972, O'Hara et al. 2002). Birds were banded with a U.S. Geological Survey aluminum band and unique combinations of Darvic® color bands. All morphological measurements and age-class classifications were made by GF. In total, we trapped 1818 Western Sandpipers; 3% (57) of unknown sex were excluded from analyses.
Between January and February of 2000 and 2001, we estimated the density of Western Sandpipers and benthic prey density as an index of resource quality in each habitat. Sandpiper density was estimated using 50 m × 50 m (0.25 ha) plots randomly situated in each habitat. Given the habitat configuration, density observation plots in mangroves and cattail marshes were relatively closer to vegetation cover (<200 m), whereas distances between plots and vegetation cover varied greatly in brackish flats (evenly sampled: close to cover, <200 m; intermediate, 200–900 m; and far from cover, >1000 m). In each plot, all Western Sandpipers were counted at 20 min intervals for at least 3 hr, and an average sandpiper density per plot per day was calculated. Sediment cores were collected where Western Sandpipers were feeding. Cores were extracted using a modified 60 cc syringe (2.6 cm inner diameter, with the apex sliced off and the edge beveled). In both years, cores were collected in a randomly selected foraging site by inserting the syringe 3 cm into the sediment. Sampling frequency varied among years and habitat types depending on access and funding. In 2000, 54 cores were collected from brackish flats and 36 from mangroves; in 2001, 36 cores were collected from brackish flats, 10 from mangroves, and 5 from cattail marshes. Sediment cores were extruded in situ, inserted into appropriately labeled plastic bags, and placed in a freezer. After thawing, samples were washed with distilled water through a 0.5 mm sieve to separate the macrofauna fraction of invertebrates following the methods of Sutherland et al. (2000). Invertebrate taxa were identified and counted to estimate potential prey density.
In 2001 and 2002, we estimated distances from sandpiper capture sites to the nearest vegetation cover. We used these relative distances as an a priori index of the relative level of predation danger of each habitat (sensu Lank and Ydenberg 2003). In all three winters, the raptor encounter rate (raptors hr−1) was estimated for each habitat using a point-count method based on the number of raptors noted in 469 hours of fieldwork. The most common predators of Western Sandpipers were Peregrine Falcons (Falco peregrinus) and Merlins (F. columbarius). In addition, sandpipers occasionally responded to encounters with Northern Harriers (Circus cyaneus).
Statistical Analysis
We assumed that trapped birds were representative of the populations using each habitat type. To assess variation in population structure among habitats and throughout the winter, we analyzed the proportion of males, and adults within each sex, using a mixed-model ANCOVA with the effects of habitat and day of capture as covariates, and controlling for annual variation. The effect of habitat on bird and prey densities was analyzed using one-way ANOVA, controlling for annual variation. To produce more normal distributions for parametric analyses, bird and prey densities were log transformed prior to analysis, and the proportion of males and adults within each sex were arcsine square-root transformed. The small sample size of invertebrate cores for cattail marshes limited our power to detect differences in this habitat type. Due to differences in habitat characteristics, the radius for raptor detection, and therefore instantaneous sampling area, differed among habitats. Typical detection radii by observers were estimated as 200 m, 140 m, and 80 m for brackish flats, mangroves, and cattail marshes, respectively. To account for these differences, raptor encounter rates were adjusted by dividing by 1.0 for brackish flats, 0.7 for mangroves, and 0.4 for cattail marshes. Habitat differences in adjusted raptor encounter rates were investigated using a mixed-model ANCOVA, weighted by relative observation time, with the effects of habitat and day of observation as covariates, and controlling for annual variation. Adjusted raptor encounter rates were log transformed prior to analysis to meet the assumptions of normality for parametric analyses.
Since females are typically larger than males (Cartar 1984), all exposed culmen and body mass analyses were done separately by sex. We compared exposed culmen length as an index of structural size for nonbreeding Western Sandpipers and analyzed variation with respect to effects of age class, habitat, and their interaction, while controlling for annual variation, using a mixed-model ANOVA. We analyzed body mass differences for effects of age class, habitat, and day of capture using these variables and their interaction terms as covariates, and controlling for annual variation, using a mixed-model ANCOVA. We considered statistical test results to be significant at P < 0.05, except for interaction terms, which we considered significant at P < 0.10, since significance tests for interaction terms have lower power than those for main effects (Littell et al. 1991). When interaction terms were not significant, models were reduced to their most parsimonious form based on Type III sum of squares. We report least-squares means (±95% CI) taking the other factors and annual variation into account. We used the Tukey-Kramer test for pair-wise post-hoc comparisons. All statistical tests were performed using SAS version 8.2 (SAS Institute 2002).
Results
Prey Density and Predation Danger
The invertebrate community sampled in sediment cores consisted of Diptera (primarily chironomid larvae, tipulids, muscids, and ephydrids), Hymenoptera, Homoptera, and Lepidoptera. Cores from brackish flats had higher invertebrate densities than those from mangroves and cattail marshes (F2,110 = 12.2, P < 0.01; Table 1). Adjusted raptor encounter rates of 0.4 ± 0.1 raptors hr−1 did not differ throughout the winter (F1,129 = 1.0, P = 0.32), nor among habitats (F2,129 = 0.4, P = 0.69; Table 1). Since our raptor encounter rates are not adjusted for the densities of Western Sandpipers in each habitat, they do not index potential per capita encounter rates. In terms of habitat-specific predation danger, brackish flats were the most open, and thus potentially the safest; cattail marshes were most enclosed, and thus potentially most dangerous; and mangrove habitat was more variable, and intermediate with regard to distance to vegetation (Fig. 1). Combining similar raptor encounter rates with differential distances to cover implies that, all else being equal, brackish flats are the safest and cattail marshes the most dangerous habitats for sandpiper (Table 2).
Western Sandpiper population structure and prey and raptor abundance with respect to habitat type in Bahía Santa María, northwestern Mexico, during the nonbreeding seasons of 1999–2001. Least-squares means (±95% CI) are reported, controlling for annual variation, and sample sizes are given in parentheses. Sample size for bird density = number of observation plots surveyed, for sex and age composition = number of birds, for prey density = number of sediment cores, and for raptor encounter rate = number of survey hours
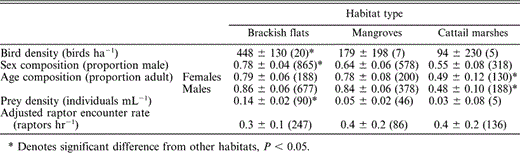
Western Sandpiper population structure and prey and raptor abundance with respect to habitat type in Bahía Santa María, northwestern Mexico, during the nonbreeding seasons of 1999–2001. Least-squares means (±95% CI) are reported, controlling for annual variation, and sample sizes are given in parentheses. Sample size for bird density = number of observation plots surveyed, for sex and age composition = number of birds, for prey density = number of sediment cores, and for raptor encounter rate = number of survey hours
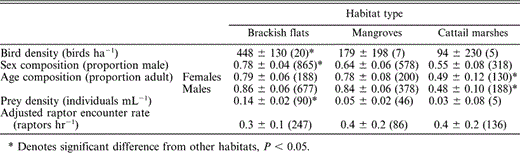
Summary of habitat rankings with respect to environmental conditions and Western Sandpiper population structure, and inferred levels of overall suitability and interference competition in Bahía Santa María, northwestern Mexico, during the nonbreeding seasons of 1999–2001. NSD = no significant difference
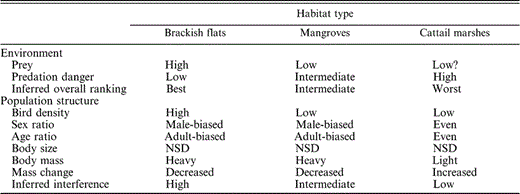
Summary of habitat rankings with respect to environmental conditions and Western Sandpiper population structure, and inferred levels of overall suitability and interference competition in Bahía Santa María, northwestern Mexico, during the nonbreeding seasons of 1999–2001. NSD = no significant difference
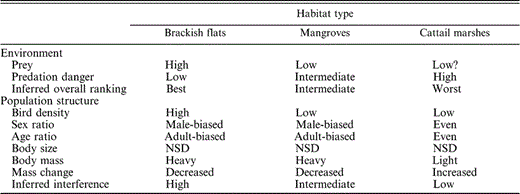
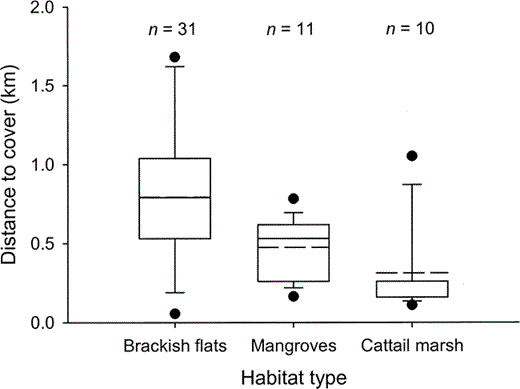
Figure 1. Distances to nearest vegetation cover from Western Sandpiper trapping sites in three habitat types in Bahía Santa María, northwestern Mexico, during the nonbreeding seasons of 2000–2001. The box and whiskers plots show the mean as a dotted line and the median as a solid line (a solid line with no dotted line indicates median = mean), and 5% and 95% (circles), 10% and 90% (whiskers), and 25% and 75% (box) quartiles
Western Sandpiper Densities and Population Structure
Densities of Western Sandpipers ranged from 94 to 448 birds ha−1 and differed among habitats (F2,28 = 4.4, P = 0.02); brackish flats had higher densities than mangroves and cattail marshes (Table 1). Sex ratios did not differ throughout the winter (F1,108 = 2.6, P = 0.20), but were significantly different among habitats (F2,108 = 12.0, P < 0.01). Samples of Western Sandpipers netted in cattail marshes had even sex ratios, while those from mangroves and brackish flats were increasingly male-biased (Table 1). Within sexes, age-class composition did not differ throughout the winter (females: F1,108 = 1.0, P = 0.32; males: F1,108 = 3.6, P = 0.06). Adults of both sexes were overrepresented in brackish flats and mangroves (78%–86%), but the adult:immature ratio was nearly even in cattail marshes (females: F2,108 = 7.4, P < 0.01; males: F2,108 = 18.8, P < 0.01; Table 1).
Western Sandpiper Body Size and Mass
The exposed culmen of females did not differ in length between age classes (F1,512 = 0.4, P = 0.53) or among habitats (F2,512 = 0.1, P = 0.90). Female body masses varied throughout the winter with respect to age class and habitat (day × age × habitat: F2,504 = 4.9, P < 0.01). Due to the statistical interaction, subsequent analyses of body mass were conducted by age class. In immature females, there were no significant differences in temporal patterns among habitats (habitat: F2,132 = 0.1, P = 0.88; day × habitat: F2,130 = 1.0, P = 0.35), nor did body mass change during the winter period (F1,132 = 2.3, P = 0.12). Conversely, body mass of adult females varied throughout the winter with respect to habitat (day × habitat: F2,372 = 9.5, P < 0.01). Adult mass decreased slightly in brackish flats and mangroves, while in cattail marshes mass increased over the winter period (Fig. 2). Controlling for seasonal variation, adult females in brackish flats and mangroves were heavier than those in cattail marshes (F2,372 = 11.4, P < 0.01; Fig. 3).

Figure 2. Body mass of adult female Western Sandpipers with respect to habitat type in Bahía Santa María, northwestern Mexico, during the nonbreeding seasons of 1999–2001
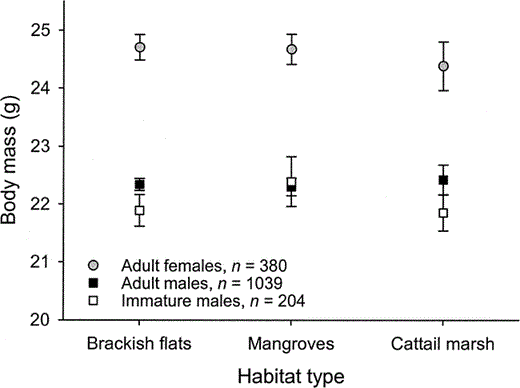
Figure 3. Body mass of Western Sandpipers with respect to sex and age class and habitat type in Bahía Santa María, northwestern Mexico, during the nonbreeding seasons of 1999–2001. Least-squares means (±95% CI) are shown controlling for seasonal and annual variation, and interaction terms
Adult males had longer exposed culmens than immature males (22.7 ± 0.0 mm vs. 22.4 ± 0.1 mm; F1,1237 = 13.1, P < 0.01), but exposed culmen length did not differ among habitats (F2,1237 = 2.0, P = 0.13). Body mass did not differ throughout the winter with respect to male age class and habitat (day × age × habitat: F2,1229 = 1.0, P = 0.35). Age classes differed in body mass among habitats (age × habitat: F2,1231 = 2.8, P = 0.05). Adult males were heavier than immature males in brackish flats and cattail marshes, and body masses of immatures were more variable among habitats than those of adults (Fig. 3). Changes in body mass throughout the winter differed between age classes (day × age: F1,1231 = 8.0, P < 0.01); immature males increased mass during the winter period, while we detected no weight change in adult males (Fig. 4a). Variation in body mass throughout the winter also differed with respect to habitat (day × habitat: F2,1231 = 7.0, P < 0.01); males maintained weight in brackish flats and mangroves, and increased weight in cattail marshes over the winter period (Fig. 4b). We summarize our results among habitats with respect to environmental variables and population structure in Table 2.
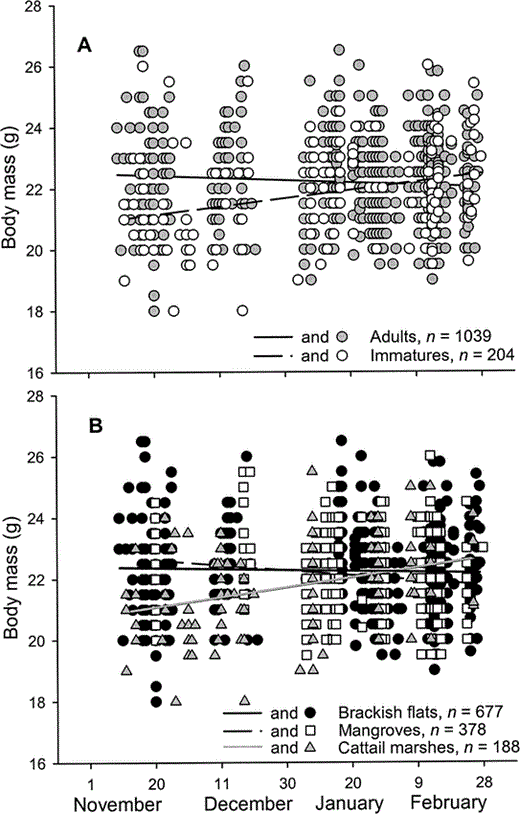
Figure 4. Body mass of male Western Sandpipers in Bahía Santa María, northwestern Mexico, during the nonbreeding seasons of 1999–2001, with respect to age classes (A) and habitat types (B)
Discussion
We documented prey density, predation danger, and Western Sandpiper population structure across three habitats at Santa María during the nonbreeding season. Brackish flats had the highest prey density and lowest level of predation danger, and we expected them to be favored over other habitats. Cattail marshes had lower prey densities and the highest level of predation danger, suggesting that they should be the least preferred habitat, all else being equal. Mangrove areas ranked as intermediate with regard to both prey densities and predation danger. Consistent with these rankings, brackish flats supported the highest densities of Western Sandpipers, which would further lower the per capita predation probability for these individuals, but also potentially increase competition for food. Indeed, we found that birds in brackish flats tended to lose weight as the season progressed. In contrast, in cattail marshes population densities were lowest and birds tended to gain weight over the winter.
There is considerable debate about the relative importance of food resources, predators, and competitors in determining local and latitudinal distributions of wintering shorebirds (Cresswell 1994, Warnock 1994, Nebel et al. 2002, Zharikov and Skilleter 2002, Whitfield 2003). At Santa María, Western Sandpiper density covaried positively with prey distribution among habitats, as generally predicted under “competition for resources” models (Parker and Sutherland 1986). In the absence of competition, all individuals should feed in the habitat with the most food per individual, and utilize the safest habitat. For shorebirds, safety is likely to be greater as density increases (Cresswell 1994, Whitfield 2003); thus, there is an additional negative selection against dispersing into sites with lower bird densities. Our observed patterns of population density thus present a prima facie case that density-dependent competition results in certain individuals using habitats with a lower “basic suitability” (sensu Fretwell and Lucas 1970) in terms of both resources and predation danger. At certain sites in brackish flats and mangroves, a small proportion of the population (5%–7%) defended territories for hours (Fernández Aceves 2005), a clear expression of competition for resources, which could cause subordinate individuals to move into less preferred sites.
The proportion of females and immatures was higher in less preferred habitats, suggesting asymmetry in competitive abilities, and possibly despotic displacement of these classes of birds (Marra and Holmes 2001). In Western Sandpipers, adults are more selective in their use of winter habitats during low tide than immatures (Warnock and Takekawa 1995), suggesting that adults may have excluded immatures from the most profitable habitat as a function of bird densities. However, there is no conclusive direct evidence supporting these competitive asymmetries between sexes and age classes in Western Sandpipers (O'Hara et al. 2005). Small sandpipers do not form stable dominance relationships, and adults do not necessarily win aggressive interactions with immatures more often than expected (Harrington and Groves 1977, Warnock 1994).
An alternative possibility to competitive displacement from a globally preferred habitat is that birds with different morphologies, experience, or other attributes specialize in different habitats (Ydenberg et al. 2002, Zharikov and Skilleter 2002). In Western Sandpipers, the sexual dimorphism in bill length, which correlates with different foraging techniques, may result in a degree of sex-specific niche segregation and consequent resource partitioning (Mathot and Elner 2004). The longer bills of females provide them with a larger potential foraging niche, thus the use of cattail marshes may not be as unprofitable for females as for males. However, the “differential resource partitioning” hypothesis does not obviously account for age-class segregation. Also, although cattail marshes appear to be resource poor, this conclusion is based on a very small sample of substrate cores. It is possible that cattail marshes have higher prey densities than observed because of their finer-grained sediments and proximity to agricultural land.
Within sexes and age classes, we found no evidence of differences in structural size of birds among habitats, but there were some intriguing patterns in mass. Western Sandpipers in brackish flats and mangroves were initially heavier on average, but tended to experience slight mass loss over the winter, while sandpipers in cattail marshes were initially lighter, but tended to gain mass during the winter. This pattern was replicated in all three winters, with large sample sizes. The initial difference in mass among habitats is consistent with mass-selected habitat choice under differential predation danger (Ydenberg et al. 2002). Lighter birds may select cattail marshes as foraging habitat because they are better able to evade predators than heavier birds (Burns and Ydenberg 2002, Ydenberg et al. 2002); reduced competition in the cattail marshes may compensate for increased predation pressure and make this habitat more profitable than the more densely populated brackish flats.
The seasonal pattern in mass changes among habitats could involve at least two processes: (i) the movement of leaner birds among habitats to increase their body condition (Ydenberg et al. 2002), and (ii) the selective predation of leaner birds in cattail marshes over the winter, perhaps because of greater risk-taking by these birds when feeding (Dierschke 2003). Resightings of individually color-banded birds argue against redistribution among habitats. Within and among winters, most birds were resighted in the same habitat in which they were banded (all years combined, within winter: 72%, n = 90 birds; among winters: 60%, n = 30 birds; GF, unpubl. data), suggesting some consistency in habitat usage (but see Warnock and Takekawa 1995). If individuals differentially changed in body mass among habitats, the observed pattern could relate to habitat-specific changes in predation danger, feeding conditions, foraging abilities, or competition (Cresswell 2003, Piersma et al. 2003). We did not detect a seasonal change in raptor encounter rates among habitats throughout the winter. Birds in cattail marshes had higher vigilance and were in larger flocks than those in brackish flats, but vigilance and flock size tended to decrease in all habitats over the season (Fernández Aceves 2005). We have no direct information about changes in food availability, but the extent of water cover in each habitat decreased throughout the winter, likely reducing foraging area, increasing forager density, and potentially increasing competition in all habitats. Consistent with this idea, birds tended to increase their foraging intensity in all habitats over the season (GF, unpubl. data).
Individuals are distributed not only in relation to the resources they require, but also in relation to their competitors (Sutherland 1996). Several shorebird species studied during the nonbreeding season aggregate in areas of higher prey density, yet avoid each other to reduce interference competition (Goss-Custard 1980). We have shown a nonrandom sex and age-class distribution across habitats, with age- and habitat-specific patterns of mass change over the winter period. Understanding the proximate mechanisms controlling individual patterns of habitat use and seasonal mass changes requires more research, in particular on the interplay between social and foraging behavior in wintering Western Sandpipers. However, the fact remains that differential habitat distributions exist within nonbreeding shorebird populations and habitat quality depends on both benefits (food abundance and safety) and costs (interference competition). Habitat loss could be a major factor affecting these interconnected patterns of habitat distribution because of increased density-dependent competition (Goss-Custard et al. 1995). Thus, effective conservation of shorebird populations must include an understanding of local habitat distributions during the nonbreeding season.
Acknowledgments
Numerous individuals provided field assistance, in particular V. Antonio, A. Castillo, M. Guevara, E. Loaiza, and U. Noriega. Patolandia Hunting Club provided logistical support during fieldwork. We thank the Cazares-Medina family for their help during fieldwork, and M. González, X. Vega, the González-Bernal family, and the Escuela de Biología–Universidad Autónoma de Sinaloa for support in Culiacán. This research was financially supported by the Centre for Wildlife Ecology at Simon Fraser University, the Canadian Wildlife Service's Latin American Program, and Patolandia Hunting Club. Additional financial support was provided by Pronatura Noroeste, American Wildlife Research Foundation, Lincoln Park Neotropic Fund, and a Segal Travel Grant. Graduate scholarships from the Mexican National Council for Science and Technology (CONACYT, No. 90768), a Government of Canada Award, and a President's Ph.D. Research Stipend were awarded to GF. Birds were banded under Mexican (Dirección General de Vida Silvestre: 3876, 3278, and 3592) and Canadian (#20383-D) bird-banding permits, and procedures were approved by the Simon Fraser University Animal Care Facility Committee (No. 552-B). We thank R. W. Butler, S. M. Haig, E. Krebs, K. J. Mathot, N. Warnock, R. C. Ydenberg, and an anonymous reviewer for constructive comments on previous versions of this manuscript. This work was part of GF's doctoral dissertation at Simon Fraser University.
Literature Cited
Author notes
Present address: Unidad Académica Mazatlán, Instituto de Ciencias del Mar y Limnología, Universidad Nacional Autónoma de México, Apdo. Postal 811, Mazatlán 82040, Sinaloa, Mexico. [email protected]