-
PDF
- Split View
-
Views
-
Cite
Cite
Jessica L. Yorzinski, Sandra L. Vehrencamp, Kevin J. McGowan, Anne B. Clark, The Inflected Alarm Caw of the American Crow: Differences in Acoustic Structure Among Individuals and Sexes, The Condor: Ornithological Applications, Volume 108, Issue 3, 1 August 2006, Pages 518–529, https://doi.org/10.1093/condor/108.3.518
- Share Icon Share
Abstract
Previous research on individual differences in the acoustic structure of vocalizations and vocal recognition has largely focused on the contexts of parent-offspring interactions, territory defense, sexual interactions, and group cohesion. In contrast, few studies have examined individual differences in the acoustic structure of mobbing and alarm calls. The purpose of this study was to explore individual differences in the acoustic structure of the inflected alarm caw of the American Crow (Corvus brachyrhynchos). The alarm caws of 15 wild, marked individuals were recorded and 25 acoustic measurements were made automatically using customized software. A stepwise discriminant function analysis showed that 20 of the 25 variables were important in discriminating among individuals, with 65% classification success. We used factor analysis to reduce the large number of variables to a set of seven meaningful call features. All of these features differed among individuals, suggesting that American Crows have the potential to discriminate among individual birds on the basis of call structure alone. Five of the features differed between the sexes, with call frequency being the most significant. One clearly subordinate male clustered with the females, raising the possibility that social status partially determines the sex-based differences. Encoding of individual identity in alarm contexts may be adaptive if receiver vigilance and approach urgency depend on the status, reliability, or family membership of the alarm signaler.
Resumen
El Llamado Declinado De Alarma De Corvus Brachyrhynchos: Diferencias En La Estructura Acústica Entre Individuos Y Sexos
Las investigaciones anteriores sobre las diferencias individuales en la estructura acústica de las vocalizaciones y sobre el reconocimiento individual se han centrado principalmente en los contextos de interacciones entre padres e hijos, defensa de territorios, interacciones sexuales y cohesión de grupo. En contraste, pocos estudios han examinado las diferencias individuales en la estructura acústica de las llamadas de alarma y de acoso a los depredadores. El propósito de este estudio fue explorar las diferencias individuales en la estructura acústica del llamado declinado de alarma de Corvus brachyrhynchos. Se grabaron las llamadas de alarma de 15 individuos silvestres marcados y se realizaron automáticamente 25 mediciones acústicas utilizando un programa de computación diseñado para este propósito. Un análisis de funciones discriminantes por etapas demostró que 20 de las 25 variables fueron importantes para la discriminación entre individuos, con un éxito de clasificación del 65%. Utilizamos un análisis de factores para reducir el amplio número de variables a un grupo de siete características significativas de los llamados. Todas estas características variaron entre individuos, lo cual sugiere que los individuos de C. brachyrhynchos pueden potencialmente distinguirse con base en tan solo la estructura de la llamada. Cinco de las características variaron entre sexos, siendo la frecuencia de la llamada la más significativa. Los llamados de un macho claramente subordinado se agruparon con los de las hembras, sugiriendo que el estatus social puede ser un determinante parcial de las diferencias entre sexos. La codificación de la identidad individual en contextos de alarma podría ser adaptativa si la vigilancia y la urgencia de aproximación del receptor dependen del estatus, fiabilidad, o membresía familiar de quien emite la alarma.
Introduction
Recognition based on individually distinctive vocalizations is a prominent and functionally important aspect of signaling among animals in several contexts (Bradbury and Vehrencamp 1998). Previous research has largely focused on differences in the acoustic structure of vocalizations and vocal recognition in parent-offspring interactions, territory defense, sexual interactions, and group cohesion (reviewed by Falls 1982, but see also Shipley et al. 1981, Rendall et al. 1996, Hare 1998, Fischer et al. 2002). Recognition in parent-offspring interactions allows parents to preferentially direct care toward their offspring (Jones et al. 1987). Being able to identify offspring is particularly important in those species where offspring from different families live close together (Beecher et al. 1981). Individual recognition of territory defense signals enables neighbors to distinguish between intruders and adjacent territory holders and avoid unnecessary aggressive interactions (Brooks and Falls 1975, Randall 1994, Temeles 1994). Mates that can identify each other can coordinate their breeding activities (Brooke 1978), and vocal recognition in group-living species can enable group members to remain in contact with each other over long distances to synchronize movements (Snowdon and Cleveland 1980, McComb et al. 2003).
Consistent individual differences in the acoustic structure of vocalizations are a prerequisite for individual recognition among animals (Falls 1982). Such differences in the acoustic structure of vocalizations have been demonstrated in many species (Jones et al. 1987, Bradbury et al. 2001, Sousa-Lima et al. 2002). While these individual differences in acoustic structure suggest the existence of individual recognition, there may not be enough variation in the vocalizations to allow discrimination (Falls 1982). Playback experiments requiring subjects to distinguish among vocalizations are necessary to show that animals are using acoustic differences to recognize individuals (Jones et al. 1987, Rendall et al. 1996).
Recognition may also be important in environmental contexts involving predators. Some studies have investigated individual recognition in alarm calls (Cheney and Seyfarth 1988, Hare 1998, Blumstein et al. 2004), and a few have documented individual differences in the acoustic features of these calls (Fischer et al. 2002, McCowan and Hooper 2002). From a signal design perspective, group or individual signature information should be most important for alarm calls that either alert or recruit nearby conspecifics (Bradbury and Vehrencamp 1998).
Species in the Corvidae family are ideal for exploring the structure and function of alarm calls, because they often live in complex social groups and exhibit intense antipredatory behaviors using a variety of alert, alarm, and mobbing vocalizations (Conner 1985, Elowson and Hailman 1991, Griesser and Ekman 2004). The American Crow (Corvus brachyrhynchos), in particular, is a cooperatively breeding bird that spends much of its time in extended family groups (Kilham 1984, 1989, Caffrey 1999, McGowan 2001a, Verbeek and Caffrey 2002). A family group may consist of up to twelve adult individuals. These individuals usually include a single breeding pair, offspring from a number of different broods, and sometimes immigrants (McGowan 2001a, Verbeek and Caffrey 2002). Family members defend a group territory, feed and defend dependent young, and guard each other while foraging together (Serrell 2003). The American Crow has a rich repertoire of calls with many modulations and intergradations associated with warning against and mobbing predators (reviewed by Verbeek and Caffrey 2002). We conducted a quantitative study of the acoustic structure of the inflected alarm caw (Brown 1985) in individually marked, free-living crows to determine whether alarm calls encode individual identity. The inflected alarm caw is a short distinctive vocalization given in response to owls, hawks, and humans (Chamberlain and Cornwell 1971, Brown 1985, Parr 1997). Although the temporal pattern of a cawing series has been hypothesized to play a role in distinguishing individuals (Thompson 1969, Richards and Thompson 1978), we restricted our analyses to the acoustic properties of the call itself to see if sufficient differences existed for separation. Consistent individual differences in the acoustic structure of the inflected alarm caw would suggest that crows possess the potential to discriminate individual callers in alarm contexts.
Methods
Study Site and Subjects
Vocalizations of the eastern subspecies of adult American Crows (C. b. brachyrhynchos) were recorded in Ithaca and Cayuga Heights, New York (42°N, 76°W) between June and August 2003 (shortly after the young had fledged). Over 200 hr of observations were made between 06:00 and 18:00 in the territories of crow families. The territories consisted of fields, cemeteries, residential neighborhoods, and campus areas (McGowan 2001a).
Since 1989, nestlings from this crow population have been marked with unique combinations of colored leg bands and with numbered or lettered patagial tags approximately one week before fledging. Two individuals (m2K and m3L) were not marked as nestlings, but were marked as adults by capturing them on their territories with a net launcher. Blood samples were taken at the time of banding to determine the sex of all individuals using 2550 F and 2718 R polymerase chain reaction primers and the methodology of Fridolfsson and Ellegren (1999).
Acoustic Sampling and Analysis
Vocalizations were collected from 15 marked individuals belonging to five different family groups. Three of the family groups (ALOT, LRIS, and MART) occupied adjacent territories. Nine crows were male and the remaining six were female. Six individuals were yearlings, four individuals were two years old, and five individuals were three years or older (Table 1). All individuals were helpers, with the exception of one breeding male (either m2K or m3L). Unless otherwise indicated, individuals will be referred to by their sex (m or f) plus the first two letters or numbers of their tag names (Table 1).
Identification of American Crows studied in Ithaca, New York, from June through August 2003. The 3+ age group indicates that the individual was at least three years old
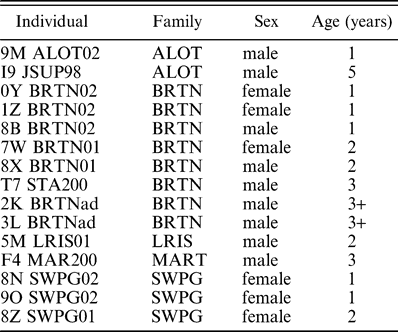
Identification of American Crows studied in Ithaca, New York, from June through August 2003. The 3+ age group indicates that the individual was at least three years old
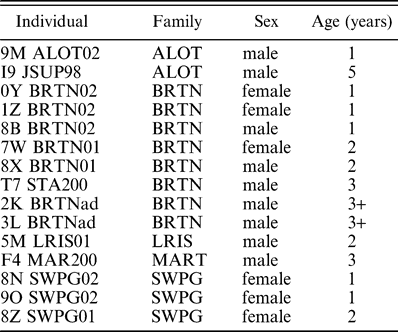
The recordist elicited alarm caws by approaching marked crows to within approximately 10 m and looking at them. Vocalizations were recorded with a Tascam DA-P1 DAT recorder (Teac America, Montebello, California) and Audio-Technica (Akron, Ohio) AT4071a directional microphone. In addition, the time of day, presence of other individuals (marked and unmarked), whether other individuals were vocalizing, and other salient contexts (e.g., aggressive interactions, mobbing events, countercawing, foraging, and affiliation) were noted. With the exception of m8X and mT7, the inflected alarm calls of individuals were recorded on multiple days (5.3 ± 0.8 SE days; range = 1.0–12.0). Most birds were recorded for one continuous session on a given day, but a few birds were recorded twice in one day, separated by at least 1 hr. Recording sessions lasted from 1 to 20 min and ended when the crow either stopped cawing or flew away. On average, 8.8 caw series were recorded in each session; a series is defined as a temporal cluster of caws separated by less than 500 msec.
Recordings were digitized (sample rate: 44 100 Hz; sample size: 16 bit) using Raven 1.2 (Charif et al. 2004). All caw vocalizations from marked individuals were extracted and converted into spectrograms (512-point FFT; window function: Hanning; 50% overlap; 124 Hz bandwidth; 256 points per frame; frequency: 86.1 Hz). The caws were visually inspected and those with high levels of background noise (e.g., other bird vocalizations, traffic, or human voices) were removed.
We used XBAT 0.3 (Figueroa 2006), a sound analysis platform that supports custom algorithm plugins, to make a variety of systematic measurements on the caw sound clips. A total of 25 acoustic measurements from two different plugins were used to characterize the frequency, duration, bandwidth, and shape of the caws. The energy distribution measurement plugin (Cortopassi 2006a, 2006b) computes robust measurements based on a user-specified interior percentage of energy in an acoustic signal, reducing the effects of outliers, background noise, and variable recording levels (Fristrup and Watkins 1992). We specified 75% of the energy within the frequency range of 400–3000 Hz. The algorithm sums the power values of the signal's spectrogram over time bins or frequency bands to generate aggregate time and frequency envelopes, which are then normalized and treated like probability density functions (spectrogram settings: FFT size = 512 points; window size = 512 points; window function = Hanning; overlap = 256 points). Measures of central tendency and dispersion are calculated using nonparametric order statistics. For bandwidth and duration measurements, the interpercentile range is computed based on the specified energy percentage. Asymmetry of the time or frequency envelope is computed as percentile skewness, and contours describing changes in frequency versus time are used to describe signal shape. We selected 16 of the energy distribution measurements that provided meaningful variation among the sound clips. The remaining nine measurements were made using the frequency contour measurement plugin (Cortopassi 2006c). This algorithm uses a spectral autoregressive technique to estimate signal frequency over short time periods, and a Viterbi-based tracking algorithm to generate the best track through the frequency estimates, resulting in a continuous trace of the best harmonic contour in the specified frequency range (analysis settings: data length = 512 points, overlap = 256 points, number of poles = 60, pole magnitude = 0, frequency range = 700–1700 Hz). Various measures of contour shape are computed such as start, peak, and end frequencies, and frequency modulation patterns. The routine successfully traced the second harmonic of all inflected alarm calls.
Statistical Analyses
Of the 10 853 caws recorded from marked individuals, only a subset was analyzed. This subset consisted of caws that were emitted in similar contexts and were of the same caw type. To be considered in context, vocalizations had to be emitted by perched crows that began cawing after being approached and were uninvolved in aggressive interactions, mobbing events, countercawing, foraging, or affiliation. The caw type was the inflected alarm caw (Brown 1985), which appears to be equivalent to the warning (Good 1952), simple scolding (Chamberlain and Cornwell 1971), high-pitched cawing (Richards and Thompson 1978), kuk (Kilham 1989), and ko (Parr 1997) calls. We visually inspected the spectrograms of all caws emitted in the above context and selected those that were chevron-shaped and possessed a harmonic stack up to 6–7 kHz. Since Brown (1985) and Parr (1997) distinguished caw types based on call duration, we examined the histogram of caw durations for our own sample and found a quadrimodal pattern similar to the distributions of Brown (1985) and Parr (1997; Fig. 1). To ensure that we were only analyzing one call type, we selected calls within the first mode of the distribution, and excluded calls with durations greater than 90 msec. Our duration measurement, which is based on the interior 75% energy fraction of the call as explained above, is approximately 50% of the duration that would be measured from the edges of a spectrogram image.
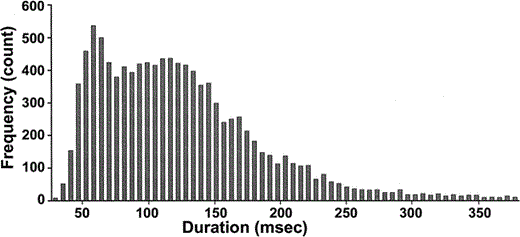
Figure 1. Frequency histogram of call duration using all recorded caws from known individual American Crows. Duration was measured automatically using the internal 75% of the energy distribution of each call. This EDM duration measurement can be converted into a standard external measurement of duration by: standard duration (msec) = 90 + 0.67(EDM duration in msec). Caws with a duration greater than 380 msec (n = 70) are not shown on the histogram
Because the number of inflected alarm caws recorded from each individual varied over a wide range (8–604 calls), a limited number of inflected alarm caws from each individual were selected to balance statistical analyses. We used a random number generator to select 20 inflected alarm caws from each individual. In the one case in which fewer than 20 calls were recorded from a particular individual (m3L, eight caws recorded on three different days), all caws were analyzed. The random subset sampled broadly from the available recordings and included 80% of the recording sessions; 74% of the calls in the subset came from different caw series. The distributions of the acoustic measures extracted from this subset of calls were tested for normality, and non-normal variables were transformed as needed. A few calls with conspicuously outlying measures were replaced with more typical calls.
A stepwise discriminant function analysis (PROC STEPDISC in SAS v. 8; SAS Institute 2000) was used to determine which acoustic parameters of inflected alarm caws differed among individuals. Discriminant function analysis uses a classification procedure to identify a linear combination of quantitative predictor variables that most accurately characterizes differences among groups. The percentage of cases correctly categorized by the classification procedure (correct classification) is compared to the percentage of cases that would be correctly classified by randomly assigning the cases into categories (prior probability). The stepwise procedure reduces the number of variables used in the discriminant function by selecting those that have the best discriminatory power. Because the data being categorized are used to build the classification function, the error rate may be biased. To reduce this bias, we used a cross-validation procedure. This procedure excludes the first observation from the data set, builds a classification function based on the remaining observations, and then classifies the first observation. Next, the first observation is returned to the data set, the second observation is removed, and the process is repeated until all of the observations have been classified (Tabachnick and Fidell 2001).
Because some of our acoustic measurements were correlated, we used factor analysis with varimax rotation (PROC FACTOR in SAS v. 8) to reduce the original 25 acoustic measurement variables to a smaller set of orthogonal factors (Tabachnick and Fidell 2001). Scores for two of the factors (bandwidth and harmonic weighting) were square-root transformed and then standardized to normalize their distributions. We submitted these factors to a discriminant function analysis using the caws of the 15 individual crows. The centroid plot of the first and second canonical variables from this discriminant analysis was created in JMP v. 5 (SAS Institute 2002). To examine the role of each acoustic factor in generating individual differences, we performed a general linear model analysis on each factor with a model of the form: y = sex + individual(sex), i.e., nesting individuals within sex to control for sex effects. We were unable to evaluate effects of family membership because our samples did not contain balanced numbers of males and females in each family. Age effects also could not be evaluated with longitudinal sampling because a majority of our focal individuals succumbed to West Nile virus or other mortality agents. Means ± SE are provided in tables and graphs to illustrate effect sizes. Significant differences were concluded for P-values less than 0.05.
Results
The inflected alarm caws of individuals can be distinguished with a linear combination of acoustic variables (Wilks' Lambda: F280,2965 = 4.9, P < 0.001; Table 2, Fig. 2). The stepwise discriminant analysis extracted 20 of the 25 acoustic measurements from the calls of the 15 individuals. Using these 20 measurements, the discriminant function correctly classified 65% of all calls (cross-validated = 45%; prior probability of random correct assignment = 6.7%). The proportion of correctly classified calls for each individual was highest for crows recorded on only one to three days (86% correct, n = 5) and decreased as the number of recording days increased, but leveled off for birds recorded on four or more days (56% correct, n = 10; two-tailed unpaired t13 = 4.5, P < 0.001).
Number of inflected alarm caws assigned to each individual crow by a discriminant function analysis using the 20 significant acoustic measurement variables identified during a prior stepwise removal process. Individuals are referred to by the first two letters or numbers of their individual tag names preceded by m for males and f for females (Table 1). The sample of calls analyzed is n = 20 for all individuals except m3L, where n = 8
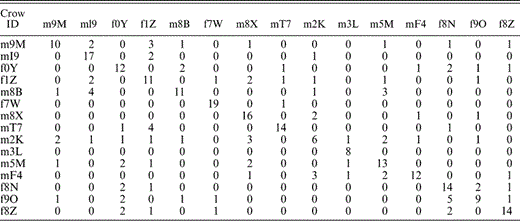
Number of inflected alarm caws assigned to each individual crow by a discriminant function analysis using the 20 significant acoustic measurement variables identified during a prior stepwise removal process. Individuals are referred to by the first two letters or numbers of their individual tag names preceded by m for males and f for females (Table 1). The sample of calls analyzed is n = 20 for all individuals except m3L, where n = 8
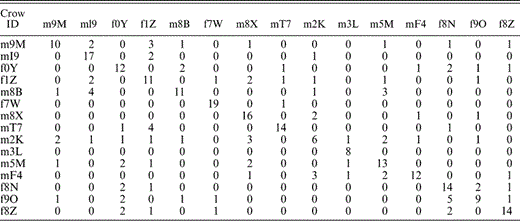
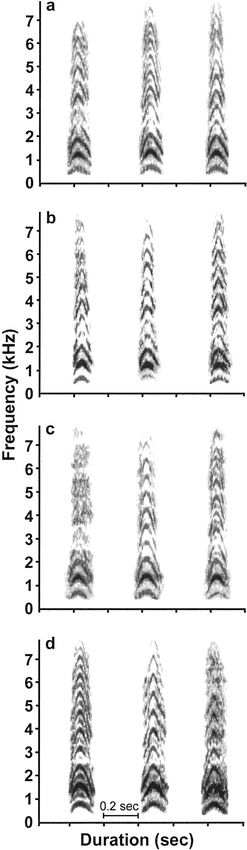
Figure 2. Spectrograms of the inflected alarm caws of four individual American Crows. The three caws from each individual were recorded on three different days (the spacing between the caws is not meaningful). Two individuals are males (a: m2K; b: m8B) and two individuals are females (c: f1Z; d: f9O)
Seven factors explaining 90% of the variation in the original acoustic variables were extracted with the factor analysis. The variables loading strongly on each factor produced meaningful composite variables (summarized in Table 3). Factor one positively loaded all of the frequency point measures such as start frequency, peak frequency, and end frequency, and is therefore a measure of the center frequency, or elevation, of the entire call. Factor two contained all of the frequency spread variables along with entropy and is best described as overall bandwidth. Call duration measures were all associated with factor three, while frequency skew of the aggregate power spectrum was characterized by factor four. Factor five described the level of frequency modulation of the second harmonic contour or, in our case, the shape (height) of the chevron. Factor six measured the level of frequency modulation in the 400–3000 Hz range. Since this factor was similar to but orthogonal from factor five by definition, it was best described as the change in relative harmonic weighting during the call. Finally, factor seven measured the temporal skew in the aggregate amplitude envelope, or the amplitude of call onset relative to call center. A discriminant function analysis performed on the seven factors correctly classified 49% of the calls (cross-validated: 41%; Wilks' Lambda: F98,1698 = 7.6, P < 0.001). All seven of the acoustic factors significantly contributed to the differentiation of individual bird's calls (Table 3).
Summary of analyses of acoustic measurement factors extracted from the inflected alarm caws of 15 individual American Crows of known sex. Each orthogonal factor was analyzed separately in a multivariate model that included sex and individual identity (nested within sex). Variance explained by each factor, F statistic, degrees of freedom, and P values are shown. Variables loading on each factor are given in footnotes and further described in Cortopassi (2006a, 2006b, 2006c)
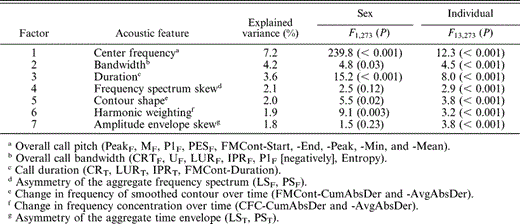
Summary of analyses of acoustic measurement factors extracted from the inflected alarm caws of 15 individual American Crows of known sex. Each orthogonal factor was analyzed separately in a multivariate model that included sex and individual identity (nested within sex). Variance explained by each factor, F statistic, degrees of freedom, and P values are shown. Variables loading on each factor are given in footnotes and further described in Cortopassi (2006a, 2006b, 2006c)
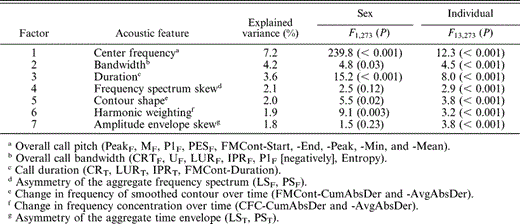
Sex of the caller was responsible for the strongest differentiation of acoustic call features. Based on the canonical plot, there was a clear distinction between the calls emitted by males and females (Fig. 3). However, one male's centroid point (mT7) clustered among the female points. Unlike the results of the individual analysis, only measures of center frequency, bandwidth, duration, contour shape, and harmonic weighting differed significantly between females and males (Table 3). Female calls had higher center frequencies, wider bandwidths, shorter durations, and taller contour heights than male calls. Females also increased the relative amplitude of higher harmonics in the middle of the call more than males. Center frequency was clearly the most significantly different acoustic factor between the sexes (Fig. 4). Excluding mT7, a discriminant function analysis of caller sex using the seven factors correctly classified 87% of the calls (Wilks' Lambda: F7,260 = 51.3, P < 0.001).
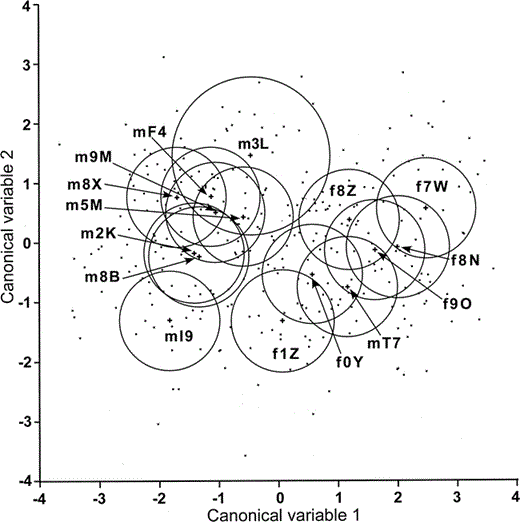
Figure 3. Location of all of the inflected alarm caws of 15 crows and their centroids (+) on the first two canonical functions. Circles represent 95% confidence intervals for the estimate of the mean centroid location. Identification numbers of individual crows are preceded by m for males and by f for females
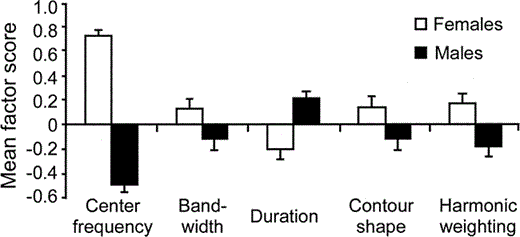
Figure 4. The least-squares mean factor scores of inflected alarm caws of male and female crows for the five acoustic features that differed significantly between the sexes. Error bars represent ±1 SE. Statistical significance is given in the Sex column of Table 3
Discussion
The acoustic characteristics of the inflected alarm caw differed significantly among individuals and sexes of American Crows. These results suggest that the birds possess the potential to identify individuals and sexes based solely on the acoustic structure of the inflected alarm caw.
All seven of the acoustic features we measured were important in differentiating among the inflected alarm caws of individuals. Unlike previous studies that have investigated individual differences in the vocalizations of American Crows (Thompson 1969, Brown 1979), we analyzed the calls of a relatively large sample of wild crows on multiple days using many different acoustic measurements. Our results were consistent with those of a similar study conducted on an equivalent “krah” vocalization of the Hooded Crow (Corvus corone cornix, Allenbacher et al. 1995). In that study, the caws of seven individuals were perfectly discriminated using a linear combination of 85 acoustic variables. Allenbacher et al. (1995) may have achieved a higher discriminatory power than we did because they sampled fewer birds and each individual was recorded during one recording session. Our results indicate that caw structure can vary somewhat over different days. Furthermore, the Hooded Crow study did not involve tagged birds, so the researchers did not know the gender, age, breeding status, and family membership of their subjects.
Sex-based differences in the inflected alarm caws provided the strongest differentiation among individual American Crows. Several prior studies have suggested that females utter higher-pitched vocalizations than males (Davis 1958, Goodwin 1976, Verbeek and Caffrey 2002; see also Verbeek and Butler 1999 and McGowan 2001b for similar information for the Northwestern Crow and Fish Crow, respectively). However, this is the first study to quantify acoustic differences between the sexes and to provide statistical evidence for higher frequencies in the inflected alarm caws of females. Moreover, the alarm caws of the two sexes differed in other acoustic features, with females producing calls with wider bandwidths, shorter durations, taller contour heights, and more frequency modulation than males. The higher frequency, wider bandwidth, taller contour height, and stronger frequency modulation of female inflected alarm caws are responsible for their shriller sound to human ears.
These differences in the acoustic structure of vocalizations among individuals and sexes may be caused by differences in both vocal fold vibrations and vocal tract resonances (McComb et al. 2003). Larger individuals in some species have larger vocal folds and consequently produce vocalizations at lower frequencies (Morton 1977). Larger individuals also often have larger or longer vocal tracts and produce vocalizations with less spacing between formants (Fitch 1997). Consistent with these trends, we found that male American Crows produced vocalizations with lower frequencies. Because males are generally larger than females (but size distributions overlap broadly; Verbeek and Caffrey 2002), it is likely that body size partially determines the acoustic structure of their vocalizations.
The exceptional location of the call centroid of one male, mT7, among the cluster of female call centroids in canonical variable space suggests that other factors also affect acoustic structure. This bird was captured as a two-year-old in the year prior to this study. His body size (based on skeletal and feather measurements) was average for a male, but his mass was below the male average and similar to the female average. He had immigrated into the BRTN group from a nearby natal group and was clearly subordinate to the two other, older adult males. Females are also socially subordinate to males (KJM and ABC, pers. obs.). Social status may therefore play an even greater role than body size in determining the center frequency and other acoustic features of alarm caws (Fischer et al. 2004). The mechanism that causes this status effect remains an intriguing question.
The ability of animals to recognize individuals, particularly their sex or status, based on their alarm calls could potentially provide extremely useful information to receivers. This ability may be particularly important among family groups of the cooperatively breeding American Crow because individuals within family groups have known each other for most of their lives and interact constantly. One possible benefit of individual recognition is that crows could change their vigilance behavior depending on the reliability of the signaler (Cheney and Seyfarth 1988). If some signalers (e.g., yearling family members) tended to emit alarm calls when little danger was present, receivers could avoid expending unnecessary energy and time by not responding to that individual's alarm calls (Ydenberg and Dill 1986). Likewise, if a signaler only emitted alarm calls in extremely dangerous situations, receivers would benefit by being especially alert (Robinson 1981). Another possible advantage is that receivers could evaluate the immediate danger associated with the predator. Because certain individuals tend to be farther away than others (e.g., neighbors are likely to be located at a greater distance than family members), receivers could determine the relative location of the danger (Hare 1998). Finally, receivers could alter their own alarm calling behavior and reciprocate with alarm calls only in the presence of individuals that have previously given alarm calls (Axelrod and Hamilton 1981).
The vocal communication system of the American Crow consists of many other caw-like vocalizations that are used in a variety of different contexts. These vocalizations can be largely categorized by their durations (Brown 1985, Parr 1997). This implies that at least one of the parameters (duration) that distinguished individuals and sexes also varies considerably within individuals, possibly reflecting differences in the perceived urgency of the situation (JLY and SLV, unpubl. data). If many of the other acoustic variables remain consistent for individuals when they utter different caw types, then crows may also be able to identify individuals based on the acoustic structure of these other vocalizations in a variety of contexts. Further studies investigating the abilities of crows to recognize individuals based on acoustic cues could enhance our understanding of the information encoded in their vocalizations.
Acknowledgments
We thank Kathryn Cortopassi, Harold Figueroa, Kurt Fristrup, Mya Thompson, Harold Mills, and Christopher Tessaglia-Hymes for help with the acoustic software and analyses. Irby Lovette was responsible for the genetic sexing of the crows. Doug Robinson organized general population monitoring and assisted in the marking of adult and nestling birds. Crow handling protocols were approved under IACUC permit #88-0210. Simona Despa, Karen Grace-Martin, and Steven Schwager provided invaluable statistical guidance. Carlos Botero kindly translated the title and abstract into Spanish. Nicholas Thompson and an anonymous referee offered useful suggestions for improving the manuscript. The sexing of the crows was supported by a Centers for Disease Control (NY-DOH) award to KJM, NSF grant (DEB 29444) to ABC, and a National Institutes of Health grant (AI064305-01) to L. Patrican (with ABC and KJM as co-Principal Investigators). JLY was funded by a Cornell Presidential Research Scholars grant.
Literature Cited
Author notes
Corresponding author. [email protected]