-
PDF
- Split View
-
Views
-
Cite
Cite
Jesse C Seegmiller, Lorin M Bachmann, Urine Albumin Measurements in Clinical Diagnostics, Clinical Chemistry, Volume 70, Issue 2, February 2024, Pages 382–391, https://doi.org/10.1093/clinchem/hvad174
- Share Icon Share
Abstract
Measurement of urine albumin is critical for diagnosis, risk classification, and monitoring of chronic kidney disease (CKD). Guidelines recommend clinical decision cutoffs for the urine albumin-to-creatinine ratio (ACR) of 30 and 300 mg/g (3 and 30 mg/mmol). However, differences among manufacturers’ routine urine albumin measurement procedures have been found to exceed 40%, suggesting CKD diagnosis and risk classification may vary depending upon the specific measurement procedure implemented in the laboratory.
This review discusses urine albumin pathophysiology and clinical practice guideline recommendations for CKD. The review also provides recommendations for urine specimen collection and storage, and results reporting for the ACR. Recent advances in measurement techniques and development of reference systems intended to facilitate standardization of urine albumin measurements are reviewed.
Urine albumin is an important measurement procedure used for diagnosis, risk classification, and management of CKD. Urine albumin results should be reported as the ACR using quantitative measurement procedures. Random urine collections used for albuminuria screening should be followed by confirmation with first morning void collections to reduce variation and increase diagnostic accuracy for urine albumin measurement. Most measurement procedures utilize immunoturbidimetric or immunonephelometric techniques. However, results vary significantly among measurement procedures, potentially resulting in differences in classification or risk assessment for CKD. The National Institute for Standards and Technology (NIST) and other laboratories are developing reference systems, including liquid chromatography–tandem mass spectrometry candidate reference measurement procedures and reference materials, to enable standardization of routine measurement procedures.
Albuminuria Pathophysiology
It is estimated that 3.3 g/day of albumin is filtered by glomeruli in healthy kidneys and approximately 3.2 g/day of this filtered albumin is taken back up by the kidneys through tubular reabsorption mechanisms (1). Thus, the amount of albumin found in the urine is an interplay between how much is allowed to pass through the glomerulus as well as the reabsorptive capacity of the renal tubules. Studies which have proposed and modeled the tubular reabsorption capacity in kidneys illustrate that when saturation of the reabsorption mechanism occurs, higher albumin concentrations will spill into the urine (2, 3). Therefore, tubular damage can cause albuminuria, even if the glomerular filtration rate is considered healthy. The amount of albumin reaching the tubules is governed by the glomerulus. As described above, in healthy kidneys most of the albumin filtered by the glomerulus is reabsorbed by the tubules. Glomerular injury increases the concentration of albumin reaching the tubular space. If tubular reabsorption is overwhelmed, albuminuria will be increased.
There are many proposed mechanisms of glomerular injury and resulting albuminuria (4). Initial theories suggested processes related to molecular size and charge are important filtration barriers for serum proteins. Most of these molecular size studies were conducted using dextran molecules of a known diameter by monitoring their clearance into the urine to estimate glomerular pore size (5, 6). Given the dextran studies and the observation that glomerular basement membranes are negatively charged due to anionic glycoproteins on the epithelial wall, it was suggested that a negative charge serves as an electrostatic barrier to repel and prevent albumin from being filtered by the glomerulus.
Other theories suggest the negative electrostatic charge on the glomerular basement membrane may not be as vital a filtration barrier as the initial models have proposed (7). These hypotheses suggest that filtration of albumin is governed by buttress forces from glomerular podocytes. As depicted in Fig. 1, injury to podocytes causes rounding of their processes, which reduces the buttress forces, leading to increased albumin permeability through the glomerular basement membrane and increased albuminuria. Podocyte damage also results in the reduction of the filtration slit area, which causes decreases in small molecule filtration (8, 9). This proposed mechanism fits well with the typical clinical findings in glomerular kidney disease where an increased amount of albumin is excreted into the urine, while the slit area filtration is reduced, leading to a decreased ability of the nephron to remove small molecule solutes through the glomerular basement membrane.
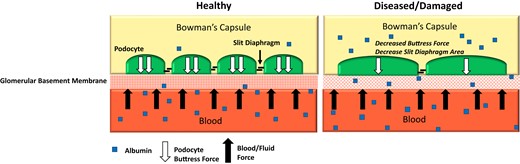
Mechanism of albuminuria for the podocyte injury theory. Healthy podocytes have increased slit diaphragm regions which small molecule solutes are able to pass through. Injured podocytes have rounded processes and a shortened slit diaphragm resulting in decreased buttress forces and a reduction in the slit area reducing glomerular ultrafiltrate. This increases albumin permeability and allows more albumin to pass into the urine with a simultaneous reduction of small molecule filtration.
Clinical Utility of Urine Albumin Measurement
Urine albumin is used for diagnosis, risk classification, and management of therapy for chronic kidney disease (CKD) (10, 11). The National Kidney Foundation Kidney Disease Outcomes Quality Initiative (KDOQI) developed a definition of CKD that was later adopted by the Kidney Disease: Improving Global Outcomes (KDIGO) Chronic Kidney Disease (CKD) Work Group (11–14). CKD is defined as a glomerular filtration rate of less than 60 mL/min/1.73 m2 or kidney damage for a duration of 3 or more months, regardless of the cause of kidney damage. The KDIGO guidelines state that abnormalities found during urine sediment analysis, tubular disorder evaluation, histological evaluation, and imaging assessment, or a history of kidney transplant, are indicative of kidney damage. However, the presence of albuminuria is a key marker for diagnosis of kidney damage and is assessed using the albumin-to-creatinine ratio (ACR) and calculated using quantitative measurement procedures for urine albumin and urine creatinine. An ACR ≥30 mg urine albumin/g creatinine (mg/g) or 3 mg urine albumin/mmol urine creatinine (mg/mmol) for a duration of 3 or more months indicates kidney damage.
The KDIGO guidelines also incorporate albuminuria assessment with serum estimated glomerular filtration rate (eGFR) categories to provide prognostic risk classification for CKD, which is illustrated in Fig. 2. There are 3 distinct albuminuria categories provided in the KDIGO guidelines representing increasing severity of albuminuria, reported as ACR: A1, <30 mg/g (<3 mg/mmol); A2, 30 to 300 mg/g (3 to 30 mg/mmol); and A3, >300 mg/g (>30 mg/mmol). The presence of albuminuria confers increased risk of CKD progression from normal to severely decreased categories of eGFR. An ACR of <30 mg/g (<3 mg/mmol) is associated with low risk of CKD progression if eGFR is normal to mildly decreased (13, 14). An ACR of ≥30 mg/g (≥3 mg/mmol) is considered a marker for kidney damage, and the range of 30 to 300 mg/g (3 to 30 mg/mmol) is associated with moderately increased risk for CKD progression when eGFR is normal or mildly decreased. An ACR of >300 mg/g (>30 mg/mmol) is associated with high risk of CKD progression if eGFR is normal or mildly decreased. In addition to diagnosis and CKD progression risk classification, urine albumin measurements are used to guide therapy for diabetics taking angiotensin-converting enzyme inhibitors or angiotensin receptor blockers (15). Angiotensin-converting enzyme inhibitors and angiotensin receptor blockers are not recommended for patients with urine albumin <30 mg/g (<3 mg/mmol), but are recommended for patients with ≥30 mg/g (≥3 mg/mmol).
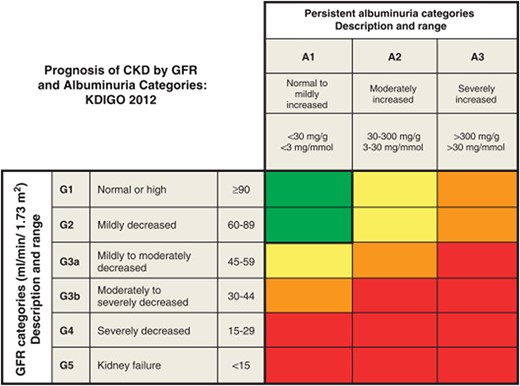
KDIGO prognosis heat map of albuminuria and eGFR classification. Green: low risk (if no other markers of kidney disease, no CKD); yellow: moderately increased risk; orange: high risk; red, very high risk. Reprinted from Kidney International Supplements, Volume 3, Issue 1, KDIGO 2012 Clinical Practice Guideline for the Evaluation and Management of Chronic Kidney Disease/Summary of Recommendation Statements, Page 6, (2013), with permission from Elsevier.
In addition to the KDOQI/KDIGO recommendations for assessment of albuminuria in kidney disease patients, professional societies including the American Diabetes Association, the European Association for the Study of Diabetes, and the National Institute of Diabetes and Digestive and Kidney Diseases (NIDDK) all recommend albuminuria screening for kidney disease in patients with diabetes mellitus (11, 12, 16, 17). These recommendations include measurement of urine albumin in type 1 diabetes 5 years following the initial diagnosis and then annually thereafter. For type 2 diabetes, measurement of urine albumin is recommended at diagnosis and then annually thereafter. The reason for this screening is the increased risk and high prevalence of kidney disease observed in diabetes, which is estimated to be between 20% and 40% (18). More frequent albuminuria screening is recommended for patients at risk for progression of kidney disease, such as patients with hypertension or cardiovascular disease (12). Increased screening frequency is also recommended for patients with evidence of increasing kidney disease severity such as the presence of persistent albuminuria, eGFR <30 mL/min/1.73 m2 or other evidence of worsening kidney damage (19).
Semiquantitative urine dipstick tests for total protein and albumin-specific dipstick tests are available for screening at the point-of-care. Although widely available, protein and ACR dipstick tests are generally less sensitive for detection of albuminuria than the measurement of the ACR using quantitative measurement procedures. In one large study, a protein dipstick result of trace or higher was associated with ACR values ≥30 mg/g (≥3 mg/mmol) with a sensitivity of only 69.4% and 86.8% specificity (20). Similarly, a meta-analysis reported pooled sensitivity and specificity of 82% and 88%, respectively, for albumin dipsticks as compared to ACR measurement (21). Performance assessment and cost/benefit evaluation of semiquantitative point-of-care ACR tests with improved sensitivity is an active area of research (22–25). These tests may become a viable option for patients at risk of CKD to self-monitor for albuminuria at home. However, in general, practice guidelines recommend use of point-of-care dipstick testing only when ACR is not available and follow-up confirmatory testing using an ACR measurement procedure is recommended (26).
Specimen Collection
The historical gold standard for assessment of albuminuria is the urine albumin excretion rate (AER) measured from a 24 h urine collection. However, several studies have shown that 24 h urine collections are subject to various pre-analytical errors ranging from laboratory mistakes in measuring the urine volume, issues with patient compliance, and incomplete urine collections, as well as problems in pediatric and other populations where patients are unable to void on demand, resulting in the need for catheterization (27, 28). The accuracy of the ACR, which can be calculated from a random or first morning void specimen, has been compared to the AER. Studies have shown ACR measurement from midstream first morning void urine collection provides better diagnostic accuracy than the AER (29–31). Therefore, evaluation of albuminuria using the ACR is recommended. The ACR calculated using first morning void specimens has been found to exhibit reduced variability as compared to random and 24 h urine specimens (31). Due to practical reasons, a midstream random urine specimen is considered acceptable for albuminuria screening if a first morning void specimen is unavailable (26). If screening is performed using a random urine specimen, ACR confirmatory testing measured from a first morning void specimen is recommended.
Specimen Stability and Storage Considerations
Although measurement of urine albumin in urine from fresh, non-frozen samples is recommended, specimens have been found to be stable for up to 8 weeks when stored refrigerated at 4°C (32). Specimens that require long-term storage for urine albumin analysis should be frozen at a temperature of −70°C or lower. Urine albumin has been found to be unstable in specimens stored at −20°C when time periods from 2 weeks to 3 years were evaluated (32, 33). Under these conditions, albumin fragments formed and the presence of the fragments likely contributed to measurement errors (33). Acceptable storage conditions should be confirmed when measuring urine albumin in specimens obtained from long-term storage, such as biobank facilities.
Reporting Recommendations
The ACR range of 30 to 300 mg/g (3 to 30 mg/mmol) was historically referred to as “microalbuminuria” and manufacturers frequently refer to their urine albumin measurement procedures as “microalbumin” assays. However, there is controversy surrounding use of the term microalbumin since it should not be confused with an abnormal small albumin molecule, nor should it be discounted as a small and clinically insignificant amount of albumin. Therefore, removal of the term “microalbuminuria” and “microalbumin” from both laboratory and clinical practice nomenclature and replacement with the terms “albuminuria” and “urine albumin” is recommended (26, 34, 35). Also, most clinical decision thresholds are applied to the ACR, therefore urine albumin results reported in mg/L are less useful reported alone. Urine albumin results should always be reported in conjunction with the ACR for evaluation of patients with CKD or at risk for CKD.
Risk of adverse kidney outcomes, cardiovascular disease, and all-cause mortality has been shown to be increased even at an ACR as low as 10 mg/g (1 mg/mmol) (36), therefore laboratories may wish to consider the lower limit of the reporting range of the analytical measurement range (AMR) when selecting measurement procedures. This level of albuminuria has been shown to impact prognosis and influence response to therapeutic interventions (14, 15). Therefore, when values exceed the upper limit of the AMR, specimens should be diluted and the result multiplied by the dilution factor. If results exceed the validated reportable range for diluted samples, the final ACR should be reported as greater than the highest valid urine albumin reporting limit divided by the creatinine result, e.g., >albumin result mg/g (>albumin result mg/mmol) (37).
Measurement Procedures
Most of the common, commercially available, clinical chemistry instrument systems include urine albumin measurement procedures. Table 1 provides a summary for select manufacturers’ instrument systems. The principles of the urine albumin measurement procedure, the measurement procedure AMRs, and the materials used for calibration traceability are shown. Immunoturbidimetric and immunonephelometric techniques are the most commonly used measurement principles for commercially available urine albumin measurement procedures due to ease of automation. These measurement principles are depicted in Fig. 3A. The principle relies on the binding of albumin in the urine by anti-albumin antibodies that are typically conjugated with a polymer. The albumin–antibody complex agglutinates, which scatters light. The scattered light is then detected using turbidimetry or nephelometry.
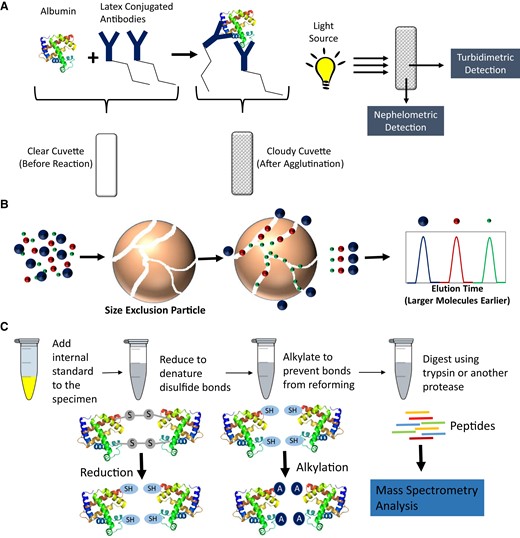
(A), Overview for common, commercially available, manufacturer's measurement procedures using immunoturbidimetry and immunonephelometry for measurement of albumin; (B), Size-exclusion chromatography theory showing how smaller molecules are able to penetrate deeper into the porous particles while the larger molecules do not and are eluted faster off the column; (C), Overview of specimen processing for bottom-up quantitative proteomic approach utilizing reduction, alkylation, and albumin digestion followed by mass spectrometry analysis for peptides.
Examples of measurement procedures and calibration traceability for routine clinical urine albumin measurement procedures.
Manufacturer/instrument system name . | Manufacturer's instructions for use (IFU)#, date . | Name of urine albumin measurement procedure . | Principle of the measurement procedure . | Analytical measurement range, mg/L . | Traceability of the calibration . |
---|---|---|---|---|---|
Abbott Architect | H15634R01, November 2020 | Microalbumin | Immunoturbidimetry | 5-500 | IRMM-ERM DA470 (CRM 470) |
Abbott Alinity | H15651R01, November 2020 | Microalbumin | Immunoturbidimetry | 5-500 | IRMM-ERM DA470 (CRM 470) |
Beckman Coulter AU | BAOSB38858 04, September 2020 | Microalbumin | Immunoturbidimetry | 7-450 | IRMM-ERM DA470 (CRM 470) |
Ortho Vitros | J21305_EN, Version 10.1 | Microalbumin | Immunoturbidimetry | 6-190 | IRMM-ERM DA470 (CRM 470) |
Roche cobas | 0208056722190c503, 2022-05, V 2.0 | Tina-quant Albumin Gen.2 | Immunoturbidimetry | 12-400 | IRMM-ERM DA470 (CRM 470) |
Siemens ADVIA XPT | 10699695_EN Rev.H, 2020-02 | Microalbumin_2 | Immunoturbidimetry | 3-380 to 420 (depending on calibrator lot) | In-house serum albumin preparation |
Siemens BN II | OSALG11E0503, 2011 | N Antiserum to Human Albumin | Immunonephelometry | 2.2-340 | IRMM-ERM DA470 (CRM 470) |
Siemens DCA Systems | 80974079 Rev. A, 2008-08 | Microalbumin/Creatinine Reagent Kit | Immunoturbidimetry | 5-300 | In-house serum albumin preparation |
Siemens Dimension | E717114.001-US, 2019-04-01 | Microalbumin, Flex reagent cartridge | Immunoturbidimetry | 1.3-100 | IRMM-ERM DA470 (CRM 470) |
Siemens Dimension Vista | 11553536_en Rev 05, 2021-11 | Microalbumin, Flex reagent cartridge | Immunonephelometry | 5-340 | IRMM-ERM DA470 (CRM 470) |
Manufacturer/instrument system name . | Manufacturer's instructions for use (IFU)#, date . | Name of urine albumin measurement procedure . | Principle of the measurement procedure . | Analytical measurement range, mg/L . | Traceability of the calibration . |
---|---|---|---|---|---|
Abbott Architect | H15634R01, November 2020 | Microalbumin | Immunoturbidimetry | 5-500 | IRMM-ERM DA470 (CRM 470) |
Abbott Alinity | H15651R01, November 2020 | Microalbumin | Immunoturbidimetry | 5-500 | IRMM-ERM DA470 (CRM 470) |
Beckman Coulter AU | BAOSB38858 04, September 2020 | Microalbumin | Immunoturbidimetry | 7-450 | IRMM-ERM DA470 (CRM 470) |
Ortho Vitros | J21305_EN, Version 10.1 | Microalbumin | Immunoturbidimetry | 6-190 | IRMM-ERM DA470 (CRM 470) |
Roche cobas | 0208056722190c503, 2022-05, V 2.0 | Tina-quant Albumin Gen.2 | Immunoturbidimetry | 12-400 | IRMM-ERM DA470 (CRM 470) |
Siemens ADVIA XPT | 10699695_EN Rev.H, 2020-02 | Microalbumin_2 | Immunoturbidimetry | 3-380 to 420 (depending on calibrator lot) | In-house serum albumin preparation |
Siemens BN II | OSALG11E0503, 2011 | N Antiserum to Human Albumin | Immunonephelometry | 2.2-340 | IRMM-ERM DA470 (CRM 470) |
Siemens DCA Systems | 80974079 Rev. A, 2008-08 | Microalbumin/Creatinine Reagent Kit | Immunoturbidimetry | 5-300 | In-house serum albumin preparation |
Siemens Dimension | E717114.001-US, 2019-04-01 | Microalbumin, Flex reagent cartridge | Immunoturbidimetry | 1.3-100 | IRMM-ERM DA470 (CRM 470) |
Siemens Dimension Vista | 11553536_en Rev 05, 2021-11 | Microalbumin, Flex reagent cartridge | Immunonephelometry | 5-340 | IRMM-ERM DA470 (CRM 470) |
Examples of measurement procedures and calibration traceability for routine clinical urine albumin measurement procedures.
Manufacturer/instrument system name . | Manufacturer's instructions for use (IFU)#, date . | Name of urine albumin measurement procedure . | Principle of the measurement procedure . | Analytical measurement range, mg/L . | Traceability of the calibration . |
---|---|---|---|---|---|
Abbott Architect | H15634R01, November 2020 | Microalbumin | Immunoturbidimetry | 5-500 | IRMM-ERM DA470 (CRM 470) |
Abbott Alinity | H15651R01, November 2020 | Microalbumin | Immunoturbidimetry | 5-500 | IRMM-ERM DA470 (CRM 470) |
Beckman Coulter AU | BAOSB38858 04, September 2020 | Microalbumin | Immunoturbidimetry | 7-450 | IRMM-ERM DA470 (CRM 470) |
Ortho Vitros | J21305_EN, Version 10.1 | Microalbumin | Immunoturbidimetry | 6-190 | IRMM-ERM DA470 (CRM 470) |
Roche cobas | 0208056722190c503, 2022-05, V 2.0 | Tina-quant Albumin Gen.2 | Immunoturbidimetry | 12-400 | IRMM-ERM DA470 (CRM 470) |
Siemens ADVIA XPT | 10699695_EN Rev.H, 2020-02 | Microalbumin_2 | Immunoturbidimetry | 3-380 to 420 (depending on calibrator lot) | In-house serum albumin preparation |
Siemens BN II | OSALG11E0503, 2011 | N Antiserum to Human Albumin | Immunonephelometry | 2.2-340 | IRMM-ERM DA470 (CRM 470) |
Siemens DCA Systems | 80974079 Rev. A, 2008-08 | Microalbumin/Creatinine Reagent Kit | Immunoturbidimetry | 5-300 | In-house serum albumin preparation |
Siemens Dimension | E717114.001-US, 2019-04-01 | Microalbumin, Flex reagent cartridge | Immunoturbidimetry | 1.3-100 | IRMM-ERM DA470 (CRM 470) |
Siemens Dimension Vista | 11553536_en Rev 05, 2021-11 | Microalbumin, Flex reagent cartridge | Immunonephelometry | 5-340 | IRMM-ERM DA470 (CRM 470) |
Manufacturer/instrument system name . | Manufacturer's instructions for use (IFU)#, date . | Name of urine albumin measurement procedure . | Principle of the measurement procedure . | Analytical measurement range, mg/L . | Traceability of the calibration . |
---|---|---|---|---|---|
Abbott Architect | H15634R01, November 2020 | Microalbumin | Immunoturbidimetry | 5-500 | IRMM-ERM DA470 (CRM 470) |
Abbott Alinity | H15651R01, November 2020 | Microalbumin | Immunoturbidimetry | 5-500 | IRMM-ERM DA470 (CRM 470) |
Beckman Coulter AU | BAOSB38858 04, September 2020 | Microalbumin | Immunoturbidimetry | 7-450 | IRMM-ERM DA470 (CRM 470) |
Ortho Vitros | J21305_EN, Version 10.1 | Microalbumin | Immunoturbidimetry | 6-190 | IRMM-ERM DA470 (CRM 470) |
Roche cobas | 0208056722190c503, 2022-05, V 2.0 | Tina-quant Albumin Gen.2 | Immunoturbidimetry | 12-400 | IRMM-ERM DA470 (CRM 470) |
Siemens ADVIA XPT | 10699695_EN Rev.H, 2020-02 | Microalbumin_2 | Immunoturbidimetry | 3-380 to 420 (depending on calibrator lot) | In-house serum albumin preparation |
Siemens BN II | OSALG11E0503, 2011 | N Antiserum to Human Albumin | Immunonephelometry | 2.2-340 | IRMM-ERM DA470 (CRM 470) |
Siemens DCA Systems | 80974079 Rev. A, 2008-08 | Microalbumin/Creatinine Reagent Kit | Immunoturbidimetry | 5-300 | In-house serum albumin preparation |
Siemens Dimension | E717114.001-US, 2019-04-01 | Microalbumin, Flex reagent cartridge | Immunoturbidimetry | 1.3-100 | IRMM-ERM DA470 (CRM 470) |
Siemens Dimension Vista | 11553536_en Rev 05, 2021-11 | Microalbumin, Flex reagent cartridge | Immunonephelometry | 5-340 | IRMM-ERM DA470 (CRM 470) |
Size-exclusion chromatography is a chromatographic approach using porous particles as the solid phase within chromatographic columns. In size-exclusion chromatography, large molecules, such as full-length proteins, cannot penetrate deep into the porous particles and therefore elute the fastest during the course of the chromatographic run. In contrast, the small molecules penetrate deeper into the porous particles causing them to elute from the column later during the chromatographic run. An overview of size-exclusion chromatographic theory is illustrated in Fig. 3B. Several studies demonstrated an increase in urine albumin when using size-exclusion chromatography compared to immunoassay measurement procedures. The researchers postulated that immunoturbidimetric and immunonephlometric measurement procedures were missing “immuno-unreactive” albumin found in clinical samples (38, 39). The “immuno-unreactive” species were theorized to consist of albumin fragments that could not be detected using immunoassay approaches. The size-exclusion chromatography approach could theoretically detect all fragments of albumin found in urine. Further studies ultimately demonstrated that the eluate from the size-exclusion measurement procedures included unresolved co-elution of other serum proteins with albumin, such as transferrin (40). These findings suggested that the theory of “immuno-unreactive” albumin is likely not the source for increased urine albumin levels observed when size-exclusion chromatography results were compared to results obtained from immunoassay-based measurement procedures.
Researchers then began seeking a gold standard measurement procedure that would exhibit high selectivity for urine albumin. Liquid chromatography–mass spectrometry (LC-MS) approaches were investigated due to the potential analytical selectivity improvements these instruments could offer compared to immunoassay and size-exclusion chromatography. One of the first approaches utilized reversed-phase liquid chromatography for separation followed by mass spectrometry using fragmentation of the albumin in the source of the mass spectrometer for specific detection and quantification (41, 42). The technique monitored the multiply charged (M + 4H) b24 fragment ion for surrogate quantitation of the intact albumin molecule. A later report by the same group compared agreement among immunoturbidimetric, size-exclusion chromatography, and LC-MS measurement procedures in clinical samples, and found −27% mean bias for the immunoturbidimetric method and +37% for the size-exclusion chromatography mean bias compared to the LC-MS method (43). However, when all measurement procedures were recalibrated using the same calibration material, the overall mean bias between the immunoturbidimetric and LC-MS measurement procedures decreased to +2.2%, but the bias between the size-exclusion approach and the LC-MS measurement procedure remained +34.4%. This finding was consistent with theory provided by Sviridov et al. that results for size-exclusion include additional co-eluting non-albumin serum proteins causing falsely elevated results (40). While studies utilizing an LC-MS measurement procedure demonstrated that urine albumin could be quantified using a measurement approach independent of antibodies, the lower limit of quantification (LLOQ) was 20 mg/L (41). Therefore, analytical sensitivity for the LC-MS measurement procedure was not sufficient for clinical use.
An alternative approach was then taken to explore “bottom-up” liquid chromatography–tandem mass spectrometry (LC-MS/MS) quantitation for albumin in urine to determine if improved analytical sensitivity could be achieved. In bottom-up LC-MS/MS measurement procedures, the full length intact protein is first digested with a protease such as trypsin prior to LC-MS/MS analysis (44). Specific peptides, unique to the human serum albumin sequence, are measured as a surrogate for quantitation of intact albumin. An overview of the bottom-up LC-MS/MS processing procedure is depicted in Fig. 3C. The full length intact albumin is first reduced to denature disulfide bonds and alkylated to prevent disulfide bonds from reforming, which improves digestion efficiency. The albumin is then digested and specific peptides are measured. Using this approach, the measurement procedure LLOQ was lowered to 3 mg/L (45). The method also employed a full length 15N-labeled human serum albumin internal standard where both the native albumin and corresponding 15N-labeled internal standard mass transitions were monitored during the analysis. The 15N-labeled human serum albumin serves as an internal standard to normalize for extraction efficiency and matrix effects, therefore decreasing variability throughout the specimen preparation processes, trypsin digestion, and LC-MS/MS analysis. Others have taken a similar approach to quantification of urine albumin using LC-MS/MS (45–47). These bottom-up quantification approaches are currently being utilized to develop candidate reference measurement procedures for standardization of urine albumin measurement procedures.
Future Needs for Urine Albumin Measurement Procedures
A study assessing agreement of 16 quantitative, commercially available, manufacturers’ measurement procedures for urine albumin compared to a candidate LC-MS/MS reference measurement procedure found a median difference range of greater than 40% for clinical samples (48). A comprehensive table showing the bias for each individual manufacturer's measurement procedure can be accessed in the referenced publication. The study determined that calibration bias, due to differences in calibration approaches or materials used for calibration traceability by measurement procedure manufacturers (Table 1), was likely to be the most significant source of the observed differences among measurement procedures. Although studies are currently ongoing to assess the influence of among-measurement procedure bias on risk of CKD misclassification and kidney disease outcomes, the lack of agreement among measurement procedures for urine albumin could potentially cause differences in detection of worsening kidney damage, depending on the specific measurement procedure utilized. Biological variation for urine albumin, expressed as an intra-individual percent coefficient of variation (%CV), has been estimated at approximately 25% (49). This estimate has been used to calculate a reference change value (RCV), or the physiologically meaningful change between 2 urine albumin measurements, of 71%. Therefore, at a urine albumin concentration of 30 mg/L, a change of 21 mg/L or greater would be considered physiologically significant. Based on the estimates of intra-individual %CV and repeatability estimates of 6% (based on state-of-the art analytical performance), bias goals of ≤13% for desirable and ≤7% for optimal bias compared to a reference measurement procedure were recommended (49). If measurement procedure agreement meets these desirable or optimal bias goals, then bias would maximally increase the RCV by 18% or 10%, respectively. Measurement procedures that fail to meet bias goals would increase the RCV by larger amounts, leading to potential misclassification of CKD risk.
A joint collaboration between the NIDDK Laboratory Working Group and the International Federation of Clinical Chemistry Working Group for Standardisation of Albumin Measurement in Urine has been working to facilitate development of formal reference systems for urine albumin (49, 50). Availability of urine albumin reference systems, comprised of reference measurement procedures, pure substance primary reference materials, and commutable secondary reference materials, will enable manufacturers to trace their calibration systems to the reference system and therefore improve agreement among the manufacturers’ routine measurement procedures (26). Once developed, the reference systems will be submitted for listing by the Joint Committee for Traceability in Laboratory Medicine (JCTLM), which evaluates candidate reference systems to ensure they meet International Organization for Standardization (ISO) international metrology standards (51). The NIST, the Mayo Clinic Renal Function Laboratory, the University of Minnesota and the Chemical Metrology Division (CML) of the Health Sciences Authority (HSA) in Singapore are working to develop liquid chromatography–mass spectrometry-based candidate reference measurement procedures for urine albumin (49, 50). The Singapore CML candidate reference measurement procedure has been published and is currently utilized for value assignment of urine albumin materials for the HSA external quality assessment program (47). The CML candidate reference measurement procedure calibration is traceable to the National Metrology Institute of Japan certified reference material 6202-a, which is listed on the JCTLM database as a primary reference material (52). In addition, the CML has developed a candidate secondary reference material that was found to be commutable with clinical samples for a routine immunoassay measurement procedure (47). The NIST has also published a candidate reference measurement procedure (46) and the NIST Standard Reference Material (SRM) 2925 Human Serum Albumin Solution has been made available for purchase (53). The NIST SRM 2925 is listed on the JCTLM database, and is currently utilized as a primary reference material for the NIST and University of Minnesota candidate reference measurement procedures. In addition, the candidate reference material NIST SRM 3666 Albumin and Creatinine in Frozen Human Urine (54), which is intended to serve as a commutable secondary reference material, is under development. Availability of the reference measurement procedures, primary, and secondary reference materials will facilitate efforts to implement metrological traceability to the higher order reference systems to improve agreement among measurement procedures for urine albumin.
Conclusions
Measurement of urine albumin is important for diagnoses, treatment, and prognosis for CKD patients. Published professional practice guidelines recommend assessment of albuminuria using the ACR measured with quantitative measurement procedures for urine albumin and creatinine. CKD patients should be tested for albuminuria annually, at minimum, or more frequently if at risk for worsening kidney damage. If random urine collections are used for screening, confirmatory testing with a first morning void collection is recommended. Albumin in urine has been shown to degrade when stored at −20°C for extended periods, therefore specimens should be frozen at −70°C or lower for long-term storage. Many clinical laboratories use commercially available immunoturbidimetric and immunonephelometric measurement procedures for measurement of urine albumin. However, lack of agreement among measurement procedures has potential to cause misclassification of CKD risk. Efforts are currently underway to achieve standardization of urine albumin measurement procedures. Once reference systems are available, the nephrology and laboratory communities should encourage commercial manufacturers to ensure their measurement procedures are traceable to reference systems.
Nonstandard Abbreviations
CKD, chronic kidney disease; ACR, urine albumin-to-creatinine ratio; KDIGO, Kidney Disease: Improving Global Outcomes; eGFR, estimated glomerular filtration rate; CML, Chemical Metrology Division.
Author Contributions
The corresponding author takes full responsibility that all authors on this publication have met the following required criteria of eligibility for authorship: (a) significant contributions to the conception and design, acquisition of data, or analysis and interpretation of data; (b) drafting or revising the article for intellectual content; (c) final approval of the published article; and (d) agreement to be accountable for all aspects of the article thus ensuring that questions related to the accuracy or integrity of any part of the article are appropriately investigated and resolved. Nobody who qualifies for authorship has been omitted from the list.
Jesse Seegmiller (Conceptualization-Lead, Data curation-Equal, Investigation-Equal, Methodology-Equal), and Lorin Bachmann (Data curation-Equal, Investigation-Equal, Methodology-Equal).
Authors’ Disclosures or Potential Conflicts of Interest
Upon manuscript submission, all authors completed the author disclosure form.
Research Funding
None declared.
Disclosures
J.C. Seegmiller has received National Institutes of Health and Centers for Disease Control and Prevention subcontracts for laboratory work; participates in the HIV in Nigeria (H3) Data and Safety Monitoring Board; and is Chair, International Federation of Clinical Chemistry and Laboratory Medicine, Working Group: Standardization of Albumin in Urine (IFCC WG-SAU). L.M. Bachmann is a Member, International Federation of Clinical Chemistry and Laboratory Medicine, Working Group: Standardization of Albumin in Urine (IFCC WG-SAU).
References
Joint Committee for Traceability in Laboratory Medicine (JCTLM). A global resource for traceability in laboratory medicine and in vitro diagnostics.