-
PDF
- Split View
-
Views
-
Cite
Cite
Yingdi Liu, Miaomiao Chen, Jing Liu, Aiping Mao, Yanling Teng, Huiming Yan, Huimin Zhu, Zhuo Li, Desheng Liang, Lingqian Wu, Comprehensive Analysis of Congenital Adrenal Hyperplasia Using Long-Read Sequencing, Clinical Chemistry, Volume 68, Issue 7, July 2022, Pages 927–939, https://doi.org/10.1093/clinchem/hvac046
- Share Icon Share
Abstract
Congenital adrenal hyperplasia (CAH) is an autosomal recessive disorder that has been included in newborn screening programs. Current approaches to gene testing for CAH are facing challenges because of the complexity of the CYP21A2 locus and genetic heterogeneity of the disease.
A comprehensive analysis of CAH (CACAH) combining long-range locus-specific PCR and long-read sequencing (LRS) was developed to perform full sequence analysis of 5 common CAH candidate genes, including CYP21A2, CYP11B1, CYP17A1, HSD3B2, and StAR. In a blind retrospective study, the clinical utility of CACAH was evaluated in 37 samples by comparing to standard CAH testing using multiplex ligation-dependent probe amplification (MLPA) plus Sanger sequencing.
Of the 37 clinical samples, a total of 69 pathogenic variants were identified, comprising 65 CYP21A2 variants, 2 HSD3B2 variants, and 2 CYP17A1 variants. For CYP21A2, the most frequent variant was c.518T > A (29.2%), followed by c.293−13C/A > G (21.5%). Compared with the current CAH testing using MLPA plus Sanger sequencing, the CACAH assay showed 100% specificity and 100% sensitivity, and precisely determined the junction sites of deletions/insertions and cis–trans configuration of multiple variants without analyzing family samples. Moreover, CACAH identified a case carrying 2 copies of CYP21A1 with the c.1451_1452delinsC variant on the same chromosome, which was not confirmed by MLPA plus Sanger sequencing.
LRS-based CACAH can determine all genotypes of CAH accurately and reliably in one assay, presenting a comprehensive approach for CAH genetic diagnosis and carrier screening.
Introduction
Congenital adrenal hyperplasia (CAH) is a group of autosomal recessive disorders caused by a deficiency of essential enzymes in the adrenal steroidogenesis pathway (1). About 95% of CAH cases are attributable to 21-hydroxylase deficiency (21-OHD) characterized by cortisol deficiency, with or without androgen excess and aldosterone deficiency (2). The clinical presentations of 21-OHD depend on the genotype and the residual enzymatic activity (3). According to clinical severity, 21-OHD is classified into 3 subtypes: classic salt-wasting, classic simple virilizing, and non-classic (mild or late onset) (4). The incidence of classic CAH ranges from 1:10 000 to 1:20 000, with a carrier frequency of 1:50 to 1:71 in the general population (5).
The 21-OHD arises from mutations of the CYP21A2 gene on chromosome 6p21.3 that cause a deficiency of 21-hydroxylase (6). The corresponding pseudogene CYP21A1P is also mapped to this region and at approximately 30 kb distance from CYP21A2. Both the functional CYP21A2 gene and the non-functional CYP21A1P pseudogene contain 10 exons and 9 introns, sharing approximately 98% sequence identity in the exons and 96% homology in the introns (7). From telomere to centromere, serine/threonine kinase RP, complement C4, steroid 21-hydroxylase CYP21, and tenascin TNX genes are arranged in tandem, forming a genetic module termed ‘RCCX’ (RP-C4-CYP21-TNX) (8). In Caucasians, bimodular RCCX is present in about 69%, while monomodular and trimodular RCCX account for about 17% and 14%, respectively (9). Due to the high homology and tandem repeating between the functional genes (RP1, CYP21A2, and TNXB) and the corresponding pseudogene (RP2, CYP21A1P, and TNXA), misalignment may occur during meiosis, resulting in gene conversions, unequal crossovers, gene deletions, and the formation of nonfunctional chimeric genes. Chimeric genes are categorized into two groups, CYP21A1P/CYP21A2 chimeras and TNXA/TNXB chimeras, based on the location of the junction sites. CYP21A1P/CYP21A2 chimeras are further classified into classic chimeras (such as CH-1, CH-2, CH-3, CH-5, CH-6, CH-7, and CH-8) and attenuated chimeras (such as CH-4 and CH-9). TNXA/TNXB chimeras, also known as CAH-X, are subdivided into three types: CAH-X CH-1, CH-2, and CH-3 (10). Currently, over 200 pathogenic variants of CYP21A2 gene have been identified in humans (11), of which approximately 75% are due to the microconversion of the CYP21A1P pseudogene mutation, 20% to 25% are large deletions or chimeric genes, and 1% to 2% are de novo mutations (3, 12, 13).
Neonatal screening for CAH based on hormonal testing is performed in many countries (1), with false-positive rates varying between 0.4% and 9.3% and the inability to detect non-classic CAH and carrier state accurately (14). Genetic analysis of CAH is essential for timely diagnosis and treatment while providing appropriate genetic counseling and prenatal diagnosis. Methods for genetic analyses include multiplex ligation-dependent probe amplification (MLPA), Sanger sequencing, Southern blot analysis (SBA), and quantitative polymerase chain reaction (qPCR) (6, 15–17). Most laboratories identify known and common pathogenic variants for CAH by using MLPA plus Sanger sequencing, although there still exist noteworthy limitations. For example, MLPA is extremely sensitive to the quality of DNA, probe binding, and small changes in experimental manipulation, and may have false- negative/positive results in some cases such as mutations/polymorphisms that locate in the probe-binding region or duplication-masked deletion. With MLPA it is also difficult to determine the phase of deletion/duplication/microconversion and distinguish attenuated chimeras CH-4 and CH-9 from class chimera CH-6 (4, 18). In addition, MLPA plus Sanger is unable to directly determine the cis- or trans-configuration if two or more variants exist.
More recently, next-generation sequencing (NGS) and long-read sequencing (LRS) techniques are becoming valuable tools for molecular diagnostic approaches (19–21). NGS-based detection targeting a panel of candidate genes has shown promise for application in CAH, but the complexities of the assay still remain, including the requirement for multiple PCR reactions and library preparations before sequencing and a follow-up MLPA test to exclude structure variation (20, 22–24). Moreover, with NGS detection it is difficult to discriminate the variants among homologous genes and pseudogenes, and the method is inapplicable to high-repeat genomic regions (6, 25). In comparison to NGS, LRS has some advantages in genetic testing of rare diseases, especially for those involving complex genotypes, owing to its much longer sequencing reads (21, 26). LRS can sequence the full length of genes dozens of kilobases long in single reads, making it straightforward to read the whole gene and discriminate between highly homologous genes like CYP21A1P and CYP21A2. By using multiplex long-range PCR, LRS can directly sequence deletions/duplications and large gene conversions, which are not reliably determined by NGS-based methods. In addition, LRS can easily detect the cis- and trans-configuration of 2 or more variants without the need for genotypic analysis of family members like parents.
Here, in combination of a long-range locus-specific PCR, we developed an LRS-based assay to analyze almost all kinds of variants simultaneously in the target candidate genes and achieve a comprehensive genetic analysis of CAH. With a retrospective analysis on 37 clinical samples, we demonstrated that our comprehensive analysis of CAH (CACAH) holds substantial promise in clinical application as a universal approach for CAH genotyping and carrier testing.
Materials and Methods
Study Subjects
Dried blood spot (DBS) samples were obtained by collecting the heel blood of well-fed newborns on Whatman 903 filter papers 72 h after birth and dried at room temperature. Peripheral blood samples were collected into EDTA tubes and genomic DNA was prepared with the QIAamp DNA blood mini kit (QIAGEN). Genomic DNA was quantitated using the Qubit dsDNA BR assay kit (ThermoFisher Scientific) with a Qubit 2.0 fluorometer (ThermoFisher Scientific). Fourteen DBS and 23 genomic DNA samples, including a complete family of 4 with an affected proband, were selected for this study from the diagnostic laboratory, and all the other 33 samples had at least one pathogenic mutation detected by Sanger sequencing and SALSA MLPA Probemix P050-C1 CAH (MRC-Holland). The CAH study was approved by the Ethics Committee of the School of Life Sciences, Central South University (Approval number 2016102101). Written informed consent for the CAH genetic test was given by all the patients or their legal guardians. Clinical forms of CAH due to 21-OHD were determined based on clinical and hormonal criteria (Table 1).
Concordance of CACAH vs MPLA plus Sanger sequencing assay for CAH genetic test.
Name . | Type . | Phenotype . | CACAH reads . | . | CACAH . | MLPA + Sanger genotype . | ||||
---|---|---|---|---|---|---|---|---|---|---|
A2 . | A1P . | Deletion . | aGC . | Affected gene . | Genotype . | Junction region . | ||||
GX01 | DBS | − | + | + | + | − | CYP21A2 | e30-KB Del-CH-1/c.293-13C/A > G | chr6: 32039133-32039426 | 30-KB Del-CH-1/c.293-13C/A > G |
GX02 | DBS | − | + | + | − | − | CYP21A2 | c.293-13C/A > G/c.853G > C | hNA | c.293-13C/A > G/c.853G > C |
GX03 | DBS | − | + | + | − | − | CYP21A2 | c.518T > A/c.518T > A | NA | c.518T > A/c.518T > A |
GX04 | DBS | − | + | + | − | − | CYP21A2 | c.518T > A/c.955C > T | NA | c.518T > A/c.955C > T |
GX06 | DBS | − | + | + | − | − | CYP21A2 | c.293-13C/A > G/c.518T > A | NA | c.293-13C/A > G/c.518T > A |
GX07 | DBS | − | + | + | − | − | CYP21A2 | c.293-13C/A > G/c.518T > A | NA | c.293-13C/A > G/c.518T > A |
GX08 | DBS | − | + | + | − | − | CYP21A2 | c.293-13C/A > G/c.518T > A | NA | c.293-13C/A > G/c.518T > A |
GX09 | DBS | − | + | + | + | − | CYP21A2 | 30-KB Del-CH-1/c.92C > T | chr6: 32039133-32039426 | 30-KB Del-CH-1/c.92C > T |
GX10 | DBS | − | + | + | − | − | CYP21A2 | c.293-13C/A > G/c.1279C > T | NA | c.293-13C/A > G/c.1279C > T |
GX11 | DBS | − | + | + | − | − | CYP21A2 | c.92C > T, c.188A > T/c.518T > A | NA | c.92C > T, c.188A > T/c.518T > A |
GX12 | DBS | − | + | + | + | − | CYP21A2 | 30-KB DEL-CH-1/c.293-13C/A > G | chr6: 32039133-32039426 | 30-KB DEL-CH-1/c.293-13C/A > G |
GX13 | DBS | − | + | + | − | − | CYP21A2 | c.518T > A/c.844G > T | NA | c.518T > A/c.844G > T |
GX14 | DBS | − | + | + | − | − | CYP21A2 | c.293-13C/A > G/c.518T > A | NA | c.293-13C/A > G/c.518T > A |
MD06757 | gDNA | bSW | + | + | + | − | CYP21A2 | fDEL-CH-1/c.293-13C > G | chr6: 32043901-32045077 | DEL-CH-1/c.293-13C > G |
MD06772 | gDNA | SW | + | + | + | − | CYP21A2 | 30-KB DEL-CH-1/c.1069C > T | chr6: 32039133-32039426 | 30-KB DEL-CH-1/c.1069C > T |
MD06797 | gDNA | cSV | + | + | − | − | CYP21A2 | c.332_339del/c.518T > A | NA | c.332_339del/c.518T > A |
MD07475 | gDNA | SW | + | + | + | − | CYP21A2 | DEL-CH-1/c.293-13C/A > G | chr6: 32043901-32045077 | DEL-CH-1/c.293-13C/A > G |
MD12141 | gDNA | SW | + | + | + | − | CYP21A2 | DEL-CH-1/c.844G > T, c. 923dup, c.955C > T, c.1069C > T | chr6: 32043901-32045077 | DEL-CH-1/c.844G > T, c. 923dup, c.955C > T, c.1069C > T |
MD12707 | gDNA | dN | + | + | − | − | CYP21A2 | c.955C > T/+ | NA | c.955C > T/+ |
MD12708 | gDNA | N | + | + | − | − | CYP21A2 | c.293-13C/A > G/+ | NA | c.293-13C/A > G/+ |
MD15928 | gDNA | SV | + | + | − | − | CYP21A2 | c.293-13C/A > G/c.518T > A | NA | c.293-13C/A > G/c.518T > A |
MD19756 | gDNA | SV | + | + | − | − | CYP21A2 | c.518T > A/c.518T > A | NA | c.518T > A/c.518T > A |
MD19757 | gDNA | SV | + | + | − | − | CYP21A2 | c.518T > A/c.518T > A | NA | c.518T > A/c.518T > A |
MD21578 | gDNA | SV | + | + | − | − | CYP21A2 | c.293-13C/A > G/c.518T > A | NA | c.293-13C/A > G/c.518T > A |
MD21581 | gDNA | SV | + | + | + | − | CYP21A2 | DEL-CH-1/c.518T > A | chr6: 32043901-32045077 | DEL-CH-1/c.518T > A |
MD22138 | gDNA | SW | + | + | + | − | CYP21A2 | 30-KB DEL-CH-1/c.1069C > T | chr6: 32039133-32039426 | 30-KB DEL-CH-1/c.1069C > T |
MD22305 | gDNA | SV | + | + | − | − | CYP21A2 | c.332_339del/c.518T > A | NA | c.332_339del/c.518T > A |
MD22910 | gDNA | SV | + | + | + | − | CYP21A2 | 30-KB Del-CH-6/gE4-E10 mutations | chr6: 32038911-32038938 | 30-KB Del-CH-6/E4-E10 mutations |
MD23162 | gDNA | SW | − | + | + | − | CYP21A2 | DEL-CH-1/DEL-CH-2 | chr6: 32043901-32045077/ chr6: 32042485-32043718 | DEL-CH-1/30-KB Del or Del |
MD23172 | gDNA | SV | + | + | + | − | CYP21A2 | DEL-CH-1/c.518T > A | chr6: 32043862-32043901 | DEL-CH-1/c.518T > A |
MD24365 | gDNA | SW | + | + | + | − | CYP21A2 | 30-KB Del-CH-1/c.293-13C/A > G | chr6: 32039133-32039426 | 30-KB Del-CH-1/c.293-13C/A > G |
MD25557 (Mother) | gDNA | N | + | + | − | + | CYP21A2 | CYP21A2 triplicate; c.1451_1452delinsC, c.1451_1452delinsC/+ | NA | CYP21A2 triplicate; c.1451_1452delinsC/+ |
MD26418 (Father) | gDNA | N | + | + | − | − | CYP21A2 | c.1025G > C/+ | NA | c.1025G > C/+ |
MD26419 (Son, proband) | gDNA | SW | + | + | − | + | CYP21A2 | CYP21A2 triplicate; c.1451_1452delinsC, c.1451_1452delinsC/ | NA | CYP21A2 triplicate; c.1451_1452delinsC / c.1025G>C |
MD26420 (Daughter) | gDNA | N | + | + | − | − | CYP21A2 | c.1025G > C/+ | NA | c.1025G > C/+ |
GX05 | DBS | − | + | + | − | − | HSD3B2 | c.674T > A/c.857C > T | NA | c.674T > A/c.857C > T |
MD20782 | gDNA | − | + | + | − | − | CYP17A1 | c.985_987delinsAA/c.1459-1467del | NA | c.985_987delinsAA/c.1459-1467del |
Name . | Type . | Phenotype . | CACAH reads . | . | CACAH . | MLPA + Sanger genotype . | ||||
---|---|---|---|---|---|---|---|---|---|---|
A2 . | A1P . | Deletion . | aGC . | Affected gene . | Genotype . | Junction region . | ||||
GX01 | DBS | − | + | + | + | − | CYP21A2 | e30-KB Del-CH-1/c.293-13C/A > G | chr6: 32039133-32039426 | 30-KB Del-CH-1/c.293-13C/A > G |
GX02 | DBS | − | + | + | − | − | CYP21A2 | c.293-13C/A > G/c.853G > C | hNA | c.293-13C/A > G/c.853G > C |
GX03 | DBS | − | + | + | − | − | CYP21A2 | c.518T > A/c.518T > A | NA | c.518T > A/c.518T > A |
GX04 | DBS | − | + | + | − | − | CYP21A2 | c.518T > A/c.955C > T | NA | c.518T > A/c.955C > T |
GX06 | DBS | − | + | + | − | − | CYP21A2 | c.293-13C/A > G/c.518T > A | NA | c.293-13C/A > G/c.518T > A |
GX07 | DBS | − | + | + | − | − | CYP21A2 | c.293-13C/A > G/c.518T > A | NA | c.293-13C/A > G/c.518T > A |
GX08 | DBS | − | + | + | − | − | CYP21A2 | c.293-13C/A > G/c.518T > A | NA | c.293-13C/A > G/c.518T > A |
GX09 | DBS | − | + | + | + | − | CYP21A2 | 30-KB Del-CH-1/c.92C > T | chr6: 32039133-32039426 | 30-KB Del-CH-1/c.92C > T |
GX10 | DBS | − | + | + | − | − | CYP21A2 | c.293-13C/A > G/c.1279C > T | NA | c.293-13C/A > G/c.1279C > T |
GX11 | DBS | − | + | + | − | − | CYP21A2 | c.92C > T, c.188A > T/c.518T > A | NA | c.92C > T, c.188A > T/c.518T > A |
GX12 | DBS | − | + | + | + | − | CYP21A2 | 30-KB DEL-CH-1/c.293-13C/A > G | chr6: 32039133-32039426 | 30-KB DEL-CH-1/c.293-13C/A > G |
GX13 | DBS | − | + | + | − | − | CYP21A2 | c.518T > A/c.844G > T | NA | c.518T > A/c.844G > T |
GX14 | DBS | − | + | + | − | − | CYP21A2 | c.293-13C/A > G/c.518T > A | NA | c.293-13C/A > G/c.518T > A |
MD06757 | gDNA | bSW | + | + | + | − | CYP21A2 | fDEL-CH-1/c.293-13C > G | chr6: 32043901-32045077 | DEL-CH-1/c.293-13C > G |
MD06772 | gDNA | SW | + | + | + | − | CYP21A2 | 30-KB DEL-CH-1/c.1069C > T | chr6: 32039133-32039426 | 30-KB DEL-CH-1/c.1069C > T |
MD06797 | gDNA | cSV | + | + | − | − | CYP21A2 | c.332_339del/c.518T > A | NA | c.332_339del/c.518T > A |
MD07475 | gDNA | SW | + | + | + | − | CYP21A2 | DEL-CH-1/c.293-13C/A > G | chr6: 32043901-32045077 | DEL-CH-1/c.293-13C/A > G |
MD12141 | gDNA | SW | + | + | + | − | CYP21A2 | DEL-CH-1/c.844G > T, c. 923dup, c.955C > T, c.1069C > T | chr6: 32043901-32045077 | DEL-CH-1/c.844G > T, c. 923dup, c.955C > T, c.1069C > T |
MD12707 | gDNA | dN | + | + | − | − | CYP21A2 | c.955C > T/+ | NA | c.955C > T/+ |
MD12708 | gDNA | N | + | + | − | − | CYP21A2 | c.293-13C/A > G/+ | NA | c.293-13C/A > G/+ |
MD15928 | gDNA | SV | + | + | − | − | CYP21A2 | c.293-13C/A > G/c.518T > A | NA | c.293-13C/A > G/c.518T > A |
MD19756 | gDNA | SV | + | + | − | − | CYP21A2 | c.518T > A/c.518T > A | NA | c.518T > A/c.518T > A |
MD19757 | gDNA | SV | + | + | − | − | CYP21A2 | c.518T > A/c.518T > A | NA | c.518T > A/c.518T > A |
MD21578 | gDNA | SV | + | + | − | − | CYP21A2 | c.293-13C/A > G/c.518T > A | NA | c.293-13C/A > G/c.518T > A |
MD21581 | gDNA | SV | + | + | + | − | CYP21A2 | DEL-CH-1/c.518T > A | chr6: 32043901-32045077 | DEL-CH-1/c.518T > A |
MD22138 | gDNA | SW | + | + | + | − | CYP21A2 | 30-KB DEL-CH-1/c.1069C > T | chr6: 32039133-32039426 | 30-KB DEL-CH-1/c.1069C > T |
MD22305 | gDNA | SV | + | + | − | − | CYP21A2 | c.332_339del/c.518T > A | NA | c.332_339del/c.518T > A |
MD22910 | gDNA | SV | + | + | + | − | CYP21A2 | 30-KB Del-CH-6/gE4-E10 mutations | chr6: 32038911-32038938 | 30-KB Del-CH-6/E4-E10 mutations |
MD23162 | gDNA | SW | − | + | + | − | CYP21A2 | DEL-CH-1/DEL-CH-2 | chr6: 32043901-32045077/ chr6: 32042485-32043718 | DEL-CH-1/30-KB Del or Del |
MD23172 | gDNA | SV | + | + | + | − | CYP21A2 | DEL-CH-1/c.518T > A | chr6: 32043862-32043901 | DEL-CH-1/c.518T > A |
MD24365 | gDNA | SW | + | + | + | − | CYP21A2 | 30-KB Del-CH-1/c.293-13C/A > G | chr6: 32039133-32039426 | 30-KB Del-CH-1/c.293-13C/A > G |
MD25557 (Mother) | gDNA | N | + | + | − | + | CYP21A2 | CYP21A2 triplicate; c.1451_1452delinsC, c.1451_1452delinsC/+ | NA | CYP21A2 triplicate; c.1451_1452delinsC/+ |
MD26418 (Father) | gDNA | N | + | + | − | − | CYP21A2 | c.1025G > C/+ | NA | c.1025G > C/+ |
MD26419 (Son, proband) | gDNA | SW | + | + | − | + | CYP21A2 | CYP21A2 triplicate; c.1451_1452delinsC, c.1451_1452delinsC/ | NA | CYP21A2 triplicate; c.1451_1452delinsC / c.1025G>C |
MD26420 (Daughter) | gDNA | N | + | + | − | − | CYP21A2 | c.1025G > C/+ | NA | c.1025G > C/+ |
GX05 | DBS | − | + | + | − | − | HSD3B2 | c.674T > A/c.857C > T | NA | c.674T > A/c.857C > T |
MD20782 | gDNA | − | + | + | − | − | CYP17A1 | c.985_987delinsAA/c.1459-1467del | NA | c.985_987delinsAA/c.1459-1467del |
aGC, large gene conversion; bSW, salt-wasting; cSV, simple virilizing; dN, normal; e30-KB DEL-CH-1, a 30-kb deletion and the formation of chimeric genes of CYP21A1P/CYP21A2; fDEL-CH-1, a 30-kb deletion and the formation of chimeric genes of TNXA/TNXB; gE4-E10 mutations, the presence of several mutations from exon 4 to exon10 of CYP21A2 gene; hNA, not applicable.
Concordance of CACAH vs MPLA plus Sanger sequencing assay for CAH genetic test.
Name . | Type . | Phenotype . | CACAH reads . | . | CACAH . | MLPA + Sanger genotype . | ||||
---|---|---|---|---|---|---|---|---|---|---|
A2 . | A1P . | Deletion . | aGC . | Affected gene . | Genotype . | Junction region . | ||||
GX01 | DBS | − | + | + | + | − | CYP21A2 | e30-KB Del-CH-1/c.293-13C/A > G | chr6: 32039133-32039426 | 30-KB Del-CH-1/c.293-13C/A > G |
GX02 | DBS | − | + | + | − | − | CYP21A2 | c.293-13C/A > G/c.853G > C | hNA | c.293-13C/A > G/c.853G > C |
GX03 | DBS | − | + | + | − | − | CYP21A2 | c.518T > A/c.518T > A | NA | c.518T > A/c.518T > A |
GX04 | DBS | − | + | + | − | − | CYP21A2 | c.518T > A/c.955C > T | NA | c.518T > A/c.955C > T |
GX06 | DBS | − | + | + | − | − | CYP21A2 | c.293-13C/A > G/c.518T > A | NA | c.293-13C/A > G/c.518T > A |
GX07 | DBS | − | + | + | − | − | CYP21A2 | c.293-13C/A > G/c.518T > A | NA | c.293-13C/A > G/c.518T > A |
GX08 | DBS | − | + | + | − | − | CYP21A2 | c.293-13C/A > G/c.518T > A | NA | c.293-13C/A > G/c.518T > A |
GX09 | DBS | − | + | + | + | − | CYP21A2 | 30-KB Del-CH-1/c.92C > T | chr6: 32039133-32039426 | 30-KB Del-CH-1/c.92C > T |
GX10 | DBS | − | + | + | − | − | CYP21A2 | c.293-13C/A > G/c.1279C > T | NA | c.293-13C/A > G/c.1279C > T |
GX11 | DBS | − | + | + | − | − | CYP21A2 | c.92C > T, c.188A > T/c.518T > A | NA | c.92C > T, c.188A > T/c.518T > A |
GX12 | DBS | − | + | + | + | − | CYP21A2 | 30-KB DEL-CH-1/c.293-13C/A > G | chr6: 32039133-32039426 | 30-KB DEL-CH-1/c.293-13C/A > G |
GX13 | DBS | − | + | + | − | − | CYP21A2 | c.518T > A/c.844G > T | NA | c.518T > A/c.844G > T |
GX14 | DBS | − | + | + | − | − | CYP21A2 | c.293-13C/A > G/c.518T > A | NA | c.293-13C/A > G/c.518T > A |
MD06757 | gDNA | bSW | + | + | + | − | CYP21A2 | fDEL-CH-1/c.293-13C > G | chr6: 32043901-32045077 | DEL-CH-1/c.293-13C > G |
MD06772 | gDNA | SW | + | + | + | − | CYP21A2 | 30-KB DEL-CH-1/c.1069C > T | chr6: 32039133-32039426 | 30-KB DEL-CH-1/c.1069C > T |
MD06797 | gDNA | cSV | + | + | − | − | CYP21A2 | c.332_339del/c.518T > A | NA | c.332_339del/c.518T > A |
MD07475 | gDNA | SW | + | + | + | − | CYP21A2 | DEL-CH-1/c.293-13C/A > G | chr6: 32043901-32045077 | DEL-CH-1/c.293-13C/A > G |
MD12141 | gDNA | SW | + | + | + | − | CYP21A2 | DEL-CH-1/c.844G > T, c. 923dup, c.955C > T, c.1069C > T | chr6: 32043901-32045077 | DEL-CH-1/c.844G > T, c. 923dup, c.955C > T, c.1069C > T |
MD12707 | gDNA | dN | + | + | − | − | CYP21A2 | c.955C > T/+ | NA | c.955C > T/+ |
MD12708 | gDNA | N | + | + | − | − | CYP21A2 | c.293-13C/A > G/+ | NA | c.293-13C/A > G/+ |
MD15928 | gDNA | SV | + | + | − | − | CYP21A2 | c.293-13C/A > G/c.518T > A | NA | c.293-13C/A > G/c.518T > A |
MD19756 | gDNA | SV | + | + | − | − | CYP21A2 | c.518T > A/c.518T > A | NA | c.518T > A/c.518T > A |
MD19757 | gDNA | SV | + | + | − | − | CYP21A2 | c.518T > A/c.518T > A | NA | c.518T > A/c.518T > A |
MD21578 | gDNA | SV | + | + | − | − | CYP21A2 | c.293-13C/A > G/c.518T > A | NA | c.293-13C/A > G/c.518T > A |
MD21581 | gDNA | SV | + | + | + | − | CYP21A2 | DEL-CH-1/c.518T > A | chr6: 32043901-32045077 | DEL-CH-1/c.518T > A |
MD22138 | gDNA | SW | + | + | + | − | CYP21A2 | 30-KB DEL-CH-1/c.1069C > T | chr6: 32039133-32039426 | 30-KB DEL-CH-1/c.1069C > T |
MD22305 | gDNA | SV | + | + | − | − | CYP21A2 | c.332_339del/c.518T > A | NA | c.332_339del/c.518T > A |
MD22910 | gDNA | SV | + | + | + | − | CYP21A2 | 30-KB Del-CH-6/gE4-E10 mutations | chr6: 32038911-32038938 | 30-KB Del-CH-6/E4-E10 mutations |
MD23162 | gDNA | SW | − | + | + | − | CYP21A2 | DEL-CH-1/DEL-CH-2 | chr6: 32043901-32045077/ chr6: 32042485-32043718 | DEL-CH-1/30-KB Del or Del |
MD23172 | gDNA | SV | + | + | + | − | CYP21A2 | DEL-CH-1/c.518T > A | chr6: 32043862-32043901 | DEL-CH-1/c.518T > A |
MD24365 | gDNA | SW | + | + | + | − | CYP21A2 | 30-KB Del-CH-1/c.293-13C/A > G | chr6: 32039133-32039426 | 30-KB Del-CH-1/c.293-13C/A > G |
MD25557 (Mother) | gDNA | N | + | + | − | + | CYP21A2 | CYP21A2 triplicate; c.1451_1452delinsC, c.1451_1452delinsC/+ | NA | CYP21A2 triplicate; c.1451_1452delinsC/+ |
MD26418 (Father) | gDNA | N | + | + | − | − | CYP21A2 | c.1025G > C/+ | NA | c.1025G > C/+ |
MD26419 (Son, proband) | gDNA | SW | + | + | − | + | CYP21A2 | CYP21A2 triplicate; c.1451_1452delinsC, c.1451_1452delinsC/ | NA | CYP21A2 triplicate; c.1451_1452delinsC / c.1025G>C |
MD26420 (Daughter) | gDNA | N | + | + | − | − | CYP21A2 | c.1025G > C/+ | NA | c.1025G > C/+ |
GX05 | DBS | − | + | + | − | − | HSD3B2 | c.674T > A/c.857C > T | NA | c.674T > A/c.857C > T |
MD20782 | gDNA | − | + | + | − | − | CYP17A1 | c.985_987delinsAA/c.1459-1467del | NA | c.985_987delinsAA/c.1459-1467del |
Name . | Type . | Phenotype . | CACAH reads . | . | CACAH . | MLPA + Sanger genotype . | ||||
---|---|---|---|---|---|---|---|---|---|---|
A2 . | A1P . | Deletion . | aGC . | Affected gene . | Genotype . | Junction region . | ||||
GX01 | DBS | − | + | + | + | − | CYP21A2 | e30-KB Del-CH-1/c.293-13C/A > G | chr6: 32039133-32039426 | 30-KB Del-CH-1/c.293-13C/A > G |
GX02 | DBS | − | + | + | − | − | CYP21A2 | c.293-13C/A > G/c.853G > C | hNA | c.293-13C/A > G/c.853G > C |
GX03 | DBS | − | + | + | − | − | CYP21A2 | c.518T > A/c.518T > A | NA | c.518T > A/c.518T > A |
GX04 | DBS | − | + | + | − | − | CYP21A2 | c.518T > A/c.955C > T | NA | c.518T > A/c.955C > T |
GX06 | DBS | − | + | + | − | − | CYP21A2 | c.293-13C/A > G/c.518T > A | NA | c.293-13C/A > G/c.518T > A |
GX07 | DBS | − | + | + | − | − | CYP21A2 | c.293-13C/A > G/c.518T > A | NA | c.293-13C/A > G/c.518T > A |
GX08 | DBS | − | + | + | − | − | CYP21A2 | c.293-13C/A > G/c.518T > A | NA | c.293-13C/A > G/c.518T > A |
GX09 | DBS | − | + | + | + | − | CYP21A2 | 30-KB Del-CH-1/c.92C > T | chr6: 32039133-32039426 | 30-KB Del-CH-1/c.92C > T |
GX10 | DBS | − | + | + | − | − | CYP21A2 | c.293-13C/A > G/c.1279C > T | NA | c.293-13C/A > G/c.1279C > T |
GX11 | DBS | − | + | + | − | − | CYP21A2 | c.92C > T, c.188A > T/c.518T > A | NA | c.92C > T, c.188A > T/c.518T > A |
GX12 | DBS | − | + | + | + | − | CYP21A2 | 30-KB DEL-CH-1/c.293-13C/A > G | chr6: 32039133-32039426 | 30-KB DEL-CH-1/c.293-13C/A > G |
GX13 | DBS | − | + | + | − | − | CYP21A2 | c.518T > A/c.844G > T | NA | c.518T > A/c.844G > T |
GX14 | DBS | − | + | + | − | − | CYP21A2 | c.293-13C/A > G/c.518T > A | NA | c.293-13C/A > G/c.518T > A |
MD06757 | gDNA | bSW | + | + | + | − | CYP21A2 | fDEL-CH-1/c.293-13C > G | chr6: 32043901-32045077 | DEL-CH-1/c.293-13C > G |
MD06772 | gDNA | SW | + | + | + | − | CYP21A2 | 30-KB DEL-CH-1/c.1069C > T | chr6: 32039133-32039426 | 30-KB DEL-CH-1/c.1069C > T |
MD06797 | gDNA | cSV | + | + | − | − | CYP21A2 | c.332_339del/c.518T > A | NA | c.332_339del/c.518T > A |
MD07475 | gDNA | SW | + | + | + | − | CYP21A2 | DEL-CH-1/c.293-13C/A > G | chr6: 32043901-32045077 | DEL-CH-1/c.293-13C/A > G |
MD12141 | gDNA | SW | + | + | + | − | CYP21A2 | DEL-CH-1/c.844G > T, c. 923dup, c.955C > T, c.1069C > T | chr6: 32043901-32045077 | DEL-CH-1/c.844G > T, c. 923dup, c.955C > T, c.1069C > T |
MD12707 | gDNA | dN | + | + | − | − | CYP21A2 | c.955C > T/+ | NA | c.955C > T/+ |
MD12708 | gDNA | N | + | + | − | − | CYP21A2 | c.293-13C/A > G/+ | NA | c.293-13C/A > G/+ |
MD15928 | gDNA | SV | + | + | − | − | CYP21A2 | c.293-13C/A > G/c.518T > A | NA | c.293-13C/A > G/c.518T > A |
MD19756 | gDNA | SV | + | + | − | − | CYP21A2 | c.518T > A/c.518T > A | NA | c.518T > A/c.518T > A |
MD19757 | gDNA | SV | + | + | − | − | CYP21A2 | c.518T > A/c.518T > A | NA | c.518T > A/c.518T > A |
MD21578 | gDNA | SV | + | + | − | − | CYP21A2 | c.293-13C/A > G/c.518T > A | NA | c.293-13C/A > G/c.518T > A |
MD21581 | gDNA | SV | + | + | + | − | CYP21A2 | DEL-CH-1/c.518T > A | chr6: 32043901-32045077 | DEL-CH-1/c.518T > A |
MD22138 | gDNA | SW | + | + | + | − | CYP21A2 | 30-KB DEL-CH-1/c.1069C > T | chr6: 32039133-32039426 | 30-KB DEL-CH-1/c.1069C > T |
MD22305 | gDNA | SV | + | + | − | − | CYP21A2 | c.332_339del/c.518T > A | NA | c.332_339del/c.518T > A |
MD22910 | gDNA | SV | + | + | + | − | CYP21A2 | 30-KB Del-CH-6/gE4-E10 mutations | chr6: 32038911-32038938 | 30-KB Del-CH-6/E4-E10 mutations |
MD23162 | gDNA | SW | − | + | + | − | CYP21A2 | DEL-CH-1/DEL-CH-2 | chr6: 32043901-32045077/ chr6: 32042485-32043718 | DEL-CH-1/30-KB Del or Del |
MD23172 | gDNA | SV | + | + | + | − | CYP21A2 | DEL-CH-1/c.518T > A | chr6: 32043862-32043901 | DEL-CH-1/c.518T > A |
MD24365 | gDNA | SW | + | + | + | − | CYP21A2 | 30-KB Del-CH-1/c.293-13C/A > G | chr6: 32039133-32039426 | 30-KB Del-CH-1/c.293-13C/A > G |
MD25557 (Mother) | gDNA | N | + | + | − | + | CYP21A2 | CYP21A2 triplicate; c.1451_1452delinsC, c.1451_1452delinsC/+ | NA | CYP21A2 triplicate; c.1451_1452delinsC/+ |
MD26418 (Father) | gDNA | N | + | + | − | − | CYP21A2 | c.1025G > C/+ | NA | c.1025G > C/+ |
MD26419 (Son, proband) | gDNA | SW | + | + | − | + | CYP21A2 | CYP21A2 triplicate; c.1451_1452delinsC, c.1451_1452delinsC/ | NA | CYP21A2 triplicate; c.1451_1452delinsC / c.1025G>C |
MD26420 (Daughter) | gDNA | N | + | + | − | − | CYP21A2 | c.1025G > C/+ | NA | c.1025G > C/+ |
GX05 | DBS | − | + | + | − | − | HSD3B2 | c.674T > A/c.857C > T | NA | c.674T > A/c.857C > T |
MD20782 | gDNA | − | + | + | − | − | CYP17A1 | c.985_987delinsAA/c.1459-1467del | NA | c.985_987delinsAA/c.1459-1467del |
aGC, large gene conversion; bSW, salt-wasting; cSV, simple virilizing; dN, normal; e30-KB DEL-CH-1, a 30-kb deletion and the formation of chimeric genes of CYP21A1P/CYP21A2; fDEL-CH-1, a 30-kb deletion and the formation of chimeric genes of TNXA/TNXB; gE4-E10 mutations, the presence of several mutations from exon 4 to exon10 of CYP21A2 gene; hNA, not applicable.
Sanger Sequencing and MLPA Analysis of CAH Genes
Primers were designed to cover the exon and exon-intron boundary regions of CYP21A2, CYP11B1, CYP17A1, HSD3B2, and StAR genes. For CYP21A2, the primers were designed to discriminate CYP21A2 and its pseudogene CYP21A1P, so that the sequencing results would only contain CYP21A2. PCR was performed using the designed primers on isolated DNA samples and the PCR product was purified for Sanger sequencing. SALSA MLPA Probemix P050-C1 CAH was used for copy number and gene conversion analyses. If two or more mutations were detected, cis–trans configurations were determined by analysis of family samples. The results of Sanger sequencing and MLPA were used collaboratively to do the genotype interpretation.
Third Generation Sequencing of CAH Gene
To cover the entire gene regions for CYP21A2, CYP11B1, CYP17A1, HSD3B2, and StAR genes, a total of 6 long-range PCR (LR-PCR) fragments were designed (Fig. 1, A). Primers were optimized to produce amplicons of similar sizes (7.0 to 8.5 kb) to minimize the difference in loading capacity and sequencing efficiency. DBS or genomics DNA samples were subjected to multiplex LR-PCR using KOD FX Neo (TOYOBO). PCR cycling conditions for optimal fragment amplification were 94 °C for 2 min (1 cycle); 98 °C for 10 s and 68 °C for 10 min (30 cycles), and 68 °C for 10 min (1 cycle). The PCR products were purified with 1× Ampure PB beads (Pacific Biosciences), quantified with Qubit dsDNA BR assay kit and confirmed by agarose gel electrophoresis for successful amplification.
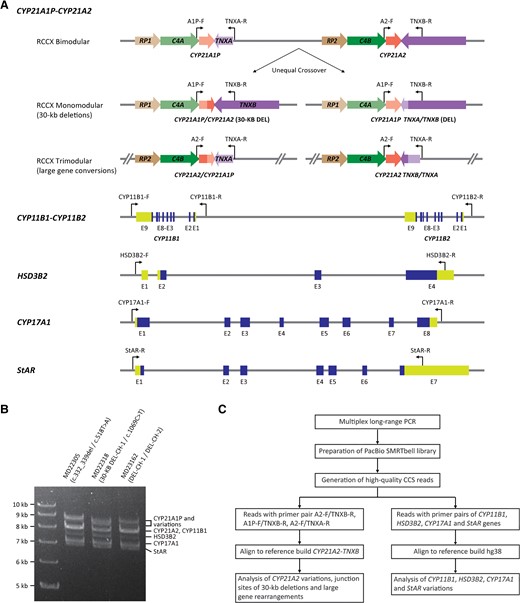
Strategy for CACAH. (A), Position of multiplex PCR primer pairs for long-range PCR; (B), agarose gel showing the expected amplicons for different CAH genes; (C), customized bioinformatics pipeline for variation analysis.
The construction of pre-sequencing single-molecule real-time (SMRT) libraries was performed as previously described with some modifications (21, 26). Briefly, for each purified PCR product, a one-step end-repair and ligation reaction was done to add a unique barcoded-adaptor. The 10 µl of reaction mix contained 4 µl of PCR product, 5 µmol/L barcoded adaptor (Integrated DNA Technologies), 1× T4 DNA ligase buffer (Enzymatics), 1 mmol/L ATP (New England Biolabs), 200 μmol/L dNTP (New England Biolabs), 2.5 units of T4 polynucleotide kinase (Enzymatics), 0.75 units of T4 DNA polymerase (Enzymatics) and 180 units of T4 DNA ligase (HC) (Enzymatics). Reaction mixes were incubated at 37 °C for 20 min, 25 °C for 15 min and 65 °C for 10 min. The failed ligation products were removed by exonuclease I (Enzymatics) and exonuclease III (Enzymatics). The pre-library was purified with 0.6× Ampure PB beads and quantified with Qubit dsDNA HS assay kit (ThermoFisher Scientific). Equal masses of uniquely barcoded pre-libraries were pooled together, purified with 0.6× Ampure PB beads and quantified with Qubit dsDNA HS assay kit.
The SMRT bell library was prepared using the Sequel Binding Kit 2.0 and Internal Control Kit 1.0 (Pacific Biosciences). Primed DNA-polymerase complexes were loading onto SMRT cells and sequenced with Sequel II Sequencing Kit 2.0 on the Sequel II platform (Pacific Biosciences).
Data Analysis and DNA Variant Calling
The circular consensus sequencing (CCS) software (Pacific Biosciences) was used to create CCS reads from the raw subreads in the BAM file output from the PacBio Sequel II platform. Then CCS reads were demultiplexed and the barcode sequences were clipped using lima in the Pbbioconda package (Pacific Biosciences). The debarcoded and filtered CCS reads were then aligned to human reference genome build hg38 using pbmn2 to specifically get the target CYP11B1, HSD3B, CYP17A1, and StAR gene fragments. FreeBayes1.3.4 was used for identification of single nucleotide variations (SNVs) and small insertions and deletions (indels). For analysis of CYP21A2 and CYP21A1P, reads with primer pair A1P-F/TNXA-R, A2-F/TNXB-R, A1P-F/TNXB-R, and A2-F/TNXA-R were assigned as pseudogene CYP21A1P (A1P), functional gene CYP21A2 (A2), 30-kb deletion CYP21A1P/CYP21A2 chimera (A1P/A2), and large gene conversion CYP21A2/CYP21A1P chimera (A2/A1P), respectively. The reads of a given primer pair were considered as background if the ratio was less than 2% among the total number of CYP21A2 and CYP21A1P reads. Pseudogene A1P reads were eliminated for further analysis. Two reference builds were made to better study the junction site of A1P/A2 and A2/A1P reads. The first reference build CYP21A2-TNXB contained genome sequence hg38 chr6:32037179-32046548, and the second reference build CYP21A1P-TNXA contained genome sequence hg38 chr6:32004843-32013648. The reference build CYP21A1P-TNXA was aligned to reference build CYP21A2-TNXB using pbmn2 and FreeBayes1.3.4 was used to identify CYP21A1P-TNXA signature SNV/indels. A2, A1P/A2, and A2/A1P reads were aligned to reference build CYP21A2-TNXB for SNV/indels analysis using pbmn2 and FreeBayes1.3.4, and haplotype was analyzed using Whatshap. The junction site of A1P/A2 and A2/A1P reads were determined by the distribution of CYP21A1P-TNXA signature SNV/indels in the reads. The pathogenic variants were displayed and confirmed in the Integrative Genomics Viewer (IGV) program with BAM files.
Results
Establishment of CACAH Assay
The CACAH assay was designed to sequence CAH candidate genes by LRS technology based on the PacBio Sequel II platform. A long-range locus-specific PCR approach was developed to specifically amplify CYP21A2, CYP21A1P, CYP11B1, HSD3B2, CYP17A1, and StAR (Fig. 1, A). To comprehensively analyze the small gene conversions in CYP21A2, 30-kb deletions, and large gene conversions caused by unequal crossover between CYP21A1P and CYP21A2, or between TNXA and TNXB, a 4-primer system was designed to amplify all the possible mutations.
Specific forward primers were designed in the promoter regions for CYP21A2 and CYP21A1P pseudogene, and specific reverse primers were designed on the divergence of the TNXB and TNXA gene sequences located downstream of CYP21A2 and CYP21A1P genes, respectively. A reverse primer was also designed in the promoter region of CYP11B2 to target rare forms of CYP11B2/CYP11B1 chimera that might cause CAH. Validation of the multiplex LR-PCR test was performed on genomic DNA samples with known CYP21A2 genotypes (Fig. 1, B). The amplified PCR products were subjected to PacBio SMRTbell library preparation, sequencing, and analyzing using a customized variant calling pipeline (Fig. 1, C).
CACAH Assay for CAH Samples
A total of 14 DBS samples and 23 genomic DNA samples from peripheral blood with genotypes detected by MLPA and Sanger sequencing in the diagnostic laboratory of the Center for Medical Genetics, Central South University (Hunan, China) were selected and sent to an independent laboratory (Berry Genomics, Beijing, China) for blind CACAH assay. Analysis of the CCS reads obtained from all the samples showed that the allelic coverage was highly qualified with mean depth of 6683 (range 144 to 28 574; see online Supplemental Table 1), which enabled confident identification of pathogenic variants.
CACAH genotyping identified 35 samples with CYP21A2 variants, one sample with HSD3B2 variants on both alleles, and one sample with CYP17A1 variants on both alleles (Table 1). Among the 35 carriers with CYP21A2 variants, 16 samples had SNVs or indels on both alleles, 12 samples had a 30-kb deletion on one allele and SNV/indels on the other allele, 4 samples were heterozygous for SNV/indels, 1 sample had 30-kb deletions on both alleles, and 2 samples had complex large gene conversion. In total, 46 alleles with SNV/indels of 10 types, 14 alleles with 30-kb deletions of 4 types, and 5 alleles with complex variations were identified (Table 2). The most frequent variation was c.518T > A (29.2%, 19/65), followed by c.293-13C/A > G (21.5%, 14/65), 30-KB DEL-CH-1 (9.2%, 6/65), and DEL-CH-1 (9.2%, 6/65). The IGV plots of selected samples were displayed to directly show the SNV/indels of CYP21A2, HSD3B2, and CYP17A1, as well as 30-kb deletions in CYP21A2 (Fig. 2, A, B, E, and F). Interestingly, sample MD12141 had 4 pathogenic mutations in exon 7-exon 8, and sample MD22190 had multiple mutations expanded from exon 4 to exon 10, which could result from multiple recombination between CYP21A2 and CYP21A1P (Fig. 2, C and D). Compared to a standard CAH test with MLPA plus Sanger sequencing, the CACAH assay demonstrated 100% sensitivity and specificity while improving the resolution on the junction site of 30-kb deletions and large gene conversions (Table 1).
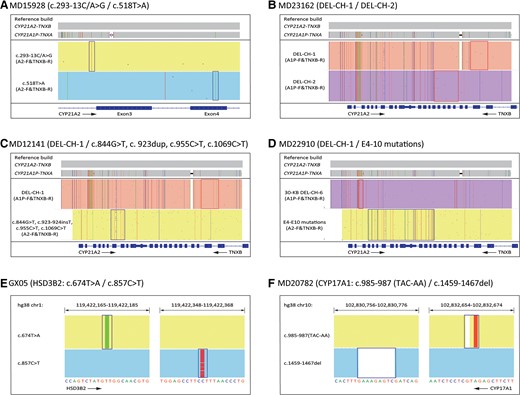
IGV plots of CACAH results showing variations of CAH genes. (A), Representative sample with CYP21A2 pathogenic SNV/indels; (B), representative sample with CYP21A2 gene deletions; (C), sample MD12141 with CYP21A2 complex variations; (D), sample MD22910 with CYP21A2 complex variations; (E), sample GX05 with HSD3B2 gene variations; (F), sample MD20782 with CYP17A1 gene variations. The boxes highlighted the pathogenic SNV/indels identified in figure A, E, and F, lower panel of figure C and D, and the boxes indicated junction sites of 30-kb deletions in figure B, upper panel of figure C and D.
Gene . | Type of variation . | Nucleotide variation . | Protein variation . | Allele number . |
---|---|---|---|---|
CYP21A2 | SNV/indels | c.518T > A | p.Ile173Asn | 19/65 (29.2%) |
c.293-13C/A > G | dNA | 14/65 (21.5%) | ||
c.1025G > C | p.Arg342Pro | 3/65 (4.6%) | ||
c.332_339del | p.Gly111Valfs | 2/65 (3.1%) | ||
c.955C > T | p.Gln319Ter | 2/65 (3.1%) | ||
c.1069C > T | p.Arg357Trp | 2/65 (3.1%) | ||
c.92C > T | p.Pro31Leu | 1/65 (1.5%) | ||
c.844G > T | p.Val282Leu | 1/65 (1.5%) | ||
c.853G > C | p.Ala285Pro | 1/65 (1.5%) | ||
c.1279C > T | p.Arg427Cys | 1/65 (1.5%) | ||
30-kb deletions | a30-KB DEL-CH-1 | NA | 6/65 (9.2%) | |
bDEL-CH-1 | NA | 6/65 (9.2%) | ||
30-KB DEL-CH-6 | NA | 1/65 (1.5%) | ||
DEL-CH-2 | NA | 1/65 (1.5%) | ||
Complex variations | Large gene convertion (c.1451_1452delinsC, c.1451_1452delinsC) | p.Arg484fs | 2/65 (3.1%) | |
cE4-E10 mutations | NA | 1/65 (1.5%) | ||
c.844G > T, c.923dup, c.955C > T, c.1069C > T | p.Val282Leu, p.L308fs | 1/65 (1.5%) | ||
c.92C > T, c.188A > T | p.Pro31Leu, p.His63Leu | 1/65 (1.5%) | ||
HSD3B2 | SNV/indels | c.674T > A | p.Val225Asp | 1/2 (50%) |
c.857C > T | p.Pro286Leu | 1/2 (50%) | ||
CYP17A1 | SNV/indels | c.985_987delinsAA | p.Tyr329fs | 1/2 (50%) |
c.1459_1467del | p.Asp487_Phe489del | 1/2 (50%) |
Gene . | Type of variation . | Nucleotide variation . | Protein variation . | Allele number . |
---|---|---|---|---|
CYP21A2 | SNV/indels | c.518T > A | p.Ile173Asn | 19/65 (29.2%) |
c.293-13C/A > G | dNA | 14/65 (21.5%) | ||
c.1025G > C | p.Arg342Pro | 3/65 (4.6%) | ||
c.332_339del | p.Gly111Valfs | 2/65 (3.1%) | ||
c.955C > T | p.Gln319Ter | 2/65 (3.1%) | ||
c.1069C > T | p.Arg357Trp | 2/65 (3.1%) | ||
c.92C > T | p.Pro31Leu | 1/65 (1.5%) | ||
c.844G > T | p.Val282Leu | 1/65 (1.5%) | ||
c.853G > C | p.Ala285Pro | 1/65 (1.5%) | ||
c.1279C > T | p.Arg427Cys | 1/65 (1.5%) | ||
30-kb deletions | a30-KB DEL-CH-1 | NA | 6/65 (9.2%) | |
bDEL-CH-1 | NA | 6/65 (9.2%) | ||
30-KB DEL-CH-6 | NA | 1/65 (1.5%) | ||
DEL-CH-2 | NA | 1/65 (1.5%) | ||
Complex variations | Large gene convertion (c.1451_1452delinsC, c.1451_1452delinsC) | p.Arg484fs | 2/65 (3.1%) | |
cE4-E10 mutations | NA | 1/65 (1.5%) | ||
c.844G > T, c.923dup, c.955C > T, c.1069C > T | p.Val282Leu, p.L308fs | 1/65 (1.5%) | ||
c.92C > T, c.188A > T | p.Pro31Leu, p.His63Leu | 1/65 (1.5%) | ||
HSD3B2 | SNV/indels | c.674T > A | p.Val225Asp | 1/2 (50%) |
c.857C > T | p.Pro286Leu | 1/2 (50%) | ||
CYP17A1 | SNV/indels | c.985_987delinsAA | p.Tyr329fs | 1/2 (50%) |
c.1459_1467del | p.Asp487_Phe489del | 1/2 (50%) |
a30-KB DEL-CH-1, a 30-kb deletion and the formation of chimeric genes of CYP21A1P/CYP21A2; bDEL-CH-1, a 30-kb deletion and the formation of chimeric genes of TNXA/TNXB; cE4-E10 mutations, the presence of several mutations from exon 4 to exon10 of CYP21A2 gene; dNA, not applicable.
Gene . | Type of variation . | Nucleotide variation . | Protein variation . | Allele number . |
---|---|---|---|---|
CYP21A2 | SNV/indels | c.518T > A | p.Ile173Asn | 19/65 (29.2%) |
c.293-13C/A > G | dNA | 14/65 (21.5%) | ||
c.1025G > C | p.Arg342Pro | 3/65 (4.6%) | ||
c.332_339del | p.Gly111Valfs | 2/65 (3.1%) | ||
c.955C > T | p.Gln319Ter | 2/65 (3.1%) | ||
c.1069C > T | p.Arg357Trp | 2/65 (3.1%) | ||
c.92C > T | p.Pro31Leu | 1/65 (1.5%) | ||
c.844G > T | p.Val282Leu | 1/65 (1.5%) | ||
c.853G > C | p.Ala285Pro | 1/65 (1.5%) | ||
c.1279C > T | p.Arg427Cys | 1/65 (1.5%) | ||
30-kb deletions | a30-KB DEL-CH-1 | NA | 6/65 (9.2%) | |
bDEL-CH-1 | NA | 6/65 (9.2%) | ||
30-KB DEL-CH-6 | NA | 1/65 (1.5%) | ||
DEL-CH-2 | NA | 1/65 (1.5%) | ||
Complex variations | Large gene convertion (c.1451_1452delinsC, c.1451_1452delinsC) | p.Arg484fs | 2/65 (3.1%) | |
cE4-E10 mutations | NA | 1/65 (1.5%) | ||
c.844G > T, c.923dup, c.955C > T, c.1069C > T | p.Val282Leu, p.L308fs | 1/65 (1.5%) | ||
c.92C > T, c.188A > T | p.Pro31Leu, p.His63Leu | 1/65 (1.5%) | ||
HSD3B2 | SNV/indels | c.674T > A | p.Val225Asp | 1/2 (50%) |
c.857C > T | p.Pro286Leu | 1/2 (50%) | ||
CYP17A1 | SNV/indels | c.985_987delinsAA | p.Tyr329fs | 1/2 (50%) |
c.1459_1467del | p.Asp487_Phe489del | 1/2 (50%) |
Gene . | Type of variation . | Nucleotide variation . | Protein variation . | Allele number . |
---|---|---|---|---|
CYP21A2 | SNV/indels | c.518T > A | p.Ile173Asn | 19/65 (29.2%) |
c.293-13C/A > G | dNA | 14/65 (21.5%) | ||
c.1025G > C | p.Arg342Pro | 3/65 (4.6%) | ||
c.332_339del | p.Gly111Valfs | 2/65 (3.1%) | ||
c.955C > T | p.Gln319Ter | 2/65 (3.1%) | ||
c.1069C > T | p.Arg357Trp | 2/65 (3.1%) | ||
c.92C > T | p.Pro31Leu | 1/65 (1.5%) | ||
c.844G > T | p.Val282Leu | 1/65 (1.5%) | ||
c.853G > C | p.Ala285Pro | 1/65 (1.5%) | ||
c.1279C > T | p.Arg427Cys | 1/65 (1.5%) | ||
30-kb deletions | a30-KB DEL-CH-1 | NA | 6/65 (9.2%) | |
bDEL-CH-1 | NA | 6/65 (9.2%) | ||
30-KB DEL-CH-6 | NA | 1/65 (1.5%) | ||
DEL-CH-2 | NA | 1/65 (1.5%) | ||
Complex variations | Large gene convertion (c.1451_1452delinsC, c.1451_1452delinsC) | p.Arg484fs | 2/65 (3.1%) | |
cE4-E10 mutations | NA | 1/65 (1.5%) | ||
c.844G > T, c.923dup, c.955C > T, c.1069C > T | p.Val282Leu, p.L308fs | 1/65 (1.5%) | ||
c.92C > T, c.188A > T | p.Pro31Leu, p.His63Leu | 1/65 (1.5%) | ||
HSD3B2 | SNV/indels | c.674T > A | p.Val225Asp | 1/2 (50%) |
c.857C > T | p.Pro286Leu | 1/2 (50%) | ||
CYP17A1 | SNV/indels | c.985_987delinsAA | p.Tyr329fs | 1/2 (50%) |
c.1459_1467del | p.Asp487_Phe489del | 1/2 (50%) |
a30-KB DEL-CH-1, a 30-kb deletion and the formation of chimeric genes of CYP21A1P/CYP21A2; bDEL-CH-1, a 30-kb deletion and the formation of chimeric genes of TNXA/TNXB; cE4-E10 mutations, the presence of several mutations from exon 4 to exon10 of CYP21A2 gene; dNA, not applicable.
CACAH Assay for Analysis of Large Gene Conversion
This study enrolled a family of 4 including the young son, also the proband MD26419, the father MD26418, the mother MD25557, and the older daughter MD26420. MLPA analysis suggested that the proband had 3 copies of CYP21A2 (Fig. 3, A), and Sanger sequencing showed the sample was heterozygous for c.1025G > C and heterozygous for c.1451_1452delinsC variations (Fig. 3, B). The father had 2 copies of CYP21A2 and carried heterozygous c.1025G > C variation, while the mother had 3 copies of CYP21A1 and carried heterozygous c.1451_1452delinsC variation (Fig. 3, B). With the help of family analysis, it was confirmed that the proband had one copy of CYP21A2 with c.1025G > C variation, however, it was not clear whether only one or both of the other two copies of CYP21A2 had c.1451_1452delinsC variation (Fig. 3). CACAH analysis of the proband clearly showed that the additional CYP21A2 copy was caused by large gene conversion and 2 copies of CYP21A2 had c.1451_1452delinsC variation (Fig. 4, A). With family analysis, it was confirmed that the allele with c.1025G > C variation was inherited from the father, and the allele with two c.1451_1452delinsC variations was inherited from the mother (Fig. 4, B and C). The older sister had 2 copies of CYP21A2 and heterozygous c.1025G > C variation (Fig. 4, D). The duplicated CYP21A2 genes identified in the proband and mother were not associated with p. (Gln318*).
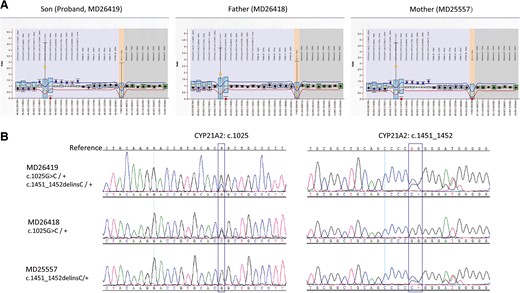
MLPA and Sanger sequencing analysis for the family samples. (A), MLPA analysis of CYP21A2 locus for the proband and parents; (B), Sanger sequencing of CYP21A2 gene for the proband and parents.
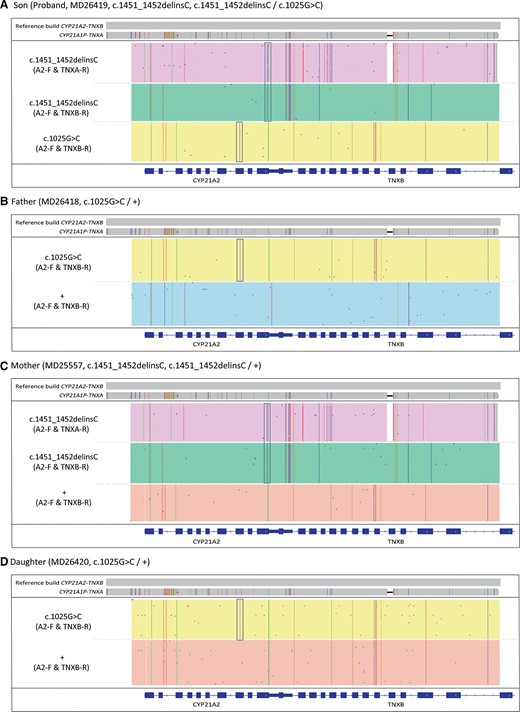
IGV plots of CACAH results of the family samples. (A), Proband MD26419; (B), father MD26418; (C), mother MD25557; (D), sister MD26420. The boxes highlighted the pathogenic SNV/indels identified in CYP21A2.
Discussion
CAH, one of the most common autosomal recessive disorders, has high clinical and genetic heterogeneity and severely affects the patient’s quality of life. Neonatal screening for CAH had been implemented successfully in regions of the world and it has effectively reduced mortality and alleviated disease symptoms. However, substantial limitations including high false-positive rates remain in conventional biochemical-based screening approaches (5, 27–29). To enhance the specificity and clinical applicability of CAH screening, molecular genetic analysis has been proposed as a second-tier test (24, 30–32).
Several approaches were available for genetic diagnosis of CAH, although combination of different techniques is required to obtain accurate genotypes (6, 15, 16). In this study, we first applied LRS as a diagnostic test of CAH and identified 69 variants including 65 CYP21A2 variants, 2 HSD3B2 variants, and 2 CYP17A1 variants in 37 clinical samples. For CYP21A2, the most frequent variant was c.518T > A (29.2%, 19/65), followed by c.293−13C/A > G (21.5%, 14/65), which is consistent with a previous study that tested CAH candidate genes using NGS (23). As a control method, MLPA plus Sanger sequencing assay confirmed a concordance of 100% with CACAH for the 69 variants in our cohort. Of note, CACAH detected 3 copies of CYP21A1 in proband MD26419 and his mother MD25557, and determined that the proband inherited one copy of CYP21A2 with c.1025G > C mutation from his father and 2 copies of CYP21A2 with c.1451_1452delinsC variant from his mother. Meanwhile, CACAH clarified that the duplicated CYP21A2 genes in the proband and mother were not associated with p. (Gln318*) (6, 33, 34). In this case, MLPA plus Sanger sequencing was unable to determine whether both copies on the same chromosome harbored the c.1451_1452delinsC variant. Furthermore, CACAH accurately determined the junction site of 30-kb deletion and large gene conversions. These results demonstrated that CACAH achieves genotyping of CAH accurately and reliably. Additionally, the clinical presentations and molecular characteristic of 17 patients with peripheral blood samples support the genotype–phenotype correlation, and in general the most serious clinical manifestations were associated with CYP21A2 variants of large deletions or conversions. By using CACAH, a precise and prompt genotyping for CAH could confirm the diagnosis and carrier state, facilitating valuable phenotype prediction, personalized treatment, and appropriate genetic counseling.
Compared with the current approaches, CACAH in the present study has proved to provide a more comprehensive, accurate, and efficient approach for CAH genotyping. LRS-based CACAH enables a direct sequencing of various variations, including point mutations, small deletions/insertions, large deletions/duplications, and gene conversions. This is especially useful for detection of complex structural variants, such as chimeric CYP21A1P/CYP21A2 genes and CAH-X chimeras. It has been proposed that the CAH-X chimeras should also be included in the molecular genetic testing of CAH (35). However, existing approaches are limited to a set of common and known variants and have unavoidable limitations in detecting chimeric genes. CACAH could directly determine if more than one duplication allele is on the same chromosome, accurately distinguishing the affected patients from carriers. In addition, LRS assay can directly use genomic DNA, peripheral blood, or DBS as a PCR template, particularly suitable for genetic screening of CAH. Importantly, with increased throughput, reductions in LRS costs, and an expanded test panel of genetic disorders such as comprehensive analysis of thalassemia alleles (CATSA) in the PacBio platform (21), CACAH will have many clinical applications in the future. Currently, with the PacBio Sequel II and IIe platform and powerful barcode system, it is possible to test 384 samples for CATSA or CACAH in one flow cell and obtain high enough sequencing depth at the same time, making the overall cost less than $20 USD per test (21, 26). The cost of LRS-based tests for other monogenic diseases would depend on the number of PCR fragments and whether the amplicons would cause bias amplification that could create uneven sequencing coverage. For other monogenic diseases that can be tested using 2 to 5 PCR fragments, we expect the cost would be similar to CATSA or CACAH. Moreover, with the expected reductions of LRS cost overtime, LRS-based tests for genetic diseases will become even more cost-effective in the future.
Nonetheless, the present study has certain limitations. CACAH covered 5 CAH genes that caused the vast majority of CAH. However, it is technically possible to expand CACAH to include other clinically significant genes. Another limitation is that we were unable to retrieve clinical symptoms in 14 patients who provided DBS samples owing to treatment beginning immediately after diagnosis. Further, this was a retrospective study with a limited sample size and the advantages of CACAH were not fully highlighted. Thus, a large-scale and multi-center prospective study is needed to most fully explore the advantages of CACAH.
Conclusion
The LRS-based CACAH assay can achieve precise genotyping of the CAH candidate genes in one step, facilitating a prompt molecular diagnosis, carrier screening, and appropriate genetic counseling or prenatal diagnosis. With the rapid development of the LRS technique, LRS-based detection holds promise as a crucial tool for molecular diagnostics in the near future.
Supplemental Material
Supplemental material is available at Clinical Chemistry online.
Nonstandard Abbreviations
CAH, congenital adrenal hyperplasia; CACAH, comprehensive analysis of CAH; LRS, long-read sequencing; MLPA, multiplex ligation-dependent probe amplification; 21-OHD, 21-hydroxylase deficiency; NGS, next-generation sequencing; DBS, dried blood spot; CCS, circular consensus sequencing; SNV, single nucleotide variation; indels, small insertions and deletions.
Human Genes
CYP21A2, cytochrome P450 family 21 subfamily A member 2; CYP11B1, cytochrome P450 family 11 subfamily B member 1; CYP17A1, cytochrome P450 family 17 subfamily A member 1; HSD3B2, hydroxy-delta-5-steroid dehydrogenase 3 beta- and steroid delta-isomerase 2; StAR, steroidogenic acute regulatory protein; CYP21A1P, cytochrome P450 family 21 subfamily A member 1 (pseudogene); RP1, RP1 axonemal microtubule associated; C4A, complement C4A (Rodgers blood group); TNXA, tenascin XA (pseudogene); RP2, RP2 activator of ARL3 GTPase; C4B, complement C4B (Chido blood group); TNXB, tenascin XB; CYP11A1, cytochrome P450 family 11 subfamily A member 1.
Author Contributions
All authors confirmed they have contributed to the intellectual content of this paper and have met the following 4 requirements: (a) significant contributions to the conception and design, acquisition of data, or analysis and interpretation of data; (b) drafting or revising the article for intellectual content; (c) final approval of the published article; and (d) agreement to be accountable for all aspects of the article thus ensuring that questions related to the accuracy or integrity of any part of the article are appropriately investigated and resolved.
Authors’ Disclosures or Potential Conflicts of Interest
Upon manuscript submission, all authors completed the author disclosure form. Disclosures and/or potential conflicts of interest:
Employment or Leadership
None declared.
Consultant or Advisory Role
None declared.
Stock Ownership
None declared.
Honoraria
None declared.
Research Funding
This work was supported by the National Key Research and Development Program of China (2021YFC1005300, 2017YFC1001802), the Science and Technology Innovation Program of Hunan Province (2019SK1010), and the National Natural Science Foundation of China (81771599, 81974240) (L. Wu).
Expert Testimony
None declared.
Patents
None declared.
Role of Sponsor
The funding organizations played no role in the design of study, choice of enrolled patients, review and interpretation of data, preparation of manuscript, or final approval of manuscript.
Acknowledgments
We thank all subjects who participated in this study.
References
Author notes
Yingdi Liu, Miaomiao Chen, and Jing Liu contributed equally to this work.