-
PDF
- Split View
-
Views
-
Cite
Cite
Yuki Kotani, Martina Baiardo Redaelli, Alessandro Pruna, Rosario Losiggio, Susanna Cocozza, Lian Kah Ti, Nikola Bradic, Marco Comis, Giovanni Landoni, Rinaldo Bellomo, Intravenous amino acid for kidney protection: current understanding and future perspectives, Clinical Kidney Journal, Volume 18, Issue 2, February 2025, sfae409, https://doi.org/10.1093/ckj/sfae409
- Share Icon Share
ABSTRACT
Acute kidney injury (AKI) is a common complication in critically ill and perioperative patients and is associated with mortality, morbidity, medical costs, and progression to chronic kidney function. Unfortunately, despite numerous research efforts, until recently, there was no AKI preventive therapy supported by level 1 evidence. Among the several factors that contribute to renal damage, two of the major triggers of AKI development are renal hypoperfusion and renal medullary hypoxia. The intravenous administration of a mixture of amino acids promotes the prevention of AKI through multiple mechanisms: the recruitment of renal functional reserve, increased renal blood flow, and improvements in renal oxygenation. Such mechanisms of action led to increased glomerular filtration rate and urine output in preclinical and pilot clinical studies. To test if these benefits on physiological parameters could be translated into clinically meaningful outcomes, a multicenter, randomized, placebo-controlled, trial was conducted in the cardiac surgery setting. Among 3511 adult patients undergoing elective cardiac surgery with cardiopulmonary bypass, intravenous amino acid administration, compared to placebo, significantly reduced the occurrence of AKI, providing the first level 1 evidence of an effective treatment for AKI prevention. In this review, we provide the epidemiology and pathophysiology of cardiac surgery-associated AKI and the concept of renal functional reserve. Then, we summarize the underlying mechanisms of intravenous amino acid infusion as a renoprotective strategy and its preclinical and clinical evidence. Finally, we discuss the existing evidence gaps and future directions of this promising intervention.
INTRODUCTION
Acute kidney injury (AKI) is a common and serious complication in patients suffering from critical illness and undergoing major cardiac or non-cardiac surgery. Traditionally, its incidence ranges widely depending on the population investigated and definition used. However, after the initial international consensus criteria [Risk, Injury, Failure, Loss of kidney function, and End-stage kidney disease (RIFLE)] [1], followed by Acute Kidney Injury Network (AKIN) [2], the current definition and diagnostic criteria were established by the Kidney Disease: Improving Global Outcomes (KDIGO) criteria, where AKI is defined as an abrupt decrease in kidney function and diagnosed with two functional markers, that is serum creatinine and urine output [3] (Table 1).
Acute kidney injury staging according to the Kidney Disease Improving Global Outcomes criteria [3].
Stage . | Serum creatinine criteria . | Urine output criteria . |
---|---|---|
1 | 1.50–1.9 times baseline 0.3 mg/dl increase | <0.5 ml/kg/h for 6–12 h |
2 | 2.0–2.9 times baseline | <0.5 ml/kg/h for ≥12 h |
3 | 3.0 times baseline Increase in serum creatinine to ≥4.0 mg/dl Initiation of renal replacement therapy Decrease to eGFR to <35 ml/min/1.73 m2 (in patients <18 years) | <0.3 ml/kg/h for ≥24 h Anuria for ≥12 h |
Stage . | Serum creatinine criteria . | Urine output criteria . |
---|---|---|
1 | 1.50–1.9 times baseline 0.3 mg/dl increase | <0.5 ml/kg/h for 6–12 h |
2 | 2.0–2.9 times baseline | <0.5 ml/kg/h for ≥12 h |
3 | 3.0 times baseline Increase in serum creatinine to ≥4.0 mg/dl Initiation of renal replacement therapy Decrease to eGFR to <35 ml/min/1.73 m2 (in patients <18 years) | <0.3 ml/kg/h for ≥24 h Anuria for ≥12 h |
eGFR, estimated glomerular filtration rate.
Acute kidney injury staging according to the Kidney Disease Improving Global Outcomes criteria [3].
Stage . | Serum creatinine criteria . | Urine output criteria . |
---|---|---|
1 | 1.50–1.9 times baseline 0.3 mg/dl increase | <0.5 ml/kg/h for 6–12 h |
2 | 2.0–2.9 times baseline | <0.5 ml/kg/h for ≥12 h |
3 | 3.0 times baseline Increase in serum creatinine to ≥4.0 mg/dl Initiation of renal replacement therapy Decrease to eGFR to <35 ml/min/1.73 m2 (in patients <18 years) | <0.3 ml/kg/h for ≥24 h Anuria for ≥12 h |
Stage . | Serum creatinine criteria . | Urine output criteria . |
---|---|---|
1 | 1.50–1.9 times baseline 0.3 mg/dl increase | <0.5 ml/kg/h for 6–12 h |
2 | 2.0–2.9 times baseline | <0.5 ml/kg/h for ≥12 h |
3 | 3.0 times baseline Increase in serum creatinine to ≥4.0 mg/dl Initiation of renal replacement therapy Decrease to eGFR to <35 ml/min/1.73 m2 (in patients <18 years) | <0.3 ml/kg/h for ≥24 h Anuria for ≥12 h |
eGFR, estimated glomerular filtration rate.
The global burden of AKI is substantial [4]. The annual number of deaths from AKI is approximately 300 000, exceeding that from common pathologies like prostate cancer, breast cancer, heart failure, and diabetes. More than 1 million people experience AKI during hospital stay per year, which corresponds to 3.5% of overall hospital admissions. The development of AKI results in excess hospital costs of US|${\$}$|7500 per admission, which accounts for US|${\$}$|9 billion worldwide every year.
In high-income countries, AKI occurs mostly in the intensive care unit (ICU). According to an international AKI epidemiological study (AKI-EPI study) in 2015, 57.3% (1032/1802) of critically ill patients admitted to the ICU had AKI. Moreover, increasing AKI severity was associated with increased hospital mortality, and the occurrence of AKI resulted in worsening renal function at hospital discharge [5]. These findings were confirmed by recent large cohort studies in patients with sepsis [6] and those undergoing major surgery [7]. Such negative effects of AKI translate into long-term outcomes, including mortality, development of chronic kidney disease (CKD), and health-related quality of life (HRQoL) [8]. Moreover, patients with severe AKI are often prescribed renal replacement therapy (RRT) [5]. Although RRT can correct life-threatening metabolic, electrolyte, and fluid derangements attributable to AKI, its use consumes considerable resources and increases the financial burden of care [9]. Patients who undergo RRT are at high risk of progression to dialysis dependence, which further reduces HRQoL and increases healthcare costs [10, 11].
Due to the complexity and inaccessibility of the kidneys, the pathophysiology of AKI remains incompletely understood. Nonetheless, several mechanisms are considered to play a key role, including renal hypoperfusion, ischemia reperfusion syndrome, tubular cell injury, and renal tissue hypoxia [12–14]. Thus, optimization of renal perfusion and oxygenation can is a logical therapeutic target for renal protection.
To reduce the occurrence of AKI or reverse progressive renal dysfunction after AKI, various interventions have been tested over several decades, without identifying an effective intervention supported by high-quality randomized evidence (i.e. multicenter, large-scale, randomized placebo-controlled trial). Recently, however, such a trial was finally published. The Intravenous Amino Acid Therapy for Kidney Protection in Cardiac Surgery (PROTECTION) trial, a multicenter, double-blind randomized controlled trial (RCT), showed that infusion of amino acids reduces the occurrence of AKI compared to placebo among adult patients undergoing elective cardiac surgery with cardiopulmonary bypass [15]. This narrative review aims to describe the concept of renal functional reserve and the possible mechanisms of action by which intravenous amino acid therapy exerts its renoprotective effects. Then, we summarize the available evidence of amino acid infusion for renal protection and its clinical implications in critically ill and perioperative settings. Finally, we discuss the current knowledge gaps and future perspectives of this novel therapeutic strategy.
RENAL FUNCTIONAL RESERVE
To clarify how intravenous amino acid infusion works for kidney protection, it is of paramount importance to understand the concept of renal functional reserve (RFR).
Several vital organs have some additional capacity to cope with increased demand. For example, the heart can increase stroke volume and heart rate during exercise to meet the increased oxygen demand. A similar mechanism applies to the lungs, which can increase carbon dioxide excretion by increasing respiratory rate and recruiting inspiratory reserve volume. In the case of the kidneys, such reserve capacity is called RFR. In subjects with normal baseline glomerular filtration rate (GFR), an increased GFR is observed after some triggers (for example, acute protein load, intravenous amino acid administration, or unilateral nephrectomy) [16–21]. Such a GFR response represents the RFR, which is the kidneys’ capacity to increase GFR by new nephron recruitment, renal blood flow (RBF) increases, and hyperfiltration [22]. Thanks to the mechanisms of RFR, estimated GFR (eGFR) is fairly maintained after some insults. Notably, the kidneys are capable of maintaining their estimated GFR within a normal range until half of the nephrotic mass is lost, such as in the case of nephrectomy [23–25]. However, RFR is gradually lost due to its progressive utilization (for example, aging, chronic pathological states). A decline in eGFR is first detected after RFR is exhausted and cannot fully compensate for reduced renal filtration capacity as the kidney function declines according to multiple factors, including aging, CKD, and diabetes mellitus [26, 27]. The RFR is calculated as the difference between the maximal GFR achieved in response to stimulation and the baseline estimated GFR [28].
Given these properties, RFR may serve as a physiological biomarker in AKI [29]. Potential clinical applications may include preoperative AKI risk assessment in high-risk surgery (for instance, cardiac surgery) [30], renal recovery assessment after AKI [26, 31–33], and risk stratification in living-donor kidney transplantation [25].
MECHANISMS OF ACTION OF INTRAVENOUS AMINO ACID
Proposed mechanisms of action of how amino acid therapy exerts its renoprotective effects involve metabolic, endocrine, and paracrine factors, tubuloglomerular feedback (TGF), and renal oxygenation [34–37] (Figure 1).
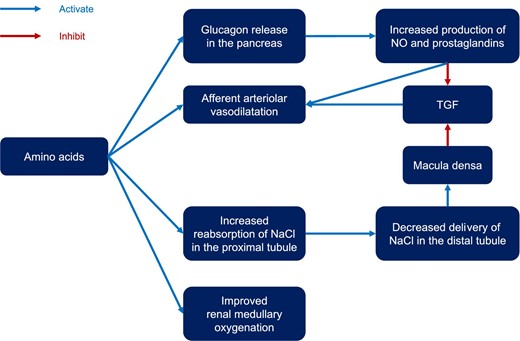
Mechanism of action of intravenous amino acids for kidney protection. TGF, tubuloglomerular feedback.
Metabolic mechanisms
One of the initial theories proposed regarding the potential mechanisms of amino acids on renal function suggested that the kidney might enhance its activity directly via renal amino acid metabolism [38]. This theory posited that increased amino acid metabolism in the kidneys could result in vasodilation, similar to the physiological responses observed in other parts of the body. However, a comparable vasodilatory effect was also noted in the afferent renal arterioles after the infusion of certain amino acids, such as alpha-amino-isobutyric acid, even though these amino acids cannot be metabolized by the kidney due to the absence of the necessary enzymes [39]. Moreover, direct infusion of metabolizable amino acids into the renal artery did not lead to comparable increases in renal vasodilatation as observed after intravenous infusion of the same amino acids [40]. Thus, the intrarenal metabolism mechanisms of amino acids do not seem central to renal vasodilatory effects of amino acids.
Endocrine and paracrine mechanisms
Endocrine and paracrine factors may play a crucial role in this process. Nitric oxide (NO) seems to play an important role in increased RBF and GFR after amino acid administration [41–43]. Indeed, NO synthase inhibitors diminish renal vasodilatation and hyperfiltration in response to amino acid infusion [44]. Moreover, the observation that NO can dilate renal afferent arteriole directly further supports the relevance of this mechanism [44]. As a substrate for NO synthase, l-arginine is considered a key component; however, other amino acids can also induce renal vasodilatation [39]. Thus, the exact key effector molecule remains uncertain. Prostaglandins are another important class of paracrine molecules in the context of amino acid infusion. Prostaglandin synthesis inhibitors blunt the GRF response after amino acid infusions [45, 46], which can be reversed when arginine infusion is applied [47]. Moreover, evidence suggests that the expression of renal cortical NO synthase [48] and cyclooxygenase-2 [49] correlates with dietary protein intake. These enzymes increase the production of NO and prostaglandins, which contribute to renal afferent arterial vasodilation.
Glucagon is released from alpha cells in the pancreas in response to sympathetic nervous stimulation, hypoglycemia, and ingestion of specific amino acids [50]. Pancreatectomized humans and dogs lose RFR [51–53]. Glucagon stimulates the production of prostaglandins [45], inducing renal afferent arteriolar vasodilatation. Branched chain amino acid infusion, which does not trigger glucagon release, does not increase RBF and GFR, while alpha-amino-isobutyric acid [40], which triggers glucagon release, increases them [39].
Tubuloglomerular feedback
As a delicate renal autoregulatory system, the TGF is a renal autoregulatory system that controls glomerular filtration at nephron level [54]. Specifically, TGF regulates renal afferent arteriolar tone, and glomerular capillary pressure according to the delivery rate of fluid and salt to the macula densa. Activation of TGF leads to afferent arteriolar vasoconstriction, which in turn results in reduced glomerular capillary pressure and GFR to maintain distal delivery of sodium chloride [55].
Recent advances have deepened our understanding of the proximal tubule, where amino acids and sodium are co-transported. This process is enhanced by amino acid administration, leading to increased reabsorption of sodium and chloride. As a result, the tubular fluid that reaches the macula densa has a lower sodium chloride concentration, which in turn reduces the signal required for activating TGF and decreases afferent arteriolar resistance [56]. Additionally, glycine appears to have vasodilatory effects, functioning as a co-agonist to N-methyl-d-aspartate receptors located on the proximal tubule membranes [57]. These mechanisms collectively improve renal perfusion and elevate GFR.
Renal oxygenation
Amino acids can improve oxygenation in the renal cortex and medulla. This mechanism was illustrated in a recent study on non-anesthetized sheep [58]. After implementing continuous measurements of renal cortical and medullary oxygenation under general anesthesia, the sheep received intravenous amino acids. Amino acid infusion not only increased renal oxygen delivery and GFR but also improved renal cortical and medullary tissue oxygenation. Given the crucial role of renal medullary hypoxia in AKI development [59–61], this mechanisms appears to be central to the renoprotective effects of the amino acids-induced recruitment of RFR.
CLINICAL EVIDENCE OF INTRAVENOUS AMINO ACIDS FOR KIDNEY PROTECTION
We performed a comprehensive literature review based on the following PICOS (patient, intervention, control, outcome, and study design) framework: critically ill and perioperative adult patients (P), intravenous amino acid infusion (I), any comparator (C), AKI (O), and randomized controlled trial (RCT). We identified six RCTs testing intravenous amino acid infusion for renal protection in critically ill and perioperative patients (the complete search strategy and study selection flowchart are found in the supplementary material and Figure S1) [15, 62–66]. Table 2 summarizes the characteristics of these six trials.
Randomized controlled trials of intravenous amino acid for kidney protection.
Author, year . | No. of centers . | No. of patients . | Patients . | Amino acid therapy . | Control . | Primary outcome . | Other findings . |
---|---|---|---|---|---|---|---|
Abel RM, 1973 [62] | 1 | 53 | Patients with AKI | 13 g/day (up to 32.5 g/day) | Glucose solution | Amino acid therapy improved survival from AKI (75% versus 44%; P = 0.02) | In patients who received RRT, survival rates were higher in the amino acid group (65% versus 19%; P = 0.02). |
Singer P, 2007 [63] | 1 | 14 | Patients receiving mechanical ventilation with a creatinine clearance <50 ml/min and urine output >2000 ml/day | 150 g/day | 75 g/day of amino acid infusion | Mortality was not different (38% versus 33%; P = 0.87) | High-dose amino acid therapy resulted in less fluid accumulation (−2407 ± 1990 versus 2003 ± 1336 ml) and lower furosemide requirement (649 ± 293 versus 1003 ± 288 mg) |
Doig GS, 2015 [64] | 16 | 474 | Critically ill adult patients on day 1 or 2 of ICU stay if they were expected to remain in ICU at least 2 days after enrolment | ≥100 g/day | Standard care | Duration of renal dysfunction (creatinine > 168 µmol/l) did not differ (P = 0.45) | Amino acid therapy improved eGFR (7.7 ml/min/1.73 m2; 95% CI, 1.0–14.5 ml/min/1.73 m2) and urine output (300 ml/day; 95% CI, 145–455 ml) |
Pu H, 2019 [65] | 1 | 69 | Adults undergoing elective cardiac surgery expected to require >1 h cardiopulmonary bypass | ≥100 g/day | Standard care | Duration of renal dysfunction (creatinine > 168 µmol/l) did not differ (P = 0.84) | Amino acid therapy reduced duration of AKI (RR, 0.02; 95% CI, 0.005–0.11), increased eGFR (+10.8%; 95% CI, 1.0–20.8%) and urine output (1.7 ± 0.9 versus 1.4 ± 0.5 l/day) |
Kazawa M, 2024 [66] | 1 | 66 | Adults undergoing aortic surgery with cardiopulmonary bypass | 60 g/day | Standard care | Amino acid therapy reduced rates of AKI (30% vs. 56%; P = 0.04). | RRT was only prescribed to 3 patients in the control group. Higher urine output and eGFR were observed in the amino acid group. |
Landoni G, 2024 [15] | 22 | 3511 | Adults undergoing elective cardiac surgery with cardiopulmonary bypass | 2 g/kg IBW/day (≤100 g/day) | Placebo (Ringer's solution) | Amino acid therapy reduced AKI occurrence (27% versus 32%; P = 0.002) | No difference was found for RRT (RR, 0.73; 95% CI, 0.43–1.22) or ICU mortality (RR, 0.89; 95% CI, 0.56–1.41) |
Author, year . | No. of centers . | No. of patients . | Patients . | Amino acid therapy . | Control . | Primary outcome . | Other findings . |
---|---|---|---|---|---|---|---|
Abel RM, 1973 [62] | 1 | 53 | Patients with AKI | 13 g/day (up to 32.5 g/day) | Glucose solution | Amino acid therapy improved survival from AKI (75% versus 44%; P = 0.02) | In patients who received RRT, survival rates were higher in the amino acid group (65% versus 19%; P = 0.02). |
Singer P, 2007 [63] | 1 | 14 | Patients receiving mechanical ventilation with a creatinine clearance <50 ml/min and urine output >2000 ml/day | 150 g/day | 75 g/day of amino acid infusion | Mortality was not different (38% versus 33%; P = 0.87) | High-dose amino acid therapy resulted in less fluid accumulation (−2407 ± 1990 versus 2003 ± 1336 ml) and lower furosemide requirement (649 ± 293 versus 1003 ± 288 mg) |
Doig GS, 2015 [64] | 16 | 474 | Critically ill adult patients on day 1 or 2 of ICU stay if they were expected to remain in ICU at least 2 days after enrolment | ≥100 g/day | Standard care | Duration of renal dysfunction (creatinine > 168 µmol/l) did not differ (P = 0.45) | Amino acid therapy improved eGFR (7.7 ml/min/1.73 m2; 95% CI, 1.0–14.5 ml/min/1.73 m2) and urine output (300 ml/day; 95% CI, 145–455 ml) |
Pu H, 2019 [65] | 1 | 69 | Adults undergoing elective cardiac surgery expected to require >1 h cardiopulmonary bypass | ≥100 g/day | Standard care | Duration of renal dysfunction (creatinine > 168 µmol/l) did not differ (P = 0.84) | Amino acid therapy reduced duration of AKI (RR, 0.02; 95% CI, 0.005–0.11), increased eGFR (+10.8%; 95% CI, 1.0–20.8%) and urine output (1.7 ± 0.9 versus 1.4 ± 0.5 l/day) |
Kazawa M, 2024 [66] | 1 | 66 | Adults undergoing aortic surgery with cardiopulmonary bypass | 60 g/day | Standard care | Amino acid therapy reduced rates of AKI (30% vs. 56%; P = 0.04). | RRT was only prescribed to 3 patients in the control group. Higher urine output and eGFR were observed in the amino acid group. |
Landoni G, 2024 [15] | 22 | 3511 | Adults undergoing elective cardiac surgery with cardiopulmonary bypass | 2 g/kg IBW/day (≤100 g/day) | Placebo (Ringer's solution) | Amino acid therapy reduced AKI occurrence (27% versus 32%; P = 0.002) | No difference was found for RRT (RR, 0.73; 95% CI, 0.43–1.22) or ICU mortality (RR, 0.89; 95% CI, 0.56–1.41) |
AKI: Acute kidney injury; CI, confidence interval; IBW, ideal body weight; ICU, intensive care unit; eGFR, estimated glomerular filtration ratio; RR, relative risk; RRT; renal replacement therapy.
Randomized controlled trials of intravenous amino acid for kidney protection.
Author, year . | No. of centers . | No. of patients . | Patients . | Amino acid therapy . | Control . | Primary outcome . | Other findings . |
---|---|---|---|---|---|---|---|
Abel RM, 1973 [62] | 1 | 53 | Patients with AKI | 13 g/day (up to 32.5 g/day) | Glucose solution | Amino acid therapy improved survival from AKI (75% versus 44%; P = 0.02) | In patients who received RRT, survival rates were higher in the amino acid group (65% versus 19%; P = 0.02). |
Singer P, 2007 [63] | 1 | 14 | Patients receiving mechanical ventilation with a creatinine clearance <50 ml/min and urine output >2000 ml/day | 150 g/day | 75 g/day of amino acid infusion | Mortality was not different (38% versus 33%; P = 0.87) | High-dose amino acid therapy resulted in less fluid accumulation (−2407 ± 1990 versus 2003 ± 1336 ml) and lower furosemide requirement (649 ± 293 versus 1003 ± 288 mg) |
Doig GS, 2015 [64] | 16 | 474 | Critically ill adult patients on day 1 or 2 of ICU stay if they were expected to remain in ICU at least 2 days after enrolment | ≥100 g/day | Standard care | Duration of renal dysfunction (creatinine > 168 µmol/l) did not differ (P = 0.45) | Amino acid therapy improved eGFR (7.7 ml/min/1.73 m2; 95% CI, 1.0–14.5 ml/min/1.73 m2) and urine output (300 ml/day; 95% CI, 145–455 ml) |
Pu H, 2019 [65] | 1 | 69 | Adults undergoing elective cardiac surgery expected to require >1 h cardiopulmonary bypass | ≥100 g/day | Standard care | Duration of renal dysfunction (creatinine > 168 µmol/l) did not differ (P = 0.84) | Amino acid therapy reduced duration of AKI (RR, 0.02; 95% CI, 0.005–0.11), increased eGFR (+10.8%; 95% CI, 1.0–20.8%) and urine output (1.7 ± 0.9 versus 1.4 ± 0.5 l/day) |
Kazawa M, 2024 [66] | 1 | 66 | Adults undergoing aortic surgery with cardiopulmonary bypass | 60 g/day | Standard care | Amino acid therapy reduced rates of AKI (30% vs. 56%; P = 0.04). | RRT was only prescribed to 3 patients in the control group. Higher urine output and eGFR were observed in the amino acid group. |
Landoni G, 2024 [15] | 22 | 3511 | Adults undergoing elective cardiac surgery with cardiopulmonary bypass | 2 g/kg IBW/day (≤100 g/day) | Placebo (Ringer's solution) | Amino acid therapy reduced AKI occurrence (27% versus 32%; P = 0.002) | No difference was found for RRT (RR, 0.73; 95% CI, 0.43–1.22) or ICU mortality (RR, 0.89; 95% CI, 0.56–1.41) |
Author, year . | No. of centers . | No. of patients . | Patients . | Amino acid therapy . | Control . | Primary outcome . | Other findings . |
---|---|---|---|---|---|---|---|
Abel RM, 1973 [62] | 1 | 53 | Patients with AKI | 13 g/day (up to 32.5 g/day) | Glucose solution | Amino acid therapy improved survival from AKI (75% versus 44%; P = 0.02) | In patients who received RRT, survival rates were higher in the amino acid group (65% versus 19%; P = 0.02). |
Singer P, 2007 [63] | 1 | 14 | Patients receiving mechanical ventilation with a creatinine clearance <50 ml/min and urine output >2000 ml/day | 150 g/day | 75 g/day of amino acid infusion | Mortality was not different (38% versus 33%; P = 0.87) | High-dose amino acid therapy resulted in less fluid accumulation (−2407 ± 1990 versus 2003 ± 1336 ml) and lower furosemide requirement (649 ± 293 versus 1003 ± 288 mg) |
Doig GS, 2015 [64] | 16 | 474 | Critically ill adult patients on day 1 or 2 of ICU stay if they were expected to remain in ICU at least 2 days after enrolment | ≥100 g/day | Standard care | Duration of renal dysfunction (creatinine > 168 µmol/l) did not differ (P = 0.45) | Amino acid therapy improved eGFR (7.7 ml/min/1.73 m2; 95% CI, 1.0–14.5 ml/min/1.73 m2) and urine output (300 ml/day; 95% CI, 145–455 ml) |
Pu H, 2019 [65] | 1 | 69 | Adults undergoing elective cardiac surgery expected to require >1 h cardiopulmonary bypass | ≥100 g/day | Standard care | Duration of renal dysfunction (creatinine > 168 µmol/l) did not differ (P = 0.84) | Amino acid therapy reduced duration of AKI (RR, 0.02; 95% CI, 0.005–0.11), increased eGFR (+10.8%; 95% CI, 1.0–20.8%) and urine output (1.7 ± 0.9 versus 1.4 ± 0.5 l/day) |
Kazawa M, 2024 [66] | 1 | 66 | Adults undergoing aortic surgery with cardiopulmonary bypass | 60 g/day | Standard care | Amino acid therapy reduced rates of AKI (30% vs. 56%; P = 0.04). | RRT was only prescribed to 3 patients in the control group. Higher urine output and eGFR were observed in the amino acid group. |
Landoni G, 2024 [15] | 22 | 3511 | Adults undergoing elective cardiac surgery with cardiopulmonary bypass | 2 g/kg IBW/day (≤100 g/day) | Placebo (Ringer's solution) | Amino acid therapy reduced AKI occurrence (27% versus 32%; P = 0.002) | No difference was found for RRT (RR, 0.73; 95% CI, 0.43–1.22) or ICU mortality (RR, 0.89; 95% CI, 0.56–1.41) |
AKI: Acute kidney injury; CI, confidence interval; IBW, ideal body weight; ICU, intensive care unit; eGFR, estimated glomerular filtration ratio; RR, relative risk; RRT; renal replacement therapy.
The first RCT of amino acid infusion for renal protection was published in 1973 [62]. This single-center, double-blind RCT enrolled 53 patients with acute renal failure and assigned them either to receive an intravenous fluid therapy consisting of 1.3% amino acid plus hypertonic glucose or hypertonic glucose alone. Survival from acute renal failure was 75% in the amino acid group versus 44% in the control group (P = 0.02). Such survival benefits were confirmed in different subgroups including patients receiving RRT. However, the medical community somehow neglected this promising intervention until recently.
A subsequent single-center RCT in 2007 aimed to evaluate the dose–response relationship between amino acid therapy and renal protection [63]. In this trial, 14 critically ill patients with non-oliguric acute renal failure were randomly allocated to 150 or 75 g/day intravenous amino acid therapy. A higher amino acid infusion resulted in less positive fluid balance (–2407 ± 1336 ml versus +2003 ± 1336 ml) and less furosemide dose (649 ± 293 mg versus 1003 ± 288 mg). In addition, creatinine clearance was improved in the higher amino acid loading group.
Based on these preliminary trial data, a multicenter, phase 2 RCT was conducted to test the renoprotective effects of 100 g/day amino acid therapy compared to usual care in 474 critically ill patients [64]. Although the primary outcome, duration of renal dysfunction [serum creatinine > 168 mmol/l (approximately 1.9 mg/d)], was not different [mean difference, 0.21 AKI days per 10 patient ICU days; 95% confidence interval (CI), –0.27 to 1.04], intravenous amino acid therapy increased eGFR and daily urine output (mean difference, 300 ml/day; 95% CI, 145 to 455 ml/day). In addition, RRT prescription was numerically reduced (5.5% versus 10.5%; P = 0.06). No serious adverse event was considered related to the study intervention. Furthermore, a post-hoc analysis of this trial found that 90-day mortality was lower in the amino acid group, among patients with normal renal function at randomization (14% versus 21%; P = 0.034) [67].
Amino acid therapy was also investigated in cardiac surgery settings. A single-center pilot RCT was performed in 69 adult patients undergoing cardiac surgery with cardiopulmonary bypass [65]. Although the duration of renal dysfunction was not different, amino acid therapy reduced the duration of AKI (0.08 versus 0.45 days of AKI per 10 hospital days), improved eGFR (+10.8%; 95% CI, +1.0 to +20.8%), and increased daily urine output (1.7 ± 0.9 l versus 1.4 ± 0.5 l). Again, no serious adverse events were reported. Similar findings were obtained in another single-center RCT on patients undergoing surgery with cardiopulmonary bypass [66]. Amino acid administration resulted in a lower rate of AKI (30% versus 56%; P = 0.04), and higher eGFR (64.8 versus 56.4 ml/min/1.73 m2; P = 0.049) and urine output (2420 versus 1865 ml; P = 0.049) than standard care.
These small to moderate-sized RCTs consistently showed that intravenous amino acid therapy improved eGFR and urine output without any safety issues, which formed the evidence-based foundation for conducting a large-scale multicenter RCT to confirm its renoprotective effects.
The PROTECTION trial was designed to test the efficacy and safety of intravenous amino acid therapy (2 g/kg of ideal body weight per day, up to 100 g per day) in 3511 adult patients undergoing scheduled cardiac surgery using cardiopulmonary bypass in 22 centers from three countries. The enrolled patients were randomly assigned to receive intravenous infusion of amino acids or an equivalent amount of placebo (Ringer's solution) [15]. The study drug administration lasted until ICU discharge, RRT initiation, or 72 hours of study treatment, whichever occurred first. Overall, the median study drug infusion duration was approximately 30 hours with a median infusion rate of 40 ml/h. Intravenous amino acid infusion reduced AKI occurrence [26.9% versus 31.7%; relative risk (RR), 0.85; 95% CI, 0.77 to 0.94]. Most patients suffered from stage 1 AKI stage according to the KDIGO criteria (24.4 versus 28.1%; RR, 0.87; 95% CI, 0.78 to 0.97), and stage 3 AKI was also reduced in the amino acid group (1.6% versus 3.0%; RR, 0.56; 95% CI, 0.35 to 0.87). These findings were confirmed by prespecified subgroup analyses. Of note, the AKI-reducing effect was maintained in a subgroup of CKD patients (RR, 0.86; 95% CI, 0.74 to 0.99) [68]. The use of RRT was 1.4% versus 1.9% (RR, 0.73; 95% CI, 0.43 to 1.22). Other clinical outcomes and adverse events were not different between the two groups. Blood urea levels were higher in the amino acid group (median highest value during the ICU stay, 53 versus 41 mg/dl); however, such an increase was expected and did not reach the threshold to initiate RRT.
Following the publication of the PROTECTION trial, a systematic review and meta-analysis was conducted to comprehensively understand the role of amino acid therapy in perioperative settings [69]. Data synthesis from 15 eligible studies totaling 8628 patients yielded that amino acid infusion was associated with reduced AKI risks compared to any comparator (RR, 0.66; 95% CI, 0.47 to 0.94; I2 = 50%). A Bayesian meta-analysis estimated a 99.1% probability of AKI reduction with amino acid therapy. Beneficial effects of amino acid therapy were also found in reduced serum creatinine levels, increased eGFR, and shortened duration of hospital stay. Therefore, the currently available evidence shows that intravenous amino acid therapy reduces AKI in perioperative settings and exerts renoprotective effects on surrogate outcomes (for example, eGFR and urine output) in critically ill settings.
CLINICAL IMPLICATIONS OF THE AVAILABLE EVIDENCE
The findings of the PROTECTION trial have substantial clinical implications in cardiac surgical settings. Thanks to its internal (for example, placebo-controlled double-blind design and high protocol compliance) and external validity (for example, multicenter design involving three countries, a wide range of the eligibility criteria), the findings are robust and thus should prompt clinicians to apply the intervention into their routine cardiac surgery practice to reduce the risk of AKI. Not only the overall AKI (especially stage 1 AKI) but also stage 3 AKI was significantly reduced, which suggests the potential mitigation of AKI severity. Hence, intravenous amino acid therapy has become the first effective prevention for AKI proven by high-quality randomized evidence. Given the widespread conduct of on-pump cardiac surgery (approximately 2 million procedures every year) and its associated AKI occurrence worldwide (30% of patients undergoing cardiac surgery), amino acid therapy might prevent AKI in hundreds of thousands of patients when this intervention is applied as routine care globally. Such AKI reduction could be translated into considerable improvements in long-term relevant outcomes (for example, development and worsening of CKD), as well as in savings for the healthcare system and society.
Interestingly, the consistent AKI reduction effects were preserved in patients with CKD. Amino acid therapy likely depends on RFR recruitment to exert its renal protective properties, together with a possible mitigation of renal damage toward improved RBF and renal tissue oxygenation. A previous trial suggested its beneficial effects are most likely exerted in patients with normal baseline renal function [67]. Thus, it would be reasonable to assume a declined treatment effect in patients with CKD. Contrary to this expectation, AKI was prevented similarly in patients with and without CKD. The occurrence of AKI on CKD is common, frequently requires RRT, and results in a worsened CKD stage [70]. Despite a hypothesis-generating subgroup analysis, the PROTECTION trial suggests the potential of amino acid therapy to delay renal function deterioration in CKD populations.
As the PROTECTION trial was not originally designed to detect a difference in RRT use and the actual incidence was quite low, the difference did not reach a statistical significance. Nonetheless, the direction of treatment effects was in favor of amino acid therapy. Consistent with previous trials, the PROTECTION trial did not observe any safety issues with amino acid therapy, which is reassuring for clinicians who consider introducing it into clinical practice.
In critically ill patients, amino acid therapy seems to convey some renal protective effects with improved eGFR, serum creatinine levels, and urine output. However, none of the available RCTs is large enough to draw a definitive conclusion when applying it to routine clinical practice. Thus, outside of cardiac surgery, the use of this intervention should be limited to research purposes.
FUTURE PERSPECTIVES
The PROTECTION trial showed AKI reduction with intravenous amino acid administration with favorable trends in RRT use. Despite these encouraging results, one may wonder if such an AKI decrease represents just a functional change in serum creatinine levels or a true renal protection from tubular injury [71]. As a pragmatic trial design, the PROTECTION trial did not report data on damage/stress biomarkers like kidney injury molecule-1 and neutrophil gelatinase-associated lipocalin [72]. Future studies collecting these AKI biomarkers will help elucidate the pharmacological mechanisms of action of amino acid therapy and the renal responses to this intervention.
Previous trials, including the PROTECTION trial, used a solution of a mixture of amino acids as the study intervention [15, 62, 64, 65]. Among the included compounds, arginine and glycine seem to play a key role through renal vasodilatation [73, 74]. However, the mechanisms of renoprotection by intravenous amino acid therapy remains incompletely understood, requiring further investigation.
The PROTECTION trial reported clinical outcomes up to 6 months. As AKI may affect renal function even years later [70], further follow-up data would be necessary to determine the long-term impacts of amino acid therapy. Moreover, quality of life is one of the core outcomes set for cardiac surgery [75] and critically ill trials [76]. Although the health-related quality of life at 6 months was not different in the PROTECTION trial, further investigation is warranted to evaluate this patient-reported outcome.
One of the concerns raised when introducing intravenous amino acid therapy into clinical practice is economic costs. For example, a 500 ml solution of 10% of a mixture of amino acids available in the United States (TrophAmine 10%®) costs approximately US|${\$}$|70, whereas 500 ml of lactated Ringer's solution costs US|${\$}$|7. The PROTECTION trial showed a significant reduction of AKI occurrence without a clear effect on RRT use. As available agents with similar amino acid compositions may cost differently in different countries, healthcare costs vary considerably. Thus, a cost-effective analysis is needed to determine whether such AKI reduction deserves an increased economic cost for the drug considering local costs in each country. Despite these uncertainties, the prevention of AKI observed with intravenous amino acid therapy remains clinically relevant, given its large sample size, double-blind design, and broad clinical applicability. These findings should encourage clinicians to consider its routine use, particularly for patients who meet the eligibility criteria outlined in the PROTECTION trial.
The promising data from the PROTECTION trial should also urge further investigations in other patient populations. For example, sepsis-associated AKI is the most common form of AKI and carries a significant burden on patient outcomes and healthcare system [5]. Non-cardiac major surgery is another candidate population with its high AKI incidence [7]. Patients with myocardial infarction-related cardiogenic shock suffer from multiple AKI risks, including inadequate renal perfusion due to shock itself, use of contrast media during coronary angiography, and hemolysis when mechanical circulatory support is applied [77]. When designing trials in emergent settings (for example, sepsis and cardiogenic shock), it seems plausible to consider a possible urea increase with amino acid infusion to avoid unnecessary RRT initiation attributable to the study intervention. Table 3 summarizes these future perspectives (Table 3).
Future research directions regarding intravenous amino acid infusion for renal protection.
1 | Assessment of tubular injury using damage/stress kidney biomarkers |
2 | Identification of key amino acid components with beneficial effects on renal protection |
3 | Long-term assessment including patient-reported outcomes (for example, quality of life) |
4 | Cost-effectiveness analysis considering local drug and healthcare costs |
5 | Investigation in different patient populations (for example, sepsis, non-cardiac major surgery, cardiogenic shock) |
1 | Assessment of tubular injury using damage/stress kidney biomarkers |
2 | Identification of key amino acid components with beneficial effects on renal protection |
3 | Long-term assessment including patient-reported outcomes (for example, quality of life) |
4 | Cost-effectiveness analysis considering local drug and healthcare costs |
5 | Investigation in different patient populations (for example, sepsis, non-cardiac major surgery, cardiogenic shock) |
Future research directions regarding intravenous amino acid infusion for renal protection.
1 | Assessment of tubular injury using damage/stress kidney biomarkers |
2 | Identification of key amino acid components with beneficial effects on renal protection |
3 | Long-term assessment including patient-reported outcomes (for example, quality of life) |
4 | Cost-effectiveness analysis considering local drug and healthcare costs |
5 | Investigation in different patient populations (for example, sepsis, non-cardiac major surgery, cardiogenic shock) |
1 | Assessment of tubular injury using damage/stress kidney biomarkers |
2 | Identification of key amino acid components with beneficial effects on renal protection |
3 | Long-term assessment including patient-reported outcomes (for example, quality of life) |
4 | Cost-effectiveness analysis considering local drug and healthcare costs |
5 | Investigation in different patient populations (for example, sepsis, non-cardiac major surgery, cardiogenic shock) |
CONCLUSION
Intravenous amino acid therapy can protect renal function through recruitment of RFR and direct improvement in renal perfusion and oxygenation. Small to moderate-sized RCTs consistently showed improvements in eGFR and urine output. The recently published PROTECTION trial, a large, multicenter, double-blind RCT reported significant AKI prevention with amino acid therapy. This trial result not only provides robust evidence to support its use in cardiac surgical settings but should also stimulate further investigations in other patient populations. Moreover, cost-effectiveness analysis, long-term evaluation, and assessment of tubular injury using damage/stress AKI biomarkers are warranted to address uncertainties regarding this promising intervention.
FUNDING
No funding is available for this work.
AUTHORS’ CONTRIBUTIONS
Y.K. and G.L. were responsible for the conception or design of the work. Y.K. drafted the manuscript. All authors were responsible for revision of the manuscript for important intellectual content.
DATA AVAILABILITY STATEMENT
All data are available in the article.
CONFLICT OF INTEREST STATEMENT
All the authors have no conflicts of interest.
Comments