-
PDF
- Split View
-
Views
-
Cite
Cite
Pietro Anastasio, Francesco Trepiccione, Natale Gaspare De Santo, Giovambattista Capasso, Davide Viggiano, Giovanna Capolongo, Regulation of urinary calcium excretion by vasopressin, Clinical Kidney Journal, Volume 13, Issue 5, October 2020, Pages 873–877, https://doi.org/10.1093/ckj/sfaa134
- Share Icon Share
Abstract
The antidiuretic hormone (ADH) or arginine vasopressin (AVP) regulates the body's water balance. Recently, modifications in AVP levels have been related to osteoporosis during ageing and microgravity/bed rest. Therefore the present study was devised to assess whether the absence of AVP, as in patients with central diabetes insipidus (CDI), modulates renal calcium excretion.
We retrospectively analysed data from 12 patients with CDI with measured 24-h urinary excretion levels of calcium. Data were available at the moment of the diagnosis when patients were drug-free and after therapy with dDAVP, an analog of AVP. Hypercalciuria was defined as 24-h urinary Ca2+ >275 mg/day in males and >250 mg/day in females and a urinary calcium (Ca):creatinine (Cr) ratio >0.20 mg/mg.
Untreated CDI patients had a daily urinary Ca2+ excretion of 383 ± 47 mg/day and a urinary Ca:Cr ratio of 0.26 ± 0.38 mg/mg. The urine osmolarity significantly increased after the administration of dDAVP by 210% and the urinary flow decreased by 72%. Furthermore, the estimated glomerular filtration rate (eGFR) increased by 7%, which did not reach statistical significance. dDAVP treatment did not significantly modify the urinary Ca2+ concentration; however, the daily calcium excretion and the urinary Ca:Cr ratio were significantly decreased (160 ± 27 mg/day and 0.11 ± 0.02 mg/mg, respectively).
Patients with CDI show hypercalciuria even though urine is more diluted than normal controls, and dDAVP reverses this effect. These data support the intriguing relationship between AVP and osteoporosis in ageing and microgravity/bed rest.
INTRODUCTION
It is commonly accepted that the antidiuretic hormone (ADH) or arginine vasopressin (AVP), secreted by the neurohypophysis, regulates the body's water balance: increased serum osmolality induces AVP secretion, which in turn increases water absorption in the renal collecting ducts [1]. These cells respond to the binding of AVP to the V2 receptor, thereby redistributing the aquaporin 2 channel (AQP2) from intracellular vesicles to the apical plasma membrane [2]. The chronic infusion of dDAVP, a non-pressor V2 receptor agonist, decreases the fractional urinary excretion of calcium in Brattleboro rats, a model of hereditary hypothalamic diabetes insipidus [3]. In humans, the action of AVP on calcium renal excretion is still debated. Data from four patients with central diabetes insipidus (CDI) and four with nephrogenic diabetes insipidus (NDI) showed increased calcium fractional excretion in 50% of CDI patients and 25% of NDI patients. Furthermore, intravenous dDAVP reduced Ca2+ fractional excretion (FE) from 1.71 to 0.58% in CDI patients 3 h after injection, whereas it did not affect, as expected, patients with NDI [4]. The reduction of calciuria by AVP is also supported by the reduction of Ca2+ FE after water load (which supposedly reduces AVP) in normal subjects. However, the same authors also found hypercalciuria in the presence of excessive secretion of ADH, as in the syndrome of in appropriate secretion of ADH (siADH) [4], which would lead to the opposite conclusion that AVP increases calciuria.
Additional insights from the role of AVP in the regulation of urinary calcium excretion derive from children affected by enuresis, a condition considered as an impaired effect of nocturnal AVP action on the collecting duct [5–8]. In that model of human disease, there were three main findings:
hypercalciuria and reduced AVP secretion are associated with reduced AQP2 excretion [6];
a low-calcium diet reduced hypercalciuria and re-established the response to dDAVP as indicated by a reduction in the frequency of enuresis, normalization of the higher night-time than day-time diuresis ratio and increased urine osmolality [7]; and
the proposal of a renal concentrating defect sustained by negative regulatory feedback of calcium-sensing receptor (CaSR) on aquaporin-2 expression [8].
However, several points are still debated on the role of hypercalciuria in enuretic children [9]. Indeed, the administration of dDAVP to adults with nocturnal polyuria before sleep, together with an improvement in urinary osmolality, increased whole-day calcium excretion and reduced whole-day urinary potassium without effects on urinary sodium excretion [10]. Finally, from the experimental point of view, recent evidence failed to identify the presence of CaSR along the collecting duct, questioning the presence of a potential functional interaction between CaSR and AQP2 [11]. Therefore the present study was devised to assess whether AVP modulates renal calcium excretion in patients with CDI and hypercalciuria, a model that seems to hold great potential.
MATERIALS AND METHODS
We retrospectively analysed patient data from the Renal Unit of our university for the period 1980–2000, enrolling all patients with CDI. A group of 12 patients was enrolled. This period was selected because the patients admitted to the Nephrology Department were studied by inulin clearance to measure the glomerular filtration rate (GFR), whereas at later times this method was no longer available, at least in CDI patients.
Clinical and anthropometric data of participants are presented in Table 1. The study was approved by the Ethical Committee of the Second University of Naples, Italy [12].
Parameter . | Values . |
---|---|
Gender (female/male), n/n | 4/8 |
Age (years) | 34 ± 3 |
Body weight (kg) | 66 ± 3 |
Plasma creatinine (mg/dL) | 0.98 ± 0.2 |
Plasma urea (mg/dL) | 29 ± 5 |
Systolic blood pressure (mmHg) | 116 ± 6 |
Diastolic blood pressure (mmHg) | 73 ± 4 |
Parameter . | Values . |
---|---|
Gender (female/male), n/n | 4/8 |
Age (years) | 34 ± 3 |
Body weight (kg) | 66 ± 3 |
Plasma creatinine (mg/dL) | 0.98 ± 0.2 |
Plasma urea (mg/dL) | 29 ± 5 |
Systolic blood pressure (mmHg) | 116 ± 6 |
Diastolic blood pressure (mmHg) | 73 ± 4 |
Data are presented as mean ± standard deviation unless stated otherwise.
Parameter . | Values . |
---|---|
Gender (female/male), n/n | 4/8 |
Age (years) | 34 ± 3 |
Body weight (kg) | 66 ± 3 |
Plasma creatinine (mg/dL) | 0.98 ± 0.2 |
Plasma urea (mg/dL) | 29 ± 5 |
Systolic blood pressure (mmHg) | 116 ± 6 |
Diastolic blood pressure (mmHg) | 73 ± 4 |
Parameter . | Values . |
---|---|
Gender (female/male), n/n | 4/8 |
Age (years) | 34 ± 3 |
Body weight (kg) | 66 ± 3 |
Plasma creatinine (mg/dL) | 0.98 ± 0.2 |
Plasma urea (mg/dL) | 29 ± 5 |
Systolic blood pressure (mmHg) | 116 ± 6 |
Diastolic blood pressure (mmHg) | 73 ± 4 |
Data are presented as mean ± standard deviation unless stated otherwise.
Patients did not use thiazide diuretic, known to reduce calcium excretion, increasing proximal tubular calcium reabsorption. No concomitant diseases that may induce hypercalciuria were known in any of these patients. They had undergone neurosurgery for the removal of tumours in the central nervous system. None of them was a renal stone former (ascertained by their narratives and by ultrasounds). The diagnosis was based on clinical presentation (polyuria, polydipsia), blood AVP and plasma and urine osmolarity at baseline and after water deprivation.
They underwent two ambulatory admissions to the University Hospital of the University of Campania Luigi Vanvitelli, immediately before starting treatment for their polyuric state and after 1 week of intranasal therapy with dDAVP (a non-pressor analogue of AVP-Minirin) at a dose of 10 μg three times a day.
Data collection and measurement of GFR
GFR was measured as the inulin clearance. Inulin was given as a bolus (40 mg/kg body weight) followed by constant intravenous infusion to keep the plasma concentration at 20 mg/dL, as described elsewhere [12, 13]. After 90 min of equilibration, the baseline GFR was measured by the inulin clearance method using the average of three determinations, each lasting for 30 min. The plasma clearance of inulin was calculated by the standard formula and corrected for 1.73 m2, as described elsewhere [14–16]. Inulin was measured by colorimetric methods [17]. A 24-h urine collection was performed the day before to determine excretion of sodium, potassium, urea, calcium, creatinine and osmolality. The following parameters were recorded: diuresis volume, urine flow rate, serum calcium, urea, creatinine, sodium, potassium and osmolality. Estimated GFR (eGFR) was determined using the Chronic Kidney Disease Epidemiology Collaboration (CKD-EPI) formula [18]. Sodium and potassium in urine and plasma were measured by ion-selective electrodes utilizing a sodium–potassium analyser (KNA1; Radiometer, Copenhagen, Denmark). Urea was measured by an autoanalyser (Beckman Coulter, Brea, CA, USA). Creatinine was measured according to the picric acid colorimetric method [19]. Calcium was measured with a model 3300 atomic absorption spectrometer (PerkinElmer, Waltham, MA, USA. Urine osmolality was measured by a freezing point depression Osmometer 3320 (Advanced Instruments, Norwood, MA, USA) as previously described [20–22].
Statistical analysis
Statistical analysis was performed with R version 3.6.1 (R Foundation, Vienna, Austria). Data are expressed as mean ± standard error of measurement (SEM). The gender effect on baseline clinical variables was tested by t-test for independent samples before pooling all data in subsequent analyses. Differences between means were evaluated by t-test for paired observations. The baseline value of GFR was calculated as the average of three 30-min clearances. P-values <0.05 were considered statically significant.
All procedures performed in the studies involving human participants were in accordance with the ethical standards of the Institutional Committee of the University of Campania and with the 1964 Helsinki Declaration and its later amendments or comparable ethical standards.
RESULTS
Baseline values of the population under study are reported in Table 1. Blood pressure was in the normal range and no increase in plasma creatinine level was observed. We have tested the presence of a gender effect at baseline using t-tests and found no effect in any of the clinical variables reported in Table 1 (P > 0.05 for all variables, t-test for non-paired data). Therefore, in subsequent analyses, we included all patients without taking into consideration gender differences.
Following administration of dDAVP, the same population showed a 7% increase in eGFR and a 10% increase in measured GFR, which did not reach statistical significance. As shown in Table 2, after the administration of dDAVP, the urine osmolarity increased by 210% (P < 0.01) and the urinary flow decreased by 72% (P < 0.01). This confirms that the dosage and time course of dDAVP were appropriate.
Variable . | CDI baseline . | CDI + dDAVP . | P-value (t-test) . |
---|---|---|---|
Plasma osmolarity (mOsm/kg) | 292 ± 4 | 284 ± 4 | 0.18 |
Urinary osmolarity (mOsm/kg) | 174 ± 10 | 556 ± 42 | ≪0.01 |
mGFR (mL/min/1.73 m2) | 86 ± 4 | 97 ± 6 | 0.14 |
eGFR (mL/min/1.73 m2) | 86 ± 6 | 92 ± 6 | 0.54 |
CrCl (mL/min) | 93 ± 5 | 101 ± 7 | 0.40 |
Volume (mL/min) | 6.8 ± 0.7 | 1.8 ± 0.1 | ≪0.01 |
Variable . | CDI baseline . | CDI + dDAVP . | P-value (t-test) . |
---|---|---|---|
Plasma osmolarity (mOsm/kg) | 292 ± 4 | 284 ± 4 | 0.18 |
Urinary osmolarity (mOsm/kg) | 174 ± 10 | 556 ± 42 | ≪0.01 |
mGFR (mL/min/1.73 m2) | 86 ± 4 | 97 ± 6 | 0.14 |
eGFR (mL/min/1.73 m2) | 86 ± 6 | 92 ± 6 | 0.54 |
CrCl (mL/min) | 93 ± 5 | 101 ± 7 | 0.40 |
Volume (mL/min) | 6.8 ± 0.7 | 1.8 ± 0.1 | ≪0.01 |
Data are presented as mean ± SEM.
mGFR, measured GFR (inulin method); eGFR, estimated by the CKD-EPI equation; CrCl, creatinine clearance.
Variable . | CDI baseline . | CDI + dDAVP . | P-value (t-test) . |
---|---|---|---|
Plasma osmolarity (mOsm/kg) | 292 ± 4 | 284 ± 4 | 0.18 |
Urinary osmolarity (mOsm/kg) | 174 ± 10 | 556 ± 42 | ≪0.01 |
mGFR (mL/min/1.73 m2) | 86 ± 4 | 97 ± 6 | 0.14 |
eGFR (mL/min/1.73 m2) | 86 ± 6 | 92 ± 6 | 0.54 |
CrCl (mL/min) | 93 ± 5 | 101 ± 7 | 0.40 |
Volume (mL/min) | 6.8 ± 0.7 | 1.8 ± 0.1 | ≪0.01 |
Variable . | CDI baseline . | CDI + dDAVP . | P-value (t-test) . |
---|---|---|---|
Plasma osmolarity (mOsm/kg) | 292 ± 4 | 284 ± 4 | 0.18 |
Urinary osmolarity (mOsm/kg) | 174 ± 10 | 556 ± 42 | ≪0.01 |
mGFR (mL/min/1.73 m2) | 86 ± 4 | 97 ± 6 | 0.14 |
eGFR (mL/min/1.73 m2) | 86 ± 6 | 92 ± 6 | 0.54 |
CrCl (mL/min) | 93 ± 5 | 101 ± 7 | 0.40 |
Volume (mL/min) | 6.8 ± 0.7 | 1.8 ± 0.1 | ≪0.01 |
Data are presented as mean ± SEM.
mGFR, measured GFR (inulin method); eGFR, estimated by the CKD-EPI equation; CrCl, creatinine clearance.
Untreated CDI patients showed a daily urinary Ca2+ excretion of 383 ± 47 mg/day, which is well above the threshold of 270 mg/day for males [23]. Furthermore, the urinary Ca:Cr ratio was 0.26 ± 0.38 mg/mg, whereas the threshold is 0.20 mg/mg. As shown in Figure 1, dDAVP treatment did not significantly modify the urinary Ca2+ concentration; however, daily calcium excretion was significantly decreased (383 ± 47 versus 160 ± 27 mg/day). Urinary calcium excretion in untreated patients, was also greater before than after receiving dDAVP (5.6 ± 0.75 versus 2.3 ± 0.39 mg/kg body weight). The urinary Ca:Cr ratio was also greater before dDAVP treatment (0.27 ± 0.03 versus 0.11 ± 0.02 mg/mg).
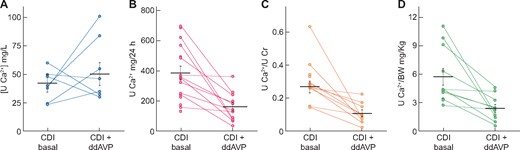
Difference in (A) urine calcium concentration, (B) 24-h urine calcium excretion, (C) urine Ca:Cr ratioand (D) urine Ca:body weight ratiobetween CDI patients under basal conditions and after dDAVP treatment. Horizontal lines represent mean ± SEM.
DISCUSSION
The main result of the present study is that, in CDI patients, dDAVP causes a significant reduction of daily excretion of urinary calcium as well as of the urinary Ca:Cr ratio. The baseline level of calcium daily excretion is >300 mg/24 h, which is above the reported thresholds for hypercalciuria (270 mg/day for males and 250 mg/day for females). The relation between AVP and calcium excretion is particularly intriguing given that both are altered with ageing [24] and microgravity/bed rest [25].
Urinary calcium excretion depends on several renal and extrarenal factors. Hypercalciuria is a common finding in hypertensive subjects and animals, potentially due to sodium and chloride reabsorption [26]. It is interesting to note the recent evidence that sodium excretion is modulated by uromodulin secretion [27] and that this, in turn, is modulated by CaSR [28], thus giving an additional mechanism of calcium–sodium interplay. This interplay seems relevant in some forms of hypertension [29]. Indeed, hypercalciuria is associated with sodium-sensitive hypertension [26], and also in this case, pendrin, a protein known to be associated with sodium-sensitive hypertension [29], as well as urinary calcium regulation [30]. Indeed, deletion of the pendrin gene results in hypercalciuria, possibly due to downregulation of sodium/clacium exchanger and epithelial calcium channel calcium-absorbing molecules in the kidney [30].
Other reports from patients and experimental models point to AVP as a regulator of urinary calcium excretion. Our study aims to address whether the administration of dDAVP in patients with no endogenous vasopressin secretion, as affected by CDI, present any variation in urinary calcium excretion after 7 days of dDAVP administration. In our model, the 1-week intranasal administration of dDAVP in patients with CDI caused a significant decrease in urinary volume, urinary sodium excretion and urinary potassium excretion, with a borderline increase in the eGFR. Indeed, the eGFR reflects both anatomical (nephron number) and functional (e.g. mesangial function) contributions [31, 32]. Several factors, such as reactive oxygen species, may functionally modulate the GFR [33]. The data on urinary volume and urinary sodium are in keeping with the well-known effects of AVP on diuresis and urinary sodium excretion, as well as with the data from four patients with CDI studied by Hanouna et al. [4]. In our study, urinary calcium excretion was affected by dDAVP administration together with a small, non-significant change in the clearance of inulin. This is in agreement with the reduction in urinary calcium reported by Hanouna et al. [4] obtained in four patients with CDI [4]. The relation between AVP and eGFR is further intriguing from the relationship that both have with cognitive functions [34].
However, in one of the patients studied by Hanouna et al. [4], creatinine clearance was severely impaired [4]. Finally, those patients were dehydrated, as indicated by their plasma sodium concentration (median 144.5 mmol/L) and osmolality (median 303.5 mOsm/L). It should be noted that in adults, nocturnal polyuria desmopressin increases whole-day calcium excretion, as happened in enuretic children [35]. In the present study, dDAVP significantly reduced urinary volume and increased urine osmolality and urinary sodium reabsorption in hypercalciuric patients allowed unrestricted calcium intake. This is at odds with data on enuretic children, with such an effect achieved only after reducing sodium and calcium intake for 3–6 months [7].
The present data are the results of clinical observations based on water and electrolyte excretion, therefore they are unable to shed light on the potential interplay between CaSR and aquaporin-2 as a pivotal mechanism proposed for hypercalciuric subjects [36, 37] to avoid calcium saturation in the urine. In rodents, AVP increases calcium reabsorption in the ascending Henle’s loop [3]. Specifically, the experiments of Bouby et al. [3] in the Brattleboro strain of rats with DI demonstrate a reduction in urinary calcium excretion after chronic dDAVP administration. This was associated with a trophic effect on the thick ascending limb of Henle’s loop [3]. Therefore, in these animals, the AVP-regulated calcium excretion is unlikely mediated by TRPC3, a member of the mammalian transient receptor potential (TRP) channel, which is expressed in the principal cells of collecting ducts in rats [36] and humans [37]. Conversely, studies in rabbits suggest involvement of the cortical collecting tubules in AVP-mediated calcium reabsorption [38]. However, in humans, regulation of calcium excretion is unlikely to occur at the level of the Henle’s loop or cortical collecting tubules, because AVP receptor is mainly located in the collecting duct [39]. Consistently, in humans, involvement of the collecting duct on AVP-regulated calcium excretion has been advocated [4].
The present study has several limitations to be considered. First, mutations of CaSR were not available at the time of the clinical study and the corresponding genetic techniques were not available. However, it is very unlikely that CaSR mutations cosegregate with CDI, because the latter has a central (brain) origin.
Similarly, other acquired and rare causes of hypercalciuria, such as hyperparathyroidism, vitamin D excess, granulomatous diseases (e.g. sarcoidosis, tuberculosis) and renal tubular acidosis (and the associated genetic forms [40]), could not be formally excluded, given the retrospective nature of the work. Indeed, since the plasma levels of calcium in these patients were normal and the urinary calcium levels in spot urine samples were below the normal values (due to the dilution of urine), no additional studies were required at the time of diagnosis. Thus it is very unlikely that these causes play a role in the effects of dDAVP.
The data suggest a trend for a positive association between urinary osmolality and inulin clearance. Most of the literature data suggest that a reduction in eGFR, as in chronic kidney disease, may cause a loss of urine concentrating capacity [41, 42]. The data from CDI patients would lend support for an inverted relationship: the change in urine osmolality caused by AVP may induce a change in GFR. This is also supported by the decrease in GFR when high hydration status (and hence low urine osmolarity) is induced in normal patients [43]. The present data also confirm that polyuria per se is associated with increased sodium excretion [43]. In conclusion, in hypercalciuric patients with CDI, urinary calcium handling is affected by dDAVP.
CONFLICT OF INTEREST STATEMENT
The authors state that there are no conflicts of interest.
Comments