-
PDF
- Split View
-
Views
-
Cite
Cite
Courtney R Lane, Judith Brett, Mark Schultz, Claire L Gorrie, Kerrie Stevens, Donna R M Cameron, Siobhan St George, Annaliese van Diemen, Marion Easton, Rhonda L Stuart, Michelle Sait, Anton Y Peleg, Andrew J Stewardson, Allen C Cheng, Denis W Spelman, Mary Jo Waters, Susan A Ballard, Norelle L Sherry, Deborah A Williamson, Finn Romanes, Brett Sutton, Jason C Kwong, Torsten Seemann, Anders Goncalves da Silva, Nicola Stephens, Benjamin P Howden, Search and Contain: Impact of an Integrated Genomic and Epidemiological Surveillance and Response Program for Control of Carbapenemase-producing Enterobacterales, Clinical Infectious Diseases, Volume 73, Issue 11, 1 December 2021, Pages e3912–e3920, https://doi.org/10.1093/cid/ciaa972
- Share Icon Share
Abstract
Multiresistant organisms (MROs) pose a critical threat to public health. Population-based programs for control of MROs such as carbapenemase-producing Enterobacterales (CPE) have emerged and evaluation is needed. We assessed the feasibility and impact of a statewide CPE surveillance and response program deployed across Victoria, Australia (population 6.5 million).
A prospective multimodal intervention including active screening, carrier isolation, centralized case investigation, and comparative pathogen genomics was implemented. We analyzed trends in CPE incidence and clinical presentation, risk factors, and local transmission over the program’s first 3 years (2016–2018).
CPE case ascertainment increased over the study period to 1.42 cases/100 000 population, linked to increased screening without a concomitant rise in active clinical infections (0.45–0.60 infections/100 000 population, P = .640). KPC-2 infection decreased from 0.29 infections/100 000 population prior to intervention to 0.03 infections/100 000 population in 2018 (P = .003). Comprehensive case investigation identified instances of overseas community acquisition. Median time between isolate referral and genomic and epidemiological assessment for local transmission was 11 days (IQR, 9–14). Prospective surveillance identified numerous small transmission networks (median, 2; range, 1–19 cases), predominantly IMP and KPC, with median pairwise distance of 8 (IQR, 4–13) single nucleotide polymorphisms; low diversity between clusters of the same sequence type suggested genomic cluster definitions alone are insufficient for targeted response.
We demonstrate the value of centralized CPE control programs to increase case ascertainment, resolve risk factors, and identify local transmission through prospective genomic and epidemiological surveillance; methodologies are transferable to low-prevalence settings and MROs globally.
Antimicrobial resistance (AMR) is a major threat to public health and patient safety. Carbapenemase-producing Enterobacterales (CPE) are considered one of the most serious classes of multiresistant organisms (MROs), requiring immediate and aggressive public health action [1]. The attributable mortality for invasive infections ranges from 29% to 75%, but improvement has not been observed despite development of novel therapies [2–6]. Most concerningly, CPE have considerable epidemic potential, and have been responsible for numerous large clonal hospital outbreaks [7, 8].
In Australia, CPE have rarely been identified and are usually associated with patients receiving overseas medical care in endemic countries, except for imipenemase (IMP)-4, which has established low-level endemicity in some states [9, 10]. Limited outbreaks of CPE within healthcare facilities have also been described [10–13]. Between 2012 and 2014 an outbreak of Klebsiella pneumoniae carbapenemase (KPC)–producing Enterobacterales occurred in Victoria (population 6.5 million) affecting multiple healthcare settings and facilities [14]. A statewide outbreak investigation coordinated by the Victorian Government Department of Health and Human Services identified multiple transmission networks involving inter- and intrafacility spread and determined that centralized collation of genomic and epidemiological data was needed to identify areas of CPE transmission due to the long colonization periods, asymptomatic transmission, and complex patient movements often experienced by patients with CPE [14].
In December 2015, the Victorian Guideline on Carbapenemase Producing Enterobacteriaceae for Health Services (the guideline) was released, implementing a comprehensive prospective genomic and epidemiological surveillance and response program for the control of CPE [15]. Here we describe the epidemiology of CPE in Victoria and assess the feasibility and effect of the first 3 years of the program.
METHODS
The Intervention
The guideline implemented a prospective, multimodal, population-based intervention for control of CPE (Supplementary Methods). Standardized active screening and carrier isolation were required in all Victorian health services accepting overnight patient admissions (Supplementary Box 1). All Victorian diagnostic laboratories servicing both inpatient and outpatient providers referred suspected CPE isolates to the state reference laboratory for confirmatory testing, whole-genome sequencing, and investigation (Supplementary Methods, Supplementary Box 2, Supplementary Figure 1). Epidemiological data were collected for all persons from whom an isolate with a confirmed carbapenemase gene originated, regardless of phenotypic susceptibility or clinical presentation [16]. Combined phylogenomic and epidemiological outbreak investigations to identify putative local transmission were conducted prospectively and iteratively where 2 or more patients with CPE isolates of the same species, multilocus sequence type (ST), and carbapenemase gene were identified, and at least 1 isolate was from a person not suspected to have acquired CPE overseas (Supplementary Methods, Supplementary Table 1). A transmission risk area (TRA) was declared where local transmission was thought to represent a risk to other persons present in the location for a defined time frame, necessitating increased screening and other infection-control actions (Supplementary Table 2).
Assessment of Program Impact
All confirmed CPE cases (a distinct carbapenemase gene, species, and/or ST combination in a given person) identified between January 2016 and December 2018 were included in analyses, performed using Stata/SE 13.1 (StataCorp, College Station, TX) or R version 3.5.1 [17]. To assess the effects of the program, rates of CPE identification and active clinical infection were calculated using population estimates for the respective time period and trends assessed using negative binomial or Poisson regression [18]. Associations between carbapenemase genes, risk factors, and clinical data were measured using a comparison of proportions (χ 2 and Fisher’s exact tests). P < .05 was considered statistically significant.
RESULTS
The occurrence of CPE remained low over the study period, with 402 cases identified in 362 people. Carbapenemase-producing Enterobacterales was considered a colonizing organism in 60% (n = 242/402) of cases (Supplementary Materials, Supplementary Table 3). Identified carbapenemase genes were diverse and varied by species (Supplementary Materials, Supplementary Table 4, Supplementary Figure 2).
Risk Factors for Carbapenemase-producing Enterobacterales Acquisition
Risk Factors Differ by Carbapenemase Gene
Overall, 95% (n = 379/399) of cases reported hospital admission in the previous 12 months, and 63% (n = 231/369) reported overseas travel in the past 4 years. Of these, 188 were suspected to have acquired their CPE overseas, representing 47% (n = 188/402) of all cases.
Risk factors differed significantly between gene groups (Figure 1), with overseas acquisition suspected in a higher proportion of New Delhi metallo-beta-lactamase (NDM) (n = 111/114, 93%), both NDM and oxacillinase (OXA)-48-like (n = 6/7, 86%), OXA-48–like (n = 60/75, 80%), and KPC (n = 11/46, 24%), when compared with IMP cases (n = 0/142, 0%; P < .001, Fisher’s exact). Region of travel differed by carbapenemase gene group (Figure 1).
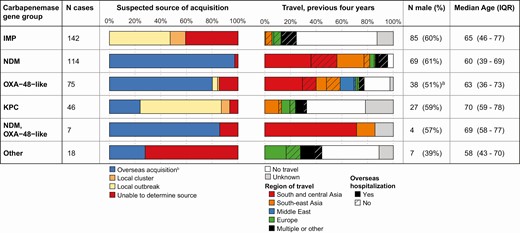
Risk factors for carbapenemase-producing Enterobacterales acquisition, by carbapenemase gene group: Victoria, Australia, 2016–2018. Abbreviations: IQR, interquartile range; IMP, imipenemase; NDM, New Delhi metallo-beta-lactamase; OXA, oxacillinase; KPC, Klebsiella pneumoniae carbapenemase.aUnknown sex, n = 1.bIncludes suspected transmission from a returned traveler.
KPC (n = 32/46, 70%), IMP (n = 84/142, 59%), and OXA-48–like (n = 4/75, 5%) cases were identified as part of defined local transmission networks based on genomic and epidemiological data (Figure 1). A source of acquisition was unable to be identified in 22% (n = 89/402) of cases, indicating potential unrecognized risk factors, colonized persons, and/or horizontal transfer of carbapenemase genes; however, this ranged from only 3% (n = 3/114) of NDM to 41% (n = 58/142) of IMP cases.
Non–Healthcare-related Acquisition of NDM-Producing Escherichia coli in Travelers Returning From South and South-East Asia
Overseas hospitalization is a known risk factor for CPE acquisition and a criterion for screening upon admission to a Victorian hospital. Of the 188 cases with suspected overseas acquisition 132 (72%, 5 unknown) reported hospital admission while overseas, and an additional 28 had attended an emergency department, medical, or dental clinic. The remaining 23 cases represent suspected overseas acquisition in the absence of healthcare contact. The majority (18/23 cases, 78%) involved NDM-producing Escherichiacoli, commonly NDM-5 (n = 14); 22 of the 23 cases travelled to South-East or South and Central Asia, most commonly India (n = 14) (Supplementary Table 5).
Cases with suspected non–healthcare-related acquisition overseas were significantly less likely to have been identified through screening (35%, n = 8/23) than other CPE cases (57%, n = 217/379) (P = .035), and most notably those with overseas hospitalization (73%, n = 117/160; P < .001).
Local Acquisition of Carbapenemase-producing Enterobacterales in Victoria
Centralized Combined Genomic and Epidemiological Surveillance Allows Identification of Small Transmission Networks Involving Cases Temporally and Geographically Dispersed at CPE Identification
A major aim of the program is early identification of putative local transmission. To enable timely action, time between isolate referral and initial assessment for local transmission was monitored, with a median delay of 11 days (interquartile range [IQR], 9–14 days) during the study period. Following process improvements, the median delay decreased from 15 days (IQR, 9–34 days) in 2016 to 10 days (IQR, 9–13 days) in 2018.
Among the 53% (n = 214/402) of cases where overseas acquisition was not suspected, routine prospective surveillance uncovered 28 clusters of genomic and epidemiologically linked cases, most commonly involving IMP-4–positive organisms (Figure 2). Identified transmission networks involved a median of 2 cases (range, 1–19) during the study period.
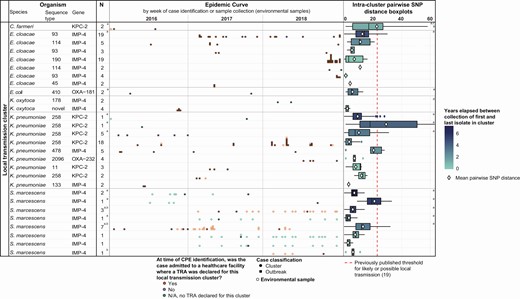
Temporal and genomic variation among CPE local transmission clusters: Victoria, Australia, 2016–2018. Note: Numbers (N) reported in the table include only human cases identified during the study period; however, clusters may include environmental isolates or presurveillance cases. Duplicate isolates are excluded from both epidemic curves and intracluster pairwise SNP distance boxplots, with environmental samples from the same ward in the same week considered duplicates. For further information, see Supplementary Materials. Abbreviations: CPE, carbapenemase-producing Enterobacterales; KPC, Klebsiella pneumoniae carbapenemase; IMP, imipenemase; NDM, New Delhi metallo-beta-lactamase; OXA, oxacillinase; SNP, single nucleotide polymorphism; TRA, transmission risk area.aIntracluster pairwise SNP distance boxplot includes human or environmental isolate(s) collected prior to the start of the study period.bTRA declared where patient is suspected to have acquired CPE from an environmental source. Collection of environmental isolate(s) must precede exposure of the patient(s) to the health service for TRA declaration to occur.cOutlying point at 72 SNPs not displayed for scale.dData not available, see Supplementary Table 1.
Previous studies have indicated that local transmission is suspected where isolates from 2 patients are within approximately 23 single nucleotide polymorphisms (SNPs) of each other [7, 19]. In our data, 97% (2296/2366) of intercluster pairwise isolate comparisons were below this threshold when a cluster was defined using both genomic and epidemiological data (median, 8; IQR, 4–13 SNPs) (Figure 2). However, where multiple clusters have been identified within a single species and ST, considerable overlap was observed between intra- and intercluster pairwise SNP comparisons (Figure 3), suggesting the use of a genomic cluster definition alone may merge epidemiologically dispersed cases, reducing power to identify risk factors and geographical or temporal focus of transmission.
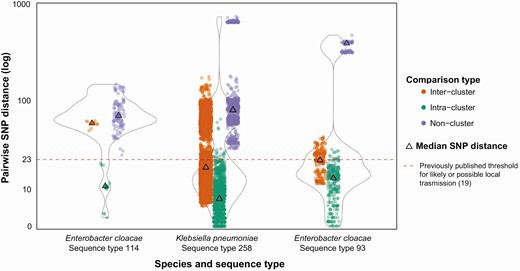
Inter- and intracluster genomic variation among carbapenemase-producing Enterobacterales local transmission clusters: Victoria, Australia, 2016–2018. Analyses include human or environmental isolate(s) of the relevant species and/or sequence type collected prior to the start of the study period as context isolates. Designated clusters must contain at least 1 human isolate collected during the study period (2016–2018). All nonclustered comparisons with ≤23 pairwise SNP distance involve only context isolates. Duplicate isolates are excluded, with environmental samples from the same ward in the same week considered duplicates. For further information, see Supplementary Materials. Abbreviation: SNP, single nucleotide polymorphism.
Twenty-eight location- and time-specific TRAs were identified across 18 of the 28 clusters, allowing targeted intervention and screening. One TRA was in a residential aged-care facility, with the remaining in single hospital wards. Organisms involved in TRAs varied by health service, with 13 of 18 (72%) IMP-4 TRAs, 6 of 8 (75%) KPC-2 TRAs, and 2 of 2 (100%) OXA-232 TRAs each occurring within different single health services. Interfacility collation and the combined use of genomic and epidemiological data were crucial in identifying transmission where patients were temporally and geographically dispersed on identification, including 6 TRAs involving patients identified with CPE postdischarge, in different health services or the community. Similarly, in 14 TRAs, more than 30 days elapsed between identification of CPE in the first and last cases involved in the transmission. The case study below further highlights the value of centralized intrafacility surveillance methods.
Case Study: Outbreak Investigation Leading to the Declaration of Transmission Risk Areas in 2 Hospitals
In July 2018, a patient with OXA-232–positive Klebsiella pneumoniae ST 2096 was identified without a history of hospitalization in a high-burden country (patient 3). Two cases of this gene, species, and ST combination had been seen previously, from patients 1 and 2, both reporting prior hospitalization in India (Supplementary Table 6). An outbreak investigation was undertaken and updated on identification of patients 4 and 5, including a phylogenetic analysis of isolates from all patients (Figure 4A). Bed-movement data were obtained from any hospital where multiple cases had attended and plotted against the phylogenetic tree (Figure 4B).
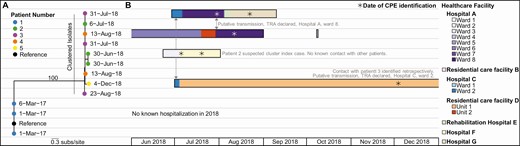
Case study: outbreak investigation of OXA-232–positive Klebsiella pneumoniae ST 2096, Victoria, Australia, 2016–2018. A, Phylogenetic tree of K. pneumoniae ST 2096 isolates colored by patient number and annotated with date of collection. Branch support values >99 are shown. B, Trace graph including hospital and ward of admissions for the corresponding patient. Admission data not displayed for duplicate isolates from the same patient. Abbreviations: CPE, carbapenemase-producing Enterobacterales; ST, sequence type; TRA, transmission risk area.
Analyzed together, centralized genomic and epidemiological surveillance enabled the following:
(1) identification of an outbreak of phylogenetically related CPE obtained from patients 2–5 over a 6-month period;
(2) inclusion of patient 5 in the outbreak, despite admission to a different health service at the time of CPE identification;
(3) exclusion of patient 1 from the outbreak;
(4) identification of patient 2 as the likely index case for this outbreak despite no known contact with other patients, suggestive of unrecognized intermediary transmission; and
(5) identification of putative transmissions in 2 different hospitals, despite identification of CPE in patient 5 at 6 months after likely transmission, resulting in the declaration of TRAs in 2 healthcare facilities.
Multiple Local Transmission Patterns Observed, Including Sustained Propagated Outbreaks and Suspected Environmental Acquisition
Among identified local outbreaks, multiple apparent patterns of local transmission were observed. Most notably, transmission of KPC-producing K. pneumoniae ST 258 persisted across all 3 years, causing small periodic outbreaks in facility B (Figure 2). All clusters of KPC-2–producing K. pneumoniae ST 258 observed were derived from outbreak clones first observed in 2012, with current clusters thought to represent epidemiological and genomic diversification over time and not separate importations [14]. A similar pattern has been observed within IMP-4–producing Enterobacter cloacae ST 93 and 114 clusters, in contrast with discrete, apparently time-limited transmissions, exemplified by IMP-4–producing Klebsiella oxytoca of novel ST (Figure 2). While plasmid transmission was not directly examined in local transmission investigations, 50 of 73 (68%) cases of an ST not associated with local clonal dissemination contained IMP-4, indicating limited plasmid transmission of other carbapenemases.
Following identification of environmental CPE contamination in some facilities, version 2 of the guideline incorporated suspected acquisition from an environmental source within the definition of a TRA. Four such TRAs were observed, all involving sink drains contaminated with IMP-4–positive S Serratia. marcescens within intensive care units across 2 health services.
Effects of Comprehensive Carbapenemase-producing Enterobacterales Surveillance
Targeting Patients Present at Time and Location of Suspected Transmission Increases Screening Yield
A TRA designation enables communication of the time and place of CPE transmission risk to other health services, with persons admitted to the TRA location during the designated period requiring isolation and screening on admission to any healthcare facility (Supplementary Table 2). Version 2 of the guideline increased follow-up of exposed patients, including a requirement to send letters informing patients of their CPE exposure [20]. Subsequently, the proportion of screened cases linked to a TRA increased 11-fold to 0.9% (n = 21/2394; P = .002, Fisher’s exact).
Intensified Screening Increases Case Ascertainment, Clinical Infections Stabilize
To assess the effect of the intervention, rates of CPE identification and active clinical infection were examined. Incidence of CPE increased by 13%/half-year (95% confidence interval [CI], 6–19%; P < .001) (Figure 5A) to 1.42 cases/100 000 population in the second half of 2018. Despite this, both the rate and number of cases identified as active clinical infections remained steady since 2017 (incidence rate ratio [IRR], .94; 95% CI, .73–1.21; P = .640) (Figure 5B), indicating the observed increase in rate of CPE cases is likely due to increased screening and case ascertainment. By the second half of 2018, 36% (n = 33/92) of cases were identified through infection-control interventions following case or transmission identification, such as contact screening (Figure 5B). This coincided with a steady decrease in cases where source of acquisition could not be determined, from 30% (n = 18/61) in the second half of 2016 to 15% (n = 14/78) in the second half of 2018 (χ 2, P = .033) (Figure 5C), indicating increased ascertainment and resolution of transmission networks.
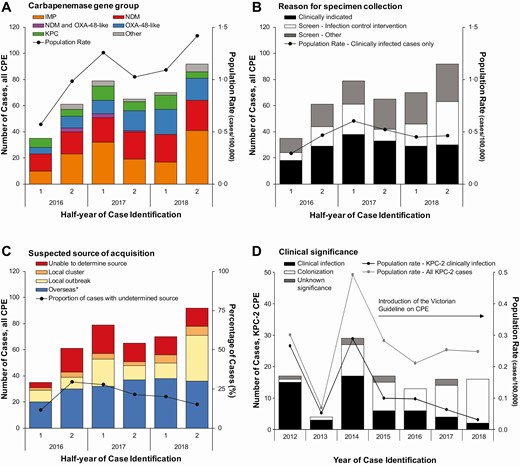
Epidemic curve and population rate of rates of CPE cases, by clinical and epidemiological characteristics: Victoria, Australia. A, Number of cases by carbapenemase gene group and population rate for all cases by half-year, 2016–2018. B, Number of cases by reason for specimen collection and population rate for clinically indicated cases by half-year, 2016–2018. C, Number of cases by suspected source of CPE acquisition and proportion of total cases where a source of acquisition could not be determined by half-year, 2016–2018. Local outbreak categorization includes patients with genomic and epidemiological links; “Overseas” includes patients with household contact with returned travelers. D, Number of cases by clinical significance and population rate of KPC-2 producing Enterobacterales pre- and postintervention, by year, 2012–2018. Abbreviations: CPE, carbapenemase-producing Enterobacterales; IMP, imipenemase; KPC, Klebsiella pneumoniae carbapenemase; NDM, New Delhi metallo-beta-lactamase; OXA, oxacillinase. *Includes suspected transmission from returned traveler.
No change in the population rate of KPC occurred over the surveillance period (IRR, 1.00; 95% CI, .85–1.19; P = .968). However, active clinical infections with KPC-2–producing Enterobacterales decreased significantly following the implementation of the program, from a high of 0.29 infections/100 000 population in 2014 to 0.03 infections/100 000 population in 2018 (P = .003). A nonsignificant decrease in total KPC-2 cases was observed over the same period (P = .554) (Figure 5D).
DISCUSSION
We have described the findings, feasibility, and impact of one of the first centralized comprehensive and systematic phylogenomic and epidemiological surveillance and response programs for a primarily healthcare-associated MRO globally [21, 22]. The aim of this program was to rapidly detect and control transmission of CPE in a low-prevalence setting. Implementation was possible due to an existing public health genomics program, enabling analysis to be completed in a time frame applicable for infection-control action, and through embedded cooperation between public health authorities, diagnostic laboratories, and healthcare facilities to implement responses. While resourcing and capacity for genomic surveillance, centralized case investigation, and patient screening differ between settings, we share this information to demonstrate the value of a statewide centralized “search and contain” intervention for a low-prevalence MRO [23].
Historically, Victoria has had limited central coordination regarding MROs, and outbreaks were managed within individual institutions. The alarming occurrence of a disseminated interfacility KPC outbreak necessitated action by public health authorities [14] and resulted in this comprehensive surveillance and response program. We have demonstrated the success of the program through increased case ascertainment with a steady rate of active clinical infections, a reduced number of cases where source of acquisition could not be determined, an increased proportion of cases identified through infection-control interventions, the identification of small transmission networks enabling early response, and through the significant decrease in KPC-2 clinical infections observed following program implementation.
As consistently demonstrated in retrospective outbreak studies, and in our present case study, the increased pathogen discrimination provided by genomic analysis enhances assessment of alternate transmission hypotheses generated by epidemiological investigation [14, 22, 24, 25]. Our results support this principle, demonstrating that due to CPE colonization patients involved in a transmission event can be temporally and/or geographically dispersed upon CPE identification, and such patients can have considerable intra-host genomic diversity, making genomic or epidemiological data alone insufficient to define transmission events. When used prospectively, this process has enabled us to identify the temporal and geographical focus of transmission networks early, while case numbers remain small, allowing targeted intervention and reducing the resources required for intense epidemiological data collection [19].
While the importation of CPE, particularly from South and Central Asia, and the predominance of IMP carbapenemase in local transmissions within Australia were anticipated [9, 10, 12, 13, 26], our results differed from those previously reported, in several ways. First, intensive case investigation identified travel to South and South-East Asia without healthcare contact as an emerging risk factor for NDM-producing E. coli; IMP transmission, thought to be endemic in Australia’s eastern states [26], and initially debated for inclusion in the program, affected only a small number of facilities; and intensive infection-control actions have resulted in a higher proportion of CPE cases identified through screening, and a lower occurrence of serious infections, than reported elsewhere [24].
There are several limitations to the current program. The system is resource intensive and sustainability may be threatened by accumulating case numbers, rising global incidence of CPE, and broadening risk factors for acquisition—all increasing the risk of CPE introduction and resources required for screening and isolation of at-risk patients [27–29]. While other comprehensive interventions have shown success in reducing incidence in outbreak or high-burden settings, rapid transmission detection and intervention may help maintain low prevalence, and costs must be assessed against the escalating economic burden of CRE infection demonstrated internationally [30, 31]. Program evaluation, including cost-effectiveness analysis, is underway. Screening all patients with travel to high-burden countries without healthcare contact, which we have identified as a risk factor for CPE acquisition, is infeasible, yet such patients may represent an ongoing threat of CPE introduction to our health services. We are encouraged by our results demonstrating increased case ascertainment through screening and low levels of active clinical infection; however, we may not identify all subsequent clinical infection in patients with known CPE colonization. Research into pathogen and host factors influencing CPE colonization in diverse, nonoutbreak settings is needed to focus resources for screening and isolation towards those at higher risk of ongoing carriage and transmission and within limits of duration of colonization [29, 32, 33].
Case ascertainment and sensitivity to detect transmission are strongly influenced by screening practices, which may differ between settings and facilities. While we have attempted to standardize screening of at-risk persons, transmissions may not be identified due to unrecognized colonized patients, difficulty in screening exposed contacts postdischarge or when presenting to another healthcare facility, and in identifying transmission in community settings. Further, we are currently unable to routinely determine transmissions that result from transfer of resistance elements between species and clones. This is under investigation for future action; however, the low proportion of cases where source was unable to be determined suggests this is not a dominant feature of CPE transmission in our setting. Finally, TRAs enable focused intervention but may be difficult to determine where large case numbers within a cluster result in multiple temporal and geographical overlaps between cases. In facilities or settings with higher CPE incidence, targeted investigation and control measures may not be feasible; protocols to identify and manage transmission in such settings are needed. Further research into infection-control interventions effective against persistent propagated outbreaks and environmental reservoirs is also required.
In an era when AMR threatens the stability of the health system globally, significant effort and resources are justified if they can reduce the burden of AMR, especially the highest-risk pathogens such as CPE. We have described the successful implementation of a comprehensive, centralized program, and some indicators of success. Further work is required to define the economic value of the intervention, and to further refine enhanced screening activities at the hospital level, enabled by the comprehensive surveillance data collected. The methodologies used are transferable to other low-prevalence settings and MROs globally and will be expanded to other emerging AMR threats, such as Candida auris.
Sequences used in phylogenetic analyses are available at NCBI BioProject PRJNA529744 and https://github.com/MDU-PHL/CPE-Paper.
Supplementary Data
Supplementary materials are available at Clinical Infectious Diseases online. Consisting of data provided by the authors to benefit the reader, the posted materials are not copyedited and are the sole responsibility of the authors, so questions or comments should be addressed to the corresponding author.
Notes
Author Contributions. F. R., B. S., B. P. H., A. v. D., N. S., M. E., C. R. L., J. B., D. R. M. C., and J. C. K. conceived, designed, and led the intervention. B. P. H., N. S., C. R. L., A.v. D., and M. E. designed the study. K. S., N. L. S., B. P. H., M. Sait, S. A. B., and D. A. W. led the microbiological testing and interpretation. M. Schultz, A. G. d. S., T. S., and C. L. G. led the bioinformatics analysis. J. B. and D. R. M. C. undertook data collection and advised on infection-control processes and interpretation. R. L. S., A. Y. P., A. J. S., A. C. C., D. W. S., and M. J. W. led and advised on health service actions. C. R. L. and S. S. G. analyzed the data. C. R. L. wrote the first draft of the manuscript. All authors reviewed the manuscript and approved and agreed to submit the final version of the manuscript.
Acknowledgments. The authors gratefully acknowledge the many staff at Victorian hospitals, medical clinics, and diagnostic laboratories who have collected data and performed testing for the surveillance of CPE in Victoria. We would particularly like to acknowledge the considerable efforts and input of infection-prevention and -control staff at affected healthcare facilities whose efforts have been critical for the control of CPE in Victoria. All data were collected in accordance with the Victorian Public Health and Wellbeing Act 2008. Ethical approval was obtained from the Human Ethics Advisory Group of the School of Biomedical Sciences, University of Melbourne (Ethics ID 1954615.2).
Financial support. This work was supported by the National Health and Medical Research Council through a partnership grant (grant number GNT1149991) and individual supporting grants (grant numbers GNT1142613 [to J. C. K.], GNT1123854 [to D. A. W.], GNT1105905 [to B. P. H.], GNT1141398 to [A. J. S]); N. L. S. and C. R. L. are supported by Australian Government Research Training Program scholarships and the Microbiological Diagnostic Unit Public Health Laboratory is funded by the Victorian Government.
Potential conflicts of interest. A. Y. P. reports an investigator-initiated research grant from MSD, outside the submitted work. All other authors report no potential conflicts.All authors have submitted the ICMJE Form for Disclosure of Potential Conflicts of Interest. Conflicts that the editors consider relevant to the content of the manuscript have been disclosed.