-
PDF
- Split View
-
Views
-
Cite
Cite
Takuo Minato, Tatsuya Kado, Ki-Seok Yoon, Seiji Ogo, Synthesis of a [NiRu] hydrogenase model complex with a modifiable OH group, Chemistry Letters, Volume 54, Issue 4, April 2025, upaf057, https://doi.org/10.1093/chemle/upaf057
- Share Icon Share
Abstract
Various types of artificial hydrogenase model complexes have been synthesized by imitating the active sites of natural [NiFe] hydrogenases. However, the control of their catalytic properties after synthesizing complexes by using modifiable groups is still difficult because predesigned modifiable groups can react easily with metal cations during multistep synthesis. In this study, we successfully synthesized a newly designed [NiRu] complex with modifiable OH groups, which could act as a homogeneous H2 oxidation catalyst by using Cu2+ as an electron acceptor.
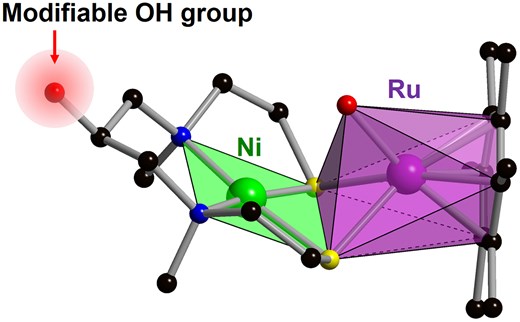
H2 is utilized not only as an energy source for fuel cells, but also as an attractive eco-friendly reducing agent that enables high atomic efficiency and produces only water as waste for various types of organic syntheses. Thus, catalytic H2 oxidation and hydrogenation are fairly important in industrial fields, including carbon dioxide fixation and fine chemical synthesis.1 In nature, on the other hand, it has been known that hydrogenases, which are mostly found in microorganisms, act as very efficient catalytic H2-uptake and H2-producing enzymes compared with artificial materials.2 For example, an immobilized hydrogenase from Citrobacter sp. S-77 shows much higher mass activity than Pt as an electrode for H2 oxidation.3 Hydrogenase can be phylogenetically classified into 3 distinct classes, including [Fe], [FeFe], and [NiFe] hydrogenases, and [NiFe] hydrogenases that possess [Ni(μ3-S)2Fe] cores are more active in H2 oxidation reactions.2,4 Although [NiFe] hydrogenases are potentially usable for catalytic H2 oxidation and hydrogenation in engineering, there are still the fundamental problems of low thermostability of protein and difficulty in mass production due to the complex biosynthesis route. Therefore, various types of artificial model complexes have been synthesized by mimicking the active sites of natural hydrogenases to achieve higher durability and catalytic activity.5 Especially in [NiFe] hydrogenase model complexes, we have synthesized several crucial structures, including [(C9H20N2)Ni2 + (μ3-S)2Ru2 + (H2O){η6-C6(CH3)6}]2+, [(C11H24N2)Ni2 + (μ3-S)2Fe2 + (CH3CN){P(OCH2CH3)}3]2+, and [(C11H24N2)Ni2 + (μ3-S)2Fe2 + (Cl)(CO){P2C2H4(C6H5)4}]+, and elucidated their high catalytic H2 oxidation properties, intermediate hydride structures, and catalytic mechanisms.6 These complexes can be utilized as homogeneous catalysts in aqueous solution and anode materials for fuel cells by mixing model complexes with graphene.7 We envisaged that the modification of catalytic properties after synthesizing these complexes and/or the immobilization of model complexes would be possible if modifiable groups could be introduced into the ligand. However, the introduction of modifiable groups whilst keeping the active site is challenging because predesigned modifiable groups can react easily with metal cations during multistep synthesis.
To synthesize the [NiRu] complex with a modifiable OH group, a new ligand N,N’-dimethyl-N,N’-bis(2-mercaptoethyl)-1,3-diamino-2-propanol (H2L, 2) was designed in this study. First, 2-hydroxy-N,N’-dimethyl-1,3-propanediammonium chloride (1) was synthesized by reacting 1,3-dichloro-2-propanol with methylamine (Fig. 1). The 1H NMR spectrum of 1 in DMSO-d6 showed a broad signal at 9.07 ppm that was assignable to 4 protons of secondary ammonium cations (Supplementary Fig. S1; see online Supplementary material for a color version of this figure). Elemental analysis of 1 also supported the successful synthesis of 1. As ligand 1 was ammonium salt, 2-hydroxy-N,N’-dimethyl-1,3-propanediamine (1′) was prepared by reacting 1 with 2 equivalents of NaOH in ethanol, and the newly designed multidentate ligand 2 was then synthesized by reacting in-situ-formed 1′ with ethylene sulfide in acetonitrile (Fig. 1).
![Synthetic scheme of the [NiRu] complex with a modifiable OH group.](https://oup.silverchair-cdn.com/oup/backfile/Content_public/Journal/chemlett/54/4/10.1093_chemle_upaf057/1/m_upaf057f1.jpeg?Expires=1750190042&Signature=pNIT9vZNSH6TOl9eYqNGEcqLk~LtRPJWfBe98L3tQVuK5NWiC3-7OfG6ewP-ObIr7K5VJD7jr0-1eK8tiUppI6rfwJrM6JEoGlLS7~3FnfrrYDA-naGY9kpGfgum2fuMz70Gx2cduskU12HenETlR1L53w7m-vssQcprokRvtXL4Xr2A1QH9pjZ5NffE6Kwnjm109dv~jC85CsbZ9ZNGxdw0tJwNZwJbTN0i88H14bOuyeLn~LytAK1G636bZlp1AmrMFxk8jRLjAd16AWZ8YnkX1jTS190p0m5WK19BBJfMsl-bFQVpW5doxCO0NzdG2rXgLX38UdwBP9VjykjlcA__&Key-Pair-Id=APKAIE5G5CRDK6RD3PGA)
Synthetic scheme of the [NiRu] complex with a modifiable OH group.
As 2 possessed 5 coordination sites (2S, 2N, and 1O), controlling the reactivity of these sites is thought to be a key factor to synthesize model complexes with a modifiable group. When utilizing acetone as a solvent, an Ni3L2 complex (3′) in which Ni was coordinated with all of 5 coordination sites was obtained (Supplementary Table S1 and Supplementary Figs. S2 and S3; see online Supplementary material for color versions of the table and figures). On the other hand, when utilizing a mixed solvent of acetone and methanol, the desired NiL complex (3) was successfully synthesized, presumably because the addition of the protic solvent with a pKa value that was between those of the SH and the secondary OH groups prevented undesired coordination with the least-reactive modifiable OH group (Fig. 1). X-ray crystallographic analysis of the product revealed that Ni was coordinated with 2N and 2S to form the square planner geometry (Fig. 2a and Supplementary Table S1; see online Supplementary material for a color version of this table). Importantly, the OH group was not reacted, indicating that this moiety can be utilized as a linker in the following reactions. The bond valence sum (BVS) value of Ni (1.83) indicates that the valence is +2. The electrospray ionization (ESI) mass spectrum of 3 in water showed sets of signals at m/z 317.1 and 613.1 that were assignable to [(C9H20N2NiOS2)Na]+ and [(C9H20N2NiOS2)2Na]+, respectively, indicating that 3 is stable in water (Supplementary Fig. S4; see online Supplementary material for a color version of this figure). Based on the above-mentioned results and elemental analysis, the formula of 3 is C9H20N2NiOS2. Finally, 3 was reacted with [Ru{η6-C6(CH3)6}(H2O)3](NO3)2 in water and hygroscopic red single crystals were obtained from a mixed solvent of dichloromethane, methanol, and diethyl ether (Fig. 1). X-ray crystallographic analysis revealed that the product was an [NiRu] complex with a modifiable OH group (4) as designed (Fig. 2b and Supplementary Table S1; see online Supplementary material for a color version of this table). The BVS value of Ni (1.93) indicates that the valence is +2. Although 1 of 2 counter anions of NO3– was coordinated with Ru in a crystal state, the ESI mass spectrum of 4 in methanol showed sets of signals at m/z 557.1, 593.0, and 620.1 that were assignable to [Ni(C9H19N2S2O)Ru(C12H18)]+, [Ni(C9H19N2S2OH)Ru(C12H18)(H2O)(OH)]+, and [Ni(C9H19N2S2OH)Ru(C12H18)(NO3)]+, respectively, indicating that the coordinated NO3– would be dissociated in a solution state (Supplementary Fig. S5; see online Supplementary material for a color version of this figure). Based on the above-mentioned results and elemental analysis, the formula of 4 is C21H38N4NiO7RuS2. Therefore, the [NiRu] hydrogenase model complex with a modifiable OH group was successfully synthesized by designing a new multidentate ligand and controlling the reactivities of the coordination sites.
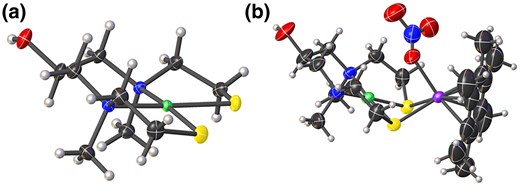
ORTEP representations of a) 3 and b) 4 with thermal ellipsoids drawn at the 50% probability level. Black, white, blue, yellow, red, green, and purple ellipsoids represent C, H, N, S, O, Ni, and Ru atoms, respectively. Non-coordinated NO3– anion in 4 was omitted for clarity.
We have recently synthesized and characterized a [NiRu] hydrogenase model complex without modifiable groups ([(C9H20N2)Ni2 + (μ3-S)2Ru2 + (H2O){η6-C6(CH3)6}](NO3)2, 4′) that acted as a homogeneous H2 oxidation catalyst in water.6a Although the crystal structure of 4′ was determined to be a sulfate salt, the bond lengths and bond angles of the [Ni(μ3-S)2Ru] cores of 4 were very close to those of 4′ (Supplementary Table S2; see online Supplementary material for a color version of this table), indicating that 4 would also act as a H2 oxidation catalyst. Density functional theory (DFT) calculations showed that the shapes of the highest-occupied molecular orbitals (HOMOs) and lowest-unoccupied molecular orbitals (LUMOs) of 4 were the same, and a difference was observed in LUMO+6, which is thought to be rarely involved in the H2 oxidation reaction (Fig. 3). Additionally, the HOMO–LUMO gap of 4 (3.896 eV) was very close to that of 4′ (3.857 eV) (Fig. 3). These observations strongly supported that the electronic states of 4 are fairly similar to those of 4′, regardless of the modifiable OH group, indicating the potential catalysis of 4 for H2 oxidation.
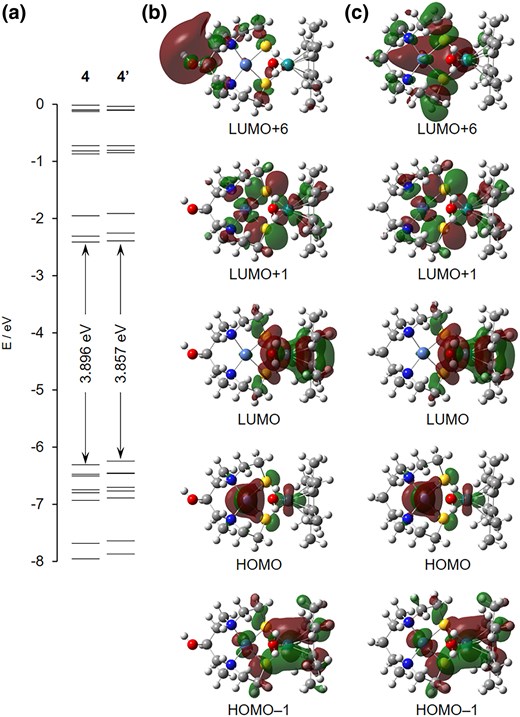
a) Energy diagrams and LUMO+6, LUMO+1, LUMO, HOMO, and HOMO–1 of b) 4 and c) 4′, in which NO3– ligand coordinated with Ru of 4 was replaced by H2O ligand. The orbitals are represented by dark red and green lobes. The atoms are represented by spheres: Ni, pale blue; Ru, turquoise; C, gray; H, white; N, blue; O, red; S, yellow.
To reveal the catalytic ability of 4, an H2 oxidation reaction by using Cu2+ as an electron acceptor was examined (see the online Supplementary Information for details).6a,8 The catalytic reactions were performed by using 0.5 mol% of 4 in acetate buffer solution (25 mM, pH = 4.0) at 323 K under H2 (0.8 MPa) for 1 h, resulting in the successful reduction of Cu2+. The turnover number (TON) of the reaction (TON = 12) was close to that of 4′ (TON = 14), showing that 4 could act as a homogeneous H2 oxidation catalyst that even possesses a modifiable OH group.
In conclusion, we report the synthesis of the [NiRu] complex with a modifiable OH group ([(C9H19N2OH)Ni2 + (μ3-S)Ru2 + (NO3){η6-C6(CH3)6}](NO3), 4) as a model complex of the active site in [NiFe] hydrogenase by designing a new multidentate ligand (N,N’-dimethyl-N,N’-bis(2-mercaptoethyl)-1,3-diamino-2-propanol, 2). A key strategy to synthesize 4 with a modifiable OH group was the addition of methanol in the reaction of Ni2+ with 2. Complex 4 showed homogeneous H2 oxidation catalysis, which was expected via the DFT calculations. The modifiable OH group of 4 is expected to facilitate further modifications, enabling both the precise control of catalytic properties and immobilization for practical applications.
Supplementary data
Supplementary material is available at Chemistry Letters online.
Funding
This work was supported in part by JST CREST (grant number JPMJCR18R2), Japan, JSPS KAKENHI (grant numbers JP18H02091, JP18J00191, and JP26000008) (Specially Promoted Research), MEXT Leading Initiative for Excellent Young Researchers (grant number JPMXS0320200400), and the World Premier International Research Centre Initiative (WPI), Japan. T.M. was supported by the JSPS through a Research Fellowship for Young Scientists.
References
Author notes
Conflict of interest statement. None declared.