-
PDF
- Split View
-
Views
-
Cite
Cite
Yang Hu, Gang Ji, Guanya Li, Peter Manza, Wenchao Zhang, Jia Wang, Ganggang Lv, Yang He, Zhida Zhang, Kai Yuan, Karen M von Deneen, Antao Chen, Guangbin Cui, Huaning Wang, Corinde E Wiers, Nora D Volkow, Yongzhan Nie, Yi Zhang, Gene-Jack Wang, Brain Connectivity, and Hormonal and Behavioral Correlates of Sustained Weight Loss in Obese Patients after Laparoscopic Sleeve Gastrectomy, Cerebral Cortex, Volume 31, Issue 2, February 2021, Pages 1284–1295, https://doi.org/10.1093/cercor/bhaa294
- Share Icon Share
Abstract
The biological mediators that support cognitive-control and long-term weight-loss after laparoscopic sleeve gastrectomy (LSG) remain unclear. We measured peripheral appetitive hormones and brain functional-connectivity (FC) using magnetic-resonance-imaging with food cue-reactivity task in 25 obese participants at pre, 1 month, and 6 month after LSG, and compared with 30 normal weight controls. We also used diffusion-tensor-imaging to explore whether LSG increases brain structural-connectivity (SC) of regions involved in food cue-reactivity. LSG significantly decreased BMI, craving for high-calorie food cues, ghrelin, insulin, and leptin levels, and increased self-reported cognitive-control of eating behavior. LSG increased FC between the right dorsolateral prefrontal cortex (DLPFC) and the pregenual anterior cingulate cortex (pgACC) and increased SC between DLPFC and ACC at 1 month and 6 month after LSG. Reduction in BMI correlated negatively with increased FC of right DLPFC-pgACC at 1 month and with increased SC of DLPFC-ACC at 1 month and 6 month after LSG. Reduction in craving for high-calorie food cues correlated negatively with increased FC of DLPFC-pgACC at 6 month after LSG. Additionally, SC of DLPFC-ACC mediated the relationship between lower ghrelin levels and greater cognitive control. These findings provide evidence that LSG improved functional and structural connectivity in prefrontal regions, which contribute to enhanced cognitive-control and sustained weight-loss following surgery.
Introduction
Neuroimaging studies have reported brain activation in regions associated with reward and cogntive control in response to food cues in obese patients compared with controls (Volkow et al. 2008; Garcia-Garcia et al. 2013). Implicated regions include the prefrontal cortex (PFC), particularly the dorsolateral PFC (DLPFC) (Hare et al. 2009; Lavagnino et al. 2016), anterior cingulate cortex (ACC) (Rosenbaum et al. 2008; Wang, Kojori, et al. 2019a), and mesolimbic regions (Hare et al. 2009; Lavagnino et al. 2016). The PFC is crucial for executive function and cognitive control (Hare et al. 2009; Hu et al. 2016; Lavagnino et al. 2016) and the DLPFC recruits inhibitory control to modulate eating behavior in response to tempting food (Batterink et al. 2010; Lopez et al. 2019). The ACC is known to regulate physiological arousal, which is highly relevant to the control of food craving during cue exposure (Zhang et al. 2012, 2014) and individual variation in impulse control (Zhang et al. 2015). Structural brain abnormalities have also been identified in obese subjects, including reduced gray matter volume in regions associated with executive-function, food-intake, reward processing, and emotion regulation (i.e., DLPFC, caudate, hippocampus, and insula) (Raji et al. 2010; Zhang et al. 2016), as well as lower white matter integrity in the corpus callosum and corona radiate (Verstynen et al. 2012; Zhang et al. 2016). Impairments in these brain circuits in obesity have been associated with elevated food craving (Pepino et al. 2009), overeating, and weight gain (Pepino et al. 2009).
Bariatric surgery, including Roux-en-Y gastric bypass (RYGB) and laparoscopic sleeve gastrectomy (LSG), is the most effective treatment for morbid obesity and producing sustained weight-loss (Diamantis et al. 2014). LSG reduces the size of the stomach in an attempt to limit caloric intake during the immediate postsurgical period (Diamantis et al. 2014). After bariatric surgery, patients are instructed to follow a staged progression diet initially consisting of low-calorie liquids followed by pureed and soft foods and then “normal food” (Laurenius et al. 2012). Thus, decreased energy intake is a main driver for the initial weight-loss phase post-LSG.
Neuroimaging studies have examined functional/structural brain changes in obese patients 1 month postsurgery (LSG, RYGB), revealing alterations in homeostatic and hedonic neurocircuits, including an association between lower postoperative craving for high-calorie (HiCal) food and diminished activity within mesolimbic areas, DLPFC and ACC (Ochner et al. 2011; Ochner et al. 2012; Li et al. 2019). Bariatric surgery (LSG, RYGB) also decreased resting-state functional connectivity (FC) within the default-mode network comprising the ventromedial PFC, posterior cingulate cortex, hippocampus, ACC, superior frontal gyrus, and orbitofrontal cortex (OFC) (Ochner et al. 2011; Ochner et al. 2012; Li et al. 2019; Zhang et al. 2019). These findings suggest that individuals with obesity exhibit stronger activation of frontal regions (DLPFC and ACC) to food-cue stimulation (Batterink et al. 2010; Lopez et al. 2019), and that bariatric surgery normalizes it. In addition, studies showed that bariatric surgery (LSG) induced structural recovery of gray matter and white matter density, white matter integrity, and cortical morphometry in frontal regions including DLPFC, OFC, and ACC (Zhang et al. 2016; Liu et al. 2019).
Studies examining bariatric surgery-induced changes in food preferences have reported postsurgical reductions in high-fat, high-sugar, and unhealthy foods, and an increased intake of healthy foods (Laurenius et al. 2012; Molin et al. 2017). Six months post-LSG, obese subjects report less enjoyment and desire for sweet/fatty food (Van Vuuren et al. 2017). Eating behaviors are influenced both by hunger and the rewarding value of food (Shin and Berthoud 2011) and modulated by self-regulation (Hare et al. 2009; Lavagnino et al. 2016). A shift away from the consumption of high-fat/sweet food as well as decreased food craving after bariatric surgery (Shin and Berthoud 2011) suggests that surgery might affect the function of brain regions involved with food reward, craving, and cognitive self-regulation, thus contributing to weight-loss. Indeed, there is evidence that higher order self-regulation processes play important roles in weight management (Gettens and Gorin 2017; Neseliler et al. 2019). Futhermore, changes in peripheral apptitive hormones may lead to modulation not only in eating behaviors but also in the activity of brain circuits implicated in reward and self-regulation (Rosenbaum et al. 2008; Goldstone et al. 2014; Heni et al. 2014; Li et al. 2019). Ghrelin administration and fasting have similar acute stimulatory effects on hedonic responses and on the activation of cortico-limbic reward-cognitive systems during food evaluation (Goldstone et al. 2014). Reductions in fasting plasma ghrelin levels have also been observed postsurgery (Tsoli et al. 2013) and were associated with decreased craving and attenuated DLPFC activation to HiCal food-cues at 1 month postsurgery (Li et al. 2019). A small pilot placebo-controlled study indicated that leptin administration reversed several changes, previously induced by weight loss, in the food-cue reactivity in numerous brain areas involved in emotional and cognitive control of food intake (Rosenbaum et al. 2008). Increased plasma insulin levels and its negative association with PFC activation were reported in obese subjects after ingesting glucose versus water (Heni et al. 2014). One fMRI study demonstrated a positive association between OFC-putamen functional connectivity and fasting insulin levels, and a negative correlation between OFC-putamen functional connectivity and insulin sensitivity (Kullmann et al. 2012). However, the biological mediators underlying self-regulation, craving, hormonal signaling, and reduced consumption of HiCal foods following LSG remain incompletely understood. While it has been reported that LSG results in a decreases in serum concentrion of ghrelin, leptin, and insulin (Li et al. 2019; Salman et al. 2020), published studies showing the neural correlates of these changes in appetitive hormones concentrations after LSG are limited.
Most prospective studies on bariatric surgery have been done 1–3 months postsurgery when diet changes dramatically, which might confound the interpretation of results (Snyder-Marlow et al. 2010; Al-Najim et al. 2018). For example, a recent study assessing body-weight changes postsurgery showed greater weight-loss within the first 6 months postsurgery than during the subsequent 6 months (Antonopulos et al. 2019). In addition, prior imaging studies have assessed only one time point postsurgery, so they have been unable to evaluate brain changes at different time points from surgery such as the temporal sequence of functional/structural changes (Hao et al. 2013; Wang, Ji, et al. 2019b). Here, we aim to investigate the sequence of functional/structural alterations in the frontal-limbic circuits and their association with hormonal/behavioral changes and weight-loss in obese patients before and following LSG. For this purpose, obese patients undergoing LSG were tested pre-LSG and at 1 and 6 months post-LSG and compared with normal weight (NW) controls. We employed fMRI to first localize the frontolimbic brain regions activated by food-cues in the LSG group. We then used these regions as seed masks for deterministic tractography using diffusion-tensor-imaging (DTI) to calculate structural connectivity (SC) and used psychophysiological interaction (PPI) analyses to assess functional connectivity (FC) between these regions. We hypothesized that LSG would improve SC/FC of prefrontal regions involved in food-cue reactivity in obese individuals and that these improvements would be associated with changes in peripheral appetitive hormones (ghrelin, leptin, and insulin) and with decreased food craving, improved eating behaviors, and sustained weight-loss.
Materials and Methods
Participants
The protocol was approved by the Institutional-Review-Board of Xijing Hospital the Fourth-Military-Medical-University in Xi’an, China and registered in the Chinese-Clinical-Trial-Registry-Center (ChiCTR-OOB-15006346; http://www.chictr.org.cn) and conducted in accordance with the Helsinki Declaration. All participants provided written informed consent. Patients with psychiatric/neurological diseases, previous intestinal surgery/inflammatory/intestinal disease/organ dysfunction, or taking any current medication that could affect the central nervous system, and individuals with a waist circumference > than the interior diameter of the MRI scanner were excluded (Zhang et al. 2016, 2019; Li et al. 2018, 2019; Liu et al. 2019). Twenty-five LSG candidates completed the presleeve gastrectomy MRI scan (PreLSG) and underwent LSG surgery. The same MRI scans were performed 1 month (PostLSG_1) and 6 months postsurgery (PostLSG_6). Thirty NW subjects were recruited from the local community as the control group who underwent the same interviews and MRI scanning procedures at one time point. The LSG and NW groups were matched for age and gender (P > 0.05, Table 1).
. | NW (30) (Mean ± SE) . | LSG (25) (Mean ± SE) . | NW versus PreLSG . | ||||
---|---|---|---|---|---|---|---|
. | PreLSG . | PostLSG_1 . | PostLSG_6 . | T . | P . | ||
Age (years) | 26.20 ± 1.33 | 27.36 ± 1.71 | 27.36 ± 1.71 | 27.40 ± 1.69 | −0.543 | 0.589 | |
*Gender | 12 M/18F | 12 M/13F | 12 M/13F | 12 M/13F | N/A | 0.551 | |
BMI (Kg/m2) | 21.31 ± 0.47 | 38.78 ± 0.75 | 34.41 ± 0.75 | 29.03 ± 0.80 | −19.748 | <0.001 | |
YFAS | 1.73 ± 0.26 | 5.20 ± 0.44 | 3.20 ± 0.41 | 2.20 ± 0.37 | −7.028 | <0.001 | |
HAMD | 6.97 ± 0.88 | 13.92 ± 2.21 | 11.88 ± 1.50 | 7.32 ± 1.09 | −2.927 | 0.006 | |
HAMA | 5.00 ± 0.65 | 11.92 ± 1.58 | 8.64 ± 1.18 | 6.28 ± 1.17 | −4.045 | <0.001 | |
HiCal craving | 49.83 ± 5.17 | 64.92 ± 5.22 | 40.00 ± 4.97 | 31.36 ± 5.40 | −2.039 | 0.046 | |
LoCal craving | 62.30 ± 3.03 | 46.92 ± 4.50 | 46.40 ± 4.81 | 34.16 ± 5.17 | 2.917 | 0.005 | |
**Ghrelin (pg/ml) | 431.82 ± 35.95 | 381.77 ± 36.79 | 298.27 ± 40.26 | 210.41 ± 36.02 | 0.097 | 0.336 | |
**Insulin (pg/ml) | 162.91 ± 24.44 | 491.75 ± 73.90 | 258.05 ± 40.77 | 215.36 ± 28.01 | −4.225 | <0.001 | |
**Leptin (pg/ml × 103) | 2.60 ± 0.64 | 13.44 ± 1.66 | 5.56 ± 1.01 | 3.19 ± 0.07 | −6.085 | <0.001 | |
***TFEQ | Cognitive control | 11.10 ± 0.62 | 7.00 ± 0.85 | 9.57 ± 1.07 | 11.79 ± 1.16 | 3.978 | <0.001 |
***TFEQ | Disinhibition | 5.00 ± 0.51 | 7.93 ± 0.40 | 6.14 ± 0.75 | 5.57 ± 0.60 | −4.167 | <0.001 |
***TFEQ | Hunger | 3.14 ± 0.50 | 7.14 ± 0.59 | 5.36 ± 0.89 | 4.00 ± 0.50 | −5.103 | <0.001 |
. | NW (30) (Mean ± SE) . | LSG (25) (Mean ± SE) . | NW versus PreLSG . | ||||
---|---|---|---|---|---|---|---|
. | PreLSG . | PostLSG_1 . | PostLSG_6 . | T . | P . | ||
Age (years) | 26.20 ± 1.33 | 27.36 ± 1.71 | 27.36 ± 1.71 | 27.40 ± 1.69 | −0.543 | 0.589 | |
*Gender | 12 M/18F | 12 M/13F | 12 M/13F | 12 M/13F | N/A | 0.551 | |
BMI (Kg/m2) | 21.31 ± 0.47 | 38.78 ± 0.75 | 34.41 ± 0.75 | 29.03 ± 0.80 | −19.748 | <0.001 | |
YFAS | 1.73 ± 0.26 | 5.20 ± 0.44 | 3.20 ± 0.41 | 2.20 ± 0.37 | −7.028 | <0.001 | |
HAMD | 6.97 ± 0.88 | 13.92 ± 2.21 | 11.88 ± 1.50 | 7.32 ± 1.09 | −2.927 | 0.006 | |
HAMA | 5.00 ± 0.65 | 11.92 ± 1.58 | 8.64 ± 1.18 | 6.28 ± 1.17 | −4.045 | <0.001 | |
HiCal craving | 49.83 ± 5.17 | 64.92 ± 5.22 | 40.00 ± 4.97 | 31.36 ± 5.40 | −2.039 | 0.046 | |
LoCal craving | 62.30 ± 3.03 | 46.92 ± 4.50 | 46.40 ± 4.81 | 34.16 ± 5.17 | 2.917 | 0.005 | |
**Ghrelin (pg/ml) | 431.82 ± 35.95 | 381.77 ± 36.79 | 298.27 ± 40.26 | 210.41 ± 36.02 | 0.097 | 0.336 | |
**Insulin (pg/ml) | 162.91 ± 24.44 | 491.75 ± 73.90 | 258.05 ± 40.77 | 215.36 ± 28.01 | −4.225 | <0.001 | |
**Leptin (pg/ml × 103) | 2.60 ± 0.64 | 13.44 ± 1.66 | 5.56 ± 1.01 | 3.19 ± 0.07 | −6.085 | <0.001 | |
***TFEQ | Cognitive control | 11.10 ± 0.62 | 7.00 ± 0.85 | 9.57 ± 1.07 | 11.79 ± 1.16 | 3.978 | <0.001 |
***TFEQ | Disinhibition | 5.00 ± 0.51 | 7.93 ± 0.40 | 6.14 ± 0.75 | 5.57 ± 0.60 | −4.167 | <0.001 |
***TFEQ | Hunger | 3.14 ± 0.50 | 7.14 ± 0.59 | 5.36 ± 0.89 | 4.00 ± 0.50 | −5.103 | <0.001 |
*Gender: chi-square test.
**Ghrelin, Insulin, Leptin: LSG (n = 22), NW (n = 24).
***TFEQ: LSG (n = 14), NW (n = 21).
Notes: LSG, laparoscopic sleeve gastrectomy; PreLSG, obese patients who received MRI scan before surgery; PostLSG_1, obese patients who received MRI scan at 1 month after surgery; PostLSG_6, obese patients who received MRI scan at 6 month after surgery; NW, normal weight; BMI, body mass index; YFAS, Yale Food Addiction Scale; HiCal, high-calorie; LoCal, low-calorie; HAMD, Hamilton Depression Rating Scale; HAMA, Hamilton Anxiety Rating Scale; TFEQ, three factors of eating questionnaire.
. | NW (30) (Mean ± SE) . | LSG (25) (Mean ± SE) . | NW versus PreLSG . | ||||
---|---|---|---|---|---|---|---|
. | PreLSG . | PostLSG_1 . | PostLSG_6 . | T . | P . | ||
Age (years) | 26.20 ± 1.33 | 27.36 ± 1.71 | 27.36 ± 1.71 | 27.40 ± 1.69 | −0.543 | 0.589 | |
*Gender | 12 M/18F | 12 M/13F | 12 M/13F | 12 M/13F | N/A | 0.551 | |
BMI (Kg/m2) | 21.31 ± 0.47 | 38.78 ± 0.75 | 34.41 ± 0.75 | 29.03 ± 0.80 | −19.748 | <0.001 | |
YFAS | 1.73 ± 0.26 | 5.20 ± 0.44 | 3.20 ± 0.41 | 2.20 ± 0.37 | −7.028 | <0.001 | |
HAMD | 6.97 ± 0.88 | 13.92 ± 2.21 | 11.88 ± 1.50 | 7.32 ± 1.09 | −2.927 | 0.006 | |
HAMA | 5.00 ± 0.65 | 11.92 ± 1.58 | 8.64 ± 1.18 | 6.28 ± 1.17 | −4.045 | <0.001 | |
HiCal craving | 49.83 ± 5.17 | 64.92 ± 5.22 | 40.00 ± 4.97 | 31.36 ± 5.40 | −2.039 | 0.046 | |
LoCal craving | 62.30 ± 3.03 | 46.92 ± 4.50 | 46.40 ± 4.81 | 34.16 ± 5.17 | 2.917 | 0.005 | |
**Ghrelin (pg/ml) | 431.82 ± 35.95 | 381.77 ± 36.79 | 298.27 ± 40.26 | 210.41 ± 36.02 | 0.097 | 0.336 | |
**Insulin (pg/ml) | 162.91 ± 24.44 | 491.75 ± 73.90 | 258.05 ± 40.77 | 215.36 ± 28.01 | −4.225 | <0.001 | |
**Leptin (pg/ml × 103) | 2.60 ± 0.64 | 13.44 ± 1.66 | 5.56 ± 1.01 | 3.19 ± 0.07 | −6.085 | <0.001 | |
***TFEQ | Cognitive control | 11.10 ± 0.62 | 7.00 ± 0.85 | 9.57 ± 1.07 | 11.79 ± 1.16 | 3.978 | <0.001 |
***TFEQ | Disinhibition | 5.00 ± 0.51 | 7.93 ± 0.40 | 6.14 ± 0.75 | 5.57 ± 0.60 | −4.167 | <0.001 |
***TFEQ | Hunger | 3.14 ± 0.50 | 7.14 ± 0.59 | 5.36 ± 0.89 | 4.00 ± 0.50 | −5.103 | <0.001 |
. | NW (30) (Mean ± SE) . | LSG (25) (Mean ± SE) . | NW versus PreLSG . | ||||
---|---|---|---|---|---|---|---|
. | PreLSG . | PostLSG_1 . | PostLSG_6 . | T . | P . | ||
Age (years) | 26.20 ± 1.33 | 27.36 ± 1.71 | 27.36 ± 1.71 | 27.40 ± 1.69 | −0.543 | 0.589 | |
*Gender | 12 M/18F | 12 M/13F | 12 M/13F | 12 M/13F | N/A | 0.551 | |
BMI (Kg/m2) | 21.31 ± 0.47 | 38.78 ± 0.75 | 34.41 ± 0.75 | 29.03 ± 0.80 | −19.748 | <0.001 | |
YFAS | 1.73 ± 0.26 | 5.20 ± 0.44 | 3.20 ± 0.41 | 2.20 ± 0.37 | −7.028 | <0.001 | |
HAMD | 6.97 ± 0.88 | 13.92 ± 2.21 | 11.88 ± 1.50 | 7.32 ± 1.09 | −2.927 | 0.006 | |
HAMA | 5.00 ± 0.65 | 11.92 ± 1.58 | 8.64 ± 1.18 | 6.28 ± 1.17 | −4.045 | <0.001 | |
HiCal craving | 49.83 ± 5.17 | 64.92 ± 5.22 | 40.00 ± 4.97 | 31.36 ± 5.40 | −2.039 | 0.046 | |
LoCal craving | 62.30 ± 3.03 | 46.92 ± 4.50 | 46.40 ± 4.81 | 34.16 ± 5.17 | 2.917 | 0.005 | |
**Ghrelin (pg/ml) | 431.82 ± 35.95 | 381.77 ± 36.79 | 298.27 ± 40.26 | 210.41 ± 36.02 | 0.097 | 0.336 | |
**Insulin (pg/ml) | 162.91 ± 24.44 | 491.75 ± 73.90 | 258.05 ± 40.77 | 215.36 ± 28.01 | −4.225 | <0.001 | |
**Leptin (pg/ml × 103) | 2.60 ± 0.64 | 13.44 ± 1.66 | 5.56 ± 1.01 | 3.19 ± 0.07 | −6.085 | <0.001 | |
***TFEQ | Cognitive control | 11.10 ± 0.62 | 7.00 ± 0.85 | 9.57 ± 1.07 | 11.79 ± 1.16 | 3.978 | <0.001 |
***TFEQ | Disinhibition | 5.00 ± 0.51 | 7.93 ± 0.40 | 6.14 ± 0.75 | 5.57 ± 0.60 | −4.167 | <0.001 |
***TFEQ | Hunger | 3.14 ± 0.50 | 7.14 ± 0.59 | 5.36 ± 0.89 | 4.00 ± 0.50 | −5.103 | <0.001 |
*Gender: chi-square test.
**Ghrelin, Insulin, Leptin: LSG (n = 22), NW (n = 24).
***TFEQ: LSG (n = 14), NW (n = 21).
Notes: LSG, laparoscopic sleeve gastrectomy; PreLSG, obese patients who received MRI scan before surgery; PostLSG_1, obese patients who received MRI scan at 1 month after surgery; PostLSG_6, obese patients who received MRI scan at 6 month after surgery; NW, normal weight; BMI, body mass index; YFAS, Yale Food Addiction Scale; HiCal, high-calorie; LoCal, low-calorie; HAMD, Hamilton Depression Rating Scale; HAMA, Hamilton Anxiety Rating Scale; TFEQ, three factors of eating questionnaire.
Experimental Design
All participants underwent 12-h of overnight fasting, and fasting blood samples were taken and MRI scans were performed between 9 AM and 10 AM.
Hormone Measurements
Blood samples during fasting state were collected at PreLSG, PostLSG_1, and PostLSG_6, and stored at −80 °C until assayed. A Bio-Plex 200TM suspension array system (BIO-RAD, Inc.) was employed to measure the plasma concentrations of total ghrelin, leptin, and insulin during the fasting state (Supplementary Material provides detailed information). The blood of 3 obese subject and 6 NW controls had hemolysis; thus, we were not able to do the blood testing in these participants.
Questionnaires
A designated clinician rated the severity of the subjects’ anxiety and depression using the Hamilton Scales (Hamilton 1959, 1960). Subjects were required to complete the Yale Food Addiction Scale (YFAS) (Gearhardt et al. 2009) and the Three Factor Eating Questionnaire (TFEQ), which assess three domains of eating behavior: cognitive control, disinhibition, and hunger (Stunkard and Messick 1985) (Table 1). Clinical measurements were identically conducted at PreLSG, PostLSG_1, and PostLSG_6 in LSG, and in NW at baseline. TFEQ data were only available for 14 subjects, and therefore, all analyses with TFEQ were limited to these 14 participants. Surgical procedures in all the patients were performed by the same surgeon.
fMRI Food-Cue-Reactivity Task
To localize the regions of interest (ROIs) involved in food-cue processing, we employed a food-cue-reactivity paradigm (Li et al. 2019) with HiCal and LoCal food pictures selected from the International Affective Picture System (Bradley and Lang 2007) and others (Stoeckel et al. 2008) presented in 3 blocks per category in pseudorandom order. Each block lasted 30 s, during which 10 pictures were presented for 3 s each without intertrial interval and with 30 s between the blocks.
MRI Acquisition
The experiment was carried out using a 3 T Signa Excite HD (GE) scanner. A gradient-echo T2*-weighted echo-planar-imaging-sequence was used for acquiring functional images (TR = 2000 ms, TE = 30 ms, matrix size = 64 × 64, FOV = 256 × 256 mm2, flip angle = 90°, isotropic resolution of 4 mm3, and 32 axial slices), and the scan lasted 360 s. Diffusion-weighted images were acquired using a single-shot spin-echo echo-planar-imaging sequence. Diffusion sensitizing gradients were applied along 60 noncollinear directions (b = 1000 s/mm2) with 10 acquisitions without diffusion weighting (b = 0 s/mm2), 75 continuous axial slices, field-of-view = 256 × 256 mm2, TR = 9400 ms, TE = 84 ms, and matrix size = 128 × 128, resulting in 2-mm isotropic voxels.
Functional ROI Definition and FC Analysis
All imaging data were analyzed using Statistic Parametric Mapping 12 (SPM12, http://www.fil.ion.uclac.uk/spm). Preprocessing consisted of slice-timing, head movement correction, co-registration, spatial normalization (resolution 3 × 3 × 3mm3) and smoothing (Gaussian kernel of 6 mm at full width half maximum) (Li et al. 2019). A general linear model including HiCal and LoCal food-cue condition regressors was constructed. ANOVA was used to assess time effects on contrast images for HiCal versus LoCal food cues in the LSG group. Finally, the clusters with significant time effects in LSG were selected as the seed regions, and then, whole-brain PPI analyses were performed to investigate alterations in task-related FC in response to HiCal versus LoCal food cues. Results were corrected for multiple comparisons using family wise error (FWE) corrections at the cluster level correction approach (PFWE < 0.05) with a minimum cluster size of k = 50 voxels and a cluster defining threshold of P < 0.001.
DTI Tractography Analysis
The ROIs were defined based on the SPM analysis and used in a two ROIs approach for DTI deterministic tractography in native space (Garcia-Gorro et al. 2018). Preprocessing of the DTI data was performed with the FMRIB Software Library (FSL, http://www.fmrib.ox.ac.uk/fsl). First, brain extraction was performed using the FSL Brain Extractor Tool (Smith 2002) to delete nonbrain tissue. Head motion and eddy-current correction were performed using the FMRIB’s Diffusion Toolbox. DTIFIT in FMRIB’s Diffusion Toolbox was used to fit a diffusion tensor model at each voxel of the preprocessed eddy current corrected diffusion weighted data and to calculate the fractional anisotropy (FA), and mean (MD), axial (AD), and radial (RD) diffusivity maps.
Fiber tracking was carried out using the Diffusion Toolkit and fiber tracts were visualized using Trackvis (www.trackvis.org). The Fiber Assignment by Continuous Tracking algorithm (Mori et al. 1999) was used to reconstruct the total collection of white matter tracts of the brain. Fiber tracking was stopped when the fiber reached a voxel with an FA value lower than 0.2, when the trajectory of the traced fiber exceeded the brain or when the eigenvector had an average angle of >45° with the main diffusion direction of the neighboring voxels. We created ROIs according to food cue-reactivity activation for each hemisphere based on a single or a combination of regions within the automated anatomical labeling (AAL) template (van den Bos et al. 2015) to ensure that the regions were sufficiently large to enable reliable tractography estimates (Uddin et al. 2011; van den Bos et al. 2015). For example, for the DLPFC (left/right) ROI, we merged the Superior and Middle Frontal (left/right) ROIs in the AAL template; for the ACC (left/right) ROI, we used the ACC (left/right) ROIs in the AAL template. Due to the fact that fiber tracking must be conducted in the individual's diffusion space, all ROIs in MNI standard space were transformed to individual diffusion space (Lei et al. 2014). Briefly, the individual FA images were first co-registered to the T1-weighted images, and they were then nonlinearly normalized to the symmetric MNI152 T1 template in MNI space using FMRIB’s Nonlinear Image Registration Tool (FNIRT, http://www.fmrib.ox.ac.uk/fsl). Finally, the inverse transformations were applied to the ROI in MNI space to obtain the ROIs in individual diffusion space. The fiber tracts connecting the ROIs in each hemisphere were extracted from the total collection of brain fibers, which were obtained through the two-ROI approach with logical AND concatenation. Only fibers that passed through both ROIs were included in the reconstructed fiber tract. Spurious fibers were removed from the fiber tract by using an additional avoidance ROI (logical NOT operation). Finally, the values of the mean FA, MD, AD, and RD of the remaining fiber tracts connecting each pair of ROIs were extracted.
Statistical analyses were performed using IBM SPSS (Statistical Package for Social Sciences, Release 22.0, Chicago: SPSS, IL). In the LSG group, we assessed the effects of time (PreLSG, PostLSG_1, PostLSG_6) on SC between ROIs using a repeated measure ANOVA. Additionally, we used two-sample t-tests to compare NW with PreLSG, NW with PostLSG_1, and NW with PostLSG_6 (P < 0.016).
Correlation Analysis
Partial correlation, conducted with age and gender as covariates (Gong et al. 2011; Inano et al. 2011; Tomasi and Volkow 2012; Zhang et al. 2016), was used to assess the association between FC/SC of the frontal-limbic areas and BMI, and between baseline/LSG-related changes in frontal-limbic connectivity (FC/SC) and BMI. Bonferroni correction was applied for multiple-comparisons, and level of significance was set at P < 0.0125.
Mediation Analysis
Mediation analysis was used to assess whether the relationship between two variables (i.e., ghrelin and cognitive control) could be explained by the values from a third variable (SC or FC), where there were six path models (Kober et al. 2010). For example, if SC is a full mediator between ghrelin and cognitive control, then this relationship would become insignificant when SC is controlled for in the model. According to standard convention (Kober et al. 2010), "a" refers to ghrelin-SC effect, "b" refers to SC-cognitive control effect, "c" refers to the total ghrelin-cognitive control effect, and "c’" refers to the direct ghrelin-cognitive control effect, controlling for the mediator changes in SC. The product "a*b" tests the significance of the mediator. As is customary, we used a bootstrapping test (5000 steps) (Preacher and Hayes 2004) for the statistical significance of the product "a*b." A significant mediating effect is defined as a 95% confidence interval that does not include zero. The present mediation analysis was implemented using SPSS macros.
Results
Demographic Characteristics
At baseline, there were no significant differences in age and gender between LSG and NW groups (P > 0.05, Table 1). The PreLSG relative to NW group had significantly higher body mass index (BMI, P < 0.001), YFAS (P < 0.001), HAMA (P < 0.001), HAMD (P = 0.006), craving for HiCal food-cues (P = 0.046), TFEQ-disinhibition (P < 0.001) and TFEQ-hunger (P < 0.001), and lower craving for LoCal food-cues (P = 0.005) and TFEQ-cognitive control (P < 0.001).
In LSG group, there were significant effects of time on BMI (F(2,48) = 242.25, P < 0.001), HAMA (F(2,48) = 15.17, P < 0.001), craving for HiCal food-cues (F(2,48) = 16.97, P < 0.001), and YFAS (F(2,48) = 20.39, P < 0.001) due to significant LSG-induced reductions in BMI (PreLSG vs. PostLSG_1:t = 22.01, P < 0.001; PreLSG vs. PostLSG_6: t = 17.45, P < 0.001; PostLSG_1 vs. PostLSG_6: t = 11.01, P < 0.001), HAMA ratings (PreLSG vs. PostLSG_1: t = 3.32, P = 0.003; PreLSG vs. PostLSG_6: t = 4.67, P < 0.001), craving for HiCal food-cues (PreLSG vs. PostLSG_1: t = 3.79, P < 0.001; PreLSG vs. PostLSG_6: t = 5.95, P < 0.001), YFAS (PreLSG vs. PostLSG_1: t = 4.17, P < 0.001; PreLSG vs. PostLSG_6: t = 5.32, P < 0.001), and disinhibition (PreLSG vs. PostLSG_1: t = 2.27, P = 0.041; PreLSG vs. PostLSG_6: t = 3.45, P = 0.004) over time (Supplementary Table 1). There were also significant effects of time on HAMD (F(2,48) = 7.23, P = 0.002), hunger (F(2,26) = 7.23, P = 0.003), and cognitive control (F(2,26) = 7.91, P = 0.002), due to LSG-induced reductions in HAMD rating (t = 3.26, P = 0.003) and hunger (t = 4.29, P < 0.001), and increases in cognitive control (t = −3.51, P = 0.004) in the LSG group at PostLSG_6 compared with PreLSG. PostLSG_6 compared with PostLSG_1 also had lower BMI (t = 11.01, P < 0.001), HAMD (t = 3.49, P = 0.002), HAMA (t = 2.75, P = 0.011), and YFAS (t = 2.29, P = 0.031) ratings (Supplementary Table 1). There were no significant effects of time on craving for LoCal food-cues (F(2,48) = 3.01, P = 0.058). Thus, BMI and cravings for HiCal food-cues showed sustained reductions, whereas cognitive control showed sustained increases across the three timepoints (Fig. 1A). LSG also induced sustained decreases in plasma total ghrelin (PreLSG vs. PostLSG_1:t = 3.07, P = 0.006; PostLSG_1 vs. PostLSG_6: t = 2.57 P = 0.018) and leptin levels (PreLSG vs. PostLSG_1:t = 6.01, P < 0.001; PostLSG_1 vs. PostLSG_6: t = 3.55, P = 0.002). Insulin levels also showed significant decreases at PostLSG_1 (t = 2.86, P = 0.009, Fig. 1B, Table 1 and Supplementary Table 1).
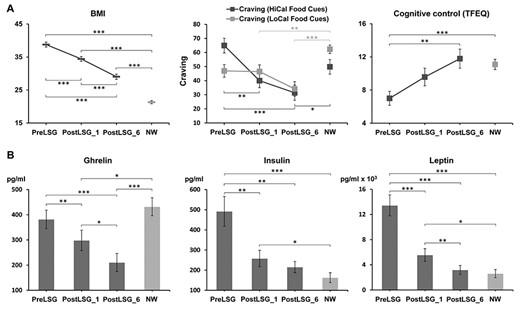
LSG-induced changes in BMI, peripheral hormones, and behaviors. (A) BMI showed sustained reduction across the three points and a trend toward the level of the NW group. Craving for HiCal food-cues also showed continued decreases across the three time points. Cognitive control showed sustained increases across the three time points and was equivalent to that in NW group at PostLSG_6. (B) Ghrelin, insulin, and leptin showed sustained decreases across the three time points, and insulin and leptin were equivalent to those in NW group at PostLSG_6. Notes: LSG, laparoscopic sleeve gastrectomy, BMI, body mass index, NW, normal weight, HiCal, high-calorie, LoCal, low-calorie; PreLSG, obese patients who received MRI scan before surgery; PostLSG_1, obese patients who received MRI scan at 1 month after surgery; PostLSG_6, obese patients who received MRI scan at 6 months after surgery.
Despite significant reductions in BMI post-LSG, BMI remained higher in the LSG group PostLSG_1 (t = −14.80, P < 0.001) and PostLSG_6 (t = −8.70, P < 0.001, Supplementary Table 1) compared with NW. Similarly, YFAS scores (t = −3.12, P = 0.003), HAMD (t = −2.82, P = 0.008), HAMA (t = −2.70, P = 0.010), and hunger ratings (t = −2.33, P = 0.026) remained higher PostLSG_1 and PostLSG_6 compared with NW (Supplementary Table 1). In contrast, craving for HiCal (NW vs. PostLSG_1: t = 1.36, P = 0.18; NW vs. PostLSG_6: t = 2.46, P = 0.017) and LoCal (NW vs. PostLSG_1; t = 2.79, P = 0.008; NW vs. PostLSG_6: t = 4.7, P < 0.001) food-cue were lower for PostLSG_1 and PostLSG_6 than for NW (Supplementary Table 1).
Food-Cue-Induced Brain Responses and LSG-Induced Changes in FC
There were significant effects of time on brain responses to HiCal versus LoCal food-cues in the right DLPFC (F(2,48) = 20.50, P < 0.001, Supplementary Table 2). PPI analysis showed significant time effects on FC between right DLPFC and pregenual ACC (pgACC) (Fig. 2A, Supplementary Table 3). Post hoc tests showed significant sustained increases in FC of DLPFC-pgACC at PostLSG_1 (t = −3.29, P = 0.003) and PostLSG_6 (t = −5.31, P < 0.001, Fig. 2A, Supplementary Table 3), and FC was equivalent to that in NW at PostLSG_6. Changes in FC of DLPFC-pgACC showed a negative correlation with reduction in BMI at PostLSG_1 (r = −0.55, P = 0.004, Fig. 3A), and changes in FC of DLPFC-pgACC showed a negative correlation with reduction in craving for HiCal food-cues (r = −0.44, P = 0.026, Fig. 3C) but not with reduction in BMI (r = 0.22, P = 0.295, Fig. 3C) at PostLSG_6. The correlation slopes between changes in FC and the reduction in HiCal food-cues craving and BMI were significantly different (z = 2.3, P = 0.021, Fig. 3C).
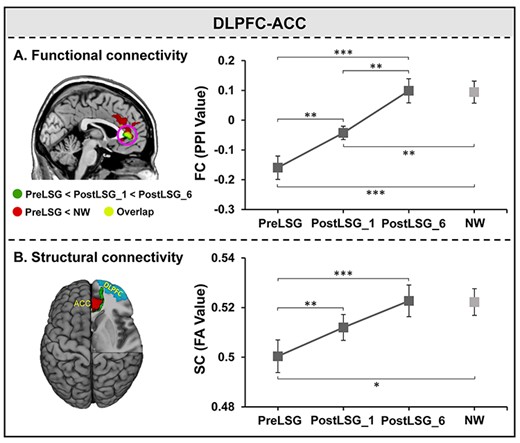
Repeated measure ANOVAs for FC and SC between DLPFC and ACC. (A) Post hoc tests showed significant sustained increases in FC between DLPFC and pgACC at PostLSG_1 and PostLSG_6, and FC was equivalent to that in NW at PostLSG_6. (B) Post hoc tests showed significant sustained increases in SC between the right DLPFC and ACC at both PostLSG_1 and PostLSG_6, and SC was equivalent to that in NW at PostLSG_6. Notes: DLPFC, dorsolateral prefrontal cortex; ACC, anterior cingulate cortex, NW, normal weight; HiCal, high-calorie; PreLSG, obese patients who received MRI scan before surgery; PostLSG_1, obese patients who received MRI scan at 1 month after surgery; PostLSG_6, obese patients who received MRI scan at 6 month after surgery; PPI, psychophysiological interaction; FA, fractional anisotropy.
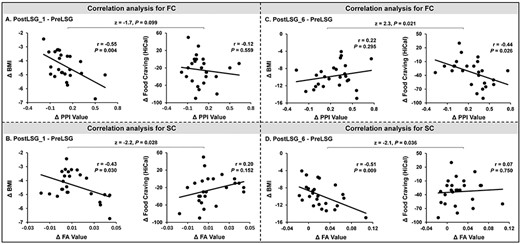
Correlations between functional/structural connectivity and behavioral measures at PostLSG_1 and PostLSG_6. (A) LSG-related increases in FC between the right DLPFC and pgACC showed a negative correlation with reduction in BMI but not with reduced craving for HiCal food-cues at PostLSG_1. (B) Increases in SC in the right DLPFC-ACC showed a negative correlation with reduction in BMI but not reduced craving for HiCal food cues at PostLSG_1. The correlation slopes for reduction in HiCal food cues and BMI were significantly different. (C) LSG-related increases in FC between the right DLPFC and pgACC showed a negative correlation with reduction in craving for HiCal food-cues but not reduced BMI at PostLSG_6. (D) Increases in SC in the right DLPFC-ACC showed a negative correlation with reduction in BMI but not reduced craving for HiCal food cues at PostLSG_6. The correlation slopes for reduction in HiCal food-cues and BMI were significantly different. Notes: BMI, body mass index; PreLSG, obese patients who received MRI scan before surgery; PostLSG_1, obese patients who received MRI scan at 1 month after surgery; PPI, psychophysiological interaction; FA, fractional anisotropy.
LSG-Induced Changes in SC
Deterministic tractography identified SC between the right DLPFC and ACC across all subjects (F(2,48) = 9.69, P < 0.001, Supplementary Table 4). Post hoc tests showed significant sustained increases in SC between DLPFC and ACC at PostLSG_1 (t = −3.71, P = 0.001) and PostLSG_6 (t = −3.81, P < 0.001, Fig. 2B, Supplementary Table 4), and SC was equivalent to that in NW at PostLSG_6 (Fig. 2B). Reduction in BMI showed a negative correlation with increases in SC of right DLPFC-ACC at both PostLSG_1 (r = −0.43, P = 0.030, Fig. 3B) and PostLSG_6 (r = −0.51, P = 0.009, Fig. 3D). There was no significant correlation between increases in DLPFC-ACC SC and reduction in craving for HiCal food-cuesat PostLSG_6, and the two correlation slopes differed (z = −2.1, P = 0.036, Fig. 3D). In addition, SC of the right DLPFC-ACC was positively correlated with TFEQ-cognitive control (r = 0.75, P = 0.002) and negatively correlated with ghrelin levels (r = −0.66, P = 0.009). TFEQ-cognitive control was negatively correlated with ghrelin levels (r = −0.63, P = 0.016) at PostLSG_6 but not at PreLSG and PostLSG_1 (Fig. 4A). The mediation analyses revealed that the relationship between ghrelin levels and cognitive control (c = −0.0218 ± 0.0077, P = 0.016) was mediated by FA of DLPFC-ACC (c’ = −0.0083 ± 0.0089, P = 0.37; a*b: 95% CI [−0.0455 to −0.0034], Fig. 4B), and there were no significant mediating effects in the other five path models (Supplementary Fig. 1). There were no significant correlations among the FC/SC of DLPFC-ACC, TFEQ-cognitive control and ghrelin (Supplementary Fig. 2), as well as leptin (Supplementary Fig. 3) and insulin (Supplementary Fig. 4). There were no significant changes in MD, RD, and AD.
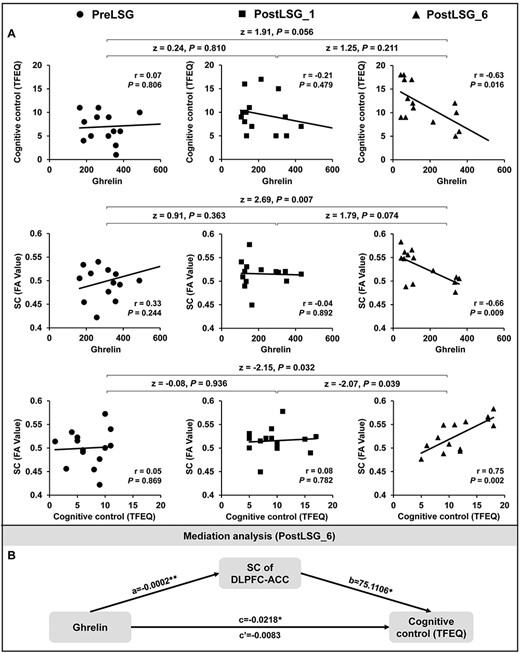
Mediation analysis among SC of the DLPFC-ACC, TFEQ-cognitive control, and ghrelin levels in 14 obese patients. (A) SC of the DLPFC-ACC was significantly positively correlated with TFEQ-cognitive control and negatively correlated with ghrelin levels, and TFEQ-cognitive control was negatively correlated with ghrelin levels at PostLSG_6 but not at PreLSG and PostLSG_1. (B) Mediation analyses showing that the realtionship between ghrelin levels and cognitive control was mediated by SC of DLPFC-ACC. Notes: PreLSG, obese patients who received MRI scan before surgery; PostLSG_1, obese patients who received MRI scan at 1 month after surgery; PostLSG_6, obese patients who received MRI scan at 6 months after surgery; FA, fractional anisotropy; TFEQ, three factors of eating questionnaire.
Discussion
In the current study, we employed fMRI with a food-cue-reactivity task and DTI to investigate sustained FC/SC changes in the frontal-limbic circuit and LSG-induced behavioral, peripheral hormones and BMI changes in obese patients undergoing LSG. LSG significantly decreased BMI, craving for HiCal food-cues, ghrelin, insulin, and leptin levels, and increased cognitive control. Analyses revealed that LSG was associated with sustained increases in FC/SC between the DLPFC and the ACC, brain regions implicated in self-regulation and salience-attribution, which could account for the observed association with reductions in BMI and food craving. Specifically, imaging measures revealed significant increases in FC between DLPFC and pgACC and in SC between DLPFC and ACC at both PostLSG_1 and PostLSG_6, compared with preLSG. FC of DLPFC-pgACC was negatively correlated with reductions in BMI at PostLSG_1 and with reductions in HiCal food craving at PostLSG_6. BMI reduction was also negative correlated with increases in SC in the right DLPFC-ACC tract at PostLSG_1 and PostLSG_6. In addition, mediation analysis revealed that the relationship between ghrelin and cognitive control was mediated by FA of DLPFC-ACC at PostLSG_6.
LSG-Induced Changes in DLPFC Activation in Response to HiCal versus LoCal Food-Cues
DLPFC plays an important role in the central regulation of eating behavior (Hare et al. 2009; Lavagnino et al. 2016) and in down regulating the motivation to consume desirable food (Hare et al. 2009; Lavagnino et al. 2016). In healthy adults, DLPFC exhibits hyperactivation during visual HiCal versus LoCal food stimulation (Killgore et al. 2003) and when individuals voluntarily suppress the desire to eat in response to food cues (Batterink et al. 2010; Hollmann et al. 2012). Our results showed significantly decreased brain activation in the right DLPFC in response to HiCal versus LoCal food-cues post-LSG. These data are in line with prior studies conducted 1 month after gastric bypass surgery (Ochner et al. 2011), including our recent work indicating that reduced ghrelin post-LSG might underlie changes in brain reactivity and eating behaviors (Li et al. 2019). Thus, some of the beneficial effects of LSG may reflect the normalization of DLPFC hyperactivity in response to HiCal food-cues.
LSG-Induced Increases in FC of DLPFC-pgACC
We performed PPI analysis to assess the FC of the right DLPFC and its associated brain regions during food-cue stimulation. Right DLPFC showed enhanced FC with the pgACC under HiCal food-cues at both PostLSG_1 and PostLSG_6. The ACC is implicated in evaluating the salience of information and modulating one’s emotional response (Bush et al. 2000). The pgACC, a rostral portion of the ACC in Brodmann Area 32, is associated with cognition and anxiety (Palomero-Gallagher et al. 2019) and activated by higher mental functions such as cognitive control (Walter 2012). Our prior study using positron emission tomography (PET) imaging with 18F-flurodeoxyglucose and a food stimulation paradigm showed that obese subjects increased energy utilization in the pgACC during attempted cognitive-inhibition (Weygandt et al. 2019). This region has also been reported to reduce reactivity to food-cues after dietary treatment (Weygandt et al. 2019). Furthermore, PET studies reported that bariatric surgery increased μ opioid receptor availability in the pgACC (Karlsson et al. 2016) and in healthy controls μ receptor availability in pgACC mediated its reactivity to palatable versus nonpalatable food-cues (Nummenmaa et al. 2018).
High-order executive-control processes have been shown to play an important role in healthy food decisions and in weight management (Gettens and Gorin 2017; Neseliler et al. 2019). The executive-control network, one of the three most relevant brain circuits underlying food decisions, relies on the function of interconnected prefrontal regions including ACC and DLPFC (Dagher 2012). Increased FC between DLPFC and pgACC may reflect an enhanced cognitive-modulation of DLPFC to pgACC (Palomero-Gallagher et al. 2019). The negative correlation between reduction in BMI and increased DLPFC-pgACC FC at 1 month post-LSG may indicate a greater modulation of the pgACC by the DLPFC, which might reduce compulsive overeating and BMI. At 6 months post-LSG, the association between increases in DLFPC-pgACC FC and decreases in BMI was not significant (putatively suggesting long-term adaptations), but these FC increases were associated with decreases in craving for HiCal food-cues. Accumulating evidence of fMRI studies showed that DLPFC normalized the activity of reward-encoding regions when subjects choose healthy over unhealthy foods and/or regulated their food cravings (Hare et al. 2009). Few studies have examined brain FC longitudinally in obese subjects undergoing bariatric surgery. We observed sustained increased DLPFC-pgACC FC which was associated with BMI reductions at 1 month. However, with time a shift occurred, and at 6 months, increased FC was no longer associated with BMI but instead was associated with reduced craving for HiCal food-cues. These findings provide evidence that improved prefrontal FC contributes to successful weight-loss and reductions in food-cue craving, which each have a distinct temporal course post-LSG.
LSG-Induced Increases in SC of the DLPFC-ACC
The DLPFC and ACC have reciprocal projections with the striatum (Bae et al. 2006). Abnormal function in this circuit may result in altered emotional and autonomic responses to stimuli (Tekin and Cummings 2002). Weaker SC as quantified with FA in white matter tracts connecting the insula, amygdala, PFC, OFC, and striatum, and stronger SC between the amygdala and ACC revealed that white matter tract changes might contribute to the pathophysiology of obesity (Riederer et al. 2016). The current study showed that LSG increased SC of the right DLPFC-ACC both at the 1- and 6-month timepoints, suggesting that LSG induced sustained structural recovery in obese patients. Structural changes within 1 month are feasible, as a number of studies have found that short-term task training induced changes in white matter FA in as little as a few days, weeks, or a month (Antonenko et al. 2016; Debowska et al. 2016; Salminen et al. 2016; Dong et al. 2017). Furthermore, reduction in BMI showed negative associations with increased SC of the right DLPFC-ACC at PostLSG_1 and an even stronger association at PostLSG_6, which provides evidence that improved prefrontal SC contributes to long-term weight-loss post-LSG. In addition, we showed a positive association between the increases in SC of DLPFC-ACC and increases in FC of the right DLPFC-pgACC at PostLSG_1, which we interpret to suggest that within 1 month of LSG, weight-loss concomitantly alters SC and FC of DLPFC-ACC, which might facilitate each other’s changes. At PostLSG_6, the enhancement of SC was associated with greater TFEQ-cognitive control and lower ghrelin levels, replicating a finding that ghrelin continues to decrease from 3 to 6 months post-LSG (Dogan et al. 2016). Mediation analysis indicated that improvements in SC of DLPFC-ACC mediated the relationship between reduced ghrelin levels and improved cognitive control of eating behaviors after LSG and thus contributed to sustained weight-loss. Improved modulation of conditioned responses during food-cue stimulation has been associated with success in long-term dietary-control for weight-loss (Weygandt et al. 2019). Importantly, the ghrelin-SC-TFEQ-cognitive control path model here was not observed at PostLSG_1, which suggests that improved prefrontal SC and cognitive control may develop gradually Post-LSG in tandem with ghrelin reductions. These data are broadly consistent with recent work showing that, in addition to achieving sustained weight-loss and ghrelin changes at 12 months post-LSG, obese individuals had improved DLPFC activation during attempts to regulate their desire for food (Holsen et al. 2018). Another study demonstrated that at 6 months post-LSG (or RYGB), ghrelin reductions were correlated with lower ventral tegmental area responses to HiCal food-cues (Faulconbridge et al. 2016). Therefore, changes in ghrelin may be impacting both the rewarding aspect of food-cue stimulation and the top-down control of food-seeking behavior by dissociable neural mechanisms. Altogether, these findings underscore the need for long-term follow-ups of at least 6 months in studies on neurobiological changes associated with LSG. However, similar correlations were not observed for leptin and insulin, which are also key appetitive peripheral hormones. Given that leptin and insulin are anorexigenic peptides while ghrelin is an orexigenic peptide, we speculate that ghrelin and other orexigenic pathways may play a more predominant role among the endocrine mechanisms that follow LSG.
The control of eating behavior and body weight clearly involves complex orchestration of other neural circuits such as homeostatic mechanisms (hypothalamus) (Volkow et al. 2011; Farr et al. 2016; Le et al. 2020), reward (ventral tegmental area, striatum), emotion/memory (amygdala, hippocampus), and attention (visual and parietal cortices) systems (Volkow et al. 2011; Hendrick et al. 2012). In the current study, we focused on the cognitive control circuit, and we did not find significant changes in those above circuits. The null findings suggest that there may be different neural mechanisms of bariatric surgery in modulating body weight versus eating behavior.
Limitations of Study
There are some limitations that should be noted. Due to strict exclusion criteria and the difficulty in patient retention post-surgery, our LSG group sample size was limited. Associations of peripheral hormones with FC/SC were only assessed during the fasting-state, and we did not test other gut peptides that reflect postprandial changes. Furthermore, cognitive control was measured using the TFEQ questionnaire instead of a cognitive control task, such as the stop-signal task (King et al. 2018). Future studies should conduct longer post-surgery assessments to examine the trajectory of brain changes over different stages of post-LSG, including the rebound in weight commonly observed after the first two years (King et al. 2018). In addition, we measured total ghrelin, although acyl-ghrelin is the form of the peptide that stimulates appetite. While this approach is a limitation, we elected to measure total ghrelin because total ghrelin was the only option in the commercial kit available to our laboratory and also keeping in mind that total ghrelin is easier to measure. Furthermore, we measured total ghrelin to keep this study consistent with our previous work (Li et al. 2019) and we also note that it is likely that total and acyl-ghrelin are highly correlated with each other. Nonetheless, it will be helpful for future studies to measure both total ghrelin and acyl-ghrelin concentration as well as other peptides related to the ghrelin system such as LEAP-2.
Conclusions
This study examined the LSG-induced alterations in FC and SC of frontal-limbic circuits in obese patients prior to and at 1 and 6 months post-LSG. Results show that LSG significantly increased the FC of the right DLPFC with pgACC and increased the SC between DLPFC and ACC, which are regions implicated in inhibitory-control and self-regulation. SC of DLPFC-ACC mediated the relationship between ghrelin and cognitive control. Our findings provide evidence that improvements in prefrontal FC/SC were associated with long-term reductions in weight-loss and with reduced sensitivity to food-cues post-LSG.
Authors’ Contribution
Conceptualization: G.J.W., Y.Z., Y.N., and G.J.; Methodology: Y.Hu., G.Y.L., P.M., W.Z., J.W., G.G.L.,Y.He., Z.D.Z., and K.Y.; Software: Y.Hu. and G.Y.L.; Investigation: A.C., G.C., and H.W.; Writing—Original Draft: Y.Z., Y.Hu., and G.J.W.; Writing—Review and Editing: K.V., C.W., and N.V. We thank Dr Lorenzo Leggio's contribution on the revision of the manuscript.
Funding
National Natural Science Foundation of China (grant nos. 61431013, 31670828, 81730016); Open Funding Project of National Key Laboratory of Human Factors Engineering (grant no. 6142222190103); National Clinical Research Center for Digestive Diseases, Xi’an, China (grant no. 2015BAI13B07); Intramural Research Program of the National Institute on Alcoholism and Alcohol Abuse (NIAAA) in the United States (Y1AA3009 to P.M., C.E.W., N.D.V., G.J.W.).
Conflict of Interest
The authors declare no biomedical financial interests or potential conflicts of interest.
References
Author notes
Yang Hu, Gang Ji, and Guanya Li have contributed equally to this work