-
PDF
- Split View
-
Views
-
Cite
Cite
Chengcong Chen, Anbang Ren, Qi Yi, Jiazuo Cai, Muhammad Khan, Yunen Lin, Zhong Huang, Jie Lin, Jian Zhang, Wei Liu, Anan Xu, Yunhong Tian, YaWei Yuan, Ronghui Zheng, Therapeutic hyperthermia regulates complement C3 activation and suppresses tumor development through HSPA5/NFκB/CD55 pathway in nasopharyngeal carcinoma, Clinical and Experimental Immunology, Volume 213, Issue 2, August 2023, Pages 221–234, https://doi.org/10.1093/cei/uxad060
- Share Icon Share
Abstract
Nasopharyngeal carcinoma (NPC) is endemic in Southern China and Southeast Asia. Hyperthermia is widely used in combination with chemotherapy and radiotherapy to enhance therapeutic efficacy in NPC treatment, but the underlying anti-tumor mechanisms of hyperthermia remain unclear. Complement C3 has been reported to participate in the activation of immune system in the tumor microenvironment, leading to tumor growth inhibition. In this study, we aimed to explore the effect and mechanisms of hyperthermia and investigate the functional role of complement C3 in NPC hyperthermia therapy (HT). The serum levels of complement C3 before and after hyperthermia therapy in patients with NPC were analyzed. NPC cell lines SUNE1 and HONE1 were used for in vitro experiment to evaluate the function of complement C3 and HT on cell proliferation and apoptosis. SUNE1 xenograft mouse model was established and tumor-bearing mice were treated in water bath at a constant temperature of 43°C. Tumor samples were collected at different time points to verify the expression of complement C3 by immunohistochemical staining and western blot. The differential expressed genes after hyperthermia were analyzed by using RNA sequencing. We found that complement could enhance hyperthermia effect on suppressing proliferation and promoting apoptosis of tumor cells in NPC. Hyperthermia decreased the mRNA expression of complement C3 in tumor cells, but promoted the aggregation and activation circulating C3 in NPC tumor tissue. By using in vitro hyperthermia-treated NPC cell lines and SUNE1 xenograft tumor-bearing mice, we found that the expression of heat shock protein 5 (HSPA5) was significantly upregulated. Knockdown of HSPA5 abrogated the anti-tumor effect of hyperthermia. Moreover, we demonstrated that hyperthermia downregulated CD55 expression via HSPA5/NFκB (P65) signaling and activated complement cascade. Our findings suggest that therapeutic hyperthermia regulates complement C3 activation and suppresses tumor development via HSPA5/NFκB/CD55 pathway in NPC.
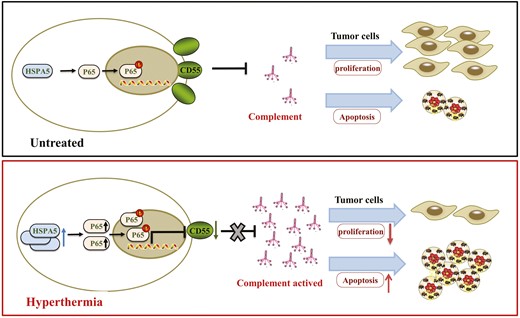
Introduction
Nasopharyngeal carcinoma (NPC) is endemic in Southern China, East and Southeast Asia [1, 2]. As NPC is sensitive to radiotherapy and chemotherapy, the prognosis has been significantly improved in the past decade [3, 4]. However, new therapeutic treatments are critically needed as the current therapies have entered a plateau phase. Hyperthermia is a therapeutic treatment of cancers via raising the tumor local temperature [5]. The combination of hyperthermia, together with chemotherapy and radiotherapy, has been demonstrated to markedly improve the therapeutic effect in multiple tumor types including NPC [6–8]. Our previous work has also indicated that whole-body hyperthermia could improve prognosis of patients with NPC who received standard chemoradiotherapy [6] However, the underlying anti-tumor mechanisms of hyperthermia remain to be fully understood.
Accumulating evidence shows that hyperthermia invokes multiple signaling pathways, regulating cell proliferation and apoptosis [9]. A study has found that hyperthermia could induce NDRG2 upregulation via suppressing ERK1/2 signaling pathway and inhibit human hepatocellular carcinoma invasion [10]. In colorectal cancer, hyperthermia enhanced ERK and WNT signaling while AKT signaling was reported to be regulated by hyperthermia in glioma and lung cancers [11, 12]. In addition, hyperthermia could enhance immune cell infiltration and regulate tumor microenvironment (TME), showing synergy effect when combined with immunotherapy [13]. Thus, it remains critical to fully understand the signaling pathways and their regulation in hyperthermia.
Complement system has been demonstrated to function as tumor promoter or suppressor in different cancers [14]. Complement has been reported to participate in the activation of immune system in the TME, leading to tumor growth inhibition [15]. In the contrast, activation of complement C3 modulated tumor-associated macrophages and resulted in resistant to immune checkpoint therapy [16]. The molecular mechanisms and the regulation of complement cascade in cancers are largely unknown. Clinical hypothermia and hyperthermia have been studied to regulate complement levels [17]. In human colon cancer, hyperthermia could enhance the antibody-complement cytotoxicity [18]. However, the function of complement in NPC and whether hyperthermia modulates complement levels in NPC have not been addressed yet.
In this study, by using both in vitro hyperthermia-treated NPC cell lines and in vivo NPC xenograft tumor model, we demonstrated that complement could enhance hyperthermia effect on suppressing proliferation and promoting apoptosis of tumor cells in NPC. Mechanistically, we found that the hyperthermia markedly enhanced the expression of heat shock protein 5 (HSPA5). Knockdown of HSPA5 abrogated the anti-tumor effect of hyperthermia. Moreover, we demonstrated that hyperthermia downregulated CD55 expression via HSPA5/NFκB (P65) signaling and activated complement cascade. Taken together, our results suggest that therapeutic hyperthermia regulates complement C3 expression and suppresses tumor development via HSPA5/NFκB/CD55 pathway in NPC.
Materials and methods
Human specimens
NPC patients were recruited from Affiliated Cancer Hospital & Institute of Guangzhou Medical University. The diagnosis of NPC was confirmed by routine pathological biopsy. Patient information was presented in Supplementary Table 1. Plasmas from patients with metastasis NPC were obtained from Affiliated Cancer Hospital & Institute of Guangzhou Medical University. The study protocol was reviewed and approved by the Ethics Committee of Guangzhou Medical University (ZN2022-13).
Cell culture
Human NPC cell line HONE1 and SUNE1 were purchased from American Type Culture Collection (USA) and cultured in Roswell Park Memorial Institute-1640 (Gibco, USA) supplemented with 10% fetal bovine serum (Gibco, USA). All these cells were incubated at 37°C with 5% CO2 in a humidified incubator.
Induction of heat treatment
The cell culture flasks or 6-well plates seeded with cells were placed in a constant temperature condition of 43°C, 5% CO2 incubator for 2 h. After the heat treatment, cells were placed back into the regular 37°C, 5% CO2 incubator to continue the culture for recovery. Cells cultured at 37°C served as the experimental control group.
Source of C3-related fragment
In this experiment, sera from patients with metastasis NPC were used as the main source of complement. Complement C3 is the most abundant complement in serum. In order to avoid the degradation of complement C3 during the process, 10% human serum (HS) was added to cell culture within 2 h after the heat treatment.
RNA extraction and quantitative reverse transcription-PCR
Total RNA from NPC cell lines was isolated with TRIzol reagent (Takara, Dalian, China) as instructed. Complementary DNA (cDNA) was synthesized using a Prime-Script™ reverse transcription reagent kit (Takara, Dalian, China). Quantitative analysis was performed using SYBR Premix Ex Taq™ kit (Takara, Dalian, China). The experiments were performed in triplicate for each sample and normalized to expression of the housekeeping gene GAPDH. The analysis was calculated by relative quantification (2−ΔΔCT). The primer sequences are shown in Supplementary Table 2.
Western blot
Total protein was extracted using RIPA buffer (Beyotime, Shanghai, China), and then quantified using Pierce BCA protein assay (ComWin Biotech, Beijing, China). Equal amounts of protein were separated by SDS–polyacrylamide gel electrophoresis and transferred to a polyvinylidene fluoride membrane (Millipore, Billerica, MD). The membranes were then blocked with 5% skim milk and incubated with anti-HSPA5/Bip (1:1000, Proteintech, Wuhan, China), anti-CD55 (1:1000, Proteintech, Wuhan, China), anti-nuclear factor kappa B p65 (Phospho-Ser536) (1:1000, Abcam, Shanghai, China), anti-NFκB p65 (1:1000, ZENBIO, Chengdu, China), or rabbit anti-GAPDH (1:1000, Proteintech, Wuhan, China) primary antibody overnight at 4°C. The species-matched secondary antibodies were then incubated at room temperature for 1 h and the proteins were detected by WesternBright™ ECL detection kit (Advasta).
Immunofluorescence staining
HONE1 or SUNE1 cells (1 × 105 cells per well in a 24-well plate) were fixed with 4% paraformaldehyde (PFA) on glass coverslips for 15 min and permeabilized with 0.5% TritonX-100. Cells were washed with phosphate buffer saline (PBS) and blocked in 3% bovine serum albumin (BSA) for 30 min. Rabbit anti-C3 primary antibody (Proteintech, Wuhan, China) was used at 1:100 dilution, and goat anti-rabbit AlexaFluor-488 secondary antibodies (Invitrogen) were used at 1:200 dilution. 2-(4-amidinophenyl)-1H-indole-6-carboxamidine (DAPI, Sigma) staining was used for co-staining analysis under a fluorescence microscope (Leica, Germany).
Flow cytometry
Cell apoptosis was analyzed by flow cytometry using an Annexin V-APC/7-AAD assay (BestBio Biotech, Shanghai, China). HONE1 and SUNE1 cells were either treated (experimental) or not treated (control) at 43°C for 2 h. Following the manufacturer’s guidelines, cells were washed twice with cold PBS, resuspended, and incubated with binding buffer containing APC/7-AAD in the dark for 10 min at room temperature. Relative fluorescence was subsequently analyzed by flow cytometry (BD FACSVerse™; BD, USA). To examine the relative expression levels of CD55 on SUNE1 cell surface, SUNE1 cells were labeled with anti-CD55 antibody (1:200, Proteintech, Wuhan, China). Goat anti-rabbit IgG (AlexaFluor-488) was used as the secondary antibody. For each experiment, 2 × 104 cells were analyzed.
Small interfering RNA transfection
Small interfering RNA (siRNA) targeting HSPA5 and the non-specific negative control siRNA were designed and synthesized by Guangzhou RiboBio Co., Ltd. Human NPC cells were seeded into a 6-well plate and cultured until they reached 60–70% density on the second day. The siRNAs were transfected using a reagent (Lipofectamine™ 3000 Transfection Reagent; Invitrogen Inc.). Downregulation of HSPA5 was confirmed by western blot and qPCR. The siRNA sequences targeting HSPA5 were listed in Supplementary Table 3.
TUNEL staining
Apoptotic cells were identified by using a DeadEnd™ Fluorometric TUNEL system (Promega, USA). Following the guidelines of the TUNEL staining method, slides were immersed in rTdT Incubation Buffer at 37°C for 1 h, then reaction was stopped and slides were washed before imaging analysis. The percentage of apoptotic cells was calculated by dividing the TUNEL-positive staining cells (including weakly positive expression cells) by the cells stained with DAPI. In addition, the ImageJ software was applied for calculating TUNEL-positive and DPAI-positive cells.
EdU staining
Cell proliferation was evaluated by using a BeyoClick™ EdU-555 Assay (Beyotime, China) following the manufacturer’s instructions.
RNA-sequencing analysis
Total RNA was extracted from heated/non-heated SUNE1 cells, followed by mRNA enrichment and purification, Oligo dT Selection to enrich the mRNA (for total RNA extracted from human whole blood, globin mRNA are depleted); RNA fragmentation and cDNA synthesis (second-strand cDNA synthesis with dUTP instead of dTTP); PCR and circularization to make DNB. Sequencing on DNBSEQ platform was conducted by BGI Group (Guangdong, China). The filtering software SOAPnuke developed by BGI independently was used for filtering. Quality control (QC) was then performed on the raw reads to determine whether the sequencing data was suitable for subsequent analysis. After QC, the filtered clean reads were aligned to the reference genomic sequences. After the alignment, the statistics of the mapping rate and the distribution of reads on the reference sequences were used to determine whether the alignment result passes the second QC of alignment. Gene quantification analysis and other analysis based on gene expression (principal component, correlation, differential gene screening, etc.) were conducted. The gene set enrichment analysis (GSEA) was performed by using the GSVA package in R. The parameters used in the GSEA are as follows: the seed is 2020, the number of calculations is 1000, the number of genes contained in each gene set is at least 15, and the number of genes contained at most is 500. The P-value correction method is the Benjamini–Hochberg method. The data set (GSE61218, GSE75127) was obtained from the publicly available GEO databases (https://www.ncbi.nlm.nih.gov/geo/). The data of The Cancer Genome Atlas were downloaded from the UCSC Cancer Genomics Browser (https://xena.ucsc.edu/public).
Tumor xenograft model in vivo
BALB/c nude mice (4-week old) were purchased from Guangdong Medical laboratory animal center (Guangdong, China). SUNE1 cells (3 × 106) were resuspended in 150 μL serum-free medium and injected into the mice subcutaneously. After 5 weeks, the mice were randomly assigned into two groups (n = 10 per group) to receive the following treatments: control group received no heat treatment; the experimental group mice were fixed on the plate, and then the local tumors of the mice in the lower limbs were soaked in a bath box at a constant temperature of 43°C for 30 min [19–22]. After euthanasia, tumor tissues were fixed to analyze the extent of complement C3 deposition by 4% PFA. The tissues were paraffin-embedded, and serial 5-μm tissue sections were prepared for subsequent experimental processing. All animal studies were conducted in accordance with the regulations approved by the Animal Care and Use Ethnic Committee of Affiliated Cancer Hospital and Institute of Guangzhou Medical University.
Immunohistochemical staining
Immunohistochemical (IHC) staining was performed using tumor tissue sections with a Histostain-Plus Kit (Zhongshan Biotechnologies, Beijing, China) according to the manufacturer’s instructions. After staining, tumor sections were photographed, and the intensity of C3 staining was captured and deposited by the side scanner Axio Scan Z1 (Zeiss). The scan of the slices was fully automated. The following primary antibodies were used: C3 (1:100 dilution, Proteintech, Wuhan, China).
Statistical analysis
Statistical analysis was performed using GraphPad Prism 7.0 (GraphPad Software, Inc., La Jolla, CA, USA). Images were edited using Adobe Photoshop CC2019 (Microsoft Corp., Redmond, WA, USA). Each experiment was repeated at least in triplicate, and the mean ± standard deviation was presented. The Z-score was used for data normalization. Student’s t test was used to compare the statistically significant differences between two groups. Multiple comparisons were performed by using ANOVA. P < 0.05 was considered statistically significant.
Results
Complements enhance hyperthermia effect on suppressing proliferation and promoting apoptosis in NPC cells
To investigate the function of complement in anti-tumor effect of hyperthermia, we treated two NPC cell lines (SUNE1 and HONE1) with control or hyperthermia heat therapy (HT), with or without adding HS or inactivated human serum (IHS). We found that in both cell lines, in comparison with control, hyperthermia significantly suppressed cell proliferation. Administration of HS-containing complements further inhibited cell proliferation while adding IHS with inactivated complements in the cell culture had no effect on cell proliferation as demonstrated by EDU incorporation assay (Fig. 1). In contrast, hyperthermia promoted SUNE1 and HONE1 cell apoptosis. HS further enhanced cell apoptosis while IHS did not change apoptosis levels in NPC cell lines (Fig. 2). Thus, the complements in the serum might contribute to the regulation of NPC cell proliferation and apoptosis.
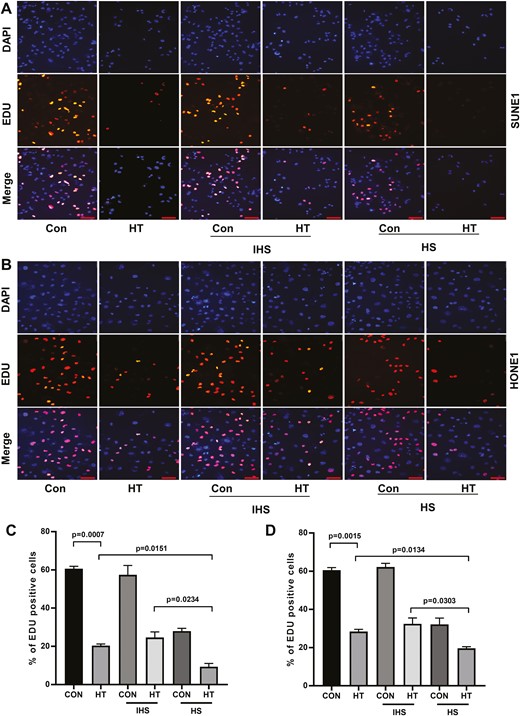
Complements enhance hyperthermia effect on suppressing NPC cell proliferation. (A) SUNE1 and (B) HONE1 cells were cultured at 37°C (con) or 43°C (hyperthermia heat therapy, HT) with 5% CO2 in a humidified incubator for 2 h. Then cells were continued to culture under 37°C with 5% CO2 in a humidified incubator, with additional 10% HS or IHS from NPC patients for 24 h. Cell proliferation of (C) SUNE1 and (D) HONE1 cells was evaluated by EDU incorporation assay. Scare bar 50 μm.
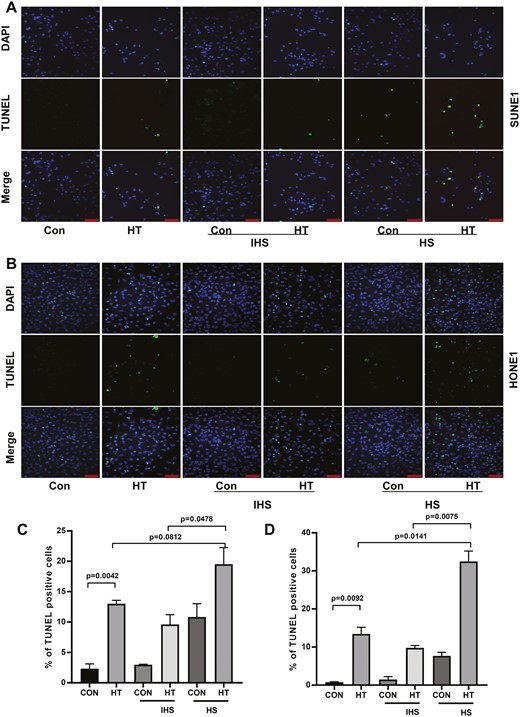
Complements enhance hyperthermia effect on promoting NPC cell apoptosis. (A) SUNE1 and (B) HONE1 cells were cultured at 37°C (con) or 43°C (hyperthermia heat therapy, HT) with 5% CO2 in a humidified incubator for 2 h. Then cells were continued to culture under 37°C with 5% CO2 in a humidified incubator, with additional 10% HS or IHS from NPC patients for 24 h. Cell apoptosis of (C) SUNE1 and (D) HONE1 cells was evaluated by TUNEL staining assay. Scare bar 50 μm.
Hyperthermia decreases the mRNA expression of complement C3 but promotes expression of circulating C3 in NPC tumor
Complement C3 plays a central role in the complement system, which is essential for activation of the classic, alternative, and mannose-binding lectin pathways [23]. We first evaluated the expression of C3 after heat therapy. As shown in Fig. 3A, 3 or 12 h post-heat therapy, C3 mRNA expression levels were markedly decreased compared with that in control tumor cells. The downregulation of C3 mRNA after heat therapy was further confirmed in SUNE1 and HONE1 cells (Fig. 3B). Intriguingly, when heat therapy-pretreated SUNE1 or HONE1 cells were cultured with HS from NPC patient for 24 h, we found that serum complements were absorbed and activated in NPC tumor cells, generating C3a, iC3b, and C3c sequential digestion (Fig. 3C). Sera from patients with metastasis NPC pre- or 1-week post-hyperthermia were collected and analyzed the complement levels. Hyperthermia significantly inhibited C3 levels in patient serum while no significant changes were found for C4 levels on Day 7 after hyperthermia (Fig. 3D). In addition, we established the SUNE1 xenograft tumor model in nude mice and tumor-bearing mice were conducted heat therapy with 43°C water bath for 30 min. Blood samples and SUNE1 tumor samples were collected at different time points after heat therapy and analyzed for complement levels. The results showed significantly upregulation of C3b levels both in blood and tumor tissues (Fig. 3E and F). The enhanced C3 expression was also confirmed by IHC staining and western blot using SUNE1 tumor tissues (Fig. 3G and H). These findings suggest that hyperthermia decreases the mRNA expression of complement C3 in tumor cells, but enhances the expression and activation of the C3 complement cascade in NPC tumor tissue.
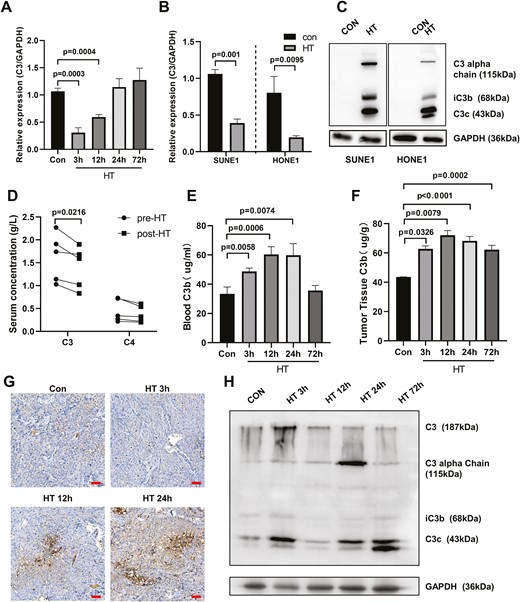
Hyperthermia decreases the mRNA expression of complement C3 but promotes enrichment of circulating C3 in NPC tumor. (A) SUNE1 cells were cultured at 37°C (con) or 43°C (hyperthermia heat therapy, HT) with 5% CO2 in a humidified incubator for 2 h. Then cells were continued to culture under 37°C with 5% CO2 in a humidified incubator for different time. The C3 mRNA levels were examined at different time points post-hyperthermia by qPCR. (B) SUNE1 and HONE1 cells were cultured at 37°C (con) or 43°C (HT) with 5% CO2 in a humidified incubator for 2 h and then cells were continued to culture under 37°C with 5% CO2 in a humidified incubator for 3 h. The C3 mRNA levels were examined in SUNE1 and HONE1 cells post-hyperthermia by qPCR. (C) Heat therapy-treated SUNE1 or HONE1 cells were cultured with sera from NPC patients for 24 h. Western blot was performed to examine the C3 complement and its sequential fragments in SUNE1 or HONE1 cells. GAPDH was used as an internal loading control. (D) Serum concentration of complement C3 and C4 in NPC patient pre- or 1-week post-hyperthermia was analyzed by ELISA assay. (E–H) SUNE1 cells were inoculated into nude mice to establish the NPC xenograft tumor model. SUNE1 tumor-bearing mice underwent heat therapy with 43°C water bath for 30 min and blood/tumor samples were collected at different time points post-HT for analysis. (E) Blood and (F) tumor C3b levels were analyzed by ELISA. (G) Tumor C3 levels were analyzed by IHC staining. Scare bar 50 μm. (H) The protein expression levels of C3, C3a, iC3b, and C3c in tumors were analyzed by western blot. GAPDH was used as an internal loading control. *P < 0.05, **P < 0.01, ***P < 0.001.
Public database analysis of complement expression in NPC
We performed bioinformatics analysis of the complement expression in NPC using public database. As shown in Fig. 4A, heatmap analysis of GSE61218 containing mRNA and lncRNA gene expression profile of NPC showed that there was no significant difference of C3 expression between NPC tumor tissues and normal healthy nasopharyngeal tissues. We also performed IHC analysis of C3 in tumor samples from NPC patients and detected low expression levels of C3 in tumor specimen (Fig. 4B). Taken together, the expression level of C3 was low and comparable between tumor tissues and normal tissues in NPC.
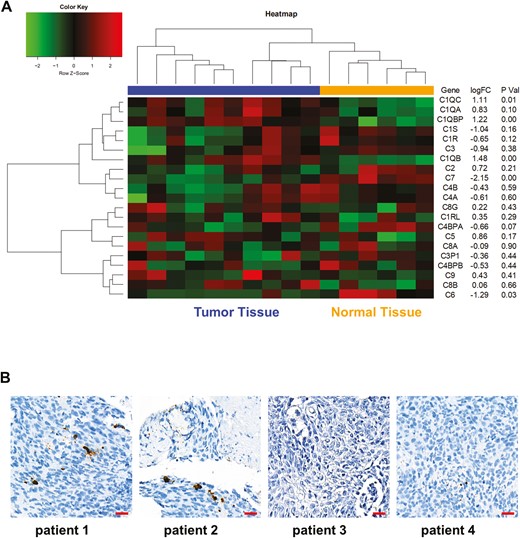
Public database analysis of complement expression in NPC. (A) Bioinformatics analysis was performed using the GSE61218 data set and heatmap showed the complement gene expression in NPC tumor tissues and normal tissues. (B) C3 IHC staining was performed using tumor specimen from NPC patients. Scare bar 20 μm. *P < 0.05.
Hyperthermia upregulates the expression of heat shock proteins
We performed bioinformatics analysis of differentially expressed genes in human oral squamous cell carcinoma cells (GSE75127) and found that the heat shock proteins (Hsps) were significantly upregulated in hyperthermia-treated tumors cell line (Fig. 5A). Consistently, in NPC cell line, hyperthermia-treated SUNE1 cells showed markedly enhanced expression of Hsps (Fig. 5B). In addition, RELA expression was upregulated in hyperthermia-treated SUNE1 cells while C3 expression was decreased (Fig. 5B). We confirmed that in both SUNE1 and HONE1 cells, hyperthermia could remarkably upregulate the expression of Hsps including HSPA1A, HSPA1L, HSPA5, and HSPA8 (Fig. 5C). Moreover, we also investigated whether hyperthermia increased Hsps expression in vivo. SUNE1 xenograft tumor-bearing mice were conducted heat therapy with 43°C water bath. SUNE1 tumor samples were collected at different time points after heat therapy and analyzed. The results showed significantly upregulation of mRNA levels of HSPA1A, HSPA1L, HSPA5, and HSPA8 (Fig. 5D). Taken together, these findings suggest that hyperthermia could upregulate the expression of Hsps in tumor cells.
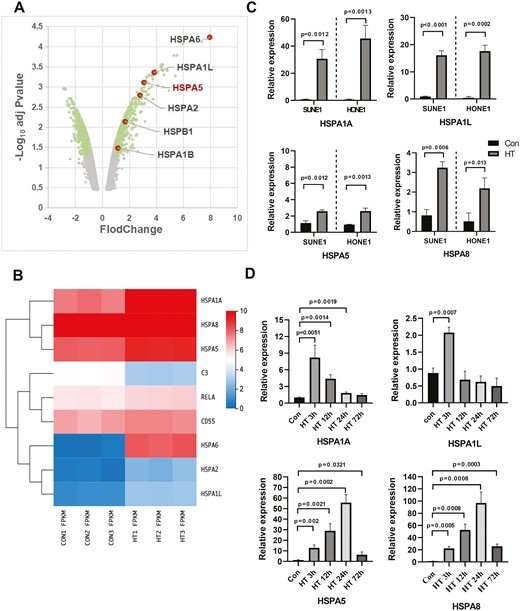
Hyperthermia upregulates the expression of Hsps. (A) A volcano plot showed the differentially expressed genes in tumors with or without heat therapy using the data set GSE75127. Hsps were significantly upregulated. (B) SUNE1 cells were cultured at 37°C (con) or 43°C (hyperthermia heat therapy, HT) with 5% CO2 in a humidified incubator for 2 h. Cells were collected for microarray analysis (PRJNA851792) and heatmap showed the expression levels of Hsps, RELA, and C3. (C) SUNE1 and HONE1 cells were cultured at 37°C (con) or 43°C (hyperthermia heat therapy, HT) with 5% CO2 in a humidified incubator for 3 h. The relative mRNA expression levels of HSPA1A, HSPA1L, HSPA5, and HSPA8 were analyzed by reverse transcription-qPCR. (D) SUNE1 cells were inoculated into nude mice to establish the NPC xenograft tumor model. SUNE1 tumor-bearing mice underwent heat therapy with 43°C water bath and tumor samples were collected at different time points post-HT for mRNA analysis of HSPA1A, HSPA1L, HSPA5, and HSPA8. *P < 0.05, **P < 0.01, ***P < 0.001.
Knockdown HSPA5 abrogates the anti-tumor effect of hyperthermia
The function of HSPA5 has been studied in multiple cancers [24]. To investigate whether HSPA5 plays an essential role mediating the anti-tumor effect of hyperthermia, we used siRNA targeting HSPA5 to silence the expression of HSPA5 in HONE1 cells (Fig. 6A and B). We found that knockdown of HSPA5 significantly dampened the absorption of C3 in HONE1 cells when cultured with HS (Fig. 6C). In addition, knockdown of HSPA5 markedly suppressed the apoptosis of hyperthermia-treated HONE1 cells cultured with HS (Fig. 6D). To understand the anti-tumor mechanism of HSPA5 in hyperthermia, a C3-targeted complement inhibitor compstatin was used to treat HONE1 cells. The EDU assay and TUNEL assay demonstrated that inhibition of C3 complement by compstatin led to enhanced cell proliferation and suppressed cell apoptosis (Fig. 6E and F). Similar results were also found in SUNE1 cells, with HSPA5 knockdown abrogated the anti-tumor effect of hyperthermia, probably mediated by complement C3 activation (Supplementary Fig. 1).
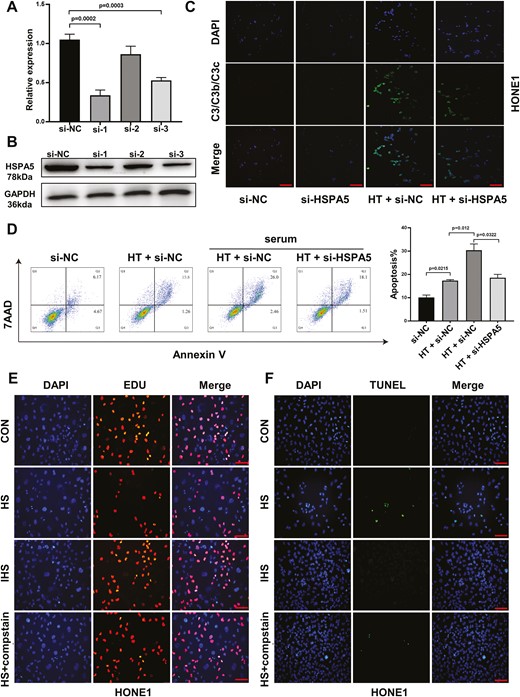
Knockdown HSPA5 abrogates the anti-tumor effect of hyperthermia in HONE1 cells. HONE1 cells were transfected with negative control (si-NC), or siRNAs targeting HSPA5. (A) The relative mRNA expression and (B) protein expression of HSPA5 in HONE1 cells were analyzed by reverse transcription-qPCR or western blot 48 h later. (C) HONE1 cells transfected with si-NC or si-HSPA5 were cultured at 37°C or 43°C hyperthermia. HS was added as a resource of complements. After culture for 24 h, the absorption of complement in HONE1 cells was analyzed by immunofluorescence staining of C3/C3b/C3c and DAPI. (D) HONE1 cells transfected with si-NC or si-HSPA5 were cultured at 37°C or 43°C HT for 48 h, with or without HS. The cell apoptosis was analyzed by Annexin V/7-AAD staining. (E, F) HONE1 cells were cultured with HS, IHS, or HS + C3 inhibitor compstatin for 48 h. The cell proliferation (E) and apoptosis (F) of HONE1 cells were evaluated by EDU staining and TUNEL staining assays. *P < 0.05, **P < 0.01, ***P < 0.001. Scare bar 50 μm.
Hyperthermia downregulates CD55 expression via HSPA5/p65 signaling to activate complement cascade
It has been reported that CD55 could suppress complement activation and inhibit complement-mediated tumor cell lysis, result in tumor progression [25, 26]. Intriguingly, we found that hyperthermia could downregulate the expression of CD55 in HONE1 cells, as demonstrated by cell surface CD55 staining and flow cytometry analysis (Fig. 7A). Knockdown of HSPA5 could elevate the expression of CD55 in HONE1 cells (Fig. 7B). GSEA revealed that hyperthermia could enrich the gene sets involving in NFκB/p65 signaling (Fig. 7C and D). Consistently, by using western blot, we demonstrated that HT downregulated the expression of CD55, while enhanced the expression of HSPA5 and phosphorylation of p65 (Fig. 7E). Knockdown of HSPA5 could antagonize the effect of hyperthermia on p65 activation and CD55 downregulation (Fig. 7E). Moreover, by using p65 inhibitor BAY-11-7821, we found that inhibition of p65 signaling could block the downregulation of CD55 mediated by hyperthermia. Together, these results indicate that hyperthermia inhibits CD55 expression and activates complement cascade to inhibit tumor progression via HSPA5/p65 signaling.
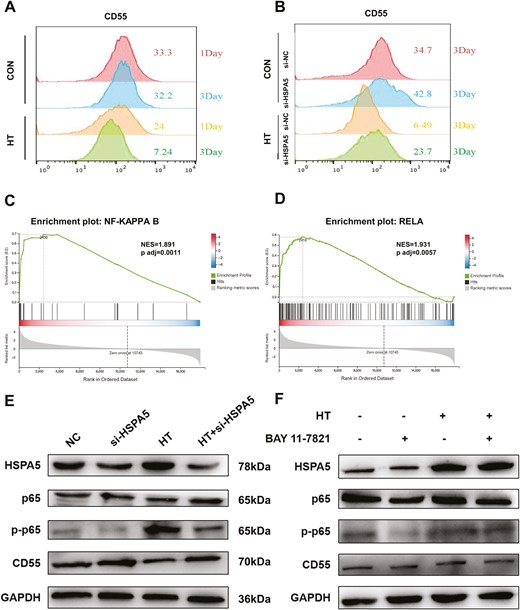
Hyperthermia downregulates CD55 expression via HSPA5/p65 signaling to activate complement cascade. (A) HONE1 cells were cultured at 37°C or 43°C HT for 1 day or 3 days. The surface expression of CD55 was analyzed by flow cytometry in HONE1 cells. (B) SUNE1 cells transfected with si-NC or si-HSPA5 were cultured at 37°C or 43°C HT for 1 day or 3 days. The surface expression of CD55 was analyzed by flow cytometry in HONE1 cells. (C, D) GSEA was performed using RNA-seq data of HONE1 cells treated with or without HT to identify the gene set enrichment. (E) HONE1 cells were cultured at 37°C or 43°C HT for 1 day, with or without HSPA5 knockdown. The protein expression of HSPA5, p65, phosphorylation of p65 (p-p65), CD55, and GAPDH was analyzed by western blot. (F) HONE1 cells were cultured at 37°C or 43°C HT for 1 day, with or without p65 inhibitor BAY-11-7821 treatment. The protein expression of HSPA5, p65, phosphorylation of p65 (p-p65), CD55, and GAPDH was analyzed by western blot.
Discussion
The anti-cancer effect of hyperthermia has been extensively studied for decades. Specifically, the cytotoxic effect of hyperthermia has been demonstrated in NPC in 1989 [27]. Hyperthermia treatment has been investigated to treat NPC in combination with chemoradiotherapy to achieve better efficacy [28–30]. A recent study demonstrated that hyperthermia could suppress NPC growth via degradation of c-Myc [31]. However, the underlying anti-cancer mechanism of hyperthermia in NPC is not fully understood. In the current study, we demonstrated that hyperthermia led to enrichment of circulating complement C3 in NPC, while complement enhanced hyperthermia effect on suppressing proliferation and promoting apoptosis in NPC. Moreover, our results suggest that hyperthermia downregulates CD55 expression via HSPA5/NFκB signaling to activate complement cascade, which provides new insights about the anti-cancer mechanisms of hyperthermia in NPC.
Complement has been suggested to play a vital role in tumor development and progression via regulating both the innate and adaptive immune response in TME [14, 15]. In NPC, alpha-2 macroglobulin and complement factor B could be used as complementary biomarkers [32]. Here we analyzed the complement expression in NPC using public database and found that there was no significant difference of C3 expression between NPC tumor tissues and normal healthy nasopharyngeal tissues. However, it is well known that the main source of complement comes from the liver [33]. Early studies have found that complement was produced in the liver and circulates to the whole body through the blood system, which was originally defined as a liver-derived, blood-circulating sentinel system [34]. The role of complement in cancer was extraordinarily complex and the current understanding was still far from complete. Studies showed that complement was not efficient in immune surveillance against cancer cells and the data supporting an anti-tumor role for complement activation were scant [35]. Consistently, we found that the expression of complement C3 in NPC was very low before hyperthermia. However, we noted an interesting phenomenon that complement expression increased in NPC tissue after hyperthermia, so we explored the molecular mechanisms in it. Through our research, we found that the expression of complement C3 increased in NPC tumor tissue after hyperthermia therapy. Result in Fig. 3D showed the decrease in the expression of complement C3 in serum of NPC patients on Day 7 after hyperthermia compared to serum C3 expression before hyperthermia. We hypothesized that serum complement chemotaxis to the tumor tissue after hyperthermia, resulting in decreased serum expression. In animal studies, we found that serum complement C3b increased within 24 h and reached its peak, and decreased to the basic level at 72 h (Fig. 3E). Therefore, we believe that the complements in serum rised first and then falled after hyperthermia. We thought that peripheral blood-circulating complement derived from liver could be activated in the TME of NPC. Consistent with this idea, our results revealed that when hyperthermia-pretreated NPC cells were cultured with HS from NPC patient, serum complements were absorbed and activated in NPC tumor cells, generating C3a, iC3b, and C3c sequential digestion. Further, we discovered that hyperthermia increased the expression of circulating blood and tissue complement C3 in SUNE1 xenograft mouse model. These findings suggest that circulating complements play essential functions in hyperthermia-mediated anti-cancer effect.
HSPA5 is a member of the molecular chaperones expressed in the endoplasmic reticulum, which could promote cell survival under endoplasmic reticulum stress [36]. Inhibition of HSPA5 via de-acetylation and degradation suppresses breast cancer development and metastasis [37, 38]. In addition, HSPA5 was reported to repress ferroptosis and promote colorectal cancer development via enhancing GPX4 stability [39]. In contrast, we found that hyperthermia enhanced the expression of HSPA5 while suppression HSPA5 antagonized the anti-cancer effect of hyperthermia. Previous study showed that HSPA5 acetylation modulated ATF4–DDIT4–mTORC1 signaling in human lung cancer [40]. HSPA5 was also reported to regulate estrogen and retinoic acid signaling [41, 42]. Here we found that HSPA5 was involved in NFκB/p65 signaling and downregulated CD55 expression. CD55 could inhibit early complement activation via accelerating the degradation of C3 convertase [43]. Thus, hyperthermia enhanced HSPA5/NFκB signaling and downregulated CD55 to activate complement cascade in NPC.
There are several limitations in our study. First, we studied the mechanisms of hyperthermia in NPC while in clinical practice, hyperthermia is usually combined with chemoradiotherapy. Second, how the immune environment has been regulated by hyperthermia and HSPA5/NFκB signaling in NPC is not addressed. Last, we investigated the hyperthermia effect using SUNE1 tumor-bearing nude mice, which are immune-deficient. Additional animal model studies are required to fully understand the immune response to hyperthermia.
Conclusion
Our research explored the anti-tumor mechanism of hyperthermia for NPC. Firstly, the complement was involved in the anti-tumor effect of hyperthermia in vitro. Further, we found that the hyperthermia markedly increased the expression of HSPA5 and decreased the expression of CD55 which regulated the activation of complement C3 and complement cascade. Finally, we demonstrated that activation of HSPA5/NFκB (P65) signaling pathway resulted in a decrease in CD55 expression (Fig. 8).
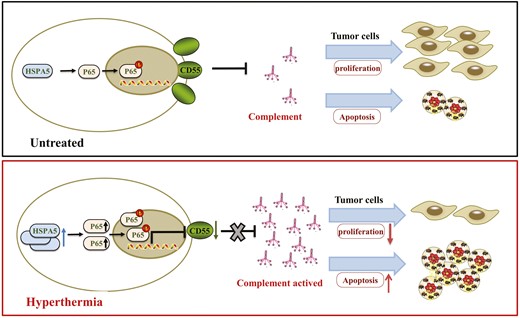
In a word, our findings suggest that therapeutic hyperthermia regulates complement C3 activation and suppresses tumor development via HPSA5/NFκB/CD55 pathway in NPC. These results might provide new therapeutic treatment for NPC patients.
Abbreviations
- cDNA
complementary DNA
- DAPI
2-(4-amidinophenyl)-1H-indole-6-carboxamidine
- GSEA
gene set enrichment analysis
- HS
human serum
- HSPA5
heat shock protein 5
- Hsps
heat shock proteins
- HT
heat treatment
- IHC
immunohistochemical
- IHS
inactivated human serum
- NPC
nasopharyngeal carcinoma
- PFA
paraformaldehyde
- QC
quality control
- siRNA
small interfering RNA
- TME
tumor microenvironment
Acknowledgements
We sincerely thank the staff at The Cancer Genome Atlas (TCGA) and Gene Expression Omnibus (CGGA) for sharing the data. We also appreciate the hard work of the R development core team and the R community (http://www.r-project.org).
Ethical approval
The study protocol followed the ethical guidelines of the 1975 Declaration of Helsinki and was approved by the Ethical Committee and Institutional Review Boards of Affiliated Cancer Hospital & Institute of Guangzhou Medical University (ZN2022-13). Informed consents were signed by all patients.
Conflict of interest
The authors declare no conflict of interest.
Funding
This work was supported by the Guangzhou Science and Technology Plan Project (202102020034), the National Natural Science Foundation of China (82102971), Guangdong Basic and Applied Basic Research Foundation, China (2020A1515111074), and Plan on enhancing scientific research in GMU.
Data availability
Data are available within the manuscript.
Author contributions
A.R. was mainly responsible for basic experiments: Western Blot (WB), fluorescence, animal-related experiments, and so on. Q.Y. repeated the reliability of the results (such as WB). J.C. was responsible for bioinformatics analysis. C.C., A.R., and R.Z. designed the study. A.R., Q.Y., J.C., and C.C. performed the experiments and analyses. C.C., Q.Y., and J.C. wrote the manuscript. Y.Y. and R.Z. supervised the study. Z.H., J.L., J.Z., W.L., A.X., Y.T., Q.Y., and J.C. enrolled patients, collected samples and laboratory data, Y.L. provided technical and material support. M.K., Y.Y., and R.Z. critically reviewed the manuscript.
Permission to reproduce
Not applicable.
References
Author notes
Chengcong Chen, Anbang Ren, Qi Yi and Jiazuo Cai. Authors share co-first authorship.