-
PDF
- Split View
-
Views
-
Cite
Cite
Jean-Baptiste Michel, José-Luis Martin-Ventura, Jesus Egido, Natzi Sakalihasan, Vladislav Treska, Jes Lindholt, Eric Allaire, Unnur Thorsteinsdottir, Gillian Cockerill, Jesper Swedenborg, For the FAD EU consortium, Novel aspects of the pathogenesis of aneurysms of the abdominal aorta in humans, Cardiovascular Research, Volume 90, Issue 1, 1 April 2011, Pages 18–27, https://doi.org/10.1093/cvr/cvq337
- Share Icon Share
Abstract
Aneurysm of the abdominal aorta (AAA) is a particular, specifically localized form of atherothrombosis, providing a unique human model of this disease. The pathogenesis of AAA is characterized by a breakdown of the extracellular matrix due to an excessive proteolytic activity, leading to potential arterial wall rupture. The roles of matrix metalloproteinases and plasmin generation in progression of AAA have been demonstrated both in animal models and in clinical studies. In the present review, we highlight recent studies addressing the role of the haemoglobin-rich, intraluminal thrombus and the adventitial response in the development of human AAA. The intraluminal thrombus exerts its pathogenic effect through platelet activation, fibrin formation, binding of plasminogen and its activators, and trapping of erythrocytes and neutrophils, leading to oxidative and proteolytic injury of the arterial wall. These events occur mainly at the intraluminal thrombus–circulating blood interface, and pathological mediators are conveyed outwards, where they promote matrix degradation of the arterial wall. In response, neo-angiogenesis, phagocytosis by mononuclear cells, and a shift from innate to adaptive immunity in the adventitia are observed. Abdominal aortic aneurysm thus represents an accessible spatiotemporal model of human atherothrombotic progression towards clinical events, the study of which should allow further understanding of its pathogenesis and the translation of pathogenic biological activities into diagnostic and therapeutic applications.
1. Introduction
The initial definition of aneurysms was morphological, as a focal loss of parallelism of the vascular wall, leading to progressive dilatation and finally to rupture.1 This definition can be now challenged by the following pathophysiological definition: the progressive loss in the capacity to resist high intraluminal pressure, related to the degradation of the arterial wall. Since aneurysms of the abdominal aorta (AAAs) are usually asymptomatic, the present clinical challenge is to diagnose them at an early stage, and to decipher the biological mechanisms responsible for the progressive dilatation and final rupture, in order to develop new diagnostic and therapeutic approaches.
2. Epidemiology
Aneurysms of the abdominal aorta develop mainly in men over 65 years old, in whom the frequency reaches 1–5%. Progression of AAAs towards rupture is not linear, but usually presents points of acceleration which can appear at any time.2,3 Conversely, aortic dilatations can remain stable and asymptomatic for many years, during which aged patients may die of other causes.4 In women, AAAs are rarer, but represent a higher relative mortality than men.5 When comparing men and women with AAA, it was found, using gender-specific criteria for normal aortic diameters, that women had a significantly higher risk of associated thoracic aneurysms.6
3. Impact of screening programmes
Abdominal aortic aneurysm includes an asymptomatic phase with a relatively low risk, which is amenable to screening. Ultrasonographic screening is a valid, suitable, and acceptable method, which is sensitive (98%) and specific (99%). Persons at the highest risk of AAA attend screening more frequently than persons at low risk,7 and repeated screening is only required in 5% of the initially negative cases.8
Evidence-based large-scale randomized trials have identified 5.5 cm diameter as the cut-off point for repairing asymptomatic AAA,9 and survivors enjoy the same quality of life as the general population of the same age. Only about 2–5% of patients refuse an offer of surgery.
The benefits of screening must outweigh the costs. All four existing randomized trials report benefit of screening of men aged 65 years and older, and the pooled mid- and long-term relative risk reduction is around 50%.10 Cost effectiveness has proven attractive,11 and recently the Viborg Study reported that the number required to be screened to save one life was only 135, and the frequency of emergency operations due to rupture was reduced by 56%.12 In all, offering men aged 65–74 years screening for AAA seems acceptable according to criteria from the European Council, but nationwide implementation in Europe is only ongoing in the UK.12
4. Environmental risk factors
Abdominal aortic aneurysm is a particular, specifically localized form of atherothrombotic disease, sharing the usual risk factors with occlusive atherothrombosis, as follows: male sex, ageing, possible genetic susceptibility and dyslipidaemia. Ageing influences AAA development through the observed progressive physiological enlargement of the aorta and the increase in pulse wave reflection linked to aortic rigidification. Peripheral arterial diameter is generally larger in AAA patients compared with age- and sex-matched control subjects.13 Smoking is the major risk factor in AAA, probably linked to its ability to oxidize α1-antitrypsin. Therefore, plasma nicotine could be used for monitoring smoking in AAA patients.14 Among lipid markers, low high-density lipoprotein level is the most sensitive predictor of AAA.15 This could be related to the impact of hypercholesterolaemia on the initial step of atheroma in the aorta, and to the low levels of α1-antitrypsin conveyed by high-density lipoprotein in human AAA.16 In contrast to occlusive atheroma, diabetes is not a risk factor, probably due to glycation of extracellular matrix molecules, which increases their resistance to proteolysis.17 The presence of AAA in a patient is a marker of atherothrombotic disease elsewhere,18 and aortic diameter a predictor of total and cardiovascular mortality.19
4.1 Genetic susceptibility
Genetic determinants of susceptibility to AAA have been approached through investigation of familial aggregation of the disease, and more recently by genomewide association studies in populations. The frequency of AAA in first-order relatives was 15–19%, whereas it was only 1–3% in unrelated patients. Numerous associations between AAA growth and polymorphisms in different target genes have been explored (see20 for review). In a nationwide register study, the relative risk of developing an AAA was approximately doubled for individuals with a first-degree relative with AAA.6 A subsequent study based on the Swedish twin register showed an increased risk for a dizygotic twin when the other one had an AAA compared with the previous nationwide study, and the odds ratio was 10-fold higher for a monozygotic twin of an AAA patient compared with a dizygotic twin.21 Moreover, the monozygotic twin of an AAA patient had a risk of developing an AAA that was 71 times higher than the monozygotic twin of an unaffected person.21
Recently, using genomewide association studies, a common sequence variant, first described to be strongly associated with coronary artery disease, was reported to be associated with human AAA and intracranial aneurysms in several populations.22 This variant, rs10757278, resides at a locus on chromosome 9p21 adjacent to the genes CDKN2A/CDKN2B, which are important regulators of cell growth and survival. An additional common sequence variant associating with AAA, situated on chromosome 9q33, has since been reported and is also associated with myocardial infarction, peripheral arterial disease, and pulmonary embolism, but not with intracranial aneurysms. This AAA risk variant was independent of smoking, lipid levels, obesity, diabetes, and hypertension, and is located within the DAB2IP gene that encodes an inhibitor of cell growth and survival. This is the second common variant that consistently associates with AAA in multiple populations.23
5. Pathophysiology of AAA, a spatiotemporal model of proteolytic atherothrombosis
Aneurysms of the abdominal aorta are a particular form of atherothrombosis, in which the pathogenic role of proteolysis is predominant.1 The main role of proteases, including matrix metalloproteinases (MMPs) and serine proteases, in the evolution of AAA was first put forward 30 years ago in human studies.24 Compared with the occlusive forms of atherothrombosis, involving necrotic core formation in the intima, AAA is characterized by its localization in the matrix-rich aortic media, the presence of a chronic intraluminal thrombus (ILT), and the association with a significant adventitial reaction.25
5.1 Convection and spatial organization
Physiological transport in the aortic wall occurs mainly by orthogonal hydraulic conductance from the lumen to the adventitia through the arterial wall. This centrifugal convection is dependent on blood pressure, low shear rate, and the filtration properties of the arterial wall.26 Such convection is probably greatly enhanced at the site of AAA due to the high porosity of the ILT,27 the lack of endothelium,28 medial elastic fibre degradation,29 low shear due to recirculation in vortices,30 and high amplitude of pulsatility due to dilatation.31 Moreover, the ILT does not decrease wall stress in AAA.32 The pathogenic mediators, generated at the blood–ILT interface, are thus centrifugally conveyed towards the media and the adventitia in AAA. The structure of AAAs is usually spatially organized, from inside to out, with a multilayered, haemoglobin-rich ILT, a thin degraded media in which the elastic components have more or less disappeared, and an inflammatory and/or fibrotic adventitia. Due to the presence of the ILT, there is no endothelium or intima in AAAs.
5.2 Haemoglobin-rich ILT
The ILT is a biologically active neo-tissue described as a laminated structure, containing several layers of fibrin clot, underlying a fresh, red haematic luminal layer containing undegraded cross-linked fibrin, and an actively fibrinolysed abluminal layer (Figure 1). This observation supports the hypothesis of a dynamic biological equilibrium between clotting at the luminal interface with circulating blood and outward progressive lysis, providing evidence of a spatial topology of temporal events (clotting and lysis). The ILT is traversed from the luminal to the abluminal surface by a continuous network of canaliculi, allowing unrestricted macromolecular penetration.27
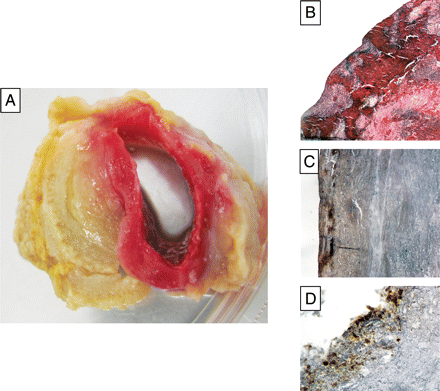
Biological features of the intraluminal thrombus (ILT). (A) Macroscopic view showing the red, most recent, luminal layer of the ILT and the abluminal proteolysed layer. (B) Microscopic aspect of the luminal layer including trapped red blood cells and a large area of nucleated, cell-rich fibrin (haematoxylin and eosin, ×4). (C) Immunostaining of platelet glycoprotein Ib on the luminal ILT surface (×4). (D) Immunostaining of neutrophils (CD66b) on the luminal surface (×4).
The luminal layer is highly biologically active, characterized by red blood cell (RBC) haemagglutination, a process that releases free haemoglobin, and fibrin formation secondary to platelet and thrombin activation. It is also responsible for tissue-plasminogen activator and plasminogen retention33 involved in the postponed progressive fibrinolysis,34 and lastly for leucocyte retention, in which neutrophils predominate. The intermediate and abluminal layers are less rich in blood-borne components.
There is now evidence that ILTs participate in aneurysmal dilatation, regardless of localization, i.e. abdominal35 and thoracic aorta36 and intracranial aneurysms.37 The presence of an ILT has been shown to be associated with a thinner arterial wall,38 more extensive elastolysis, a lower density of smooth muscle cells in the media, and a higher level of immuno-inflammation in the adventitia,39 suggesting that an important part of the protease activity originates from the ILT, rather than being directly generated within the AAA wall.
The biological activities generated by the blood interface first involve platelet activation, which releases microparticles and exposes phospholipids.40 Haemagglutination of RBCs is also an important activity associated with the blood interface, and RBCs are usually rapidly degraded in the subjacent layer, releasing free haemoglobin, which is a powerful pro-oxidant mediator due to its haem/iron component (see41 for review). In parallel, the globin component undergoes proteolytic degradation, generating specific peptides.42 The haem group is able to engage chemical reactions that result in the generation of free radicals,41 and oxidative stress is involved in AAA pathogenesis (see43 for review), through its ability to damage subjacent tissue.
Enhanced activity of oxidant enzymes and/or reduced activity of antioxidant chelators lead to oxidative stress. Myeloperoxidase, NADPH oxidase, and catalysis by iron have been described in human AAA. However, lipoxygenase and leukotriene hydrolase44 could also participate. In relation to this dysregulation, antioxidant enzymes, superoxide dismutase, glutathione peroxidise, and glutathione reductase activities were reduced in AAA tissue compared with non-diseased aorta.45,46 In an experimental model of AAA, catalase supplementation inhibited aneurysm formation.47 Thioredoxin is released from cells in response to oxidative stress,48 and increased thioredoxin levels have been recently reported in the conditioned medium of ILT.49 Moreover, circulating thioredoxin levels were correlated with AAA size and growth, highlighting the importance of oxidative stress.
The in vivo formation of fibrin is always associated with the retention of plasminogen and tissue-plasminogen activator, due to the biochemical ability of these two proteins to bind to free lysine residues present in the fibrin polymer. The process of fibrinolysis is postponed in vitro and in vivo to reinforce coagulation. In AAA, the initial retention of plasminogen and tissue-plasminogen activator from plasma, and urokinase-type plasminogen activator (u-PA), conveyed by neutrophils, together with some of their inhibitors, takes place in the most luminal ILT layer.33 However, active fibrinolysis is spatially and temporarily delayed, and the release of fibrin degradation products increases from the inner to the outer ILT layers.34 The strongest fibrinolytic activity is present at the abluminal interface between the ILT and the wall.34 Besides its ability to proteolyse fibrin, plasmin is able to activate MMPs, mobilize transforming growth factor-β from its extracellular matrix storage site, and degrade adhesive pericellular proteins, such as fibronectin, inducing mesenchymal cell detachment and death.50
Neutrophils are 12 times more numerous in clots than in circulating blood because these cells have a high affinity for the fibrin–fibronectin network, via integrins,51 and bind to platelet-exposed P-selectin via the expression of the sialyl Lewis-X-containing polysaccharidic ligand.52 Neutrophils are terminally differentiated cells, which undergo constitutive apoptosis after binding. Localization of neutrophils in the luminal part of the thrombus is associated with increased level of MMP-8, MMP-9,40 and elastase compared with other layers.53 Neutrophils could enhance both coagulation and fibrinolysis, because elastase can cleave tissue factor plasma inhibitor54 and fibrin.55
Leucocytes release granular serine proteases, such as u-PA, elastase, proteinase 3, and cathepsins (major components of the azurophilic granules), MMP-9 and MMP-8 (gelatinase granules), and pro-oxidant activities, such as NADPH oxidase and myeloperoxidase. The ILT is particularly rich in pro- and active forms of MMP-9,56 and MMP-9–lipocalin complexes are of neutrophil origin.57 Neutrophil proteases can degrade all matrix fibrillar proteins and thus provoke wall rupture. In particular, retention of neutrophils in the ILT impairs the ability of mesenchymal cell progenitors to colonize the thrombus, thus inhibiting the endogenous healing process.53 Cysteine proteases are also potent elastolytic and collagenolytic enzymes associated with AAA development. Several cathepsins42,58,59 and didpeptidyl peptidase I60 have been reported to be elevated in AAA tissue, in association with a decrease in their cystatin inhibitors.
5.3 Medial destruction
Lopez-Candales et al. demonstrated that medial smooth muscle cell density was decreased in human AAA tissues, associated with evidence of smooth muscle cell apoptosis.61 These data have been largely confirmed,62,63 including reports of accelerated replicative senescence in cultured smooth muscle cells from AAAs.64
The mediators responsible for medial degradation could either be conveyed from outside to inside by centripetal angiogenesis or from inside to outside by centrifugal mass transport. Centripetal angiogenesis is the main means of leucocyte extravasation from neo-capillaries into the aortic medial and adventitial tissues. Nevertheless, in the context of AAA, proteases generated by, stored and conveyed by the ILT are predominant.
The site of final adventitial rupture is characterized by a high level of protease expression65 and an important enrichment in leucocytes and focal neovascularization.66
5.4 Adventitial response
The adventitia reacts to the centrifugal insults by generating angiogenic, immuno-inflammatory, and fibrotic responses. Depending on the stage of AAA evolution, the immuno-inflammatory response includes accumulation of varying numbers of macrophages in the inner part of the adventitia, capable of phagocytosing iron originating from ILT haemoglobin, and adventitial tertiary lymphoid organ formation, providing evidence of the shift from innate to adaptive immunity (Figure 2). The adventitia can also be the site of an important fibroblastic reaction, probably driven by transforming growth factor-β, leading to collagen accumulation, thus delaying the risk of rupture.
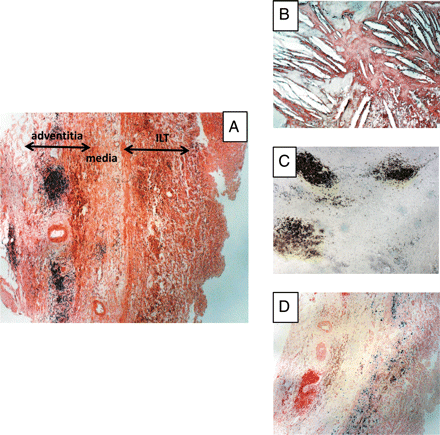
Biological features of the outer media and adventitia in human aneurysm of the abdominal aorta (AAA). (A) General view of the outer part of an AAA showing the inner ILT, the remaining media (difficult to delimit exactly in the absence of elastic laminae), and the highly vascularized outer adventitia, containing well-organized inflammatory granuloma (haematoxylin and eosin, ×4). (B) Cholesterol crystal clefts in the outer media (haematoxylin and eosin, ×10). (C) Granuloma can be easily identified as adventitial tertiary lymphoid organs by their high density of B lymphocytes (CD 20 immunostaining, ×4). (D) Presence of a high density of phagocytosed haemosiderin mainly in the outer part of the media (Perls blue staining, ×4), providing evidence of convection towards the adventitia of haem released by the ILT.
Neo-angiogenesis in the outer part of the AAA was described 15years ago.67 A strong spatial correlation between neo-capillaries, degradation of elastic fibres, and the extent of leucocyte infiltrate in the outer aortic wall of AAAs has been observed.68 However, in contrast to haemorragic plaques or occlusive thrombus, neo-vessels remain localized in the adventitia and the outer part of the media, but do not invade the ILT in large AAAs, probably because of an excess of local proteolytic activity.53
The main early function of macrophages in the AAA adventitia is probably phagocytosis. This is easily observed by Prussian blue staining of haemosiderin in macrophages of the outer media or inner adventitia, providing evidence of the convection of RBC degradation products from the luminal thrombus to the adventitia.
The observation of chronic peri-aortic infiltrates, consisting mainly of lymphocytes, monocytes, plasma cells, and sparse mast cells, usually on a background of fibrous tissue,69 has prompted use of the term ‘inflammatory AAA’ and retroperitoneal fibrosis70 as a distinct clinical entity, and the concept of an adventitial shift from innate to adaptive immunity in AAAs. Koch et al.71 observed that the adventitia in AAAs was enriched with lymphoid aggregates containing dendritic cells and activated endothelium.72 The similarity with lymphoid germinal centres has led to the proposal that lymphoid neogenesis takes place in the AAA adventitia.73 Attempts were then made to characterize the lymphocyte populations74 and to evaluate T-helper 1–T-hleper-2 balance,75 presence of B cells,76 and clonality77 but the neo-antigens, generated by the active thrombus and/or the degraded media and involved in this shift, remain to be identified.
Mast cells are tissue-resident leucocytes related to allergic reaction, including oedema, due to IgE–antigen interactions, leading to degranulation. Mast cells have numerous granules that contain chymases, and tryptase, histamine, growth factors, and cytokines, partly secreted in complexes with proteoglycans.78 Chymases and tryptase could act directly on the matrix but could also activate MMPs, degrade adhesion molecules,79 or activate the renin–angiotensin system.80 The role of mast cells in AAAs has been suggested in experimental transgenic mouse models.81 Mast cells, present in the outer media and adventitia of human AAAs,82 were reported to correlate with AAA diameter83 and the presence of an ILT, and to be in close association with adventitial angiogenesis.84 Since ‘inflammatory AAA’ has been recently shown to be related to high circulating levels of IgG4 and IgE,85 the relationship between secreted immuglobulins, the presence of adventitial mast cells, and inflammatory AAA remains to be established.
Fibroblasts play a critical role in the adventitial response to injury. They can proliferate and secrete procollagen-1, leading to perivascular fibrosis and resistance to rupture. Transforming growth factor-β, synthesized by polarized M2 macrophages and immune cells, is probably the main molecular link between inflammation and the peri-aortic fibrotic healing process.86 Pathogenic fibrosis of the adjacent retroperitoneum and rigid adherence to adjacent structures is usually observed in association with inflammatory AAAs.87
5.5 Possible involvement of weak pathogens
The clinical acceleration of AAA growth, the enrichment of ILT by neutrophils, and the potential impact of doxycycline,88 suggest the involvement of exogenous weak pathogens.
The first one to be explored in AAA was Chlamydia pneumoniae,89 infection by which could directly90 or indirectly (via lipopolysaccharides91 or antibodies92) contribute to AAA progression. However, the impact of C. pneumoniae on AAA progression remains highly controversial.
Recently bacterial DNA of Porphyromonas gingivalis (periodontal) and Streptococcus mutans (cariogen) have been identified in AAA tissue.93–95 Nevertheless, the results obtained with polymerase chain reaction technologies may be skewed by methodological contaminants.96 Oral bacteria are commensal or weak pathogens, but able to interact directly with activated platelets,97 making the ILT a privileged site for bacterial adhesion during transitory bacteraemia, without proliferation and possible phagocytosis by neutrophils.98 The interactions between coagulation, neutrophils, and bacterial retention have been recently emphasized in a model of blood clotting in mice.54
Periondotal disease has been epidemiologically linked to atherothrombotic clinical events.99 However, epidemiological data linking periodontal diseases and AAA are currently lacking.
6. Applications to diagnosis
Available molecular diagnostic tools are directly linked to the spatiotemporal pathophysiology of AAA as described above, and include circulating biomarkers and functional and molecular imaging.
6.1 Biomarkers
Circulating biomarkers of AAA have been recently reviewed.100,101 In terms of pathophysiology, circulating biomarkers can be classified in relation to ILT activities, extracellular matrix degradation in the wall, and adventitial immuno-inflammatory response. The discovery of new circulating biomarkers can be driven by pathophysiological knowledge or approached by modern open technologies, such as proteomics.102
The earliest biomarkers were directly related to extracellular matrix proteolysis. Serum elastin peptides and amino terminal propeptide of type III procollagen have been shown to be elevated in plasma of AAA patients,103 but with a relatively low sensitivity and specificity.104 Therefore, direct measurements of proteolytic enzymes have been used. Circulating plasmin–anti-plasmin complexes105 are particularly sensitive in AAA, associated or not with fibrin degradation products.106 It has been recently reported that plasmin–anti-plasmin and D-dimers107 were correlated with aortic diameter, ILT thickness, aneurysmal growth, and also with decreased pulmonary function108 in patients with AAA.109,110
Since numerous neutrophils are trapped within the ILT, several circulating markers of neutrophil activation (elastase–α1-antitrypsin complexes,14 myeloperoxidase, α-defensin) are increased in AAA.111 Moreover, α1-antitrypsin associated with circulating high-density lipoprotein is decreased in plasma of patients with AAA,16 providing evidence of its excess consumption. In a similar way, the plasma level of cystatin C, an inhibitor of cysteine proteases, is decreased in inverse relation to AAA dimensions.112 In particular, MMP-9113 or MMP-9–neutrophil gelatinase-associated lipocalin (and MMP-8) plasma levels could be used for monitoring treatment efficiency.114
Circulating markers of platelet activation are also increased in AAA,40 as are circulating plasma thrombin–antithrombin complexes.40 The simultaneous association of increased biomarkers of both coagulation and fibrinolysis in AAA patients provides evidence of the biological activity of the ILT.109,110 However, circulating markers of oxidative stress remain to be identified and validated in AAAs.
Circulating inflammatory biomarkers and markers of the shift from innate to adaptive immunity are elevated in patients with AAA. Plasma inflammatory cytokines,115 such as interferon-γ, tumour necrosis factor-α,116 interleukin-6, and C-reactive protein,117 are all elevated in the plasma of AAA patients, providing evidence of an inflammatory process associated with AAA development.
Chlamydia pneumoniae antibodies in serum of AAA patients have been searched for with variable success,118 questioning the specificity of this analysis.119 More interesting is the detection of elevated levels of IgG4 and IgE in the serum of patients with inflammatory AAA.85 The IgG4 syndrome is reported to be associated with an active sclerotic process,120 and the IgE syndrome with a different aneurysmal localization.121
6.2 Imaging
Besides the technological progress, new knowledge in tissue biology has an impact on the evolution of imaging from simply viewing morphology to detecting function, and on the development of new molecular imaging of biological processes. This progress not only impacts the pathophysiology of the disease but could also provide surrogate markers of therapeutic efficiency.
6.2.1 Calcifications
Calcifications, easily identifiable by X-ray, the oldest method for visualizing AAAs, are common features in AAA and are not devoid of biological significance. Hydroxyapatite precipitation in soft tissue is linked to cell death and membrane particle formation and binding to the extracellular matrix. In AAA, calcifications are usually localized in the outer part of the media, thus delimiting the external side of the aneurysmal dilatation. Lindholt et al. have recently reported a protective effect of calcification in the evolution of AAA,122 probably linked to the greater resistance of calcified tissue to proteolysis.
6.2.2 Bleeding into the ILT
This was initially named the ‘crescent sign', and it is caused by contrast medium entering the ILT. High attenuation within the ILT, detected by ultrasonography and computed tomography scan, was reported early,123 and correlated with ‘liquefaction’ of the thrombus, providing evidence of mechanical failure of the ILT when exposed to a pulsatile load.124 Subsequently, this crescent sign in the ILT was suggested to signal an impending risk of rupture.125,126
6.2.3 Iron oxide contrast in magnetic resonance imaging
The ILT-trapped RBCs release paramagnetic iron from haem that causes signal loss in magnetic resonance imaging. Nevertheless, spontaneous signal loss obtained using conventional approaches may lack sensitivity and specificity. Therefore, imaging of iron phagocytic activity could be enhanced by injections of exogenous iron particle contrast agents, such small paramagnetic iron oxide.127 Using this technique, both luminal and adventitial iron phagocytosis, by neutrophils and macrophages, respectively, have been identified by magnetic resonance imaging.
6.2.4 Platelet activation in scintigraphy
Annexin-V binds specifically, with nanomolar affinity, to phosphatidylserine, which is exposed on the surface of activated platelets and apoptotic cells. Therefore, radiolabelled 99mTc-annexin-V has been used for assessing the renewal activity of the ILT in an in vivo experimental model of AAA, and ex vivo in human ILT.128 A form for human use is now under development, which should soon be validated in clinical investigations.
6.2.5 18-FDG PET scan
Positron emission tomography (PET) studies are usually performed using 18-fluorodeoxyglucose (FDG) as radiotracer, which reflects glucose uptake depending on cell metabolic activity. Modern hybrid scanners are coupled with morphological computed tomography or with magnetic resonance imaging for attenuation correction and anatomical mapping.
Sakalihasan et al.129 have investigated by PET the increased metabolism of an expanding AAA and/or one threatening rupture and observed, in some cases, an association between 18-FDG uptake by the aneurysm wall and a rapid expansion of the aneurysm. The 18-FDG was usually observed in the outer part of the AAA wall and thus probably reflects the presence of a high density of active leucocytes in the adventitia.130 These preliminary observations were recently confirmed.131 However, in accordance with the reports of Sakalihasan129 and Truijers,132 no correlation between maximal standard uptake value and maximal cross-sectional infrarenal AAA diameter was found. Recently, Xu et al. observed an association between high wall stress and increased metabolic activity in aneurysmal wall evaluated by PET–computed tomography.133 Therefore, this method constitutes a new tool for exploring some aspects of wall biology in AAAs.
7. Therapeutic implications
Apart from surgical or endovascular interventional therapeutics for large aneurysms, and control of cardiovascular risk factors, including smoking cessation, the development of specific medical treatment able to limit aneurysmal growth, or even to induce regression, remains an unattained challenge. This challenge is particularly important in the context of screening programmes aimed at detecting asymptomatic small aneurysms. Experimental pharmacological134 and clinical medical135 treatments of AAA have been recently reviewed, including statins.136 In a recent complement, doxycycline has been reported to decrease AAA wall infiltration by neutrophils and lymphocytes.88,137 An annual 4 week treatment by roxithromycin has been reported to significantly inhibit AAA growth rate,138,139 but randomized trials with large numbers of patients and long follow-up are now necessary.
Since ILT renewal associated with AAA progression could be a therapeutic target, the ability of platelet inhibition to prevent growth of aneurysms was tested experimentally.140 This hypothesis was then tested through an observational study in patients with small AAAs. Treatment of patients with aspirin delayed AAA growth and reduced the number of cases of interventional therapeutics.141 This beneficial effect has been recently confirmed in another observational study,118 but not by the UK study.142 Nevertheless, as for other medical approaches, a prospective interventional assay is needed.
Since angiotensin II has been demonstrated to be possibly involved in experimental aneurysm in apoE–/– mice,143 the effects of renin–angiotensin system inhibition on AAA progression have been evaluated in observational case–control studies. The first Canadian study reported that angiotensin-converting enzyme inhibitors decreased the risk of rupture,144 whereas the UK AAA screening programme showed an increased growth rate associated with angiotensin-converting enzyme inhibitors.142 This observation underscores the urgent need for prospective randomized trials.
8. Conclusions: aneurysm of the abdominal aorta, a spatiotemporal model of atherothrombosis
There is now observational evidence that the intraluminal, haemoglobin-rich thrombus, together with the adventitial angiogenic145 and immune responses, play important roles in the evolution of atherothrombosis from the initial stages to clinical complications (Figure 3). Therefore, AAA represents a unique, well-organized, spatiotemporal model of human atherothrombosis, associating all the tissue, cell, and molecular components of the pathology, offering a unique opportunity to decipher the pathophysiology of human atherothrombosis and to develop new concepts, as well as future diagnostic and therapeutic tools.
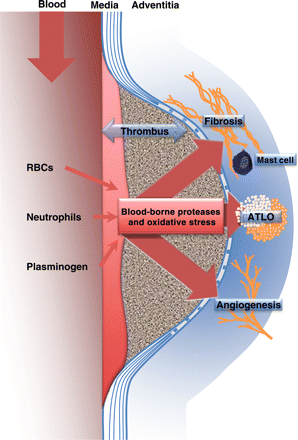
Schematic representation of the impact of the blood–ILT interface on medial degradation and the adventitial inflammatory, angiogenic and fibrotic responses in human AAA. Abbreviations: ATLO, adventitial tertiary lymphoid organ; and RBCs, red blood cells.
Conflict of interest: none declared.
Funding
All partners were supported by the European Community FAD project (FP-7, HEALTH F2-2008-200647). Funding to pay the Open Access publication charge was provided by the FP-7 EU integrated project ‘Fighting Aneurysmal Disease, FAD'.
References
Author notes
The ‘Fighting Aneurysmal Disease' (FAD, #217) European integrated project (Health-F2-2008-200647), http://www.fighting-aneurysm.org/