-
PDF
- Split View
-
Views
-
Cite
Cite
Carolina Magdalen Greco, Enzo Nisoli, The ketogenic diet is unable to improve cardiac function in ischaemic heart failure: an unexpected result?, Cardiovascular Research, Volume 120, Issue 10, July 2024, Pages 1097–1099, https://doi.org/10.1093/cvr/cvae126
- Share Icon Share
This editorial refers to ‘The ketogenic diet does not improve cardiac function and blunts glucose oxidation in ischaemic heart failure’, by K.L. Ho et al., https://doi.org/10.1093/cvr/cvae092.
Heart failure (HF) poses a significant public health challenge as a primary cause of morbidity and mortality worldwide. Even though HF with preserved ejection fraction (HFpEF, defined as having a left ventricular ejection fraction of 50% or higher) has emerged as the most common form of HF, heart failure with reduced ejection fraction (HFrEF, ejection fraction under 40%)—mainly consequent to coronary artery disease and myocardial infarction (MI)—continues to be a critical clinical issue. Despite a wide array of available drug-based and non-drug treatments, the prognosis for HFrEF patients five years after diagnosis remains poor, highlighting the urgent need for new treatment strategies.1 HFrEF was seminally defined nearly two decades ago as ‘An engine out of fuel’ as the consequence of major changes in cardiac metabolism leading to the reduction of energetic equivalents, i.e. reduction in phosphocreatine/adenosine triphosphate (ATP) ratio.2 Consequently, understanding how changes in the heart’s energy metabolism affect the onset and progression of HF has become a key area of investigation.
Until recently, a detailed understanding of how the human heart utilizes nutrients to sustain its continuous contraction was lacking. Through metabolomic analysis of blood samples from the artery, coronary sinus, and femoral vein of more than 100 individuals, research team led by Arany has conclusively shown that the heart predominantly uses fatty acids (FFAs) as an energy fuel, while surprisingly consuming minimal amount of glucose.3 Furthermore, the study revealed that the heart secretes glutamine and other nitrogen-rich amino acids—suggestive of active protein degradation—at a rate approximately 10 times higher than that of the leg muscles. It also showed that the heart releases intermediates of the tricarboxylic acid (TCA) cycle; the calculated anaplerotic carbon input from amino acid consumption exceeds carbon released as TCA cycle intermediates.3 Additionally, the research found that both the heart and leg muscles metabolize ketone bodies, glutamate, and acetate in direct correlation with their levels in circulation, highlighting the importance of substrate availability in their utilization.3
Remarkably, healthy heart tissue can adapt its energy source based on the availability of different nutrients, a capability known as metabolic flexibility. This adaptability is crucial, especially in HFrEF, where losing this metabolic flexibility marks a significant progression of the disease. Although there is debate over whether the rate of fatty acid β-oxidation (FAO) is elevated, remains unchanged, or decreases as the disease advances, it is generally agreed that the heart’s capacity to metabolize glucose (i.e. glucose oxidation) becomes impaired. This impairment leads to an energy deficit within the heart, exacerbating HF. Interestingly, this effect is partially counteracted by increased ketone body (KB) oxidation, an adaptive response to mitigate the energy shortfall.4
Enhancing ketone oxidation in such hearts boosts cardiac energy output without significantly impacting glucose or FAO.4 Both animal studies and preliminary clinical research have highlighted the potential benefits of ketones, ranging from improvements in endothelial function, reduction of oxidative stress and enhancements in mitochondrial activity, decreased inflammation, and overall leading to positive effects on cardiac remodelling (reviewed in Matsuura et al.5). Furthermore, ketones are associated with decreased body weight and better control of blood glucose and lipid levels.5 However, the cardiovascular implications of increased ketone supply to the heart still need to be fully understood, with studies in rodent models and humans yielding inconsistent outcomes.
Ketone supplementation can be achieved via dietary interventions or via delivery or exogenous ketone esters. In this issue of Cardiovascular Research, Ho et al.6 investigated the efficacy of a ketogenic diet (KD) in ameliorating cardiac function in a mouse model of ischaemic heart failure. The KD is a popular high-fat, low-carbohydrate diet that causes blood ketone levels to increase due to the restriction of carbohydrates and decreased insulin signalling. As such, it could be used as a strategy to augment ketone delivery to the heart. Two weeks after sham or permanent left anterior descending (LAD) coronary artery ligation surgery, C57BL/6J male mice were fed with either a regular, chow diet (80% carbohydrates, 10% fat, and 10% protein) or a KD (5% carbohydrates, 85% fat, and 10% protein) for three weeks. Firstly, the authors assessed in vivo cardiac function and structure with transthoracic echocardiographic analysis. The results demonstrated that, while the KD promoted the expected metabolic effects—such as reduced body weight in healthy mice, modified fasted blood glucose, and increased ketone body levels in HF mice—these did not translate into improvements in cardiac function. Specifically, the diet did not ameliorate ejection fraction or diastolic function in the MI mice, indicating no beneficial effect on systolic or diastolic HF conditions (Figure 1).
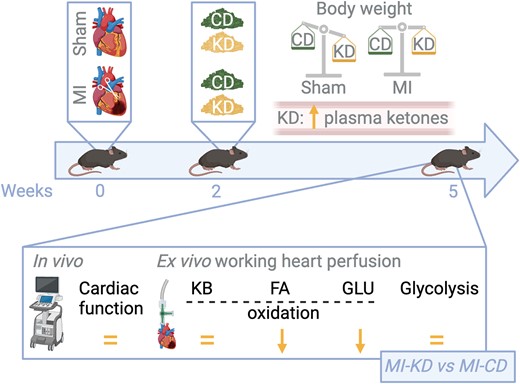
The ketogenic diet is unable to improve cardiac function in ischaemic heart failure (referred to as MI, myocardial infarction). C57BL/6J male mice underwent either a sham surgery or permanent left anterior descending coronary artery ligation surgery to induce heart failure. After two weeks, mice were treated with either a control diet (regular chow diet, CD) or a ketogenic diet (KD) for three weeks. Although promoting the expected metabolic effects, including reduction of body weight in healthy mice, in addition to increasing circulating ketone bodies and reducing fasted blood glucose levels in failing heart mice, KD did not ameliorate systolic and diastolic dysfunction (in vivo echocardiographic measurements) or cardiac work (ex vivo measurements in isolated working heart perfusions). The KD diet did not increase cardiac ketone oxidation (KB oxidation) rates. Instead, it increased the heart’s dependence on fatty acids (FA) for energy production due to a decrease in glucose (GLU) oxidation in the failing heart. FA oxidation (FAO) and glycolytic rates were unchanged in sham or ischaemic mice fed CD or KD. The KD diet decreases cardiac efficiency (measured as cardiac work/O2 consumed ratio). Oxygen consumption was measured in the isolated working hearts: in sham hearts, KD did not affect oxygen consumption or cardiac efficiency (regardless of whether insulin was present or absent); while MI CD hearts had lower oxygen consumption than sham CD hearts, cardiac efficiency was not decreased, primarily due to the lower cardiac work in the MI CD hearts. On the contrary, the KD decreased cardiac efficiency in the MI hearts, consistently with the increased dependence of these hearts on FAO. The figure was created with BioRender.com.
Second, after working hearts were isolated from these animals, glucose, fatty acid, and ketone oxidation rates were measured with the appropriately labelled markers, in addition to glycolysis. This investigation revealed that, although KD increased circulating levels of ketone bodies, it did not increase ketone oxidation rates in the heart, contrary to initial hypotheses. This finding is significant when considering the potential of increased ketone body supplementation to support cardiac function in HF. Instead, in line with a systemic switch to fatty acids as the main energy source, the diet markedly decreased glucose oxidation and shifted the heart’s metabolic reliance towards FAO. This metabolic alteration led to a substantial decrease in glucose-derived ATP production even in the presence of insulin. Indeed, while sham and LAD-operated animals fed with the control diet efficiently oxidized glucose upon insulin stimulation, mice fed the KD failed to do so, suggesting that KD may lead to cardiac insulin resistance. FAO and glycolysis were not different among the diverse experimental groups. This was particularly noticeable in conditions simulating insulin presence, further hinting for decreased cardiac insulin sensitivity under the KD.
Third, protein expression analyses related to metabolic pathways, highlighted that KD suppressed the induction of BDH1 expression in MI hearts. BDH1 (β-hydroxybutyrate dehydrogenase 1) is a critical enzyme in ketone metabolism. Its expression typically rises in HF as the organ shifts to the usage of alternative fuels and increasing its reliance on ketone bodies for energy production (Figure 1). However, the unchanged ketone body oxidation rates, alongside the impaired induction of BDH1, suggest a complex metabolic response to the KD that does not straightforwardly translate into enhanced ketone utilization by the heart.
Finally, the study assessed the KD’s effect on cardiac efficiency (i.e. cardiac work/oxygen consumption), considering the relative inefficiency of FAO (compared to glucose oxidation) as an energy source for the failing heart. FAO requires more oxygen per unit of energy produced, making it a less efficient process. The findings confirmed a decrease in cardiac efficiency in MI hearts under the KD, attributed to the diet’s promotion of FAO as the primary energy source. Overall, this detailed exploration into the effects of a KD on HF in mice revealed significant metabolic shifts towards FAO at the expense of glucose oxidation. However, these changes did not improve cardiac function or efficiency, challenging the potential therapeutic benefits of KDs for the treatment of HF.
The authors designed a KD to include 5% carbohydrates to represent the diet composition of a human KD more closely, yet there are animal studies that utilize 0% carbohydrates. A critical point regarding the isocaloric vs. non-isocaloric effects of a KD remains to be considered. In a previous study, the same group had investigated the effects of a KD on various metabolic parameters and found that the caloric intake between the control diet vs. the KD was not significantly different in wildtype or ob/ob mice.7 However, the possibility remains that isocaloric administration of the control and KDs can result in changes in whole-body metabolism (e.g. weight, blood glucose levels, and blood ketone levels); nonetheless, chances that isocaloric administration of these diets would produce significantly different cardiac metabolic findings is unlikely, as the heart would still be increasingly relying more on fatty acids than glucose for energy. Future studies investigating the isocaloric effects of a KD would be interesting in the setting of HF or the diverse types of HF.
Another critical point is that when designing the diet, the researchers can source the fat from different products with various saturated and polyunsaturated FA amounts (e.g. lard or cocoa butter). An important factor that needs to be considered when choosing between the two sources of fat is how food is administered to the mice; since the KD has such a high percentage of calories coming from fat (85%), a diet sourcing fat from lard does not pellet adequately, so one should consider introducing a different method of feeding to mice than what they are used to (paste vs. pellets). Ho et al.6 chose to have the source of fat in their KD from cocoa butter since this allowed them to keep the same feeding method between studies and experiments. However, the saturated and polyunsaturated FA concentrations differ between lard and cocoa butter. Lard has a higher total polyunsaturated FA percentage (53.86%) compared to cocoa butter (33.74%); cocoa butter has a total saturated fatty acid value of 66.27%.8 A traditional KD consists of a higher concentration of saturated than polyunsaturated FAs; the used KD was high in saturated FAs and could have contributed to the lack of benefits observed in the present study. Therefore, a KD higher in polyunsaturated fatty acids (i.e. source of fat from lard) could produce different results, since replacing saturated fatty acids with polyunsaturated fatty acids has been associated with decreased cardiac risk. Future studies that investigate the effects of different types of KDs (source of fat as well as percentage of fat) in the setting of HF should be carried out.
Recent studies have shed light on the crucial role of mitochondrial pyruvate carriers 1 and 2 (MPC1 and MPC2) for cardiac metabolism. These proteins are essential for transporting pyruvate into mitochondria, which serves as a substrate for the tricarboxylic acid (TCA) cycle through acetyl-CoA. Genetic experiments that involved the cardiac-specific deletion of MPC1 or MPC2 revealed severe consequences, including cardiac hypertrophy, HF, premature death, and compromised cardiac mitochondrial pyruvate utilization.9–11 On the other hand, enhancing MPC1 expression, thereby stimulating MPC activity in the heart, led to improved cardiac function and reduced hypertrophy following transverse aortic constriction (TAC). KD was shown to reverse the metabolic defects of mice with MPC deletion. However, only pre-treatment with KD for three weeks prior to TAC offered protection; while administration of KD with the onset of injury did not mitigate the effects post-TAC.10,11 Investigations into the mechanisms behind these outcomes revealed that the beneficial effects were due to enhanced FAO rather than oxidation of ketones. This conclusion was further supported by feeding the mice with a high-fat diet, which yielded similar results to the KD. Also in a different HF model, Ho et al.6 found that the KD did not increase ketone oxidation in the heart; instead, KD markedly decreased glucose oxidation and shifted the heart’s metabolic reliance towards FAO.
The study of chronic alterations in the structure and function of the heart muscle, the development of left ventricle dysfunction, and the progression towards HF is possible thanks to various murine models. Cardiac remodelling and dysfunction may result from myocardial injury or pressure overload due to TAC or can be studied in genetic models of dilated cardiomyopathy. Therefore, examining the impact of different KDs in these HF models, extending beyond the context of LAD coronary artery ligation as proposed by Ho et al.,6 is warranted. An essential consideration in this research will be the duration of dietary intervention: could extended periods on the KD yield beneficial or even detrimental outcomes?
Furthermore, investigating the relationship between the KD and intestinal microbiota is a crucial aspect, especially in translating findings to human applications. The gut microbiome is known to be responsive both to HF conditions and to changes introduced by experimental diets.12 The possibility that the KD might influence liver ketone production differently in humans with HF compared to mice could alter the level of ketosis achieved and, consequently, the amount of ketones delivered to the heart. This aspect underscores the complex interplay between diet, metabolic health, and heart function, highlighting the need for comprehensive research to unravel these relationships and their implications for HF therapy.
Acknowledgements
We are indebted to Chiara Ruocco for her valuable support in preparing the figure and discussion, as well as to Maurizio Ragni.
Funding
C.M.G. has been supported by the Italian Ministry of University and Research (grant #P2022CXN7X and #MSCA_0000075) and by the CARIPLO Foundation (grant #2022-0523). E.N. has been supported by the Italian Ministry of University and Research (grant # 20205X4C9E and # 2022XZ7MBC) and by Professional Dietetics (Milan, Italy) liberal contributions.
Data availability
No new data were generated or analysed in support of this research.
References
Author notes
The opinions expressed in this article are not necessarily those of the Editors of Cardiovascular Research or of the European Society of Cardiology.
Conflict of interest: none declared.