Abstract
The contribution of the right ventricle (RV) to cardiac output is negligible in normal resting conditions when pressures in the pulmonary circulation are low. However, the RV becomes relevant in healthy subjects during exercise and definitely so in patients with increased pulmonary artery pressures both at rest and during exercise. The adaptation of RV function to loading rests basically on an increased contractility. This is assessed by RV end-systolic elastance (Ees) to match afterload assessed by arterial elastance (Ea). The system has reserve as the Ees/Ea ratio or its imaging surrogate ejection fraction has to decrease by more than half, before the RV undergoes an increase in dimensions with eventual increase in filling pressures and systemic congestion. RV-arterial uncoupling is accompanied by an increase in diastolic elastance. Measurements of RV systolic function but also of diastolic function predict outcome in any cause pulmonary hypertension and heart failure with or without preserved left ventricular ejection fraction. Pathobiological changes in the overloaded RV include a combination of myocardial fibre hypertrophy, fibrosis and capillary rarefaction, a titin phosphorylation-related displacement of myofibril tension–length relationships to higher pressures, a metabolic shift from mitochondrial free fatty acid oxidation to cytoplasmic glycolysis, toxic lipid accumulation, and activation of apoptotic and inflammatory signalling pathways. Treatment of RV failure rests on the relief of excessive loading.
1. Introduction: Relevance of the right ventricle
The right ventricle (RV) has long been thought to be dispensable. In 1943, Starr et al. showed that ablation of the RV in dogs is compatible with life with little change in venous pressures.1 In 1971, Fontan and Baudet introduced the cavo-pulmonary anastomosis as a palliative intervention for certain cardiac malformations.2 So-called Fontan patients indeed enjoy a near-normal sedentary life without RV for several decades.3 However, they have a 30–50% decrease in aerobic exercise capacity.4 This indirectly discloses the contribution of the RV to maximum cardiac output.4 Furthermore, any cause increase in pulmonary artery pressure (PAP) in Fontan patients is a cause of circulatory failure.3 Thus, the RV may be dispensable but only in a quiet sedentary life, with a normal cardiac output and a low-resistance pulmonary circulation.
The RV in mammals and in birds is a thin-walled crescent shape volume generator coupled to systemic venous return on one side and to the pulmonary circulation on the other side. This structural particularity is the result from a Darwinian selection process.5 Evolution from poikilothermic reptiles and amphibians to endothermic mammals and birds requires an increased gas exchange to meet metabolic needs. Sufficient oxygen and carbon dioxide exchange are only possible through an extremely thin blood–gas barrier. Such a structure is efficient but fragile. It is therefore protected from high systemic vascular pressures within a separate low resistance pulmonary circulation.5
The structure of the RV is not designed to cope with brisk increases in PAP. In anaesthetized animals, pulmonary ensnarement to a systolic pressure exceeding 60 mmHg is associated with extreme RV dilatation and circulatory collapse.6 However, if given time, the RV adapts by an increased contractility. This ‘homeometric' or inotropic adaptation is turned-on within 3–5 min and is not therefore dependent on hypertrophic remodelling. In Starling's heart–lung preparation, an acute increase of either preload or afterload is immediately associated with an increase in end-diastolic volume and end-systolic volume (EDV and ESV), a decrease ejection fraction (EF), and a preserved stroke volume (SV).7 After several minutes, this ‘heterometric adaptation', which conforms to so-called Starling's law of the heart, is replaced by a homeometric adaptation as EDV returns to normal, ESV is decreased, EF is increased, and SV is back to baseline.8 It is now better understood that Starling's law of the heart applies to beat-by-beat adaptations, for example with changes in body position or when the homeometric adaptation eventually fails in patients with chronically overloaded hearts.
In spite of embryologic and structural differences, homeometric and heterometric adaptations to changes in loading conditions are basically the same for the RV and for the left ventricle (LV).9 However, contractility responses to increased preload or afterload vary in magnitude depending on time course of increased loading, volume status, reactive ventricular hypertrophy, the presence or not of systemic diseases, and yet unknown differences in molecular signalling pathways. The progression from homeometric adaptation to predominant heterometric adaptation is not an on–off phenomenon but evolves in variable proportions. In permanently increased loading conditions such as in severe pulmonary hypertension (PH), functional state, exercise capacity, and cardiac output may remain reasonably well preserved at rest, while right heart dimensions progressively increase along with a decreased EF, altered exercise capacity, and shortened life expectancy.10,11
The RV has attracted a lot of attention in recent years with accumulation of evidence of its prognostic relevance in severe PH, any cause heart failure (HF), and systemic diseases. The present review aims at an update of gold standard vs. clinically applicable methods to assess RV function with consideration of new pathophysiological insights and a selection of basic science advances of translational pertinence.
2. RV overload
Ventricular function is determined by preload, afterload, and contractility. While RV preload is easily defined as EDV, which is the moment in the cardiac cycle when myocardial fibres are maximally stretched before the isovolumic contraction, there are several equally valid but conceptually different definitions of RV afterload.10–12
The first is maximum wall tension, or the maximum value of the product of ventricular volume by ventricular pressure, divided by wall thickness (T) as an application of Laplace's law for spherical structures.
Maximum wall tension is increased by volume loading as well as by pressure loading. Starling taught that preload and afterload are as inseparable as the ebb and the flow. However, while maximum wall tension may be considered as the reference definition of afterload, it is difficult to apply to the RV because of its irregular shape and inhomogeneous contraction.
The second is the assessment of the forces that oppose RV ejection by hydraulic power (WTOT), calculated from the integration of instantaneous pulmonary arterial (PA) pressure and flow waves. Total power WTOT is the sum of steady power (WST), which defines the work of mean forward flow, and oscillatory power (WOSC), which defines the additional work entailed to oscillations.
WST is equal to the product of mean PAP (mPAP) by SV.
Because of the near-constancy of PA compliance to pulmonary vascular resistance (PVR) relationship, or ‘time constant' of the pulmonary circulation, WOSC can be calculated to be stable at 23% of WTOT,13 and therefore WTOT is estimated at 1.3 times mean power WST.
However, this estimation carries uncertainty because of inevitable errors on mPAP calculated from systolic (sPAP) and diastolic or derived from the integration of a PAP wave, and imprecision of the time constant calculation.13–15
The third is pulmonary vascular impedance (PVZ) or oscillatory PA pressure as a function of oscillatory PA flow.15 A PVZ calculation integrates all the forces that oppose PA forward flow. The determination of PVZ requires high-fidelity instantaneous pressure and flow measurements and a frequency analysis. The result is graphically represented by a PVZ spectrum or pressure-flow ratio and phase lag as functions of frequency. Relevant numbers of the spectrum are 0 Hz impedance (Zo), or mPAP divided by cardiac output also called total PVR, high-frequency impedance also called characteristic impedance (Zc), first minimum and maximum of the pressure-flow moduli and low-frequency phase. In this analysis, Zo is sensitive to changes in peripheral resistance vessels, while Zc is sensitive to proximal PA elastance. Measuring a PVZ spectrum is unpractical at the bedside because of the needs of expensive and sophisticated catheter technology and results expressed as several numbers in the frequency domain most clinicians are not familiar with. None of the numbers defining a PVZ spectrum can be considered as a sole measure of afterload.
The fourth is arterial elastance (Ea) or RV end-systolic pressure (ESP) divided by SV.
Ea measures afterload at the moment of the cardiac cycle when RV elastance is maximal.10–12 Maximum elastance as a gold standard estimate of contractility in an intact animal occurs before end-systole in the normal RV but coincides with end-systole in the RV coupled to a hypertensive pulmonary circulation. End-systolic elastance (Ees) is generally an acceptable approximation for maximum elastance of the RV.10–12
It is sometimes assumed that mPAP could be a reasonable approximation of ESP, so that,
or total PVR divided by heart rate.
However, in patients with PH, mPAP under-estimates ESP in proportion to increased systolic pressures roughly following the relationship.16
Because of relatively wide scattering of data points and insufficient data from patients with normal or mildly hypertensive pulmonary circulation, it is preferable to calculate Ea from directly measured ESP. Defining ESP requires the determination of a PV loop.
3. Coupling the RV to the pulmonary circulation
Measurements of either right or LV Ees and Ea can be obtained from a family of pressure–volume loops at several levels of preload17,18 or from single beat ventricular pressure and flow output measurements.19,20 This is illustrated in Figure 1. The single beat method is convenient as it avoids technically demanding measurements at variable levels of loading and may be preferred for the RV to circumvent the difficulty of absolute ventricular volume measurements.
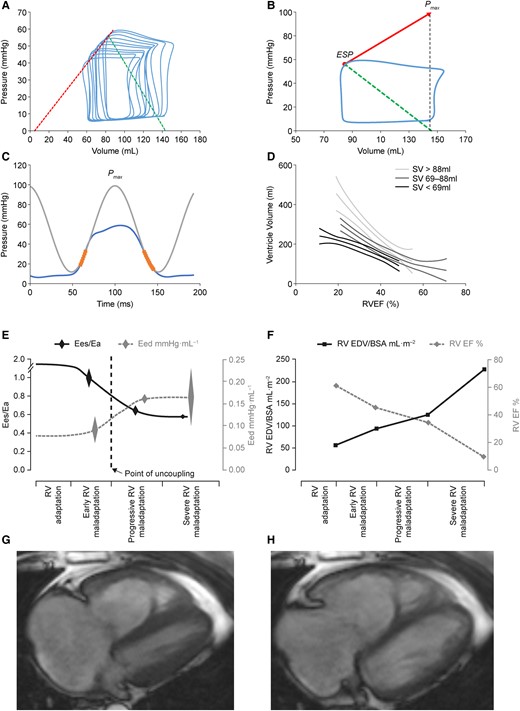
Figure 1
Multiple (A) and single beat (B) pressure–volume loops to calculate RV-arterial coupling, RV pressure curve with Pmax calculation (C) and EDV to EF relationships (D), evolution of respectively Ees/Ea, Eed (E) and EF and EDV (F) in patients with progressively severe PH, and MRI of end-systolic (G) and EDVs (H) to calculate EF. After reference21 with permission. BSA, body surface area.
Single beat RV-PA coupling relies on a maximum RV pressure (Pmax) calculation from the non-linear extrapolation of isovolumic portions of a ventricular RV pressure curve (thus corresponding to peak RV pressure during a non-ejecting beat) and measurement of P as a function of V during RV ejection.20 A straight line tangent to upper left shoulders of a family of PV loops or from Pmax tangent to a single PV curve defines Ees. A straight line drawn from the Ees to end-diastolic elastance (Eed) points of any single PV loop defines Ea (Figure 1).
The coupling of RV function to the pulmonary circulation in PA hypertension (PAH) was first reported with single-beat calculation of the Ees/Ea ratio from magnetic resonance imaging (MRI) measurements of RV volumes and standard fluid-filled catheter measurements of RV pressures in six stable patients.22 The striking finding of that study was that Ees was increased, but insufficiently so to match an even more important increase in Ea, so that Ees/Ea was halved as compared to controls indicating insufficient contractility (‘homeometric') adaptation to afterload. Yet, EDV and ESV were not increased, indicating adequate compensation of RV function in keeping with considerable Ees/Ea reserve. These results have been confirmed in small cohorts of patients with either idiopathic or systemic sclerosis (SSc)-associated PAH or chronic thromboembolic PH (CTEPH) and in one patient with a systemic RV.23–28 In these studies, Ees/Ea was measured using gold standard micromanometre-tipped catheter technology and the single beat method22,23,25,26,28 or the multiple-beat method using a decrease in venous return induced by a Valsalva manoeuvre.24,27 Exercise decreased Ees/Ea in PH patients, in contrast to preserved Ees/Ea during exercise in controls.26 Exercise-induced decrease in Ees/Ea was associated with an increase in EDV, which was typically observed in SSc-associated PAH.27 These clinical studies confirmed observations in experimental animal models of HF, sepsis, and PH.10
How does the overloaded RV evolve from adaptation (preserved volumes) to failure (increased volumes)? The critical levels of decoupling associated with onset of increased dimension (‘heterometric') adaptation and eventual clinically identifiable congestion in patients with severe PH are not exactly known.10–12 The optimal Ees/Ea ratio for ejection at minimal energy cost was initially modelled to be higher than 1, actually in the range of 1.5–2.10 However, the limits of normal of the Ees/Ea ratio have not been exactly defined in clinical studies. In experimental animal models of embolic PH, the RV dilated when the Ees/Ea ratio decreased to 0.7–1.0.29,30 It was recently shown in a study combining experimental animal observations and measurements in patients with CTEPH that the Ees/Ea ratio could be decreased down to 0.68 before the onset of decreased SV.28 These observations have been confirmed.21
Thus, while being gold standard measures of contractility and afterload, Ees and Ea and Ees/Ea determinations may not be sensitive to clinical deterioration until advanced stages of PH.10,11 On the other hand, the persistence of increased Ees and maintained Ees/Ea in severe PH makes the measurement insensitive to efficient therapeutic interventions. Epoprostenol in experimental PH decreased Ees along with decreased PVR, but this did not indicate a negative inotropic effect as the Ees/Ea ratio was maintained.31 A similar observation was reported in PAH patients on chronic prostanoid therapy, in whom PVR and RV volumes decreased, SV and EF increased, and indices of diastolic function improved in spite of decreased Ees and unchanged Ees/Ea.32 These observations may be explained by the active adaptation of contractility to afterload, by yet unknown mechanisms.31 An alternative explanation is in the non-linearity of end-systolic PV curves.32 These have been shown to present with increased slopes when ventricular pressure increases, so that Ees decreases along with the unstressed ventricular volume with effective PH therapies.33
The PV loop has of the LV a square shape with easily identifiable Ees at its upper left corner.17 The normal RV PV loop has a rounded triangular shape with early systolic peaking of pressure and persisting ejection after Ees which is therefore more difficult to discern.18 However, the shape of RV PV loops changes with progression of PH, from a triangular shape with early systolic peaking of pressure, to trapezoid and triangular shapes with late systolic peaking of pressure and mid systolic dip of pressure (‘notching').34,35 These morphological changes have been shown to be of prognostic relevance.35 The coupling of RV function to the pulmonary circulation assessed by Ees/Ea is better preserved at similar severity of PH in female compared to male patients.36 In general, the Ees/Ea ratio is an independent predictor of outcome in PH patients, with a cut-off value for prognostic discrimination of 0.65 to 0.8.37,38
Single beat and multi-beat measurements of Ees and Ea produce essentially the same results.37 However, because of sympathetically mediated increase in Ea at reduced cardiac output20 and the non-linearity of end-systolic P-V relationships,33 this may not be confirmed at lower or higher than normal cardiac output.
High-fidelity measurements of instantaneous RV pressures and volumes and derived elastance calculations have contributed to current understanding of RV function adaptation to loading conditions in a variety of cardiopulmonary conditions but are technically demanding and therefore not suitable for daily bedside clinical practice.39
4. Simplified measurements of RV-arterial coupling at the bedside
Since the Ees/Ea ratio has pressure as a common term, it can be simplified to a ratio of volumes, easy to measure with MRI40:
Simple modelling predicts that ESV and EDV increase above the upper limits of normal in a SV-dependent fashion when SV/ESV decreases below 54% and SV is normal.41 Since SV is equal to EDV−ESV and thus SV/ESV = EF/(1−EF), this corresponds to a RV EF of 35%.41 It has to be noted that because of their curvilinear relationship, SV/ESV and EF are more sensitive to early and late stages of RV dysfunction, respectively.41
It is interesting that both SV/ESV and EF of 54 and 35% are effectively associated with RV volumes above the upper limit of normal21 and correspond to cut-off values discriminating poor from favourable outcome in severe PH.42,43
The Ees/Ea ratio can also be simplified to a ratio of pressures42,44:
Based on the above-developed equations, RVEF can be derived from a RV pressure curve45:
Pressure-derived EF has been validated against MRI-derived EF, with Bland and Altman analysis showing no or minimal bias (a measure of accuracy) but relatively large limits of agreement (a measure of precision).46 Both RV volume- or pressure-derived estimations of Ees/Ea have been shown to provide reasonably accurate but with insufficient precision for individual assessments.37
Simplified measurements of the Ees/Ea ratio rest on incorrect assumption that the end-systolic PV relationship is a straight line crossing the origin.44 Thus, single point SV/ESV or EF may be dependent on the absolute level of P or V. Furthermore, assuming zero-crossing of PV neglects the unstressed volume of the RV, which may be of clinical relevance.32 Replacement of ESP by easier to measure mPAP in the pressure-derived equation has been proposed in some studies.26,44 However, as already mentioned, mPAP under-estimates PES in proportion to PH severity.16,35
5. The importance of volume measurements
The assessment of RV-PA coupling is easier in clinical practice using non-invasive imaging modalities. The measurements are preferably focused on RVEF because of its relevance to Ees/Ea and conceptual familiarity with EF measurements in left HF. Several studies have demonstrated that MRI-determined RVEF is an independent predictor of outcome in severe PH, with a cut-off value of 35–40% down from normal values around of 60–70%.47–50
An interesting alternative to cardiac magnetic resonance (CMR) is three-dimensional (3D) echocardiography. While RV volumes measured by 3D echocardiography tend to be slightly lower than those measured by MRI, derived RVEFs are within the same range and have been shown to be of prognostic relevance in patients with HF51 or with PAH.52 As 3D echocardiography is not yet widely available, two-dimensional (2D) echocardiography remains a useful alternative with reasonable validation of fractional area change (FAC) by high correlation coefficients against RVEF.53 The FAC has been shown to be predictive of outcome in PH, also with a rigorously determined cut-off value of ∼35%.54
Reversing increased RV dimensions or ‘reverse remodelling' by targeted therapies may restore PAH life expectancy to normal.55–58 Reverse RV remodelling with improved EF or FAC in severe PH is related to decreased PVR in a sigmoid fashion, with the steepest portion of the curve at a % decrease in PVR around 50%.55 Such a decrease in PVR in PAH requires the combination of at least two therapies targeting different pathways and may be more consistently achieved with one of those as parenteral prostanoids.55–57 Evidence is emerging that initial combination of three therapies including a parenteral prostanoid in PAH patients consistently improves functional class, exercise capacity, and survival in relation to a decrease in PVR by 60–70%,58–60 and reverses RV volumes to within the normal range.59 It may be that with the advent of drugs targeting additional signalling pathways, initial quadruple therapy of PAH will bring PVR back to normal or close and normalize RV function.61
6. Diastolic function
Diastolic function on a PV function loop is defined by an Eed curve. As such, it is a measure of stiffness. More complete definitions of diastolic function include the maximum rate of isovolumic pressure decline (dP/dtmin) and tau (τ), the time constant of isovolumic relaxation.
The Eed curve is more curvilinear than the Ees curve and therefore less adequately described by a linear approximation.10–12 Diastolic stiffness of the RV is best estimated fitting a non-linear exponential curve through the origin and early and late diastolic PV relationships, with the formula:
where α is a curve fitting constant, and β a diastolic stiffness constant.
The RV diastolic stiffness constant β is a function of ventricular volume and intrinsic changes in myocardial fibre elasticity in relation with titin phosphorylation62 and closely associated with disease severity in PH.63 Diastolic stiffness is a cause of increased filling pressures of the RV and backward flow with systemic organ damage.64
Diastolic stiffness simply quantified as a one-point end-diastolic P/V ratio retains the prediction capability of more complex β calculation.63 It has been suggested that diastolic stiffness may evolve independently of Ees and emerge as a stronger predictor of outcome.63 Increased diastolic stiffness is an early change in RV adaptation to increased afterload in experimental PH.65
Diastolic stiffness is positively related to the ventilatory response to exercise in severe PH66 and has been recently reported to be decreased during exercise by β-blocker therapy.67
More studies are needed to assess the clinical and prognostic relevance of different indices of RV diastolic function.
7. Ventricular interactions
RV function has also to be understood in the context of diastolic and systolic interactions with LV function.9,68
A negative diastolic ventricular interaction may occur in PH because of competition for space within the non-distensible pericardium. This alters LV filling and may eventually be the cause of altered LV systolic function and decreased cardiac output. RV volume overload has a more pronounced depressive effect on LV systolic function as assessed by EF than RV pressure overload. This may be explained by differences in systolic ventricular interaction, that is the inotropic effect of LV contraction on RV contraction.9,68
A positive systolic interaction can be shown experimentally by an improved RV function by an aortic constriction manoeuvre.69 In electrically isolated ventricular preparations, LV contraction contributes a significant amount (∼30%) to RV contraction.70 Systolic interaction is explained not only by a mechanical entrainment effect of the contraction of shared myocardial fibres but also by LV systolic function determining systemic blood pressure, which is essential to RV coronary perfusion.71 Increased RV filling pressures and excessive decrease in blood pressure may be a cause of RV ischaemia and decreased contractility.72
An important cause of negative ventricular interaction is regional and inter-ventricular asynchrony with post-systolic contraction or ‘shortening'.73 This develops in parallel to increased PAP and contributes to both altered RV systolic function and LV under-filling.73 Inter-ventricular asynchrony is associated with regional dyssynchrony of RV myocardial fibre contraction. Both RV-LV asynchrony and RV dyssynchrony can be identified and quantified by speckle tracking echocardiography and shown to be associated with a poor outcome in advanced PAH.74 However, inhomogeneous regional RV contraction may already be present in early stage or borderline PH75 or even in healthy subjects breathing low oxygen mixtures and mild increase in PAP.76
8. Stressing the RV-pulmonary circulation unit
Stressing the RV to measure its ‘contractile reserve' defined by systolic function adaptation to afterload may disclose latent failure. The RV may be stressed by an exercise test, fluid loading, or a pharmacologic challenge.
Exercise in normal subjects is associated with an increased Ees and unchanged Ea.77 In PAH patients with either normal or decreased Ees/Ea at rest, exercise is associated with a decrease in Ees/Ea.26,27 This is illustrated in Figure 2. Exercise-induced systolic RV pressure estimated from a tricuspid regurgitation has been shown to be a strong predictor of survival in patients with PAH or CTEPH.78 However, a single measurement of tricuspid regurgitation offers an only incomplete description of RV systolic function adaptation to afterload.26 The functional reserve of the RV is ideally measured by a stress-induced increase in Ees. It has been shown that a blunted Ees response to exercise in PH patients is associated with increased RV dimensions and decreased Ees/Ea or EF.79 In that study, exercise EF was predictive of outcome with a cut-off value of 37%. A measurement of EF does not require cardiac catheterization and may be adequate to assess RV functional reserve because it is related to Ees/Ea and integrates exercise-induced changes in Ees and RV volumes.79 Effects of exercise stress tests on RV PV loops with preserved or exhausted contractile reserve are illustrated in Figure 2.
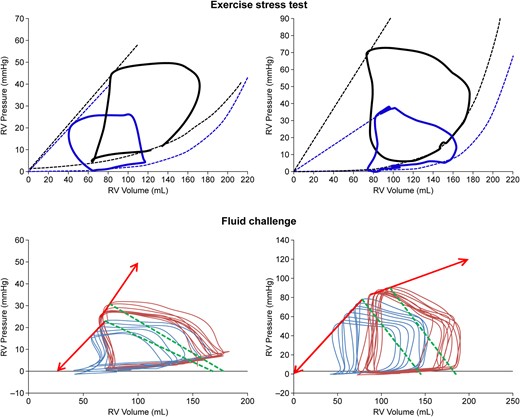
Figure 2
Upper range: single beat pressure–volume (PV) loops of the RV at rest (blue) and during exercise (black). Lower range: multiple beat PV loops before (blue) and after (red) a fluid challenge. Exercise was associated with either an unchanged Ees with an increase in ESV and EDV (left) or an increase in Ees and unchanged ESV with moderate increase in EDV (right). Exercise revealed a preserved contractile reserve to prevent increased RV volumes. Fluid loading was associated either with an increase in Ees with an increase in EDV more than ESV (left) or a decrease in Ees with increases in both ESV and EDV (right). Fluid loading revealed exhausted contractile reserve and pending RV failure.
There has been thought of replacing exercise by a low-dose dobutamine infusion and measuring the RV contractile response by the echocardiographic tricuspid annular plane systolic excursion (TAPSE) or tricuspid annulus S wave.80 There is experimental work showing that dobutamine-induced increase in these indices of RV systolic function reflects the resting state of RV-arterial coupling.81 A dobutamine stress test has also been applied with assessment of the RV contractile response by CMR imaging in congenital cardiac disease. In patients with a tetralogy of Fallot, absence of decreased ESV and increased RVEF in response to low-dose dobutamine has been shown to predict a poor outcome.82
A fluid challenge stresses the RV by a sole increase in venous return. In Starling's heart–lung preparation, an increase in venous return increased RV volumes and SV within a matter of several beats. However, after 3–5 min, EDV returned back to baseline, ESV decreased, and SV remained increased.8 Accordingly, in intact human beings, fluid loading may transiently increase EDV, but this is rapidly associated with an increase in Ees, so that Ees/Ea remains unchanged.10 In patients with severe PH, Ees fails to adapt, resulting in a decreased Ees/Ea.83 This is illustrated in Figure 2.
9. Pathobiology
The pathobiology of the RV response to loading involves changes in cardiomyocyte passive and active tension, a re-emergence of foetal gene expression with an increased expression of natriuretic peptides, a ‘proteomic switch' from α- to β-myosin heavy chain, a metabolic shift, cardiomyocyte hypertrophy and apoptosis, inflammatory cell infiltrates, fibrosis, loss of capillaries, and increased expressions of pro-inflammatory and apoptotic signalling pathways.84
In vitro mounted RV cardiomyocytes of idiopathic PAH or congenital cardiac shunt-associated PAH present with an increase in both active and passive tension–length relationships, in relation with decreased titin phosphorylation, and correlated to in vivo Ees and Eed measurements.62,85 In these studies, myocardial tissue stiffness is explained by a combination of fibrosis and increased myofibril elastance that is partially restored by protein kinase A.85 Cardiomyocyte force generating capacity is decreased in SSc-associated PAH compared to idiopathic PAH, in line with in vivo measurements of decreased contractile reserve,27 while diastolic stiffness is not different86 (Figure 3). This observation is in keeping with previous suggestion of a dissociation between systolic and diastolic elastances in PAH.62

Figure 3
Upper left: importance of BMPR2 mutation and sex in determining overload-induced RV fibrosis, inflammatory cell infiltration, sarcomeric cross-bridge interactions, and mitochondrial oxidation in PAH. Lower left: histopathological views of right atrium (RA) and RV in a PAH patient and a control subject with Masson's trichrome staining of fibrosis, wheat germ agglutinin staining of RV hypertrophy, and a CD31 staining demonstrating RV capillary rarefaction. Upper right: increased passive tension–length relationships of RV cardiomyocytes from patients with idiopathic PAH and SSc-PAH compared to controls. Lower right: active tension responses to a calcium challenge were increased in IPAH but decreased in SSc-PAH. The figure is generated with biorender.com, after references,62,86 with permission.
Evidence has been accumulated in experimental PH models that RV failure is associated with a metabolic remodelling characterized by a switch from oxidative phosphorylation (i.e. generation of energy from the mitochondrial-based fatty acid oxidation) to a glycolytic state (i.e. generation of energy from the cytoplasmic glycolysis).87 This metabolic switch has been confirmed in PAH patients along with increased sympathetic tone and insulin resistance and shown to be associated with a toxic lipid accumulation in the myocardium.88 These changes were most prominent in patients with loss of function bone morphogenetic protein receptor-2 (BMPR2) mutations, which is typically found in 80% of patients with heritable PAH.89,90 Mutations of BMPR2 have been reported to limit cardiac hypertrophy and to be linked to defective peroxisome proliferator-activated receptor-γ signalling as well as increased glycolysis, decreased oxidative phosphorylation, and impairment in fatty acid oxidation.89,90 Presently, it is unknown if interventions aiming at enhancing fatty acid oxidation or improving glucose oxidation might improve RV function.
Microneurographic studies have shown that the sympathetic nervous system is activated in PAH91 and that this is of prognostic relevance.92 A decreased parasympathetic activity in PAH is suggested by impaired post-exercise heart rate recovery and increased RV tissue α-7 nicotinic acetylcholine receptor together with a decreased acetyl-cholinesterase activity.93 This sympatho-vagal imbalance is associated with a down-regulation of RV myocardial β1 receptors though with an over-expression of β2 receptors94 affecting downstream β-adrenergic signalling and sarcomeric function.85 Furthermore, PAH patients also present with an activation of the renin-angiotensin-aldosterone system.95 However, interventional or pharmacological neurohumoral modulation strategies studies have not been shown to be effective until now in severe PH.96
Survival is shorter in male PAH patients, and this is explained by a better adaptation of RV function to afterload in women.36,97,98 These differences are essentially explained by differential effects of sex hormones,99 but Y chromosome determined BMPR2 signalling might also play a role.100 Clinical trials of estrogen therapies to prevent RV failure in PH are currently underway.
Patients with idiopathic pulmonary hypertension (IPAH) and a BMPR2 mutation have a worse prognosis than patients in whom this mutation is not found.101,102 Specific RV abnormalities in calcium handling and sarcomeric function are observed in BMPR2 mutated rats.103 The RV of BMPR2 mutated mice presents with altered hypertrophic responses and excessive fibrosis in response to pressure overload.89,104 Cardiac dysfunction in BMPR2-mutated patients and animals is associated with metabolic derangements and lipotoxicity.80,89
Inflammation is an inevitable component of the pathophysiology of RV failure on excessive loading,105 with over-expression of pro-inflammatory and pro-apoptotic mediators with recruitment of macrophages in acute as well as chronic RV failure models in proportion to RV-PA uncoupling.106,107 A recent study has demonstrated that the nucleotide binding domain, leucine-rich-containing family, pyrin-domain containing 3 (NLRP3) inflammasome in macrophages contributes to RV failure development.108 The NLRP3 was activated by exposure to damage-associated molecular patterns (DAMPs) resulting in the generation of pro-inflammatory cytokines such as interleukin 1β (IL1β). These findings suggest that RV failure is caused by a vicious circle starting with mitochondrial damage as source of DAMPs to trigger activation of NLRP3 inflammasome, resulting in secretion of pro-inflammatory cytokines such as IL1β and interleukin 6, causing recruitment of monocytes to the right heart.108
10. The RV in left HF
Left HF is by far the commonest cause of RV failure.109 Increased pulmonary artery wedge pressure (PAWP) because of increased LV filling pressures or mitral stenosis is initially transmitted upstream to PAP in a close to 1/1 ratio defining an isolated post-capillary pulmonary hypertension (IpcPH). Pulmonary vascular distension sooner or later triggers a remodelling of PA arterioles and capillaries thereby increasing the gradient between PAP and PAWP, defining a combined pre- and post-capillary pulmonary hypertension (CpcPH), formerly called ‘out of proportion' PH.110,111 The differential diagnosis between IpcPH and CpcPH therefore rests on increased gradients between PAP (mean or diastolic) and PAWP and thus normal or near-normal vs. increased PVR.109–111 Pulmonary hypertension may complicate HF with reduced LVEF (HFrEF) as well as with preserved LVEF (HFpEF).109–111 Both IpcPH and CpcPH are associated with a decreased survival rate in HF.111
Like in other types of PH, survival of either IpcPH or CpcPH is largely determined by RV function adaptation to increased loading.111 A preserved RV EF as measured with radionuclide technology has long been shown to predict exercise capacity and survival in advanced HF.112 Concomitant pulmonary vascular and RV function measurements in HF have shown RVEF to be an independent predictor of outcome independently of PH severity.113
The failing right heart in patients with HF gives rise to a ‘right heart phenotype', in which symptomatology of systemic congestion predominates.114,115 The RV and LV are encircled by common fibres and share the septum,9,68 which is why LV myocardial conditions affect RV systolic function adaptation to afterload. Experimental HFrEF is associated with profound RV-PA uncoupling and very low Ees/Ea in spite of marginally increased PAP.116 Systolic ventricular interactions in HFpEF would be expected to preserve RV function, but these patients also more commonly present with higher PAP on CpcPH, resulting in a disproportional impact of afterload to decrease RV Ees/Ea.111 This notion has been confirmed by invasive exercise measurements in HFpEF patients,117 with interestingly a better RV contractile adaptation to exercise-induced increase in pulmonary vascular pressures in women than in men.118
Because of the importance of the RV in HF, simple bedside non-invasive methods for evaluation of RV/PA coupling have been actively developed. The most successful is the ratio of TAPSE (to estimate Ees) to sPAP (to estimate afterload) measured during a standard 2D echocardiography.119 The TAPSE/sPAP ratio is correlated to invasively determined Ees/Ea120,121 though not always tightly111 and sometimes not better than TAPSE alone in HFrEF.122 Measurements of RVEF by 3D echocardiography and TAPSE/sPAP are illustrated in Figure 4.
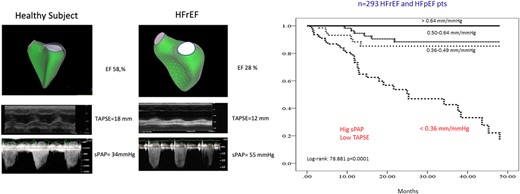
Figure 4
Measurements of RVEF by 3D echocardiography (upper panels, left), M-mode TAPSE (middle panels), and maximum velocity of tricuspid regurgitation to estimate sPAP (lower panels) are shown in a healthy subject and in a subject with HFrEF. The prognostic relevance of the TAPSE/sPAP ratio in a cohort of patients with either HFrEF or HF with preserved EF reduced or preserved LV EF is also shown (after reference,110 with permission).
The TAPSE/sPAP ratio has emerged as a strong predictor of outcome in PH on HF,110,111,119,122–126 PAH,127 or chronic lung conditions.128 The prognostic impact of the TAPSE/sPAP ratio on HF is illustrated in Figure 4. The TAPSE/sPAP ratio also predicts outcome of trans-catheter tricuspid,129 mitral,130 and aortic131 valve repairs. It is of interest that the TAPSE/sPAP ratio retains its strong prognostic relevance even when PAP is only mildly elevated in chronic obstructive pulmonary disease,132 mild uncomplicated systemic hypertension,133 or patients hospitalized with severe acute respiratory syndrome - Corona virus 2 (SARS-CoV2) pneumonia.134,135
Normal values of the TAPSE/sPAP ratio aggregate around 1.2 mm/mmHg, slightly higher by an average of 0.2 mm/mHg in men than in women and tend to decrease with aging.136,137 The TAPSE/sPAP ratio decreases with exercise by an average of 0.4 mm/mmHg.137,138 Rigorously determined prognostic cut-off values for the TAPSE/sPAP ratio vary from 0.31 in patients with severe PH127 to 0.64 mm/mmHg in SARS-CoV2 pneumonia with mild increase in PAP.134
Other echocardiographic indices of RV-PA coupling, such as the FAC/mPAP ratio, the RV area change/end-systolic area, the TAPSE/PA acceleration time, and the tricuspid S′ wave/sPAP, have attracted less attention than the TAPSE/sPAP ratio and, when evaluated, do not appear significantly correlated to Ees/Ea.121
There has been interest in assessing the TAPSE/sPAP ratio during an exercise stress test. This was first reported in patients who underwent cardiac resynchronization therapy.139 In these patients, the procedure did not affect the TAPSE/sPAP ratio at rest and was associated with a marked improvement of the TAPSE/sPAP ratio during exercise, in correlation to improved exercise capacity.139 Exercise TAPSE/sPAP better than resting TAPSE/sPAP may disciminate HFpEF from HF with mild reduction in LVEF.140
Table 1 provides an overview of indices of RV function, including imaging parameters which may serve as a ‘to do' list for those performing echocardiography or cardiac MRI.
Parameter
. | Modality
. | Advantages
. | Disadvantages
. |
---|
TAPSE | Echocardiography | Simple to assess and routinely available Measurement not limited by recognition/detection of the endocardial border High inter- and intra-observer reliability141 Prognostic relevance in combination with TR velocity in PAH142
| Solely a measure of longitudinal contraction of the basal free wall Load-dependent Angle-dependent Contribution of ventricular septum and RVOT missed (complex 3D structure of RV ignored) Regional abnormalities missed
|
TAPSE/PASP | Echocardiography | Invasively validated surrogate of RV-PA coupling in HFrEF and PAH121,122 Prognostic relevance in PAH, HFpEF, and HFrEF119,127 Simple to assess and routinely available
| Load-dependent Angle-dependent Contribution of ventricular septum and RVOT missed Regional abnormalities missed PASP not determinable in every patient
|
FAC | Echocardiography | Simple to assess and routinely available Compared with TAPSE and S′: Includes contribution of the free wall Correlates well with RV volumes
| |
RVEF (2D and 3D) | Echocardiography | Simple to assess and routinely available Strong correlation with MRI-based RVEF (r = 0.66–0.86 for 3D echocardiographic RVEF) with negligible bias when border tracking is manually edited143,144
| Uncertainties of RVOT assessment Inaccurate in volume-/pressure-overload states Volumes are load-dependent
|
SV/ESV | Echocardiography | | |
S′ | Echocardiography | Non-invasive, commonly used, established parameter Reproducible with good prognostic power141,147,148
| |
RIMP/Tei Index | Echocardiography | | |
Non-invasive pressure-volume loop-based Ees/Ea | Echocardiography | | Time-consuming Only offline assessment possible Only available in specialized centres Dependent on the TR velocity
|
RV GLS and strain rate | Echocardiography | Angle-independent No (significant) tethering Global and regional assessment of RV contractility Prognostic relevance (shown for RV free wall longitudinal strain/PASP ratio)149 Validated against gold standard150
| |
Eccentricity index | Echocardiography | | |
RV dyssynchrony | Echocardiography | | Only in narrow QRS Requires post-processing
|
SV/ESV | MRI | | |
dP/dt | Echocardiography, invasive (RHC) | | |
RVEF | MRI | | |
RV mass | MRI (and echocardiography) | | |
Cardiac index | Invasive (RHC) | | |
Ees | Invasive (conductance) | | Special (conductance) catheter required Expensive, time consuming, and complex to interpret
|
Ea | Invasive (conductance or RHC) | | |
Ees/Ea | Invasive (conductance) | | |
Parameter
. | Modality
. | Advantages
. | Disadvantages
. |
---|
TAPSE | Echocardiography | Simple to assess and routinely available Measurement not limited by recognition/detection of the endocardial border High inter- and intra-observer reliability141 Prognostic relevance in combination with TR velocity in PAH142
| Solely a measure of longitudinal contraction of the basal free wall Load-dependent Angle-dependent Contribution of ventricular septum and RVOT missed (complex 3D structure of RV ignored) Regional abnormalities missed
|
TAPSE/PASP | Echocardiography | Invasively validated surrogate of RV-PA coupling in HFrEF and PAH121,122 Prognostic relevance in PAH, HFpEF, and HFrEF119,127 Simple to assess and routinely available
| Load-dependent Angle-dependent Contribution of ventricular septum and RVOT missed Regional abnormalities missed PASP not determinable in every patient
|
FAC | Echocardiography | Simple to assess and routinely available Compared with TAPSE and S′: Includes contribution of the free wall Correlates well with RV volumes
| |
RVEF (2D and 3D) | Echocardiography | Simple to assess and routinely available Strong correlation with MRI-based RVEF (r = 0.66–0.86 for 3D echocardiographic RVEF) with negligible bias when border tracking is manually edited143,144
| Uncertainties of RVOT assessment Inaccurate in volume-/pressure-overload states Volumes are load-dependent
|
SV/ESV | Echocardiography | | |
S′ | Echocardiography | Non-invasive, commonly used, established parameter Reproducible with good prognostic power141,147,148
| |
RIMP/Tei Index | Echocardiography | | |
Non-invasive pressure-volume loop-based Ees/Ea | Echocardiography | | Time-consuming Only offline assessment possible Only available in specialized centres Dependent on the TR velocity
|
RV GLS and strain rate | Echocardiography | Angle-independent No (significant) tethering Global and regional assessment of RV contractility Prognostic relevance (shown for RV free wall longitudinal strain/PASP ratio)149 Validated against gold standard150
| |
Eccentricity index | Echocardiography | | |
RV dyssynchrony | Echocardiography | | Only in narrow QRS Requires post-processing
|
SV/ESV | MRI | | |
dP/dt | Echocardiography, invasive (RHC) | | |
RVEF | MRI | | |
RV mass | MRI (and echocardiography) | | |
Cardiac index | Invasive (RHC) | | |
Ees | Invasive (conductance) | | Special (conductance) catheter required Expensive, time consuming, and complex to interpret
|
Ea | Invasive (conductance or RHC) | | |
Ees/Ea | Invasive (conductance) | | |
Parameter
. | Modality
. | Advantages
. | Disadvantages
. |
---|
TAPSE | Echocardiography | Simple to assess and routinely available Measurement not limited by recognition/detection of the endocardial border High inter- and intra-observer reliability141 Prognostic relevance in combination with TR velocity in PAH142
| Solely a measure of longitudinal contraction of the basal free wall Load-dependent Angle-dependent Contribution of ventricular septum and RVOT missed (complex 3D structure of RV ignored) Regional abnormalities missed
|
TAPSE/PASP | Echocardiography | Invasively validated surrogate of RV-PA coupling in HFrEF and PAH121,122 Prognostic relevance in PAH, HFpEF, and HFrEF119,127 Simple to assess and routinely available
| Load-dependent Angle-dependent Contribution of ventricular septum and RVOT missed Regional abnormalities missed PASP not determinable in every patient
|
FAC | Echocardiography | Simple to assess and routinely available Compared with TAPSE and S′: Includes contribution of the free wall Correlates well with RV volumes
| |
RVEF (2D and 3D) | Echocardiography | Simple to assess and routinely available Strong correlation with MRI-based RVEF (r = 0.66–0.86 for 3D echocardiographic RVEF) with negligible bias when border tracking is manually edited143,144
| Uncertainties of RVOT assessment Inaccurate in volume-/pressure-overload states Volumes are load-dependent
|
SV/ESV | Echocardiography | | |
S′ | Echocardiography | Non-invasive, commonly used, established parameter Reproducible with good prognostic power141,147,148
| |
RIMP/Tei Index | Echocardiography | | |
Non-invasive pressure-volume loop-based Ees/Ea | Echocardiography | | Time-consuming Only offline assessment possible Only available in specialized centres Dependent on the TR velocity
|
RV GLS and strain rate | Echocardiography | Angle-independent No (significant) tethering Global and regional assessment of RV contractility Prognostic relevance (shown for RV free wall longitudinal strain/PASP ratio)149 Validated against gold standard150
| |
Eccentricity index | Echocardiography | | |
RV dyssynchrony | Echocardiography | | Only in narrow QRS Requires post-processing
|
SV/ESV | MRI | | |
dP/dt | Echocardiography, invasive (RHC) | | |
RVEF | MRI | | |
RV mass | MRI (and echocardiography) | | |
Cardiac index | Invasive (RHC) | | |
Ees | Invasive (conductance) | | Special (conductance) catheter required Expensive, time consuming, and complex to interpret
|
Ea | Invasive (conductance or RHC) | | |
Ees/Ea | Invasive (conductance) | | |
Parameter
. | Modality
. | Advantages
. | Disadvantages
. |
---|
TAPSE | Echocardiography | Simple to assess and routinely available Measurement not limited by recognition/detection of the endocardial border High inter- and intra-observer reliability141 Prognostic relevance in combination with TR velocity in PAH142
| Solely a measure of longitudinal contraction of the basal free wall Load-dependent Angle-dependent Contribution of ventricular septum and RVOT missed (complex 3D structure of RV ignored) Regional abnormalities missed
|
TAPSE/PASP | Echocardiography | Invasively validated surrogate of RV-PA coupling in HFrEF and PAH121,122 Prognostic relevance in PAH, HFpEF, and HFrEF119,127 Simple to assess and routinely available
| Load-dependent Angle-dependent Contribution of ventricular septum and RVOT missed Regional abnormalities missed PASP not determinable in every patient
|
FAC | Echocardiography | Simple to assess and routinely available Compared with TAPSE and S′: Includes contribution of the free wall Correlates well with RV volumes
| |
RVEF (2D and 3D) | Echocardiography | Simple to assess and routinely available Strong correlation with MRI-based RVEF (r = 0.66–0.86 for 3D echocardiographic RVEF) with negligible bias when border tracking is manually edited143,144
| Uncertainties of RVOT assessment Inaccurate in volume-/pressure-overload states Volumes are load-dependent
|
SV/ESV | Echocardiography | | |
S′ | Echocardiography | Non-invasive, commonly used, established parameter Reproducible with good prognostic power141,147,148
| |
RIMP/Tei Index | Echocardiography | | |
Non-invasive pressure-volume loop-based Ees/Ea | Echocardiography | | Time-consuming Only offline assessment possible Only available in specialized centres Dependent on the TR velocity
|
RV GLS and strain rate | Echocardiography | Angle-independent No (significant) tethering Global and regional assessment of RV contractility Prognostic relevance (shown for RV free wall longitudinal strain/PASP ratio)149 Validated against gold standard150
| |
Eccentricity index | Echocardiography | | |
RV dyssynchrony | Echocardiography | | Only in narrow QRS Requires post-processing
|
SV/ESV | MRI | | |
dP/dt | Echocardiography, invasive (RHC) | | |
RVEF | MRI | | |
RV mass | MRI (and echocardiography) | | |
Cardiac index | Invasive (RHC) | | |
Ees | Invasive (conductance) | | Special (conductance) catheter required Expensive, time consuming, and complex to interpret
|
Ea | Invasive (conductance or RHC) | | |
Ees/Ea | Invasive (conductance) | | |
11. RV cardiomyopathies
RV failure may result from direct myocardial insults and/or diseases, even though increased afterloading will at some point occur because of decreased inotropy and heterometric increase in volume.10
Perhaps, the commonest cause of initial RV cardiomyopathy is ischaemia. Post-mortem examinations156 as well as imaging studies157,158 have identified RV necrotic, inflammatory, and oedematous lesions in up to 50% of patients with an acute myocardial infarction. Ischaemic RV cardiomyopathy is more common in inferior infarcts but is also present in a substantial proportion of anterior infarcts.157 Reported prevalences of decreased RVEF after an acute myocardial infarction range from 20 to 60%.159,160 However, post-infarction RV dysfunction will most often result from a combination of factors including ischaemia-related decrease in contractility, negative ventricular interactions, mitral regurgitation, and increased pulmonary vascular pressures and is not therefore a good example of initial isolated RV cardiomyopathy with secondary volume overload.160
Non-ischaemic dilated cardiomyopathy is also associated with decreased RVEF, in one-third to half of the patients, but this is explained by negative ventricular interactions and increased loading in addition to decreased inotropy.161 In hypertrophic cardiomyopathy, CMR studies show a prognostically relevant RV involvement in approximately one-third of the patients.162,163 Even when standard indices of systolic function are normal, RV free wall deformation, diastolic function, and contractile reserve in response to exercise are reduced in hypertrophic cardiomyopathy.164
In patients with cardiac amyloidosis, abnormalities in both RV systolic and diastolic function are observed in relation to amyloid deposition but also severity of LV involvement.165
Acute myocarditis involves the RV in approximately one-fifth of the patients and is then associated with RV dilatation, hypertrophy, decreased EF, and impaired outcome.166 Altered echocardiographic indices of RV-PA coupling have been reported in COVID-19 patients with no apparent alteration of LV function.134,135
Systemic diseases such as sarcoidosis167 or SSc27,86 may be associated with altered RV function in the absence of overt PH.
A rare but more specifically RV myocardial disease is arrhythmogenic cardiomyopathy.168 These patients present with a general pattern of progression from a subclinical phase, an electrical phase, and a final structural phase with RV regional or global dilatation and systolic dysfunction.169 However, there is a lot of overlap and individual variability, as imaging in early stage disease may disclose prognostically relevant abnormal myocardial deformation170 and increased RV dimensions and dyssynchrony.171 A clinical diagnosis of HF predominantly right sided is made in up to 50% of the patients at time of diagnosis.172 Gold standard RV-PA coupling measurements have not been reported in arrhythmogenic cardiomyopathy and are therefore of undefined prognostic relevance.10
12. Conclusions
Recent years have witnessed an improved understanding of the RV in health and disease, with application of rigorous invasive determinations for basic research questions and validation of non-invasive imaging to assess clinical relevance. More clinical research is needed to define optimal RV stress test conditions, but tools are now sufficiently validated for more in depth exploring of the pathobiology of RV failure and testing of therapeutic interventions.
Funding
This study was supported by German Research Foundation, SFB 1213, Project B08.
References
1Starr
I
, Jeffers
WA
, Meade
RH
Jr.
The absence of conspicuous increments of venous pressure after severe damage to the right ventricle of the dog, with a discussion of the relation between clinical congestive failure and heart disease
.
Am Heart J
1943
;
26
:
291
–
301
.
2Fontan
F
, Baudet
F
.
Surgical repair of tricuspid atresia
.
Thorax
1971
;
26
:
240
–
248
.
3Gewillig
M
.
The Fontan circulation
.
Heart
2005
;
91
:
839
–
846
.
4Paridon
SM
, Mitchell
PD
, Colan
SD
, Williams
RV
, Blaufox
A
, Li
JS
, Margossian
R
, Mital
S
, Russell
J
, Rhodes
J
;
Pediatric Heart Network Investigators
.
A cross-sectional study of exercise performance during the first 2 decades of life after the Fontan operation
.
J Am Coll Cardiol
2008
;
52
:
99
–
107
.
5West
JB
.
The role of the fragility of the pulmonary blood-gas barrier in the evolution of the pulmonary circulation
.
Am J Physiol Regul Integr Comp Physiol
2013
;
304
:
R171
–
R176
.
6Guyton
AC
, Lindsey
AW
, Gilluly
JJ
.
The limits of right ventricular compensation following acute increase in pulmonary circulatory resistance
.
Circ Res
1054
;
2
:
326
–
332
.
7Patterson
SW
, Piper
H
, Starling
EH
.
The regulation of the heartbeat
.
J Physiol
1914
;
48
:
465
–
513
.
8Rosenblueth
A
, Alanis
J
, Lopez
E
, Rubio
R
.
The adaptation of ventricular muscle to different circulatory conditions
.
Arch Int Physiol Biochim
1959
;
67
:
358
–
373
.
9Friedberg
MK
, Redington
AN
.
Right versus left ventricular failure. Differences, similarities and interactions
.
Circulation
2014
;
129
:
1033
–
1044
.
10Sanz
J
, Sanchez-Quintana
D
, Bossone
E
, Bogaard
HJ
, Naeije
R
.
Anatomy, function, and dysfunction of the right ventricle: JACC state-of-the-art review
.
J Am Coll Cardiol
2019
;
73
:
1463
–
1482
.
11Vonk Noordegraaf
A
, Chin
KM
, Haddad
F
, Hassoun
PM
, Hemnes
AR
, Hopkins
SR
, Kawut
SM
, Langleben
D
, Lumens
J
, Naeije
R
.
Pathophysiology of the right ventricle and of the pulmonary circulation in pulmonary hypertension: an update
.
Eur Respir J
2019
;
53
:.
12Lahm
T
, Douglas
IS
, Archer
SL
, Bogaard
HJ
, Chesler
NC
, Haddad
F
, Hemnes
AR
, Kawut
SM
, Kline
JA
, Kolb
TM
, Mathai
SC
, Mercier
O
, Michelakis
ED
, Naeije
R
, Tuder
RM
, Ventetuolo
CE
, Vieillard-Baron
A
, Voelkel
NF
, Vonk-Noordegraaf
A
, Hassoun
PM
; American Thoracic Society Assembly on Pulmonary Circulation
.
Assessment of right ventricular function in the research setting: knowledge gaps and pathways forward. An official American Thoracic Society Research statement
.
Am J Respir Crit Care Med
2018
;
198
:
e15
–
e43
.
13Saouti
N
, Westerhof
N
, Helderman
F
, Marcus
JT
, Boonstra
A
, Postmus
PE
, Vonk-Noordegraaf
A
.
Right ventricular oscillatory power is a constant fraction of total power irrespective of pulmonary artery pressure
.
Am J Respir Crit Care Med
2010
;
182
:
1315
–
1320
.
14Chemla
D
, Lau
EMT
, Papelier
Y
, Attal
P
, Hervé
P
.
Pulmonary vascular resistance and compliance relationship in pulmonary hypertension
.
Eur Respir J
2016
;
46
:
1178
–
1189
.
15Kussmaul
WG
, Noordergraaf
A
, Laskey
WK
.
Right ventricular-pulmonary arterial interactions
.
Ann Biomed Eng
1992
;
20
:
63
–
80
.
16Tello
K
, Richter
MJ
, Axmann
J
, Buhmann
M
, Seeger
W
, Naeije
R
, Ghofrani
HA
, Gall
H
.
More on single beat estimation of right ventriculo-arterial coupling in pulmonary arterial hypertension
.
Am J Respir Crit Care Med
2018
;
198
:
816
–
818
.
17Suga
H
, Sagawa
K
, Shoukas
AA
.
Load independence of the instantaneous pressure-volume ratio of the canine left ventricle and effects of epinephrine and heart rate on the ratio
.
Circ Res
1973
;
32
:
314
–
322
.
18Maughan
WL
, Shoukas
AA
, Sagawa
K
, Weisfeldt
ML
.
Instantaneous pressure-volume relationship of the canine right ventricle
.
Circ Res
1979
;
44
:
309
–
315
.
19Sunagawa
K
, Yamada
A
, Senda
Y
, Kikuchi
Y
, Nakamura
M
, Shibahara
T
.
Estimation of the hydromotive source pressure from ejecting beats of the left ventricle
.
IEEE Trans Biomed Eng
1980
;
57
:
299
–
305
.
20Brimioulle
S
, Wauthy
P
, Ewalenko
P
, Rondelet
B
, Vermeulen
F
, Kerbaul
F
, Naeije
R
.
Single-beat estimation of right ventricular end-systolic pressure-volume relationship
.
Am J Physiol Heart Circ Physiol
2003
;
284
:
H1625
–
H1630
.
21Tello
K
, Dalmer
A
, Axmann
J
, Vanderpool
R
, Ghofrani
HA
, Naeije
R
, Roller
F
, Seeger
W
, Sommer
N
, Wilhelm
J
, Gall
H
, Richter
MJ
.
Reserve of right ventricular-arterial coupling in the setting of chronic overload
.
Circ Heart Fail
2019
;
12
:.
22Kuehne
T
, Yilmaz
S
, Steendijk PMoore
P
, Groenink
M
, Saaed
M
, Weber
O
, Higgins
CB
, Ewert
P
, Fleck
E
, Nagel
E
, Schulze-Neick
I
, Lange
P
.
Magnetic resonance imaging analysis of right ventricular pressure-volume loops: in vivo validation and clinical application in patients with pulmonary hypertension
.
Circulation
2004
;
110
:
2010
–
2016
.
23Wauthy
P
, Naeije
R
, Brimioulle
S
.
Left and right ventriculo-arterial coupling in a patient with congenitally corrected transposition
.
Cardiol Young
2005
;
15
:
647
–
649
.
24Tedford
RJ
, Mudd
JO
, Girgis
RE
, Mathai
SC
, Zaiman
AL
, Housten-Harris
T
, Boyce
D
, Kelemen
BW
, Bacher
AC
, Shah
AA
, Hummers
LK
, Wigley
FM
, Russell
SD
, Saggar
R
, Saggar
R
, Maughan
WL
, Hassoun
PM
, Kass
DA
.
Right ventricular dysfunction in systemic sclerosis associated pulmonary arterial hypertension
.
Circ Heart Fail
2013
;
6
:
953
–
963
.
25McCabe
C
, White
PA
, Hoole
SP
, Axell
RG
, Priest
AN
, Gopalan
D
, Taboada
D
, MacKenzie Ross
R
, Morrell
NW
, Shapiro
LM
, Pepke-Zaba
J
.
Right ventricular dysfunction in chronic thromboembolic obstruction of the pulmonary artery: a pressure-volume study using the conductance catheter
.
J Appl Physiol
2014
;
116
:
355
–
363
.
26Spruijt
OA
, de Man
FS
, Groepenhoff
H
, Oosterveer
F
, Westerhof
N
, Vonk-Noordegraaf
A
, Bogaard
HJ
.
The effects of exercise on right ventricular contractility and right ventricular-arterial coupling in pulmonary hypertension
.
Am J Respir Crit Care Med
2015
;
191
:
1050
–
1057
.
27Hsu
S
, Houston
BA
, Tampakakis
E
, Bacher
AC
, Rhodes
PS
, Mathai
SC
, Damico
RL
, Kolb
TM
, Hummers
LK
, Shah
AA
, McMahan
Z
, Corona-Villalobos
CP
, Zimmerman
SL
, Wigley
FM
, Hassoun
PM
, Kass
DA
, Tedford
RJ
.
Right ventricular functional reserve in pulmonary arterial hypertension
.
Circulation
2016
;
133
:
2413
–
2422
.
28Axell
RG
, Messer
SJ
, White
PA
, McCabe
C
, Priest
A
, Statopoulou
T
, Drozdzynska
M
, Viscasillas
J
, Hinchy
EC
, Hampton-Till
J
, Alibhai
HI
, Morrell
N
, Pepke-Zaba
J
, Large
SR
, Hoole
SP
.
Ventriculo-arterial coupling detects occult RV dysfunction in chronic thromboembolic pulmonary vascular disease
.
Physiol Rep
2017
;
5
(
7
):
e13227
.
29Ghuysen
A
, Lambermont
B
, Kolh
P
, Tchana-Sato
V
, Magis
D
, Gerard
P
, Mommens
V
, Janssen
N
, Desaive
T
, D'Orio
V
.
Alteration of right ventricular-pulmonary vascular coupling in a porcine model of progressive pressure overloading
.
Shock
2008
;
29
:
197
–
204
.
30Fourie
PR
, Coetzee
AR
, Bolliger
CT
.
Pulmonary artery compliance: its role in right ventricular-arterial coupling
.
Cardiovasc Res
1992
;
26
:
839
–
834
.
31Rex
S
, Missant
C
, Segers
P
, Rossaint
R
, Wouters
PF
.
Epoprostenol treatment of acute pulmonary hypertension is associated with a paradoxical decrease in right ventricular contractility
.
Intens Care Med
2008
;
34
:
179
–
189
.
32Vanderpool
RR
, Desai
AA
, Knapp
SM
, Simon
MA
, Abidov
A
, Yuan
JXJ
, Garcia
JGN
, Hansen
LM
, Knoper
SR
, Naeije
R
, Rischard
FP
.
How prostacyclin improves right ventricular function in pulmonary arterial hypertension
.
Eur Respir J
2017
;
50
(
2
):
1700764
.
33Kass
DA
, Maughan
WL
.
From ‘Emax' to pressure-volume relations: a broader view
.
Circulation
1988
;
77
:
1203
–
1212
.
34Redington
AN
, Rigby
RL
, Shinebourne
EA
, Oldershaw
PJ
.
Changes in pressure-volume relation of the right ventricle when its loading conditions are modified
.
Br Heart J
1990
;
63
:
45
–
49
.
35Richter
MJ
, Hsu
S
, Yogeswaran
A
, Husain-Syed
F
, Vadász
I
, Ghofrani
HA
, Naeije
R
, Harth
S
, Grimminger
F
, Seeger
W
, Gall
H
, Tedford
RJ
, Tello
K
.
Right ventricular pressure-volume loop shape and systolic pressure change in pulmonary hypertension
.
Am J Physiol Lung Cell Mol Physiol
2021
;
320
:
L715
–
L725
.
36Tello
K
, Richter
MJ
, Yogeswaran
A
, Ghofrani
HA
, Naeije
R
, Vanderpool
R
, Gall
H
, Tedford
RJ
, Seeger
W
, Lahm
T
.
Sex differences in right ventriculo-arterial coupling in pulmonary arterial hypertension
.
Am J Respir Crit Care Med
2020
;
202
:
1042
–
1046
.
37Richter
MJ
, Peters
D
, Ghofrani
HA
, Naeije
R
, Roller
F
, Sommer
N
, Gall
H
, Grimminger
F
, Seeger
W
, Tello
K
.
Evaluation and prognostic relevance of right ventricular-arterial coupling in pulmonary hypertension
.
Am J Respir Crit Care
2020
;
201
:
116
–
119
.
38Hsu
S
, Simpson
CE
, Houston
BA
, Wand
A
, Sato
T
, Kolb
TM
, Mathai
SC
, Kass
DA
, Hassoun
PM
, Damico
RL
, Tedford
RJ
.
Multi-beat right ventricular-arterial coupling predicts clinical worsening in pulmonary arterial hypertension
.
J Am Heart Assoc
2020
;
9
:
e016031
.
39Brener
MI
, Masoumi
A
, Ng
VG
, Tello
K
, Bastos
MB
, Cornwell
WK
, Hsu
S
, Tedford
RJ
, Lurz
P
, Rommel
KP
, Kresoja
KP
, Nagueh
SF
, Kanwar
MK
, Kapur
NK
, Hiremath
G
, Sarraf
M
, Van Den Enden
AJM
, Van Mieghem
NM
, Heerdt
PM
, Hahn
RT
, Kodali
SK
, Sayer
GT
, Uriel
N
, Burkhoff
D
.
Invasive right ventricular pressure-volume analysis: basic principles, clinical applications and practical recommendations
.
Circ Heart Fail
2022
;
15
:
e009101
.
40Sanz
J
, García-Alvarez
A
, Fernández-Friera
L
, Nair
A
, Mirelis
JG
, Sawit
ST
, Pinney
S
, Fuster
V
.
Right ventriculo-arterial coupling in pulmonary hypertension: a magnetic resonance study
.
Heart
2012
;
98
:
238
–
243
.
41Vanderpool
RR
, Rischard
F
, Naeije
R
, Hunter
K
, Simon
MA
.
Simple functional imaging of the right ventricle in pulmonary hypertension: can right ventricular ejection fraction be improved?
Int J Cardiol
2016
;
223
:
93
–
94
.
42Vanderpool
RR
, Pinsky
MR
, Naeije
R
, Deible
C
, Kosaraju
V
, Bunner
C
, Mathier
MA
, Lacomis
J
, Champion
HC
, Simon
MA
.
RV-pulmonary arterial coupling predicts outcome in patients referred for pulmonary hypertension
.
Heart
2015
;
101
:
37
–
43
.
43Brewis
MJ
, Bellofiore
A
, Vanderpool
RR
, Chesler
NC
, Johnson
MK
, Naeije
R
, Peacock
AJL
.
Imaging right ventricular function to predict outcome in pulmonary arterial hypertension
.
Int J Cardiol
2016
;
218
:
206
–
211
.
44Trip
P
, Kind
T
, van de Veerdonk
MC
, Marcus
JT
, de Man
FS
, Westerhof
N
, Vonk Noordegraaf
A
.
Accurate assessment of load-independent right ventricular systolic function in patients with pulmonary hypertension
.
J Heart Lung Transplant
2013
;
32
:
50
–
55
.
45Heerdt
PM
, Kheyfets
V
, Charania
S
, Elassal
A
, Singh
I
.
A pressure based single beat method for estimation of right ventricular ejection fraction: proof of concept
.
Eur Respir J
2020
;
55
:
1901635
.
46Heerdt
PM
, Singh
I
, Elassal
A
, Kheyfets
V
, Richter
MJ
, Tello
K
.
Pressure-based estimation of right ventricular ejection fraction
.
ESC Heart Fail
2022
;
9
:
1436
–
1443
.
47Freed
BH
, Gomberg-Maitland
M
, Chandra
S
, Mor-Avi
V
, Rich
S
, Archer
SL
, Jr JE, Lang
RM
, Patel
AR
.
Late gadolinium enhancement cardiovascular magnetic resonance predicts clinical worsening in patients with pulmonary hypertension
.
J Cardiovasc Magn Reson
2012
;
14
:
11
.
48van de Veerdonk
MC
, Kind
T
, Marcus
JT
, Mauritz
GJ
, Heymans
MW
, Bogaard
HJ
, Boonstra
A
, Marques
KM
, Westerhof
N
, Vonk-Noordegraaf
A
.
Progressive right ventricular dysfunction in patients with pulmonary arterial hypertension responding to therapy
.
J Am Coll Cardiol
2011
;
58
:
2511
–
2519
.
49Lewis
RA
, Johns
CS
, Cogliano
M
, Capener
D
, Tubman
E
, Elliot
CA
, Charalampopoulos
A
, Sabroe
I
, Thompson
AAR
, Billings
CG
, Hamilton
N
, Baster
K
, Laud
PJ
, Hickey
PM
, Middleton
J
, Armstrong
IJ
, Hurdman
JA
, Lawrie
A
, Rothman
AMK
, Wild
JM
, Condliffe
R
, Swift
AJ
, Kiely
DG
.
Identification of cardiac magnetic resonance imaging thresholds for risk stratification in pulmonary arterial hypertension
.
Am J Respir Crit Care Med
2020
;
201
:
458
–
468
.
50Alabed
S
, Shahin
Y
, Garg
P
, Alandejani
F
, Johns
CS
, Lewis
RA
, Condliffe
R
, Wild
JM
, Kiely
DG
, Swift
AJ
.
Cardiac-MRI predicts clinical worsening and mortality in pulmonary arterial hypertension: a systematic review and meta-analysis
.
JACC Cardiovasc Imaging
2020
;
14
:
931
–
942
.
51Nagata
Y
, Wu
VCC
, Kado
Y
, Otani
K
, Lin
FC
, Otsuji
Y
, Negishi
K
, Takeushi
M
.
Prognostic value of right ventricular ejection fraction assessed by transthoracic 3D echocardiography
.
Circ Cardiovasc Imaging
2017
;
10
(
2
):
e005384
.
52Liu
BY
, Wu
WC
, Zeng
QX
, Liu
ZH
, Niu
LL
, Tian
Y
, Luo
Q
, Zhao
ZH
, Quan
RL
, Lin
JR
, Wang
H
, He
JG
, Xiong
CM
.
The value of three-dimensional echocardiography in risk stratification in pulmonary arterial hypertension
.
Int J Cardiovasc Imaging
2020
;
36
:
577
–
584
.
53Shiran
H
, Zamanian
RT
, McConnell
MV
, Liang
DH
, Dash
R
, Heidary
S
, Sudini
NL
, Wu
JC
, Haddad
F
, Yang
PC
.
Relationship between echocardiographic and magnetic resonance derived measures of right ventricular size and function in patients with pulmonary hypertension
.
J Am Soc Echocardiogr
2014
;
27
:
405
–
412
.
54Badagliacca
R
, Papa
S
, Valli
G
, Pezzuto
B
, Poscia
R
, Manzi
G
, Giannetta
E
, Sciomer
S
, Palange
P
, Naeije
R
, Fedele
F
, Vizza
CD
.
Echocardiography combined with cardiopulmonary exercise testing for the prediction of outcome in idiopathic pulmonary arterial hypertension
.
Chest
2016
;
150
:
1313
–
1322
.
55Badagliacca
R
, Papa
S
, Manzi
G
, Miotti
C
, Luongo
F
, Sciomer
S
, Cedrone
N
, Fedele
F
, Naeije
R
, Vizza
CD
.
Usefulness of adding echocardiography of the right heart to risk-assessment scores in prostanoid-treated pulmonary arterial hypertension
.
JACC Cardiovasc Imaging
2020
;
13
:
2054
–
2056
.
56van de Veerdonk
MC
, Huis in t Veld
AE
, Marcus
JT
, Westerhof
N
, Heymans
MW
, Bogaard
HJ
, Vonk-Noordegraaf
A
.
Upfront combination therapy reduces right ventricular volumes in pulmonary arterial hypertension
.
Eur Respir J
2017
;
49
(
6
):.
57Badagliacca
R
, Raina
A
, Ghio
S
, D'Alto
M
, Confalonieri
M
, Correale
M
, Corda
M
, Paciocco
G
, Lombardi
C
, Mulè
M
, Poscia
R
, Scelsi
L
, Argiento
P
, Sciomer
S
, Benza
RL
, Vizza
CD
.
Influence of various therapeutic strategies on right ventricular morphology, function and hemodynamics in pulmonary arterial hypertension
.
J Heart Lung Transplant
2018
;
37
:
365
–
375
.
58Sitbon
O
, Jaïs
X
, Savale
L
, Cottin
V
, Bergot
E
, Macari
EA
, Bouvaist
H
, Dauphin
C
, Picard
F
, Bulifon
S
, Montani
D
, Humbert
M
, Simonneau
G
.
Upfront triple combination therapy in pulmonary arterial hypertension: a pilot study
.
Eur Respir J
2014
;
43
:
1691
–
1697
.
59D'Alto
M
, Badagliacca
R
, Argiento
P
, Romeo
E
, Farro
A
, Papa
S
, Sarubbi
B
, Russo
MG
, Vizza
CD
, Golino
P
, Naeije
R
.
Risk reduction and right heart reverse remodeling by upfront triple combination therapy in pulmonary arterial hypertension
.
Chest
2020
;
157
:
376
–
383
.
60Boucly
A
, Savale
L
, Jaïs
X
, Bauer
F
, Bergot
E
, Bertoletti
L
, Beurnier
A
, Bourdin
A
, Bouvaist
H
, Bulifon
S
, Chabanne
C
, Chaouat
A
, Cottin
V
, Dauphin
C
, Degano
B
, De Groote
P
, Favrolt
N
, Feng
Y
, Horeau-Langlard
D
, Jevnikar
M
, Jutant
EM
, Liang
Z
, Magro
P
, Mauran
P
, Moceri
P
, Mornex
JF
, Palat
S
, Parent
F
, Picard
F
, Pichon
J
, Poubeau
P
, Prévot
G
, Renard
S
, Reynaud-Gaubert
M
, Riou
M
, Roblot
P
, Sanchez
O
, Seferian
A
, Tromeur
C
, Weatherald
J
, Simonneau
G
, Montani
D
, Humbert
M
, Sitbon
O
.
Association between initial treatment strategy and long-term survival in pulmonary arterial hypertension
.
Am J Respir Crit Care Med
2021
;
204
:
842
–
854
.
61Naeije
R
, Rubin
LJ
.
Sotatercept in pulmonary arterial hypertension: something old, something new
.
Eur Respir J
2023
;
61
(
1
):
2201972
.
62Rain
S
, Handoko
ML
, Trip
P
, Gan
CT
, Westerhof
N
, Stienen
GJ
, Paulus
WJ
, Ottenheijm
CA
, Marcus
JT
, Dorfmüller
P
, Guignabert
C
, Humbert
M
, Macdonald
P
, Dos Remedios
C
, Postmus
PE
, Saripalli
C
, Hidalgo
CG
, Granzier
HL
, Vonk-Noordegraaf
A
, van der Velden
J
, de Man
FS
.
Right ventricular diastolic impairment in patients with pulmonary arterial hypertension
.
Circulation
2013
;
128
:
2016
–
2025
.
63Trip
P
, Rain
S
, Handoko
ML
, van der Bruggen
C
, Bogaard
HJ
, Marcus
JT
, Boonstra
A
, Westerhof
N
, Vonk-Noordegraaf
A
, de Man
FS
.
Clinical relevance of right ventricular diastolic stiffness in pulmonary hypertension
.
Eur Respir J
2015
;
45
:
1603
–
1612
.
64Marcus
JT
, Westerhof
BE
, Groeneveldt
JA
, Bogaard
HJ
, de Man
FS
, Vonk Noordegraaf
A
.
Vena cava backflow and right ventricular stiffness in pulmonary arterial hypertension
.
Eur Respir J
2019
;
54
:.
65Alaa
M
, Abdellatif
M
, Tavares-Silva
M
, Oliveira-Pinto
J
, Lopes
L
, Leite
S
, Leite-Moreira
AF
, Lourenço
AP
.
Right ventricular end-diastolic stiffness heralds right ventricular failure in monocrotaline-induced pulmonary hypertension
.
Am J Physiol Heart Circ Physiol
2016
;
311
:
H1004
–
H1013
.
66Tello
K
, Dalmer
A
, Vanderpool
R
, Ghofrani
HA
, Naeije
R
, Roller
F
, Seeger
W
, Dumitrescu
D
, Sommer
N
, Brunst
A
, Gall
H
, Richter
MJ
.
Impaired lusitropy is associated with ventilatory inefficiency in pulmonary arterial hypertension
.
Eur Respir J
2019
;
54
:.
67Samaranayake
CB
, Kempny
A
, Naeije
R
, Gatzoulis
M
, Price
LC
, Dimopoulos
K
, Zhao
L
, Wort
SJ
, McCabe
C
.
Beta-blockade improves right ventricular diastolic function in exercising pulmonary arterial hypertension
.
Eur Respir J
2023
;
61
:.
68Naeije
R
, Badagliacca
R
.
The overloaded right ventricle and ventricular interdependence
.
Cardiovasc Res
2017
;
113
:
1474
–
1485
.
69Belenkie
I
, Horne
SG
, Dani
R
, Smith
ER
, Tyberg
JV
.
Effects of aortic constriction during experimental acute right ventricular pressure loading. Further insights into diastolic and systolic ventricular interaction
.
Circulation
1995
;
92
:
546
–
554
.
70Yamaguchi
S
, Harasawa
H
, Li
KS
, Zhu
D
, Santamore
WP
.
Comparative significance in systolic ventricular interaction
.
Cardiovasc Res
1991
;
25
:
774
–
783
.
71van Wolferen
SA
, Marcus
JT
, Westerhof
N
, Spreeuwenberg
MD
, Marques
KMJ
, Bronzwaer
JGF
, Henkens
IR
, Gan
CTJ
, Boonstra
A
, Postmus
PE
, Vonk-Noordegraaf
A
.
Right coronary artery flow impairment in patients with pulmonary hypertension
.
Eur Heart J
2008
;
29
:
120
–
127
.
72Gómez
A
, Bialostozky
D
, Zajarias
A
, Santos
E
, Palomar
A
, Martínez
ML
, Sandoval
J
.
Right ventricular ischemia in patients with primary pulmonary hypertension
.
J Am Coll Cardiol
2001
;
38
:
1137
–
1141
.
73Marcus
JT
, Gan
CT
, Zwanenburg
JJM
, Boonstra
A
, Allaart
CP
, Götte
MJW
, Vonk-Noordegraaf
A
.
Interventricular mechanical asynchrony in pulmonary arterial hypertension: left-to-right delay in peak shortening is related to right ventricular overload and left ventricular underfilling
.
J Am Coll Cardiol
2008
;
51
:
750
–
757
.
74Badagliacca
R
, Reali
M
, Poscia
R
, Pezzuto
B
, Papa
S
, Mezzapesa
M
, Nocioni
M
, Valli
G
, Giannetta
E
, Sciomer
S
, Iacoboni
C
, Fedele
F
, Vizza
CD
.
Right intraventricular dyssynchrony in idiopathic, heritable, and anorexigen-induced pulmonary arterial hypertension: clinical impact and reversibility
.
JACC Cardiovasc Imaging
2015
;
8
:
642
–
652
.
75Lamia
B
, Muir
JF
, Molano
LC
, Viacroze
C
, Benichou
J
, Bonnet
P
, Quieffin
J
, Cuvelier
A
, Naeije
R
.
Altered synchrony of right ventricular contraction in borderline pulmonary hypertension
.
Int J Cardiovasc Imaging
2017
;
33
:
1331
–
1339
.
76Pezzuto
B
, Forton
K
, Badagliacca
R
, Motoji
Y
, Faoro
V
, Naeije
R
.
Right ventricular dyssynchrony during hypoxic breathing but not during exercise in healthy subjects a speckle tracking echocardiography study
.
Exp Physiol
2018
;
103
:
1338
–
1346
.
77Cornwell
WK
, Tran
T
, Cerbin
L
, Coe
G
, Muralidhar
A
, Hunter
K
, Altman
N
, Ambardekar
AV
, Tompkins
C
, Zipse
M
, Schulte
M
, O'Gean
K
, Ostertag
M
, Hoffman
J
, Pal
JD
, Lawley
JS
, Levine
BD
, Wolfel
E
, Kohrt
WM
, Buttrick
P
.
New insights into resting and exertional right ventricular performance in the healthy heart through real-time pressure-volume analysis
.
J Physiol
2020
;
598
:
2575
–
2587
.
78Grünig
E
, Tiede
H
, Enyimayew
EO
, Ehlken
N
, Seyfarth
HJ
, Bossone
E
, D'Andrea
A
, Naeije
R
, Olschewski
H
, Ulrich
S
, Nagel
C
, Halank
M
, Fischer
C
.
Assessment and prognostic relevance of right ventricular contractile reserve in patients with severe pulmonary hypertension
.
Circulation
2013
;
128
:
2005
–
2015
.
79Ireland
CG
, Damico
RL
, Kolb
TM
, Mathai
SC
, Mukherjee
M
, Zimmerman
SL
, Shah
AA
, Wigley
FM
, Houston
BA
, Hassoun
PM
, Kass
DA
, Tedford
RJ
, Hsu
S
.
Exercise right ventricular ejection fraction predicts right ventricular contractile reserve
.
J Heart Lung Transplant
2021
;
40
:
504
–
512
.
80Sharma
T
, Lau
EMT
, Choudhary
P
, Torzillo
PJ
, Munoz
PA
, Simmons
LR
, Naeije
R
, Celermajer
DS
.
Dobutamine stress for evaluation of right ventricular reserve in pulmonary arterial hypertension
.
Eur Respir J
2015
;
45
:
700
–
708
.
81Guihaire
J
, Haddad
F
, Noly
PE
, Boulate
D
, Decante
B
, Dartevelle
P
, Humbert
M
, Verhoye
JP
, Mercier
O
, Fadel
E
.
Right ventricular reserve in a piglet model of chronic pulmonary hypertension
.
Eur Respir J
2015
;
45
:
709
–
717
.
82van den Bosch
E
, Cuypers
JAAE
, Luijnenburg
SE
, Duppen
N
, Boersma
E
, Budde
RPJ
, Krestin
GP
, Blom
NA
, Breur
HMPJ
, Snoeren
MM
, Roos-Hesselink
JW
, Kapusta
L
, Helbing
WA
.
Ventricular response to dobutamine stress cardiac magnetic resonance imaging is associated with adverse outcome during 8-year follow-up in patients with repaired tetralogy of fallot
.
Eur Heart J Cardiovasc Imaging
2020
;
21
:
1039
–
1046
.
83Kremer
N
, Rako
Z
, Douschan
P
, Gall
H
, Ghofrani
HA
, Grimminger
F
, Guth
S
, Naeije
R
, Rieth
A
, Schulz
R
, Seeger
W
, Tedford
RJ
, Vadász
I
, Vanderpool
R
, Wiedenroth
CB
, Richter
MJ
, Tello
K
.
Unmasking right ventricular-arterial uncoupling during fluid challenge in pulmonary hypertension
.
J Heart Lung Transplant
2022
;
41
:
345
–
355
.
84Bogaard
HJ
, Abe
K
, Vonk Noordegraaf
A
, Voelkel
NF
.
The right ventricle under pressure: cellular and molecular mechanisms of right-heart failure in pulmonary hypertension
.
Chest
2009
;
135
:
794
–
804
.
85Rain
S
, Andersen
S
, Najafi
A
, Gammelgaard Schultz
J
, da Silva Goncalves Bós
D
, Handoko
ML
, Bogaard
HJ
, Vonk-Noordegraaf
A
, Andersen
A
, van der Velden
J
, Ottenheijm
CAC
, de Man
FS
.
Right ventricular myocardial stiffness in experimental pulmonary arterial hypertension: relative contribution of fibrosis and myofibril stiffness
.
Circ Heart Fail
2016
;
9
(
7
):
e002636
.
86Hsu
S
, Kokkonen-Simon
KM
, Kirk
JA
, Kolb
TM
, Damico
RL
, Mathai
SC
, Mukherjee
M
, Shah
AA
, Wigley
FM
, Margulies
KB
, Hassoun
PM
, Halushka
MK
, Tedford
RJ
, Kass
DA
.
Right ventricular myofilament functional differences in humans with systemic sclerosis-associated versus idiopathic pulmonary arterial hypertension
.
Circulation
2018
;
137
:
2360
–
2370
.
87Piao
L
, Marsboom
G
, Archer
SL
.
Mitochondrial metabolic adaptation in right ventricular hypertrophy and failure
.
J Mol Med (Berl)
2010
;
88
:
1011
–
1020
.
88Brittain
EL
, Talati
M
, Fessel
JP
, Zhu
H
, Penner
N
, Calcutt
MW
, West
JD
, Funke
M
, Lewis
GD
, Gerszten
RE
, Hamid
R
, Pugh
ME
, Austin
ED
, Newman
JH
, Hemnes
AR
.
Fatty acid metabolic defects and right ventricular lipotoxicity in human pulmonary arterial hypertension
.
Circulation
2016
;
133
:
1936
–
1944
.
89Hemnes
AR
, Brittain
EL
, Trammell
AW
, Fessel
JP
, Austin
ED
, Penner
N
, Maynard
KB
, Gleaves
L
, Talati
M
, Absi
T
, Disalvo
T
, West
J
.
Evidence for right ventricular lipotoxicity in heritable pulmonary arterial hypertension
.
Am J Respir Crit Care Med
2014
;
189
:
325
–
334
.
90Talati
MH
, Brittain
EL
, Fessel
JP
, Penner
N
, Atkinson
J
, Funke
M
, Grueter
C
, Jerome
WG
, Freeman
M
, Newman
JH
, West
J
, Hemnes
AR
.
Mechanisms of lipid accumulation in the bone morphogenetic protein receptor type 2 mutant right ventricle
.
Am J Respir Crit Care Med
2016
;
194
:
719
–
728
.
91Velez-Roa
S
, Ciarka
A
, Najem
B
, Vachiery
JL
, Naeije
R
, van de Borne
P
.
Increased sympathetic nerve activity in pulmonary artery hypertension
.
Circulation
2004
;
110
:
1308
–
1312
.
92Ciarka
A
, Doan
V
, Velez-Roa
S
, Naeije
R
, van de Borne
P
.
Prognostic significance of sympathetic nervous system activation in pulmonary arterial hypertension
.
Am J Respir Crit Care Med
2010
;
181
:
1269
–
1275
.
93Bristow
MR
, Ginsburg
R
, Umans
V
, Fowler
M
, Minobe
W
, Rasmussen
R
, Zera
P
, Menlove
R
, Shah
P
, Jamieson
S
.
Beta 1- and beta 2-adrenergic-receptor subpopulations in nonfailing and failing human ventricular myocardium: coupling of both receptor subtypes to muscle contraction and selective beta 1-receptor down-regulation in heart failure
.
Circ Res
1986
;
59
:
297
–
309
.
94da Silva Gonçalves Bós
D
, Van Der Bruggen
CEE
, Kurakula
K
, Sun
XQ
, Casali
KR
, Casali
AG
, Rol
N
, Szulcek
R
, Dos Remedios
C
, Guignabert
C
, Tu
L
, Dorfmüller
P
, Humbert
M
, Wijnker
PJM
, Kuster
DWD
, van der Velden
J
, Goumans
MJ
, Bogaard
HJ
, Vonk-Noordegraaf
A
, de Man
FS
, Handoko
ML
.
Contribution of impaired parasympathetic nervous system activity to right ventricular dysfunction and pulmonary vascular remodeling in pulmonary arterial hypertension
.
Circulation
2018
;
137
:
910
–
924
.
95de Man
FS
, Tu
L
, Handoko
ML
, Rain
S
, Ruiter
G
, François
C
, Schalij
I
, Dorfmüller
P
, Simonneau
G
, Fadel
E
, Perros
F
, Boonstra
A
, Postmus
PE
, van der Velden
J
, Vonk-Noordegraaf
A
, Humbert
M
, Eddahibi
S
, Guignabert
C
.
Dysregulated renin-angiotensin-aldosterone system contributes to pulmonary arterial hypertension
.
Am J Respir Crit Care Med
2012
;
186
:
780
–
789
.
96Peters
EL
, Bogaard
HJ
, Vonk Noordegraaf
A
, de Man
FS
.
Neurohumoral modulation in pulmonary arterial hypertension
.
Eur Respir J
2021
;
58
(
4
):
2004633
.
97Jacobs
W
, van de Veerdonk
MC
, Trip
P
, de Man
F
, Heymans
MW
, Marcus
JT
, Kawut
SM
, Bogaard
HJ
, Boonstra
A
, Vonk Noordegraaf
A
.
The right ventricle explains sex differences in survival in idiopathic pulmonary arterial hypertension
.
Chest
2014
;
145
:
1230
–
1236
.
98van Wezenbeek
J
, Groeneveldt
JA
, Llucià-Valldeperas
A
, van der Bruggen
CE
, Jansen
SMA
, Smits
AJ
, Smal
R
, van Leeuwen
JW
, Remedios
C
, Keogh
A
, Humbert
M
, Dorfmüller
P
, Mercier
O
, Guignabert
C
, Niessen
HWM
, Handoko
ML
, Marcus
JT
, Meijboom
LJ
, Oosterveer
FPT
, Westerhof
BE
, Heijboer
AC
, Bogaard
HJ
, Vonk Noordegraaf
A
, Goumans
MJ
, de Man
FS
.
Interplay of sex hormones and long-term right ventricular adaptation in a Dutch PAH-cohort
.
J Heart Lung Transplant
2022
;
41
:
445
–
457
.
99Hester
J
, Ventetuolo
C
, Lahm
T
.
Sex, gender and sex hormones in pulmonary hypertension and right ventricular failure
.
Compr Physiol
2019
;
10
:
125
–
170
.
100Frump
A
, Lahm
T
.
The Y chromosome takes the field to modify BMPR2 expression
.
Am J Respir Crit Care Med
2018
;
198
:
1476
–
1478
.
101Evans J
D
, Girerd
B
, Montani
D
, Wang X
J
, Galie
N
, Austin E
D
, Elliott
G
, Asano
K
, Grunig
E
, Yan
Y
, Jing Z
C
, Manes
A
, Palazzini
M
, Wheeler L
A
, Nakayama
I
, Satoh
T
, Eichstaedt
C
, Hinderhofer
K
, Wolf
M
, Morrell N
W
.
BMPR2 Mutations and survival in pulmonary arterial hypertension: an individual participant data meta-analysis
.
Lancet Respir Med
2016
;
4
:
129
–
137
.
102van der Bruggen
CE
, Happé
CM
, Dorfmüller
P
, Trip
P
, Spruijt
OA
, Rol
N
, Hoevenaars
FP
, Houweling
AC
, Girerd
B
, Marcus
JT
, Mercier
O
, Humbert
M
, Handoko
ML
, van der Velden
J
, Vonk Noordegraaf
A
, Bogaard
HJ
, Goumans
MJ
, de Man
FS
.
Bone morphogenetic protein receptor type 2 mutation in pulmonary arterial hypertension: a view on the right ventricle
.
Circulation
2016
;
133
:
1747
–
1760
.
103Hautefort
A
, Mendes-Ferreira
P
, Sabourin
J
, Manaud
G
, Bertero
T
, Rucker-Martin
C
, Riou
M
, Adão
R
, Manoury
B
, Lambert
M
, Boet
A
, Lecerf
F
, Domergue
V
, Brás-Silva
C
, Gomez A
M
, Montani
D
, Girerd
B
, Humbert
M
, Antigny
F
, Perros
F
.
BMPR2 mutant rats develop pulmonary and cardiac characteristics of pulmonary arterial hypertension
.
Circulation
2019
;
139
:
932
–
948
.
104Boehm
M
, Tian
X
, Ali
MK
, Mao
Y
, Ichimura
K
, Zhao
M
, Kuramoto
K
, Dannewitz Prosseda
S
, Fajardo
G
, Dufva
MJ
, Qin
X
, Kheyfets
VO
, Bernstein
D
, Reddy
S
, Metzger
RJ
, Zamanian
RT
, Haddad
F
, Spiekerkoetter
E
.
Improving right ventricular function by increasing BMP signaling with FK506
.
Am J Respir Cell Mol Biol
2021
;
66
:
272
–
287
.
105Dewachter
L
, Dewachter
C
.
Inlammation in right ventricular failure. Does it matter?
Front Physiol
2018
;
9
:
1056
.
106Rondelet
B
, Dewachter
C
, Kerbaul
F
, Kang
X
, Fesler
P
, Brimioulle
S
, Naeije
R
, Dewachter
L
.
Prolonged overcirculation-induced pulmonary arterial hypertension as a cause of right ventricular failure
.
Eur Heart J
2012
;
33
:
1017
–
1026
.
107Dewachter
C
, Belhaj
A
, Rondelet
B
, Vercruyssen
M
, Schraufnagel
DP
, Remmelink
M
, Brimioulle
S
, Kerbaul
F
, Naeije
R
, Dewachter
L
.
Myocardial inflammation in acute right ventricular failure. Effects of prostacyclin therapy
.
J Heart Lung Transplant
2015
;
34
:
1334
–
1345
.
108Al-Qazazi
R
, Lima
PDA
, Prisco
SZ
, Potus
F
, Dasgupta
A
, Chen
KH
, Tian
L
, Bentley
RET
, Mewburn
J
, Martin
AY
, Wu
D
, Jones
O
, Maurice
DH
, Bonnet
S
, Provencher
S
, Prins
KW
, Archer
SL
.
Macrophage–NLRP3 activation promotes right ventricle failure in pulmonary arterial hypertension
.
Am J Respir Crit Care Med
2022
;
206
:
608
–
624
.
109Vachiéry
JL
, Adir
Y
, Barberà
JA
, Champion
H
, Coghlan
JG
, Cottin
V
, De Marco
T
, Galiè
N
, Ghio
S
, Gibbs
JSR
, Martinez
F
, Semigran
M
, Simonneau
G
, Wells
A
, Seeger
W
.
Pulmonary hypertension due to left heart diseases
.
J Am Coll Cardiol
2013
;
62
(
25, Suppl
):
D100
–
D108
.
110Guazzi
M
, Naeije
R
.
Pulmonary hypertension in heart failure: pathophysiology, pathobiology, and emerging clinical perspectives
.
J Am Coll Cardiol
2017
;
69
:
1718
–
1734
.
111Gerges
M
, Gerges
C
, Pistritto
AM
, Lang
MB
, Trip
P
, Jakowitsch
J
, Binder
T
, Lang
IM
.
Pulmonary hypertension in heart failure: epidemiology, right ventricular function and survival
.
Am J Respir Crit Care Med
2015
;
192
:
1234
–
1246
.
112Di Salvo
TG
, Mathier
M
, Semigran
M
, Dec
WG
.
Preserved right ventricular ejection fraction predicts exercise capacity and survival in advanced heart failure
.
J Am Coll Cardiol
1995
;
25
:
1143
–
1153
.
113Ghio
S
, Gavazzi
A
, Campana
C
, Inserra
C
, Klersy
C
, Sebastiani
R
, Arbustini
E
, Recusani
F
, Tavazzi
L
.
Independent and additive prognostic value of right ventricular systolic function and pulmonary artery pressure in patients with chronic heart failure
.
J Am Coll Cardiol
2001
;
37
:
183
–
188
.
114Dini
FL
, Pugliese
NR
, Ameri
P
, Attanasio
U
, Badagliacca
R
, Correale
M
, Mercurio
V
, Tocchetti
CG
, Agostoni
P
, Palazzuoli
A
; Heart Failure Study Group of the Italian Society of Cardiology
.
Right ventricular failure in left heart disease. From pathophysiology to clinical manifestations and prognosis
.
Heart Fail Rev
2023
;
28
:
757
–
766
.
115Guazzi
M
, Naeije
R
.
Right heart phenotype in heart failure with preserved ejection fraction
.
Circ Heart Fail
2021
;
14
:
e007840
.
116Pagnamenta
A
, Dewachter
C
, McEntee
K
, Fesler
P
, Brimioulle
S
, Naeije
R
.
Early right ventriculo-arterial uncoupling in borderline pulmonary hypertension on experimental heart failure
.
J Appl Physiol
2010
;
109
:
1080
–
1085
.
117Singh
I
, Rahaghi
FN
, Naeije
R
, Oliveira
RKF
, Systrom
DM
, Waxman
AB
.
Right ventricular-arterial uncoupling during exercise in heart failure with preserved ejection fraction: role of pulmonary vascular dysfunction
.
Chest
2019
;
156
:
933
–
943
.
118Singh
I
, Oliveira
RKF
, Heerdt
PM
, Pari
R
, Systrom
DM
, Waxman
AB
.
Sex-related differences in dynamic right ventricular-pulmonary vascular coupling in heart failure with preserved ejection fraction
.
Chest
2021
;
159
:
2402
–
2416
.
119Guazzi
M
, Bandera
F
, Pelissero
G
, Castelvecchio
S
, Menicanti
L
, Ghio
S
, Temporelli
PL
, Arena
R
.
Tricuspid annular plane systolic excursion and pulmonary arterial systolic pressure relationship in heart failure: an index of right ventricular contractile function and prognosis
.
Am J Physiol Heart Circ Physiol
2013
;
305
:
H1373
–
H1381
.
120Guazzi
M
, Dixon
D
, Labate
V
, Beussink-Nelson
L
, Bandera
F
, Cuttica
MJ
, Shah
SJ
.
RV contractile function and its coupling to pulmonary circulation in heart failure with preserved ejection fraction: stratification of clinical phenotypes and outcomes
.
JACC Cardiovasc Imaging
2017
;
10
:
1211
–
1221
.
121Tello
K
, Wan
J
, Dalmer
A
, Vanderpool
R
, Ghofrani
HA
, Naeije
R
, Roller
F
, Mohajerani
E
, Seeger
W
, Herberg
U
, Sommer
N
, Gall
H
, Richter
MJ
.
Validation of the tricuspid annular plane systolic excursion/systolic pulmonary artery pressure ratio for the assessment of right ventricular-arterial coupling in severe pulmonary hypertension
.
Circ Cardiovasc Imaging
2019
;
12
(
9
):
e009047
.
122Schmeisser
A
, Rauwolf
T
, Groscheck
T
, Kropf
S
, Luani
B
, Tanev
I
, Hansen
M
, Meißler
S
, Steendijk
P
, Braun-Dullaeus
RC
.
Pressure-volume loop validation of TAPSE/PASP for right ventricular arterial coupling in heart failure with pulmonary hypertension
.
Eur Heart J Cardiovasc Imaging
2021
;
22
:
168
–
176
.
123Guazzi
M
, Naeije
R
, Arena
R
, Corrà
U
, Ghio
S
, Forfia
P
, Rossi
A
, Cahalin
LP
, Bandera
F
, Temporelli
P
.
Echocardiography of right ventriculo-arterial coupling combined to cardiopulmonary exercise testing to predict outcome in heart failure
.
Chest
2015
;
148
:
226
–
234
.
124Ghio
S
, Guazzi
M
, Scardovi
AB
, Klersy
C
, Clemenza
F
, Carluccio
E
, Temporelli
PL
, Rossi
A
, Faggiano
P
, Traversi
E
, Vriz
O
.
Dini FL; all investigators. Different correlates but similar prognostic implications for right ventricular dysfunction in heart failure with reduced or preserved ejection fraction
.
Eur J Heart Fail
2017
;
19
:
873
–
879
.
125Bosch
L
, Lam
CSP
, Gong
L
, Chan
SP
, Sim
D
, Yeo
D
, Jaufeerally
F
, Leong
KTG
, Ong
HY
, Ng
TP
, Richards
AM
, Arslan
F
, Ling
LH
.
Right ventricular dysfunction in left-sided heart failure with preserved versus reduced ejection fraction
.
Eur J Heart Fail
2017
;
19
:
1664
–
1671
.
126Gorter
TM
, van Veldhuisen
DJ
, Voors
AA
, Hummel
YM
, Lam
CSP
, Berger
RMF
, van Melle
JP
, Hoendermis
ES
.
Right ventricular-vascular coupling in heart failure with preserved ejection fraction and pre- vs. Post-capillary pulmonary hypertension
.
Eur Heart J Cardiovasc Imaging
2018
;
19
:
425
–
432
.
127Tello
K
, Axmann
J
, Ghofrani
HA
, Naeije
R
, Rieth
A
, Seeger
W
, Gall
H
, Richter
MJ
.
Relevance of the TAPSE/PAPS ratio in pulmonary arterial hypertension
.
Int J Cardiol
2018
;
266
:
229
–
235
.
128Tello
K
, Ghofrani
HA
, Heinze
C
, Krueger
K
, Naeije
R
, Raubach
C
, Seeger
W
, Sommer
N
, Gall
H
, Richter
MJ
.
A simple echocardiographic estimate of right ventricular-arterial coupling to assess severity and outcome in pulmonary hypertension on chronic lung disease
.
Eur Respir J
2019
;
54
:
1802435
.
129Brener
MI
, Lurz
P
, Hausleiter
J
, Rodés-Cabau
J
, Fam
N
, Kodali
SK
, Rommel
KP
, Muntané-Carol
G
, Gavazzoni
M
, Nazif
TM
, Pozzoli
A
, Alessandrini
H
, Latib
A
, Biasco
L
, Braun
D
, Brochet
E
, Denti
P
, Lubos
E
, Ludwig
S
, Kalbacher
D
, Estevez-Loureiro
R
, Connelly
KA
, Frerker
C
, Ho
EC
, Juliard
JM
, Harr
C
, Monivas
V
, Nickenig
G
, Pedrazzini
G
, Philippon
F
, Praz
F
, Puri
R
, Schofer
J
, Sievert
H
, Tang
GHL
, Andreas
M
, Thiele
H
, Unterhuber
M
, Himbert
D
, Alcázar
MU
, Von Bardeleben
RS
, Windecker
S
, Wild
MG
, Maisano
F
, Leon
MB
, Taramasso
M
, Hahn
RT
.
J right ventricular-pulmonary arterial coupling and afterload reserve in patients undergoing transcatheter tricuspid valve repair
.
J Am Coll Cardiol
2022
;
79
:
448
–
461
.
130Karam
N
, Stolz
L
, Orban
M
, Deseive
S
, Praz
F
, Kalbacher
D
, Westermann
D
, Braun
D
, Näbauer
M
, Neuss
M
, Butter
C
, Kassar
M
, Petrescu
A
, Pfister
R
, Iliadis
C
, Unterhuber
M
, Park
SD
, Thiele
H
, Baldus
S
, Stephan von Bardeleben
R
, Blankenberg
S
, Massberg
S
, Windecker
S
, Lurz
P
, Hausleiter
J
.
Impact of right ventricular dysfunction on outcomes after transcatheter edge-to-edge repair for secondary mitral regurgitation
.
JACC Cardiovasc Imaging
2021
;
14
:
768
–
778
.
131Cahill
TJ
, Pibarot
P
, Yu
X
, Babaliaros
V
, Blanke
P
, Clavel
MA
, Douglas
PS
, Khalique
OK
, Leipsic
J
, Makkar
R
, Alu
MC
, Kodali
S
, Mack
MJ
, Leon
MB
, Hahn
RT
.
Impact of right ventricle-pulmonary artery coupling on clinical outcomes in the PARTNER 3 trial
.
JACC Cardiovasc Interv
2022
;
15
:
1823
–
1833
.
132Yogeswaran
A
, Kuhnert
S
, Gall
H
, Faber
M
, Krauss
E
, Rako
ZA
, Keranov
S
, Grimminger
F
, Ghofrani
HA
, Naeije
R
, Seeger
W
, Richter
MJ
, Tello
K
.
Relevance of cor pulmonale in COPD with and without pulmonary hypertension. A retrospective cohort study
.
Front Cardiovasc
2022
;
9
:
826369
.
133Vriz
O
, Pirisi
M
, Bossone
E
, Fadl ElMula
FEM
, Palatini
P
, Naeije
R
.
Right ventricular-arterial uncoupoling in mild-to-moderate systemic hypertension
.
J Hypertens
2020
;
38
:
374
–
381
.
134D'Alto
M
, Marra
AM
, Severino
S
, Salzano
A
, Romeo
E
, De Rosa
R
, Stagnaro
FM
, Pagnano
G
, Verde
R
, Murino
P
, Farro
A
, Ciccarelli
G
, Vargas
M
, Fiorentino
G
, Servillo
G
, Gentile
I
, Corcione
A
, Cittadini
A
, Naeije
R
, Golino
P
.
Right ventricular-arterial uncoupling predicts survival in COVID-19 ATDS
.
Crit Care
2020
;
24
:
670
.
135Bursi
F
, Santangelo
G
, Barbieri
A
, Vella
AM
, Toriello
F
, Valli
F
, Sansalone
D
, Carugo
S
, Guazzi
M
.
Impact of right ventricular-pulmonary circulation coupling on mortality in SARS-CoV-2 infection
.
J Am Heart Assoc
2022
;
11
:
e023220
.
136Ferrara
F
, Rudski
LG
, Vriz
O
, Gargani
L
, Afilalo
J
, D'Andrea
A
, D'Alto
M
, Marra
AM
, Acri
E
, Stanziola
AA
, Ghio
S
, Cittadini
A
, Naeije
R
, Bossone
E
.
Physiologic correlates of tricuspid annular plane systolic excursion in 1168 healthy subjects
.
Int J Cardiol
2016
;
223
:
736
–
743
.
137D'Alto
M
, Pavelescu
A
, Argiento
P
, Romeo
E
, Correra
A
, Di Marco
GM
, D'Andrea
A
, Sarubbi
B
, Russo
MG
, Naeije
R
.
Echocardiographic assessment of right ventricular contractile reserve in healthy subjects
.
Echocardiography
2017
;
34
:
61
–
68
.
138Forton
K
, Motoji
Y
, Caravita
S
, Faoro
V
, Naeije
R
.
Exercise stress echocardiography of the pulmonary circulation and right ventricular-arterial coupling in healthy adolescents
.
Eur Heart J Cardiovasc Imaging
2021
;
2
:
688
–
694
.
139Martens
P
, Verbrugge
FH
, Bertrand
PB
, Verhaert
D
, Vandervoort
P
, Dupont
M
, Tang
WHW
, Janssens
S
, Mullens
W
.
Effect of cardiac resynchronization therapy on exercise-induced pulmonary hypertension and right ventricular-arterial coupling
.
Circ Cardiovasc Imaging
2018
;
11
:
e007813
.
140Rieth
AJ
, Richter
MJ
, Tello
K
, Gall
H
, Ghofrani
HA
, Guth
S
, Wiedenroth
CB
, Seeger
W
, Kriechbaum
SD
, Mitrovic
V
, Schulze
PC
, Hamm
CW
.
Exercise hemodynamics in heart failure patients with preserved and mid-range ejection fraction: key role of the right heart
.
Clin Res Cardiol
2022
;
111
:
393
–
405
.
141Pinedo
M
, Villacorta
E
, Tapia
C
, Arnold
R
, Lopez
J
, Revilla
A
, Gomez
I
, Fulquet
E
, San Roman
JA
.
Inter- and intra-observer variability in the echocardiographic evaluation of right ventricular function
.
Rev Esp Cardiol
2010
;
63
:
802
–
809
.
142Ghio
S
, Mercurio
V
, Fortuni
F
, Forfia
PR
, Gall
H
, Ghofrani
A
, Mathai
SC
, Mazurek
JA
, Mukherjee
M
, Richter
M
, Scelsi
L
, Hassoun
PM
, Tello
K
.
TAPSE In PAH investigators. A comprehensive echocardiographic method for risk stratification in pulmonary arterial hypertension
.
Eur Respir J
2020
;
56
:
2000513
.
143Grapsa
J
, O'Regan
DP
, Pavlopoulos
H
, Durighel
G
, Dawson
D
, Nihoyannopoulos
P
.
Right ventricular remodelling in pulmonary arterial hypertension with three-dimensional echocardiography: comparison with cardiac magnetic resonance imaging
.
Eur J Echocardiogr
2010
;
11
:
64
–
73
.
144Muraru
D
, Spadotto
V
, Cecchetto
A
, Romeo
G
, Aruta
P
, Ermacora
D
, Jenei
C
, Cucchini
U
, Iliceto
S
, Badano
LP
.
New speckle-tracking algorithm for right ventricular volume analysis from three-dimensional echocardiographic data sets: validation with cardiac magnetic resonance and comparison with the previous analysis tool
.
Eur Heart J Cardiovasc Imaging
2016
;
17
:
1279
–
1289
.
145Jone
PN
, Schäfer
M
, Pan
Z
, Ivy
DD
.
Right ventricular-arterial coupling ratio derived from 3-dimensional echocardiography predicts outcomes in pediatric pulmonary hypertension
.
Circ Cardiovasc Imaging
2019
;
12
:
e008176
.
146Richter
MJ
, Yogeswaran
A
, Husain-Syed
F
, Vadász
I
, Rako
Z
, Mohajerani
E
, Ghofrani
HA
, Naeije
R
, Seeger
W
, Herberg
U
, Rieth
A
, Tedford
RJ
, Grimminger
F
, Gall
H
, Tello
K
.
A novel non-invasive and echocardiography-derived method for quantification of right ventricular pressure-volume loops
.
Eur Heart J Cardiovasc Imaging
2022
;
23
:
498
–
507
.
147Meluzin
J
, Spinarova
L
, Dusek
L
, Toman
J
, Hude
P
, Krejci
J
.
Prognostic importance of the right ventricular function assessed by Doppler tissue imaging
.
Eur J Echocardiogr
2003
;
4
:
262
–
271
.
148Zhao
K
, Zhou
J
, Guo
JT
, Wu
CH
, Li
SL
, Zhang
Q
, Tian
X
, Shan
WC
, Ding
ZJ
, Yuan
LS
, Zheng
Q
, Gao
XL
, Guo
N
, Tian
HS
, Wei
QM
, Hu
XT
, Cui
YK
, Geng
X
, Wang
Q
, Cui
W
.
Association between tricuspid annular systolic velocity and poor short-term prognosis in patients with acute decompensated heart failure
.
Ann Med
2022
;
54
:
2898
–
2908
.
149Ünlü
S
, Bézy
S
, Cvijic
M
, Duchenne
J
, Delcroix
M
, Voigt
JU
.
Right ventricular strain related to pulmonary artery pressure predicts clinical outcome in patients with pulmonary arterial hypertension
.
Eur Heart J Cardiovasc Imaging
2023
;
24
:
635
–
642
.
150Richter
MJ
, Rako
ZA
, Tello
K
.
Ratio between right ventricular strain and systolic pulmonary artery pressure as a surrogate for right ventricular to pulmonary arterial coupling: validation against the gold standard
.
Eur Heart J Cardiovasc Imaging
2023
;
24
:
e50
–
e52
.
151Feneley
M
, Gavaghan
T
.
Paradoxical and pseudoparadoxical interventricular septal motion in patients with right ventricular volume overload
.
Circulation
1986
;
74
:
230
–
238
.
152Burkett
DA
, Patel
SS
, Mertens
L
, Friedberg
MK
, Ivy
DD
.
Relationship between left ventricular geometry and invasive hemodynamics in pediatric pulmonary hypertension
.
Circ Cardiovasc Imaging
2020
;
13
:
e009825
.
153Badagliacca
R
, Poscia
R
, Pezzuto
B
, Papa
S
, Pesce
F
, Manzi
G
, Giannetta
E
, Raineri
C
, Schina
M
, Sciomer
S
, Parola
D
, Francone
M
, Carbone
I
, Fedele
F
, Vizza
CD
.
Right ventricular concentric hypertrophy and clinical worsening in idiopathic pulmonary arterial hypertension
.
J Heart Lung Transplant
2016
;
35
:
1321
–
1329
.
154Simpson
CE
, Damico
RL
, Kolb
TM
, Mathai
SC
, Khair
RM
, Sato
T
, Bourji
K
, Tedford
RJ
, Zimmerman
SL
, Hassoun
PM
.
Ventricular mass as a prognostic imaging biomarker in incident pulmonary arterial hypertension
.
Eur Respir J
2019
;
53
:
1802067
.
155Tampakakis
E
, Shah
SJ
, Borlaug
BA
, Leary
PJ
, Patel
HH
, Miller
WL
, Kelemen
BW
, Houston
BA
, Kolb
TM
, Damico
R
, Mathai
SC
, Kasper
EK
, Hassoun
PM
, Kass
DA
, Tedford
RJ
.
Pulmonary effective arterial elastance as a measure of right ventricular afterload and its prognostic value in pulmonary hypertension due to left heart disease
.
Circ Heart Fail
2018
;
11
:
e004436
.
156Andersen
HR
, Falk
E
, Nielsen
D
.
Right ventricular infarction: frequency, size and topography in coronary heart disease: a prospective study comprising 107 consecutive autopsies from a coronary care unit
.
J Am Coll Cardiol
1987
;
10
:
1223
–
1232
.
157Masci
PG
, Francone
M
, Desmet
W
, Ganame
J
, Todiere
G
, Donato
R
, Siciliano
V
, Carbone
I
, Mangia
M
, Strata
E
, Catalano
C
, Lombardi
M
, Agati
L
, Janssens
S
, Bogaert
J
.
Right ventricular ischemic injury in patients with acute ST-segment elevation myocardial infarction: characterization with cardiovascular magnetic resonance
.
Circulation
2010
;
122
:
1405
–
1412
.
158Grothoff
M
, Elpert
C
, Hoffmann
J
, Zachrau
J
, Lehmkuhl
L
, de Waha
S
, Desch
S
, Eitel
D
, Mende
M
, Thiele
H
, Gutberlet
M
.
Right ventricular injury in ST-elevation myocardial infarction: risk stratification by visualization of wall motion, edema, and delayed-enhancement cardiac magnetic resonance
.
Circ Cardiovasc Imaging
2012
;
5
:
60
–
68
.
159Di Bella
G
, Siciliano
V
, Aquaro
GD
, De Marchi
D
, Rovai
D
, Carerj
S
, Molinaro
S
, Lombardi
M
, Pingitore
A
.
Right ventricular dysfunction: an independent and incremental predictor of cardiac deaths late after acute myocardial infarction
.
Int J Cardiovasc Imaging
2015
;
31
:
379
–
387
.
160Sabe
MA
, Sabe
SA
, Kusunose
K
, Flamm
SD
, Griffin
BP
, Kwon
DH
.
Predictors and prognostic significance of right ventricular ejection fraction in patients with ischemic cardiomyopathy
.
Circulation
2016
;
134
:
656
–
665
.
161Gulati
A
, Ismail
TF
, Jabbour
A
, Alpendurada
F
, Guha
K
, Ismail
NA
, Raza
S
, Khwaja
J
, Brown
TD
, Morarji
K
, Liodakis
E
, Roughton
M
, Wage
R
, Pakrashi
TC
, Sharma
R
, Carpenter
JP
, Cook
SA
, Cowie
MR
, Assomull
RG
, Pennell
DJ
, Prasad
SK
.
The prevalence and prognostic significance of right ventricular systolic dysfunction in nonischemic dilated cardiomyopathy
.
Circulation
2013
;
128
:
1623
–
1633
.
162Kuribayashi
T
, Roberts
WC
.
Myocardial disarray at junction of ventricular septum and left and right ventricular free walls in hypertrophic cardiomyopathy
.
Am J Cardiol
1992
;
70
:
1333
–
1340
.
163Nagata
Y
, Konno
T
, Fujino
N
, Hodatsu
A
, Nomura
A
, Hayashi
K
, Nakamura
H
, Kawashiri
MA
, Yamagishi
M
.
Right ventricular hypertrophy is associated with cardiovascular events in hypertrophic cardiomyopathy: evidence from study with magnetic resonance imaging
.
Can J Cardiol
2015
;
31
:
702
–
708
.
164D'Andrea
A
, Limongelli
G
, Baldini
L
, Verrengia
M
, Carbone
A
, Di Palma
E
, Vastarella
R
, Masarone
D
, Tagliamonte
G
, Riegler
L
, Calabrò
R
, Russo
MG
, Bossone
E
, Pacileo
G
.
Exercise speckle-tracking strain imaging demonstrates impaired right ventricular contractile reserve in hypertrophic cardiomyopathy
.
Int J Cardiol
2017
;
227
:
209
–
216
.
165Bodez
D
, Ternacle
J
, Guellich
A
, Galat
A
, Lim
P
, Radu
C
, Guendouz
S
, Bergoend
E
, Couetil
JP
, Hittinger
L
, Dubois-Randé
JL
, Plante-Bordeneuve
V
, Deux
JF
, Mohty
D
, Damy
T
.
Prognostic value of right ventricular systolic function in cardiac amyloidosis
.
Amyloid
2016
;
23
:
158
–
167
.
166Aquaro
GD
, Negri
F
, De Luca
A
, Todiere
G
, Bianco
F
, Barison
A
, Camastra
G
, Monti
L
, Dellegrottaglie
S
, Moro
C
, Lanzillo
C
, Scatteia
A
, Di Roma
M
, Pontone
G
, Perazzolo Marra
M
, Di Bella
G
, Donato
R
, Grigoratos
C
, Emdin
M
, Sinagra
G
.
Role of right ventricular involvement in acute myocarditis, assessed by cardiac magnetic resonance
.
Int J Cardiol
2018
;
271
:
359
–
365
.
167Smedema
JP
, van Geuns
RJ
, Ector
J
, Heidbuchel
H
, Ainslie
G
, Crijns
H
.
Right ventricular involvement and the extent of left ventricular enhancement with magnetic resonance predict adverse outcome in pulmonary sarcoidosis
.
ESC Heart Fail
2018
;
5
:
157
–
171
.
168Gandjbakhch
E
, Redheuil
A
, Pousset
F
, Charron
P
, Frank
R
.
Clinical diagnosis, imaging, and genetics of arrhythmogenic right ventricular cardiomyopathy/dysplasia: JACC state-of-the-art review
.
J Am Coll Cardiol
2018
;
72
:
784
–
804
.
169te Riele
ASJM
, James
CA
, Rastegar
N
, Bhonsale
A
, Murray
B
, Tichnell
C
, Judge
DP
, Bluemke
DA
, Zimmerman
SL
, Kamel
IR
, Calkins
H
, Tandri
H
.
Yield of serial evaluation in at-risk family members of patients with ARVD/C
.
J Am Coll Cardiol
2014
;
64
:
293
–
301
.
170Mast
TP
, Taha
K
, Cramer
MJ
, Lumens
J
, van der Heijden
JF
, Bouma
BJ
, van den Berg
MP
, Asselbergs
FW
, Doevendans
PA
, Teske
AJ
.
The prognostic value of right ventricular deformation imaging in early arrhythmogenic right ventricular cardiomyopathy
.
JACC Cardiovasc Imaging
2019
;
12
:
446
–
455
.
171Leren
IS
, Saberniak
J
, Haland
TF
, Edvardsen
T
, Haugaa
KH
.
Combination of ECG and echocardiography for identification of arrhythmic events in early ARVC
.
JACC Cardiovasc Imaging
2017
;
10
:
503
–
513
.
172Gilotra
NA
, Bhonsale
A
, James
CA
, Te Riele
ASJ
, Murray
B
, Tichnell
C
, Sawant
A
, Ong
CS
, Judge
DP
, Russell
SD
, Calkins
H
, Tedford
RJ
.
Heart failure is common and under-recognized in patients with arrhythmogenic right ventricular cardiomyopathy/dysplasia
.
Circ Heart Fail
2017
;
10
(
9
):
e003819
.
Author notes
© The Author(s) 2023. Published by Oxford University Press on behalf of the European Society of Cardiology. All rights reserved. For permissions, please e-mail:
[email protected]