-
PDF
- Split View
-
Views
-
Cite
Cite
Roberto Bolli, Mitesh Solankhi, Xiang-Liang Tang, Arunpreet Kahlon, Cell therapy in patients with heart failure: a comprehensive review and emerging concepts, Cardiovascular Research, Volume 118, Issue 4, March 2022, Pages 951–976, https://doi.org/10.1093/cvr/cvab135
- Share Icon Share
Abstract
This review summarizes the results of clinical trials of cell therapy in patients with heart failure (HF). In contrast to acute myocardial infarction (where results have been consistently negative for more than a decade), in the setting of HF the results of Phase I–II trials are encouraging, both in ischaemic and non-ischaemic cardiomyopathy. Several well-designed Phase II studies have met their primary endpoint and demonstrated an efficacy signal, which is remarkable considering that only one dose of cells was used. That an efficacy signal was seen 6–12 months after a single treatment provides a rationale for larger, rigorous trials. Importantly, no safety concerns have emerged. Amongst the various cell types tested, mesenchymal stromal cells derived from bone marrow (BM), umbilical cord, or adipose tissue show the greatest promise. In contrast, embryonic stem cells are not likely to become a clinical therapy. Unfractionated BM cells and cardiosphere-derived cells have been abandoned. The cell products used for HF will most likely be allogeneic. New approaches, such as repeated cell treatment and intravenous delivery, may revolutionize the field. As is the case for most new therapies, the development of cell therapies for HF has been slow, plagued by multifarious problems, and punctuated by many setbacks; at present, the utility of cell therapy in HF remains to be determined. What the field needs is rigorous, well-designed Phase III trials. The most important things to move forward are to keep an open mind, avoid preconceived notions, and let ourselves be guided by the evidence.
1. Introduction
Heart failure (HF) is a common, expensive, lethal, and disabling condition. Its prevalence in industrialized nations has reached epidemic proportions (e.g. ∼6.5 million in the USA), and continues to rise as the population ages.1 Despite significant advances over the last three decades, the prognosis of patients hospitalized with HF remains poor, and the 5-year mortality approaches 50%. Therefore, HF constitutes a major public health problem worldwide, a leading cause of morbidity and mortality, and an increasing burden on healthcare systems around the globe.1
Cell therapy is emerging as a promising new approach to HF.2,3 However, its development has been plagued by a host of problems,4 including a still unclear mechanism of action; some Phase I and II clinical trials that were underpowered, poorly-designed, or inconclusive; unrealistic expectations of rapid Phase III evidence of efficacy; differences in biological properties of the same cell product manufactured in different locations; hype and misinformation by the media; skepticism or negative bias by some members of academia and funding agencies; claims of efficacy by unscrupulous charlatans who seek profit by administering unproven cell products and charging patients for these procedures; conflicts of interest of investigators who own equity in cell therapy-related companies; and above all, tragic instances of scientific misconduct5 that have shaken public confidence in the entire field, undermining the efforts of hundreds of principled investigators worldwide who are working in earnest to evaluate the therapeutic potential of cell therapy and its mechanism of action.6 Few experimental therapies have been beset by so many issues.
Will cell therapy survive this perfect storm? No one can answer this question at present. Dogmatism, prejudice, and a priori nihilism are not the way forward. Nor is the attempt to extrapolate mouse data to humans; given the enormous (and obvious) species differences, such attempts are misleading. Medicine, and science in general, advances only through solid scientific evidence. As we pointed out before,4 the answer to the question of whether cell therapy works can only come from rigorous Phase III clinical trials. There is no telling as to whether or when this will happen, as it takes courage for investigators to continue working in a field that is bedevilled by so much controversy and polarization.
The purpose of this review is to summarize current factual information pertaining to the use of cell therapy in patients with HF. Because all clinical trials reported heretofore have focused on HF with reduced ejection fraction (HFrEF), for simplicity we will use the term ‘HF’ to designate HFrEF. We will limit our discussion to clinical studies and will examine them in chronological order. Our overarching goal is objectivity. Inasmuch as possible, we will avoid speculations or extrapolations from rodents to humans; instead, we will emphasize the results of randomized clinical trials, focusing on those published in the past 10 years. Our interest in HF stems from the fact that current evidence suggests this to be the cardiovascular condition in which cell therapy is most likely to find application.7,8 Trials of cytokines, such as granulocyte colony-stimulating factor (G-CSF), will not be addressed because randomized, double-blind, placebo-controlled studies have failed to show a beneficial effect of this therapy on cardiac function or other endpoints in patients with HF,9 a finding that is also supported by meta-analyses.10
2. Mechanism of action of cell therapy: present status
Before reviewing cell-based therapies for HF, it is important to clarify some key concepts that are at the very foundation of this field.
Despite two decades of intense investigation, the mechanism(s) whereby transplantation of cells improves the function and structure of the diseased heart remains elusive. What is clear is that this mechanism is different from the one which was originally postulated—that transplanted cells work by regenerating functional cardiomyocytes. Over the past 10 years, a series of 12 animal studies by our group11–22 has demonstrated that although cell therapy consistently improves function in the infarcted heart, the transplanted cells do not engraft in the myocardium and do not become cardiomyocytes; instead, they disappear almost completely within a few weeks of transplantation11–22 (reviewed in ref.6) We found that this dissociation between functional improvement and cell engraftment was consistent and independent of the cell type studied, because it occurred both with c-kit-positive cardiac cells6,11–17,20–22 and with cardiac mesenchymal cells,18,19 two types of cells that are unrelated. As we pointed out in several reviews,7,23–25 a similar phenomenon has been observed with virtually all cell types tested heretofore (including mesenchymal stromal cells (MSCs)26 and embryonic stem cells),27,28 irrespective of their phenotype, regardless of the delivery technique, and even despite preconditioning manipulations.20 The universal failure of cells to engraft implies that the beneficial effects of cell therapy must be mediated by the release of signals/mediators that modify the host myocardium in a favourable manner—the so-called paracrine hypothesis, a concept that is now accepted by virtually all researchers working in the cell therapy field.2,4,7,23–25 Of note, our results with c-kit-positive cardiac cells11–17,20–22 were contrary to the prevailing views on the mechanism of action of these cells and prompted a paradigm shift in the understanding of this product.6
The paracrine hypothesis, however, raises more questions than it answers. Although it is now clear that transplanted cells ameliorate the function of the injured heart via paracrine (or possibly endocrine) mechanisms, the nature of these mechanisms (i.e. the specific mediator(s) and the cellular processes affected) remains enigmatic. This is perhaps the most significant conceptual problem facing cell therapy today. What exactly are the reparative mediators? And what are their specific actions? Given that most cells are veritable factories secreting a panoply of growth factors, cytokines, non-coding RNAs, vesicles, bioactive lipids, etc., pinpointing the specific components of the secretome that are responsible for mediating the cells’ actions will be a Herculean task that may require a very long time, and ultimately may even not be possible.
As for the biological processes that underlie the functional improvement, there are several candidates but none has been proven to be involved thus far. Over the past decade, our laboratory has published many papers showing that although cardiac function improves after cell transplantation, there is little or no formation of new cardiomyocytes, either from transplanted cells or from endogenous sources (i.e. from pre-existing cardiac cells).11–18,22 Based on these results, we concluded that the paracrine actions of the cells do not involve myogenesis or ‘remuscularization’, i.e. the secretome of transplanted cells does not promote formation of new myocytes from endogenous sources.4,23–25 It is important to keep in mind that formation of new contractile cardiomyocytes need not be the only mechanism whereby the function of a diseased heart can improve. In principle, other mechanisms unrelated to myogenesis could result in functional improvement, including angiogenesis, a reduction in apoptosis, modulation of the extracellular matrix (e.g. reduction in fibrosis), or a change in the contractile properties of native cardiomyocytes.4,23–25 Importantly, there is mounting evidence that a persistent systemic inflammatory state contributes to the progressive deterioration in left ventricular (LV) function after myocardial infarction (MI) (ischaemic cardiomyopathy)29–31; since MSCs (the most widely studied cell type) are well-known to have anti-inflammatory properties,8 this is another potential mechanism for the salubrious effects of MSCs transplantation.25
The realization that transplanted cells work by paracrine actions has significant implications. One of the common criticisms of cell therapy is that the products used are inappropriately claimed to consist of stem cells. This is true: many studies, both preclinical and clinical, have used cells that were incorrectly referred to as ‘stem cells’ (i.e. cells that can differentiate into new cardiac cells) even though they did not meet the strict criteria for stem cells, or at least they were not demonstrated to meet those criteria. However, this incorrect labelling is hardly relevant to the clinical utility of cell-based therapies. It does not matter whether the cells being transplanted are truly stem cells or not and whether or not they have the potential to differentiate into cardiomyocytes or other cell types; since they do not engraft, their stemness would not lead to remuscularization anyway. What matters is what cells do, not how we call them. We believe it is important to move beyond nomenclature and focus on the therapeutic effects of a cell product rather than its taxonomy.
While our understanding of how transplanted cells work is inadequate and evolving, this should not be a reason for stopping clinical research. Unfortunately, the uncertainty regarding the mechanism of action of cell therapy has been used by anonymous32 and non-anonymous33,34 editorialists as an argument to advocate a moratorium on all cell therapy trials. This position is unwarranted. The experience garnered with cell therapy over the past 20 years in thousands of patients has shown it to be remarkably safe,2,4,8 and Phase I and II trials of MSCs have been encouraging, as reviewed below. Moreover, the practice of medicine is replete with examples of widely-used and accepted therapies whose mechanism of action remains unclear. A case in point: administration of statins is considered a class IA indication (strongest possible indication) for acute coronary syndromes,35 yet just how statins exert their beneficial effects in these patients is unknown. The argument that only therapies with a well-delineated mechanism of action should be investigated in humans would eliminate many treatments that are an integral part of our therapeutic armamentarium. Given that cell therapy is safe and promising (as discussed in this review), it seems unreasonable to cease clinical trials until the mechanism of action is ascertained—an outcome that may not be achievable for a long time, if ever. It is more reasonable to conduct rigorous, well-designed trials while also probing the mechanism of action in basic studies.4
In summary, countless studies performed over the past 20 years have demonstrated beyond reasonable doubt that transplantation of various types of cells improves the function of the injured heart in many different animal models, particularly in models of acute or chronic MI.24,25 However, the underlying mechanism of action remains poorly understood. A large body of work from our laboratory, published during the past decade,2,4,6,7,11–25 along with work by other groups,26–28 has debunked the initial hypothesis that cell therapy results in cardiac regeneration; instead, these studies have shown that transplanted cells do not engraft, do not become myocytes, and do not promote formation of new myocytes from endogenous sources, which means that they improve cardiac function despite the absence of detectable myocardial regeneration or remuscularization.2,4,6,7,11–25 Consequently, the salubrious effects of cell therapy must be underlain by other mechanisms, such as reduction in myocardial fibrosis, inflammation, and/or apoptosis and augmented vasculogenesis. Identification of these mechanism(s) is arguably the most important question in the field of cell therapy.
3. Clinical trials of cell therapy in heart failure
3.1 Unfractionated bone marrow mononuclear cells
Unfractionated bone marrow (BM) mononuclear cells (BM-MNCs) are a heterogeneous population that encompasses a variety of myeloid and lymphoid cells as well as small fractions of MSCs, endothelial progenitors, and haematopoietic stem cells. They are relatively easy to obtain via BM aspiration, since they do not require culture or expansion. Probably because of this, BM-MNCs were the first cell type to be investigated in patients with HF.
3.1.1 Ischaemic cardiomyopathy
In the setting of ischaemic cardiomyopathy (Table 1), early reports of efficacy64–66 were not confirmed in the largest and most rigorous study of BM-MNCs in HF to date, FOCUS CCTRN, published in 2012 (Table 1).36 FOCUS was a randomized, placebo-controlled, double-blind, Phase II trial in which 92 patients with ischaemic HF were given 100 × 106 autologous BM-MNCs or placebo by transendocardial injection. LV volumes and LV ejection fraction (LVEF) were assessed by echocardiography. At 6 months, there was no improvement in LV end-systolic volume (LVESV) index (the primary endpoint), LVEF, functional capacity (maximal O2 consumption), or myocardial perfusion defect. These negative results are congruent with those of TAC-HFT, which injected the same dose of BM-MNCs in the same population (patients with ischaemic HF).42
Trial . | Phase . | Placebo controlled; randomized; double-blind . | Follow-up . | n . | Cell type, dose, and treatment groups . | Delivery method . | Endpoint evaluation . | LVEF . | LV volumes . | Scar size . | NYHA class . | Functional capacity . | QoL . |
---|---|---|---|---|---|---|---|---|---|---|---|---|---|
FOCUS CCTRN36 Perin et al. | II | Yes; yes; yes | 6 months | 92 | 100 × 106 BM-MNCs vs. placebo | TE | Echo/SPECT | NS | NS | NS | NS | NS | NA |
POSEIDON37 Hare et al. | I/II | No; yes; no | 13 months | 30 | 20, 100, or 200 × 106 BM-MSCs (allo vs. auto) | TE | MRI | NS | Allo ↓ EDV | Both ↓ | NS | Auto ↑ (VO2 max NS) | Auto ↑ |
CADUCEUS38 Makkar et al. | I | No; yes; no | 6 months | 31 | 12.5–25 × 106 CDCs vs. control | IC | MRI | NS | NS | ↓ | NS | NS | NS |
C-CURE39 Bartunek et al. | II | No; yes; no | 24 months for safety; 6 months for efficacy | 48 | BM-derived cardiopoietic cells plus standard care vs. standard care | TE | Echo | ↑ at 6 months | ↓ ESV at 6 months | NA | ↑ at 6 months | ↑ at 6 months | ↑ at 6 months |
IMPACT-DCM and Catheter-DCM40,a | II | No; yes; no | 12 months | 39 and 22 | Ixmyelocel-T cellsb (dose unspecified) vs. standard of care | Intra-myocardial (mini-thoracotomy) or TE | Echo/SPECT | NS | NS | NA | ↑ in ischaemic HF | ↑ in ischaemic HF | NS |
PROMETHEUS41 Karantalis et al. | I/II | No; yes; yes | 18 months | 6 | 2 × 107 vs. 2 × 108 auto BM-MSCs vs. baseline | TE (non-revascularized segments) | MRI | ↑ with both cell doses | NA | ↓ ESV with both cell doses | NA | NA | NA |
TAC-HFT42 Heldman et al. | I/II | Yes; yes; yes | 12 months | 65 | 100 × 106 BM-MSCs vs. 100 × 106 BM-MNCs vs. placebo | TE | MRI/CT | NS | NS | MSC: ↓ | NS | MSCs ↑ | Both ↑ |
PRECISE43 Perin et al. | I | Yes; yes; yes | 36 months | 27 | 0.4 × 106, 0.8 × 106 or 1.2 × 106 ADRCs vs. placebo (3:1 randomization)c | TE | MRI/Echo | NS | NS | NA | NA | Preserved with ADRCs (VO2 max at 18 months) | NA |
MESAMI I44 Guijarro et al. | I | No; no; no | 2 years | 10 | 61.5 × 106 BM-MSCs vs. baseline | TE | Echo | ↑ | ↓ | NA | ↑ | ↑ (including VO2 max) | NS |
ixCELL-DCM45a Patel et al. | IIb | Yes; yes; yes | 12 months | 126 | 40–200 × 106 Ixmyelocel-T cellsb vs. placebo | TE | Echo | NS | NS | NA | NS | NS | NA |
ATHENA trials46 Henry et al. | II | Yes; yes; yes | 12 months | 31 | 80 × 106 vs. 40 × 106 ARDCs vs. placebo | TE | Echo | NS | NS | NA | ↑ | ↑ (including VO2 max) | ↑ |
CHART-147 Bartunek et al. | III | No (Sham); yes; yes | 39 weeks | 315 | 9.7 × 108–1.2 × 109 cardiopoietic cells vs. sham | TE | Echo | NS | NS | NA | NA | NS | NS |
PERFECT48 Steinhoff et al. | III | Yes; yes; yes | 6 months | 82 | 0.5–5 × 106 CD133+ cells plus CABG vs. placebo plus CABG | Intra-myocardial | MRI | NS | NS | ↓ | NA | NS | NA |
TRIDENT49 Hare et al. | II | No; yes; yes | 12 months | 30 | 20 × 106 vs. 100 × 106 allo-MSCs | TE | CT | Higher dose ↑ | NS | Both ↓ | Both ↑ | Both ↑ (no change in VO2 max) | NS |
RIMECARD50 Bartolucci et al. | I/II | Yes; yes; yes | 12 months | 30 | 1×/kg UC-MSCs vs. placebo | IV | Echo/MRI | ↑ | NS | NA | ↑ | ↑ (improvement in VE/VCO2, no change in VO2 max) | ↑ |
Kastrup et al.51 | I | No; no; no | 6 months | 10 | 100 × 106 cryo preserved adipose-derived MSCs | TE | Echo/CT | ↑ | ↓ | NA | ↑ | ↑ | NS |
ESCORT52e Menasche et al. | I | No; no; no | 12 months | 6 | 5–10 × 106 hESC-derived cardiovascular progenitors embedded in a fibrin patch | Epicardial | Echo/CT | ↑ | ↓ | NA | 4 pts ↑ | 4 pts ↑ | 4 pts ↑ |
RECARDIO53 Bassetti et al. | I | No; no; no | 1 year | 10 | 1–12 × 106 BM-MSCs vs. baseline | TE | Echo/SPECT | NS | NS | NA | ↑ | NS | NS |
II | Yes; yes; yes | 6 months, 4 years | 60 | 77.5 + 67.9 × 106 (inter-quartile range 53.8 × 106) BM-MSCs vs. placebo | TO | MRI/CT | ↑ | ↓ ESV | NS | NS | NS | ↑ | |
II | Yes; yes; yes | 6 months | 142 | Allo CDCs (25 × 106) vs. placebo (2:1 randomization) | IC | MRI | NS | ↓ EDV and ESV | NS | NA | NA | NA | |
I | Not; not; not | 12 months | 14 | 37.5–75 allo CDCs; no control group | IC (3 coronaries) | ??? | ↑ f | ↑ f | ? f | ↑ f | ? f | ↑ f | |
II | Yes; yes; yes | 12 months | 125 | 150 × 106 auto BM-MSCs vs. 5 × 106 auto CPCs vs. both vs. placebo | TO | MRI | NS | NS | NS | NA | NS | MSCs and MSCs+CPCs | |
III | No (sham controlled); yes; yes | At least 12 months (median ∼30 months) | 565 randomized; 535 treated | 150 × 106 BM-derived allo MPCs (selected with anti-STRO-3 antibodies) vs. sham procedure | TO | Echo | ? | ? | ? | ? | ? | ? |
Trial . | Phase . | Placebo controlled; randomized; double-blind . | Follow-up . | n . | Cell type, dose, and treatment groups . | Delivery method . | Endpoint evaluation . | LVEF . | LV volumes . | Scar size . | NYHA class . | Functional capacity . | QoL . |
---|---|---|---|---|---|---|---|---|---|---|---|---|---|
FOCUS CCTRN36 Perin et al. | II | Yes; yes; yes | 6 months | 92 | 100 × 106 BM-MNCs vs. placebo | TE | Echo/SPECT | NS | NS | NS | NS | NS | NA |
POSEIDON37 Hare et al. | I/II | No; yes; no | 13 months | 30 | 20, 100, or 200 × 106 BM-MSCs (allo vs. auto) | TE | MRI | NS | Allo ↓ EDV | Both ↓ | NS | Auto ↑ (VO2 max NS) | Auto ↑ |
CADUCEUS38 Makkar et al. | I | No; yes; no | 6 months | 31 | 12.5–25 × 106 CDCs vs. control | IC | MRI | NS | NS | ↓ | NS | NS | NS |
C-CURE39 Bartunek et al. | II | No; yes; no | 24 months for safety; 6 months for efficacy | 48 | BM-derived cardiopoietic cells plus standard care vs. standard care | TE | Echo | ↑ at 6 months | ↓ ESV at 6 months | NA | ↑ at 6 months | ↑ at 6 months | ↑ at 6 months |
IMPACT-DCM and Catheter-DCM40,a | II | No; yes; no | 12 months | 39 and 22 | Ixmyelocel-T cellsb (dose unspecified) vs. standard of care | Intra-myocardial (mini-thoracotomy) or TE | Echo/SPECT | NS | NS | NA | ↑ in ischaemic HF | ↑ in ischaemic HF | NS |
PROMETHEUS41 Karantalis et al. | I/II | No; yes; yes | 18 months | 6 | 2 × 107 vs. 2 × 108 auto BM-MSCs vs. baseline | TE (non-revascularized segments) | MRI | ↑ with both cell doses | NA | ↓ ESV with both cell doses | NA | NA | NA |
TAC-HFT42 Heldman et al. | I/II | Yes; yes; yes | 12 months | 65 | 100 × 106 BM-MSCs vs. 100 × 106 BM-MNCs vs. placebo | TE | MRI/CT | NS | NS | MSC: ↓ | NS | MSCs ↑ | Both ↑ |
PRECISE43 Perin et al. | I | Yes; yes; yes | 36 months | 27 | 0.4 × 106, 0.8 × 106 or 1.2 × 106 ADRCs vs. placebo (3:1 randomization)c | TE | MRI/Echo | NS | NS | NA | NA | Preserved with ADRCs (VO2 max at 18 months) | NA |
MESAMI I44 Guijarro et al. | I | No; no; no | 2 years | 10 | 61.5 × 106 BM-MSCs vs. baseline | TE | Echo | ↑ | ↓ | NA | ↑ | ↑ (including VO2 max) | NS |
ixCELL-DCM45a Patel et al. | IIb | Yes; yes; yes | 12 months | 126 | 40–200 × 106 Ixmyelocel-T cellsb vs. placebo | TE | Echo | NS | NS | NA | NS | NS | NA |
ATHENA trials46 Henry et al. | II | Yes; yes; yes | 12 months | 31 | 80 × 106 vs. 40 × 106 ARDCs vs. placebo | TE | Echo | NS | NS | NA | ↑ | ↑ (including VO2 max) | ↑ |
CHART-147 Bartunek et al. | III | No (Sham); yes; yes | 39 weeks | 315 | 9.7 × 108–1.2 × 109 cardiopoietic cells vs. sham | TE | Echo | NS | NS | NA | NA | NS | NS |
PERFECT48 Steinhoff et al. | III | Yes; yes; yes | 6 months | 82 | 0.5–5 × 106 CD133+ cells plus CABG vs. placebo plus CABG | Intra-myocardial | MRI | NS | NS | ↓ | NA | NS | NA |
TRIDENT49 Hare et al. | II | No; yes; yes | 12 months | 30 | 20 × 106 vs. 100 × 106 allo-MSCs | TE | CT | Higher dose ↑ | NS | Both ↓ | Both ↑ | Both ↑ (no change in VO2 max) | NS |
RIMECARD50 Bartolucci et al. | I/II | Yes; yes; yes | 12 months | 30 | 1×/kg UC-MSCs vs. placebo | IV | Echo/MRI | ↑ | NS | NA | ↑ | ↑ (improvement in VE/VCO2, no change in VO2 max) | ↑ |
Kastrup et al.51 | I | No; no; no | 6 months | 10 | 100 × 106 cryo preserved adipose-derived MSCs | TE | Echo/CT | ↑ | ↓ | NA | ↑ | ↑ | NS |
ESCORT52e Menasche et al. | I | No; no; no | 12 months | 6 | 5–10 × 106 hESC-derived cardiovascular progenitors embedded in a fibrin patch | Epicardial | Echo/CT | ↑ | ↓ | NA | 4 pts ↑ | 4 pts ↑ | 4 pts ↑ |
RECARDIO53 Bassetti et al. | I | No; no; no | 1 year | 10 | 1–12 × 106 BM-MSCs vs. baseline | TE | Echo/SPECT | NS | NS | NA | ↑ | NS | NS |
II | Yes; yes; yes | 6 months, 4 years | 60 | 77.5 + 67.9 × 106 (inter-quartile range 53.8 × 106) BM-MSCs vs. placebo | TO | MRI/CT | ↑ | ↓ ESV | NS | NS | NS | ↑ | |
II | Yes; yes; yes | 6 months | 142 | Allo CDCs (25 × 106) vs. placebo (2:1 randomization) | IC | MRI | NS | ↓ EDV and ESV | NS | NA | NA | NA | |
I | Not; not; not | 12 months | 14 | 37.5–75 allo CDCs; no control group | IC (3 coronaries) | ??? | ↑ f | ↑ f | ? f | ↑ f | ? f | ↑ f | |
II | Yes; yes; yes | 12 months | 125 | 150 × 106 auto BM-MSCs vs. 5 × 106 auto CPCs vs. both vs. placebo | TO | MRI | NS | NS | NS | NA | NS | MSCs and MSCs+CPCs | |
III | No (sham controlled); yes; yes | At least 12 months (median ∼30 months) | 565 randomized; 535 treated | 150 × 106 BM-derived allo MPCs (selected with anti-STRO-3 antibodies) vs. sham procedure | TO | Echo | ? | ? | ? | ? | ? | ? |
Trials are listed in chronological order of publication.
↑indicates increase; ↓, decrease; allo, allogeneic; ARDCs, adipose-derived regenerative cells; auto, autologous; BMMNCs, bone marrow mononuclear cells; BM-MSCs, bone marrow-derived mesenchymal stem cells; CDCs, cardiosphere-derived cells; CPCs, cardiopoietic cells; CT, computed tomography; Echo, echocardiography; EDV, end-diastolic volume; EF, ejection fraction; ESV, end-systolic volume; hESCs, human embryonic stem cells; IC, intracoronary; IV, intravenous; LV, left ventricle; LVEF, left ventricular ejection fraction; MRI, magnetic resonance imaging; n, number of patients; NA, not assessed; NS, not significant; NYHA, New York Heart Association; pt, patient; QoL, quality of life; SPECT, single-photon emission tomography; TE, transendocardial; UC-MSCs, umbilical cord-derived mesenchymal stem cells; VCO2, volume of exhaled carbon dioxide; VE, ventilation; VO2 max, maximal oxygen consumption.
ixCELL-DCM showed significant reduction of the primary endpoint (all-cause deaths and cardiovascular admissions to hospital) in patients who received ixmyelocel-T.
Ixmyelocel-T is a product containing allogenic bone marrow cells produced by selectively expanding two key types of bone marrow mononuclear cells: CD90+ mesenchymal stem cells and CD45+ CD14+ auto-fluorescent+ activated macrophages.
Three-dose escalation of ADRCs; 3rd escalation dose was not used.
Trial stopped prematurely due to futility; NT-proBNP levels reduced by CDCs at 6 months.
Patients received immunosuppression for 1 month. One patient died early post-operatively from treatment-unrelated comorbidities. All others had uneventful recoveries. No tumour was detected during follow-up, and none of the patients presented with arrhythmias. Three patients developed clinically silent alloimmunization. One patient had only followed up for 6 months.
Data not interpretable due to lack of control group.
In CONCERT-HF, there was a significant reduction in HF-MACE (all cause death, HF admission, or HF exacerbation) in patients randomized to CPCs alone or CPCs + MSCs.
In DREAM-HF, there was a significant reduction in MI or stroke and in MACE (cardiac death, MI, or stroke) among all patients; there was also a reduction in cardiac death in NYHA class II patients; other (secondary) endpoints have not been released yet.
Trial . | Phase . | Placebo controlled; randomized; double-blind . | Follow-up . | n . | Cell type, dose, and treatment groups . | Delivery method . | Endpoint evaluation . | LVEF . | LV volumes . | Scar size . | NYHA class . | Functional capacity . | QoL . |
---|---|---|---|---|---|---|---|---|---|---|---|---|---|
FOCUS CCTRN36 Perin et al. | II | Yes; yes; yes | 6 months | 92 | 100 × 106 BM-MNCs vs. placebo | TE | Echo/SPECT | NS | NS | NS | NS | NS | NA |
POSEIDON37 Hare et al. | I/II | No; yes; no | 13 months | 30 | 20, 100, or 200 × 106 BM-MSCs (allo vs. auto) | TE | MRI | NS | Allo ↓ EDV | Both ↓ | NS | Auto ↑ (VO2 max NS) | Auto ↑ |
CADUCEUS38 Makkar et al. | I | No; yes; no | 6 months | 31 | 12.5–25 × 106 CDCs vs. control | IC | MRI | NS | NS | ↓ | NS | NS | NS |
C-CURE39 Bartunek et al. | II | No; yes; no | 24 months for safety; 6 months for efficacy | 48 | BM-derived cardiopoietic cells plus standard care vs. standard care | TE | Echo | ↑ at 6 months | ↓ ESV at 6 months | NA | ↑ at 6 months | ↑ at 6 months | ↑ at 6 months |
IMPACT-DCM and Catheter-DCM40,a | II | No; yes; no | 12 months | 39 and 22 | Ixmyelocel-T cellsb (dose unspecified) vs. standard of care | Intra-myocardial (mini-thoracotomy) or TE | Echo/SPECT | NS | NS | NA | ↑ in ischaemic HF | ↑ in ischaemic HF | NS |
PROMETHEUS41 Karantalis et al. | I/II | No; yes; yes | 18 months | 6 | 2 × 107 vs. 2 × 108 auto BM-MSCs vs. baseline | TE (non-revascularized segments) | MRI | ↑ with both cell doses | NA | ↓ ESV with both cell doses | NA | NA | NA |
TAC-HFT42 Heldman et al. | I/II | Yes; yes; yes | 12 months | 65 | 100 × 106 BM-MSCs vs. 100 × 106 BM-MNCs vs. placebo | TE | MRI/CT | NS | NS | MSC: ↓ | NS | MSCs ↑ | Both ↑ |
PRECISE43 Perin et al. | I | Yes; yes; yes | 36 months | 27 | 0.4 × 106, 0.8 × 106 or 1.2 × 106 ADRCs vs. placebo (3:1 randomization)c | TE | MRI/Echo | NS | NS | NA | NA | Preserved with ADRCs (VO2 max at 18 months) | NA |
MESAMI I44 Guijarro et al. | I | No; no; no | 2 years | 10 | 61.5 × 106 BM-MSCs vs. baseline | TE | Echo | ↑ | ↓ | NA | ↑ | ↑ (including VO2 max) | NS |
ixCELL-DCM45a Patel et al. | IIb | Yes; yes; yes | 12 months | 126 | 40–200 × 106 Ixmyelocel-T cellsb vs. placebo | TE | Echo | NS | NS | NA | NS | NS | NA |
ATHENA trials46 Henry et al. | II | Yes; yes; yes | 12 months | 31 | 80 × 106 vs. 40 × 106 ARDCs vs. placebo | TE | Echo | NS | NS | NA | ↑ | ↑ (including VO2 max) | ↑ |
CHART-147 Bartunek et al. | III | No (Sham); yes; yes | 39 weeks | 315 | 9.7 × 108–1.2 × 109 cardiopoietic cells vs. sham | TE | Echo | NS | NS | NA | NA | NS | NS |
PERFECT48 Steinhoff et al. | III | Yes; yes; yes | 6 months | 82 | 0.5–5 × 106 CD133+ cells plus CABG vs. placebo plus CABG | Intra-myocardial | MRI | NS | NS | ↓ | NA | NS | NA |
TRIDENT49 Hare et al. | II | No; yes; yes | 12 months | 30 | 20 × 106 vs. 100 × 106 allo-MSCs | TE | CT | Higher dose ↑ | NS | Both ↓ | Both ↑ | Both ↑ (no change in VO2 max) | NS |
RIMECARD50 Bartolucci et al. | I/II | Yes; yes; yes | 12 months | 30 | 1×/kg UC-MSCs vs. placebo | IV | Echo/MRI | ↑ | NS | NA | ↑ | ↑ (improvement in VE/VCO2, no change in VO2 max) | ↑ |
Kastrup et al.51 | I | No; no; no | 6 months | 10 | 100 × 106 cryo preserved adipose-derived MSCs | TE | Echo/CT | ↑ | ↓ | NA | ↑ | ↑ | NS |
ESCORT52e Menasche et al. | I | No; no; no | 12 months | 6 | 5–10 × 106 hESC-derived cardiovascular progenitors embedded in a fibrin patch | Epicardial | Echo/CT | ↑ | ↓ | NA | 4 pts ↑ | 4 pts ↑ | 4 pts ↑ |
RECARDIO53 Bassetti et al. | I | No; no; no | 1 year | 10 | 1–12 × 106 BM-MSCs vs. baseline | TE | Echo/SPECT | NS | NS | NA | ↑ | NS | NS |
II | Yes; yes; yes | 6 months, 4 years | 60 | 77.5 + 67.9 × 106 (inter-quartile range 53.8 × 106) BM-MSCs vs. placebo | TO | MRI/CT | ↑ | ↓ ESV | NS | NS | NS | ↑ | |
II | Yes; yes; yes | 6 months | 142 | Allo CDCs (25 × 106) vs. placebo (2:1 randomization) | IC | MRI | NS | ↓ EDV and ESV | NS | NA | NA | NA | |
I | Not; not; not | 12 months | 14 | 37.5–75 allo CDCs; no control group | IC (3 coronaries) | ??? | ↑ f | ↑ f | ? f | ↑ f | ? f | ↑ f | |
II | Yes; yes; yes | 12 months | 125 | 150 × 106 auto BM-MSCs vs. 5 × 106 auto CPCs vs. both vs. placebo | TO | MRI | NS | NS | NS | NA | NS | MSCs and MSCs+CPCs | |
III | No (sham controlled); yes; yes | At least 12 months (median ∼30 months) | 565 randomized; 535 treated | 150 × 106 BM-derived allo MPCs (selected with anti-STRO-3 antibodies) vs. sham procedure | TO | Echo | ? | ? | ? | ? | ? | ? |
Trial . | Phase . | Placebo controlled; randomized; double-blind . | Follow-up . | n . | Cell type, dose, and treatment groups . | Delivery method . | Endpoint evaluation . | LVEF . | LV volumes . | Scar size . | NYHA class . | Functional capacity . | QoL . |
---|---|---|---|---|---|---|---|---|---|---|---|---|---|
FOCUS CCTRN36 Perin et al. | II | Yes; yes; yes | 6 months | 92 | 100 × 106 BM-MNCs vs. placebo | TE | Echo/SPECT | NS | NS | NS | NS | NS | NA |
POSEIDON37 Hare et al. | I/II | No; yes; no | 13 months | 30 | 20, 100, or 200 × 106 BM-MSCs (allo vs. auto) | TE | MRI | NS | Allo ↓ EDV | Both ↓ | NS | Auto ↑ (VO2 max NS) | Auto ↑ |
CADUCEUS38 Makkar et al. | I | No; yes; no | 6 months | 31 | 12.5–25 × 106 CDCs vs. control | IC | MRI | NS | NS | ↓ | NS | NS | NS |
C-CURE39 Bartunek et al. | II | No; yes; no | 24 months for safety; 6 months for efficacy | 48 | BM-derived cardiopoietic cells plus standard care vs. standard care | TE | Echo | ↑ at 6 months | ↓ ESV at 6 months | NA | ↑ at 6 months | ↑ at 6 months | ↑ at 6 months |
IMPACT-DCM and Catheter-DCM40,a | II | No; yes; no | 12 months | 39 and 22 | Ixmyelocel-T cellsb (dose unspecified) vs. standard of care | Intra-myocardial (mini-thoracotomy) or TE | Echo/SPECT | NS | NS | NA | ↑ in ischaemic HF | ↑ in ischaemic HF | NS |
PROMETHEUS41 Karantalis et al. | I/II | No; yes; yes | 18 months | 6 | 2 × 107 vs. 2 × 108 auto BM-MSCs vs. baseline | TE (non-revascularized segments) | MRI | ↑ with both cell doses | NA | ↓ ESV with both cell doses | NA | NA | NA |
TAC-HFT42 Heldman et al. | I/II | Yes; yes; yes | 12 months | 65 | 100 × 106 BM-MSCs vs. 100 × 106 BM-MNCs vs. placebo | TE | MRI/CT | NS | NS | MSC: ↓ | NS | MSCs ↑ | Both ↑ |
PRECISE43 Perin et al. | I | Yes; yes; yes | 36 months | 27 | 0.4 × 106, 0.8 × 106 or 1.2 × 106 ADRCs vs. placebo (3:1 randomization)c | TE | MRI/Echo | NS | NS | NA | NA | Preserved with ADRCs (VO2 max at 18 months) | NA |
MESAMI I44 Guijarro et al. | I | No; no; no | 2 years | 10 | 61.5 × 106 BM-MSCs vs. baseline | TE | Echo | ↑ | ↓ | NA | ↑ | ↑ (including VO2 max) | NS |
ixCELL-DCM45a Patel et al. | IIb | Yes; yes; yes | 12 months | 126 | 40–200 × 106 Ixmyelocel-T cellsb vs. placebo | TE | Echo | NS | NS | NA | NS | NS | NA |
ATHENA trials46 Henry et al. | II | Yes; yes; yes | 12 months | 31 | 80 × 106 vs. 40 × 106 ARDCs vs. placebo | TE | Echo | NS | NS | NA | ↑ | ↑ (including VO2 max) | ↑ |
CHART-147 Bartunek et al. | III | No (Sham); yes; yes | 39 weeks | 315 | 9.7 × 108–1.2 × 109 cardiopoietic cells vs. sham | TE | Echo | NS | NS | NA | NA | NS | NS |
PERFECT48 Steinhoff et al. | III | Yes; yes; yes | 6 months | 82 | 0.5–5 × 106 CD133+ cells plus CABG vs. placebo plus CABG | Intra-myocardial | MRI | NS | NS | ↓ | NA | NS | NA |
TRIDENT49 Hare et al. | II | No; yes; yes | 12 months | 30 | 20 × 106 vs. 100 × 106 allo-MSCs | TE | CT | Higher dose ↑ | NS | Both ↓ | Both ↑ | Both ↑ (no change in VO2 max) | NS |
RIMECARD50 Bartolucci et al. | I/II | Yes; yes; yes | 12 months | 30 | 1×/kg UC-MSCs vs. placebo | IV | Echo/MRI | ↑ | NS | NA | ↑ | ↑ (improvement in VE/VCO2, no change in VO2 max) | ↑ |
Kastrup et al.51 | I | No; no; no | 6 months | 10 | 100 × 106 cryo preserved adipose-derived MSCs | TE | Echo/CT | ↑ | ↓ | NA | ↑ | ↑ | NS |
ESCORT52e Menasche et al. | I | No; no; no | 12 months | 6 | 5–10 × 106 hESC-derived cardiovascular progenitors embedded in a fibrin patch | Epicardial | Echo/CT | ↑ | ↓ | NA | 4 pts ↑ | 4 pts ↑ | 4 pts ↑ |
RECARDIO53 Bassetti et al. | I | No; no; no | 1 year | 10 | 1–12 × 106 BM-MSCs vs. baseline | TE | Echo/SPECT | NS | NS | NA | ↑ | NS | NS |
II | Yes; yes; yes | 6 months, 4 years | 60 | 77.5 + 67.9 × 106 (inter-quartile range 53.8 × 106) BM-MSCs vs. placebo | TO | MRI/CT | ↑ | ↓ ESV | NS | NS | NS | ↑ | |
II | Yes; yes; yes | 6 months | 142 | Allo CDCs (25 × 106) vs. placebo (2:1 randomization) | IC | MRI | NS | ↓ EDV and ESV | NS | NA | NA | NA | |
I | Not; not; not | 12 months | 14 | 37.5–75 allo CDCs; no control group | IC (3 coronaries) | ??? | ↑ f | ↑ f | ? f | ↑ f | ? f | ↑ f | |
II | Yes; yes; yes | 12 months | 125 | 150 × 106 auto BM-MSCs vs. 5 × 106 auto CPCs vs. both vs. placebo | TO | MRI | NS | NS | NS | NA | NS | MSCs and MSCs+CPCs | |
III | No (sham controlled); yes; yes | At least 12 months (median ∼30 months) | 565 randomized; 535 treated | 150 × 106 BM-derived allo MPCs (selected with anti-STRO-3 antibodies) vs. sham procedure | TO | Echo | ? | ? | ? | ? | ? | ? |
Trials are listed in chronological order of publication.
↑indicates increase; ↓, decrease; allo, allogeneic; ARDCs, adipose-derived regenerative cells; auto, autologous; BMMNCs, bone marrow mononuclear cells; BM-MSCs, bone marrow-derived mesenchymal stem cells; CDCs, cardiosphere-derived cells; CPCs, cardiopoietic cells; CT, computed tomography; Echo, echocardiography; EDV, end-diastolic volume; EF, ejection fraction; ESV, end-systolic volume; hESCs, human embryonic stem cells; IC, intracoronary; IV, intravenous; LV, left ventricle; LVEF, left ventricular ejection fraction; MRI, magnetic resonance imaging; n, number of patients; NA, not assessed; NS, not significant; NYHA, New York Heart Association; pt, patient; QoL, quality of life; SPECT, single-photon emission tomography; TE, transendocardial; UC-MSCs, umbilical cord-derived mesenchymal stem cells; VCO2, volume of exhaled carbon dioxide; VE, ventilation; VO2 max, maximal oxygen consumption.
ixCELL-DCM showed significant reduction of the primary endpoint (all-cause deaths and cardiovascular admissions to hospital) in patients who received ixmyelocel-T.
Ixmyelocel-T is a product containing allogenic bone marrow cells produced by selectively expanding two key types of bone marrow mononuclear cells: CD90+ mesenchymal stem cells and CD45+ CD14+ auto-fluorescent+ activated macrophages.
Three-dose escalation of ADRCs; 3rd escalation dose was not used.
Trial stopped prematurely due to futility; NT-proBNP levels reduced by CDCs at 6 months.
Patients received immunosuppression for 1 month. One patient died early post-operatively from treatment-unrelated comorbidities. All others had uneventful recoveries. No tumour was detected during follow-up, and none of the patients presented with arrhythmias. Three patients developed clinically silent alloimmunization. One patient had only followed up for 6 months.
Data not interpretable due to lack of control group.
In CONCERT-HF, there was a significant reduction in HF-MACE (all cause death, HF admission, or HF exacerbation) in patients randomized to CPCs alone or CPCs + MSCs.
In DREAM-HF, there was a significant reduction in MI or stroke and in MACE (cardiac death, MI, or stroke) among all patients; there was also a reduction in cardiac death in NYHA class II patients; other (secondary) endpoints have not been released yet.
3.1.2 Non-ischaemic cardiomyopathy
Similar results have been obtained in the setting of non-ischaemic cardiomyopathy (Table2). The positive results of an early, open-label pilot study (TOPCARE-DCM), published in 2009, that assessed the effects of intracoronary infusion of BM-MNCs at 3 months77 were not confirmed in the subsequent larger, double-blind MiHeart study, published in 2017.70 MiHeart was a randomized, double-blind, placebo-controlled trial of BM-MNCs, given intracoronarily, in 160 patients with non-ischaemic dilated cardiomyopathy. At 12 months, there were no differences in LVEF or LV volumes (assessed by echocardiography), functional capacity, or quality of life between the two treatment groups. Similar negative results were reported in the Chagas disease arm of the MiHeart study, published in 2012,78 and in the study by Xiao et al.,73 published in 2017, where 53 patients with non-ischaemic cardiomyopathy were given BM-MNCs or BM-MSCs intracoronarily; at 12 months, LVEF [assessed by magnetic resonance imaging (MRI)] was improved in the BM-MSC group but not in the BM-MNC group.
Trial . | Phase . | Placebo controlled; randomized; double-blind . | Follow-up . | n . | Cell type, dose, and treatment groups . | Delivery method . | Endpoint evaluation . | LVEF . | LV volumes . | NYHA class . | Functional capacity . | Qol . |
---|---|---|---|---|---|---|---|---|---|---|---|---|
Vrtovec et al.67 | II | No; yes; no | 12 months | 55 | 123 ± 23 × 106 CD34+ cells from peripheral blood after G-CSF vs. control | IC | Echo | ↑ | NS | NA | ↑ | NA |
Vrtovec et al.68,b | II | No; yes; no | 5 years | 110 | 113 ± 26 × 106 CD34+cells from peripheral blood after G-CSF vs. control | IC | Echo | ↑ | NS | NA | ↑ | NA |
Vrtovec et al.69 | II | No; yes; yes | 6 months | 40 | 105 ± 31 × 106 CD34+ cells from peripheral blood after G-CSF, TE route, vs. 103 ± 27 × 106 CD34+ cells after G-CSF, IC route | TE, IC | Echo | ↑ (TE>IC) | NS | NA | ↑ (TE>IC) | NA |
MiHeart70 Martino et al. | III | Yes; yes; yes | 12 months | 160 | BM-MNCs vs. placebo | IC | Echo | NS | NS | NS | NS | NS |
REGENERATE-DCM9 Hamshere et al. | II | Yes; yes; yes | 12 months | 60 | SC placebo vs. SC G-CSF vs. SC G-CSF and IC placebo vs. SC G-CSF and IC 216.0 × 106 ± 221.8 BM-MNCs | IC | MRI/CT | BM-MNCs ↑ | NS | BM-MNCs ↑ | BM-MNCs ↑ (↑ VO2 max) | BM-MNCs ↑ |
Butler et al.71 | IIa | Yes; yes; no | 3 months (crossover) | 22 | Ischaemia-tolerant allo BM-MSCs 1.5 × 106/kg vs. placebo | IV | MRI / Echo | NS | NS | ↑ | ↑ | ↑ |
Poseidon DCM72 Hare et al. | I/II | No; yes; no | 12 months | 37 | 1 × 106 allo BM-MSCs vs. 1 × 106 auto BM-MSCs | TO | MRI/CT | ↑ Allo | NS | NS | ↑ Allo (VO 2 max NS) | ↑ Allo |
Xiao et al.73 | ? | Yes; yes; yes | 12 months | 53 | 5.1 × 108 BM-MNCs vs. 4.9 × 108 BM-MSCs vs. placebo | IC | MRI | ↑ BM-MSCs (both groups ↑ @ 3 months) | NS | ↑ BM-MSCs | NA | NA |
REMEDIUM74 Vrtovec et al. | II/III | No; yes; no | 12 months | 60 | Two doses 6 months apart vs. single dose of 80 × 106 peripheral blood CD 34+ cells after G-CSF | TO | Echo | NS | NS | NA | NS | NA |
I | Yes; yes; yes | 12 months | 37 | 100 × 106 allo BM-MSCs vs. placebo (cancer survivors with anthracycline-induced cardiomyopathy) | TO | MRI | NS | NS | NA | ↑ | ↑ |
Trial . | Phase . | Placebo controlled; randomized; double-blind . | Follow-up . | n . | Cell type, dose, and treatment groups . | Delivery method . | Endpoint evaluation . | LVEF . | LV volumes . | NYHA class . | Functional capacity . | Qol . |
---|---|---|---|---|---|---|---|---|---|---|---|---|
Vrtovec et al.67 | II | No; yes; no | 12 months | 55 | 123 ± 23 × 106 CD34+ cells from peripheral blood after G-CSF vs. control | IC | Echo | ↑ | NS | NA | ↑ | NA |
Vrtovec et al.68,b | II | No; yes; no | 5 years | 110 | 113 ± 26 × 106 CD34+cells from peripheral blood after G-CSF vs. control | IC | Echo | ↑ | NS | NA | ↑ | NA |
Vrtovec et al.69 | II | No; yes; yes | 6 months | 40 | 105 ± 31 × 106 CD34+ cells from peripheral blood after G-CSF, TE route, vs. 103 ± 27 × 106 CD34+ cells after G-CSF, IC route | TE, IC | Echo | ↑ (TE>IC) | NS | NA | ↑ (TE>IC) | NA |
MiHeart70 Martino et al. | III | Yes; yes; yes | 12 months | 160 | BM-MNCs vs. placebo | IC | Echo | NS | NS | NS | NS | NS |
REGENERATE-DCM9 Hamshere et al. | II | Yes; yes; yes | 12 months | 60 | SC placebo vs. SC G-CSF vs. SC G-CSF and IC placebo vs. SC G-CSF and IC 216.0 × 106 ± 221.8 BM-MNCs | IC | MRI/CT | BM-MNCs ↑ | NS | BM-MNCs ↑ | BM-MNCs ↑ (↑ VO2 max) | BM-MNCs ↑ |
Butler et al.71 | IIa | Yes; yes; no | 3 months (crossover) | 22 | Ischaemia-tolerant allo BM-MSCs 1.5 × 106/kg vs. placebo | IV | MRI / Echo | NS | NS | ↑ | ↑ | ↑ |
Poseidon DCM72 Hare et al. | I/II | No; yes; no | 12 months | 37 | 1 × 106 allo BM-MSCs vs. 1 × 106 auto BM-MSCs | TO | MRI/CT | ↑ Allo | NS | NS | ↑ Allo (VO 2 max NS) | ↑ Allo |
Xiao et al.73 | ? | Yes; yes; yes | 12 months | 53 | 5.1 × 108 BM-MNCs vs. 4.9 × 108 BM-MSCs vs. placebo | IC | MRI | ↑ BM-MSCs (both groups ↑ @ 3 months) | NS | ↑ BM-MSCs | NA | NA |
REMEDIUM74 Vrtovec et al. | II/III | No; yes; no | 12 months | 60 | Two doses 6 months apart vs. single dose of 80 × 106 peripheral blood CD 34+ cells after G-CSF | TO | Echo | NS | NS | NA | NS | NA |
I | Yes; yes; yes | 12 months | 37 | 100 × 106 allo BM-MSCs vs. placebo (cancer survivors with anthracycline-induced cardiomyopathy) | TO | MRI | NS | NS | NA | ↑ | ↑ |
Trials are listed in chronological order of publication.
↑indicates increase; ↓, decrease; allo, allogeneic; auto, autologous; BMMNCs, bone marrow mononuclear cells; BM-MSCs, bone marrow-derived mesenchymal stem cells; CT, computed tomography; Echo, echocardiography; EDV, end-diastolic volume; EF, ejection fraction; ESV, end-systolic volume; G-CSF, granulocyte colony-stimulating factor; IC, intracoronary; IV, intravenous; LV, left ventricle; LVEF, left ventricular ejection fraction; MRI, magnetic resonance imaging; n, number of patients; NA, not assessed; NS, not significant; NYHA, New York Heart Association; QoL, quality of life; SC, subcutaneous; TE, transendocardial; VO2 max, maximal oxygen consumption.
DREAM-HF (detailed in Table 1) included both ischaemic and non-ischaemic cardiomyopathy.
Five-year survival as evaluated by Kaplan–Meier analysis was 2.3 times higher in the SC group than in controls (P = 0.015).
Trial . | Phase . | Placebo controlled; randomized; double-blind . | Follow-up . | n . | Cell type, dose, and treatment groups . | Delivery method . | Endpoint evaluation . | LVEF . | LV volumes . | NYHA class . | Functional capacity . | Qol . |
---|---|---|---|---|---|---|---|---|---|---|---|---|
Vrtovec et al.67 | II | No; yes; no | 12 months | 55 | 123 ± 23 × 106 CD34+ cells from peripheral blood after G-CSF vs. control | IC | Echo | ↑ | NS | NA | ↑ | NA |
Vrtovec et al.68,b | II | No; yes; no | 5 years | 110 | 113 ± 26 × 106 CD34+cells from peripheral blood after G-CSF vs. control | IC | Echo | ↑ | NS | NA | ↑ | NA |
Vrtovec et al.69 | II | No; yes; yes | 6 months | 40 | 105 ± 31 × 106 CD34+ cells from peripheral blood after G-CSF, TE route, vs. 103 ± 27 × 106 CD34+ cells after G-CSF, IC route | TE, IC | Echo | ↑ (TE>IC) | NS | NA | ↑ (TE>IC) | NA |
MiHeart70 Martino et al. | III | Yes; yes; yes | 12 months | 160 | BM-MNCs vs. placebo | IC | Echo | NS | NS | NS | NS | NS |
REGENERATE-DCM9 Hamshere et al. | II | Yes; yes; yes | 12 months | 60 | SC placebo vs. SC G-CSF vs. SC G-CSF and IC placebo vs. SC G-CSF and IC 216.0 × 106 ± 221.8 BM-MNCs | IC | MRI/CT | BM-MNCs ↑ | NS | BM-MNCs ↑ | BM-MNCs ↑ (↑ VO2 max) | BM-MNCs ↑ |
Butler et al.71 | IIa | Yes; yes; no | 3 months (crossover) | 22 | Ischaemia-tolerant allo BM-MSCs 1.5 × 106/kg vs. placebo | IV | MRI / Echo | NS | NS | ↑ | ↑ | ↑ |
Poseidon DCM72 Hare et al. | I/II | No; yes; no | 12 months | 37 | 1 × 106 allo BM-MSCs vs. 1 × 106 auto BM-MSCs | TO | MRI/CT | ↑ Allo | NS | NS | ↑ Allo (VO 2 max NS) | ↑ Allo |
Xiao et al.73 | ? | Yes; yes; yes | 12 months | 53 | 5.1 × 108 BM-MNCs vs. 4.9 × 108 BM-MSCs vs. placebo | IC | MRI | ↑ BM-MSCs (both groups ↑ @ 3 months) | NS | ↑ BM-MSCs | NA | NA |
REMEDIUM74 Vrtovec et al. | II/III | No; yes; no | 12 months | 60 | Two doses 6 months apart vs. single dose of 80 × 106 peripheral blood CD 34+ cells after G-CSF | TO | Echo | NS | NS | NA | NS | NA |
I | Yes; yes; yes | 12 months | 37 | 100 × 106 allo BM-MSCs vs. placebo (cancer survivors with anthracycline-induced cardiomyopathy) | TO | MRI | NS | NS | NA | ↑ | ↑ |
Trial . | Phase . | Placebo controlled; randomized; double-blind . | Follow-up . | n . | Cell type, dose, and treatment groups . | Delivery method . | Endpoint evaluation . | LVEF . | LV volumes . | NYHA class . | Functional capacity . | Qol . |
---|---|---|---|---|---|---|---|---|---|---|---|---|
Vrtovec et al.67 | II | No; yes; no | 12 months | 55 | 123 ± 23 × 106 CD34+ cells from peripheral blood after G-CSF vs. control | IC | Echo | ↑ | NS | NA | ↑ | NA |
Vrtovec et al.68,b | II | No; yes; no | 5 years | 110 | 113 ± 26 × 106 CD34+cells from peripheral blood after G-CSF vs. control | IC | Echo | ↑ | NS | NA | ↑ | NA |
Vrtovec et al.69 | II | No; yes; yes | 6 months | 40 | 105 ± 31 × 106 CD34+ cells from peripheral blood after G-CSF, TE route, vs. 103 ± 27 × 106 CD34+ cells after G-CSF, IC route | TE, IC | Echo | ↑ (TE>IC) | NS | NA | ↑ (TE>IC) | NA |
MiHeart70 Martino et al. | III | Yes; yes; yes | 12 months | 160 | BM-MNCs vs. placebo | IC | Echo | NS | NS | NS | NS | NS |
REGENERATE-DCM9 Hamshere et al. | II | Yes; yes; yes | 12 months | 60 | SC placebo vs. SC G-CSF vs. SC G-CSF and IC placebo vs. SC G-CSF and IC 216.0 × 106 ± 221.8 BM-MNCs | IC | MRI/CT | BM-MNCs ↑ | NS | BM-MNCs ↑ | BM-MNCs ↑ (↑ VO2 max) | BM-MNCs ↑ |
Butler et al.71 | IIa | Yes; yes; no | 3 months (crossover) | 22 | Ischaemia-tolerant allo BM-MSCs 1.5 × 106/kg vs. placebo | IV | MRI / Echo | NS | NS | ↑ | ↑ | ↑ |
Poseidon DCM72 Hare et al. | I/II | No; yes; no | 12 months | 37 | 1 × 106 allo BM-MSCs vs. 1 × 106 auto BM-MSCs | TO | MRI/CT | ↑ Allo | NS | NS | ↑ Allo (VO 2 max NS) | ↑ Allo |
Xiao et al.73 | ? | Yes; yes; yes | 12 months | 53 | 5.1 × 108 BM-MNCs vs. 4.9 × 108 BM-MSCs vs. placebo | IC | MRI | ↑ BM-MSCs (both groups ↑ @ 3 months) | NS | ↑ BM-MSCs | NA | NA |
REMEDIUM74 Vrtovec et al. | II/III | No; yes; no | 12 months | 60 | Two doses 6 months apart vs. single dose of 80 × 106 peripheral blood CD 34+ cells after G-CSF | TO | Echo | NS | NS | NA | NS | NA |
I | Yes; yes; yes | 12 months | 37 | 100 × 106 allo BM-MSCs vs. placebo (cancer survivors with anthracycline-induced cardiomyopathy) | TO | MRI | NS | NS | NA | ↑ | ↑ |
Trials are listed in chronological order of publication.
↑indicates increase; ↓, decrease; allo, allogeneic; auto, autologous; BMMNCs, bone marrow mononuclear cells; BM-MSCs, bone marrow-derived mesenchymal stem cells; CT, computed tomography; Echo, echocardiography; EDV, end-diastolic volume; EF, ejection fraction; ESV, end-systolic volume; G-CSF, granulocyte colony-stimulating factor; IC, intracoronary; IV, intravenous; LV, left ventricle; LVEF, left ventricular ejection fraction; MRI, magnetic resonance imaging; n, number of patients; NA, not assessed; NS, not significant; NYHA, New York Heart Association; QoL, quality of life; SC, subcutaneous; TE, transendocardial; VO2 max, maximal oxygen consumption.
DREAM-HF (detailed in Table 1) included both ischaemic and non-ischaemic cardiomyopathy.
Five-year survival as evaluated by Kaplan–Meier analysis was 2.3 times higher in the SC group than in controls (P = 0.015).
The REGENERATE-DCM study, published in 2015, differs from the above trials because it tested the combination of BM-MNCs and G-CSF.9 In this randomized, double-blind, placebo-controlled, Phase II study, patients with non-ischaemic cardiomyopathy received subcutaneous injections of G-CSF for 5 days; in addition, they were given either autologous BM-MNCs (15 patients) or serum (15 patients) intracoronarily (all three coronary arteries). The trial met its primary endpoint, which was the change in LVEF from baseline to 3 months. The group treated with G-CSF and BM-MNCs exhibited a significant (5.4%) increase in LVEF at 3 months, which was maintained at 1 year and was associated with significant improvements in New York Heart Association (NYHA) class, exercise capacity, and quality of life, and a decrease in N-terminal pro-brain natriuretic peptide (NT-proBNP) at 1 year. In contrast, there was no improvement in any of these endpoints in the group treated with G-CSF and serum. These results demonstrate that the combination of intracoronary BM-MNCs and G-CSF is superior to G-CSF alone, but do not clarify whether BM-MNCs alone would be efficacious.
In summary, current evidence indicates that unfractionated BM-MNCs are not effective in either ischaemic or non-ischaemic HF. Consequently, investigation of these cells has been largely abandoned, and the focus has turned to specific cell types, such as BM-MSCs.
3.2 Mesenchymal stromal cells
3.2.1 Definition and properties of MSCs
MSCs are defined as cells that (i) express the surface markers CD105, CD73, and CD90, (ii) lack haematopoietic markers (CD45, CD34, CD14/CD11b, CD79 alpha, CD19, and HLA-DR), (iii) adhere to plastic in permissive culture conditions, and (iv) are able to differentiate into cells of mesodermal origin, including chondrocytes, osteoblasts, adipocytes, and fibroblasts.79 Although differentiation of MSCs into skeletal myocytes, endothelial cells, and cardiomyocytes has been reported,80–83 it is highly controversial. MSCs can be isolated from various tissues including BM, adipose tissue,84 umbilical cord (UC) matrix (Wharton’s jelly),85 UC blood,86 and dental pulp. We have recently demonstrated that MSCs with reparative properties can be isolated from myocardium.18 MSCs are rare cells: for example, their prevalence is ∼1 in 10–15 000 cells in BM and ∼1 in 100 000 cells in peripheral blood.87
MSCs have many characteristics that make them attractive candidates for cell therapy in HF.88 They are relatively easy to isolate and expand. They exert powerful paracrine actions via secretion of a wide variety of antifibrotic, antiapoptotic, and proangiogenic factors (including cytokines, growth factors, miRs, and bioactive lipids), release of extracellular vesicles, transfer of mitochondria,89 and production of matrix metalloproteinases and other molecules that favourably affect the composition of the extracellular matrix90,91 (reviewed in refs.88,91) In addition, MSCs possess anti-inflammatory and immunosuppressive properties.88,92–94 All of these actions would be expected to be beneficial in chronic HF, where increased apoptosis,95 reduced vasculogenesis,96 chronic, low-grade inflammation,29,30 and progressive deposition of interstitial collagen31,97 are thought to play an important role in the progression of the disease. Indeed, experimental studies have demonstrated that MSCs exert antifibrotic, immunomodulatory, and pro-angiogenic effects in vivo in experimental models of HF.23,25,88 Clinical trials have shown systemic anti-inflammatory and immunomodulatory actions of i.v. MSC therapy in various conditions, including HF,72 graft-vs.-host disease (GVHD), multiple sclerosis, amyotrophic lateral sclerosis, systemic lupus erythematosus, chronic obstructive pulmonary disease, and Crohn’s disease.98–103
Although early studies used autologous MSCs, the field of cell therapy has now shifted decisively towards allogeneic MSCs (and allogeneic cells in general). Allogeneic cell products offer many advantages, including freedom from patient-to-patient variability, greater cost-effectiveness for large scale production, and the ability to use cells from young, healthy donors, thereby avoiding the functional impairment of cell products obtained from elderly patients with multiple co-morbidities.104–107 The fact that MSCs lack major histocompatibility type II antigens, coupled with their ability to secrete anti-inflammatory factors, makes them particularly suitable for allogeneic cell therapy. Numerous clinical trials have shown that infusion of allogeneic MSCs is safe and does not elicit immune rejection against the transplanted cells,37,50,72,98,99,102,103,108–115 even when repeated multiple times.98,99,102,103,109–112
For all of the above reasons, among the various cell types tested in HF, MSCs (initially autologous, more recently allogeneic) have been studied most intensely and hold the greatest potential for clinical application in the near future.
3.2.2 Bone marrow-derived MSCs
Although their precise mechanism of action remains unclear,25 the use of BM-derived MSCs (BM-MSCs) in chronic HF has yielded promising results both at the preclinical and clinical levels,2,8 and translation has advanced quickly. At the preclinical level, considerable evidence supports the ability of autologous or allogeneic BM-MSCs to improve cardiac function and decrease scar size in small and large animal models of chronic ischaemic cardiomyopathy.2,23 This robust body of evidence has provided the groundwork for a bevy of clinical trials of BM-MSCs in patients with ischaemic and non-ischaemic HF, which are summarized below and in Tables 1 and 2. Two trials44,53 are not discussed here because they were not randomized, not blinded, and not placebo-controlled. In addition, the PROMETHEUS41 and PERFECT 48 trials are not discussed because intramyocardial injection of cells cell was performed during coronary artery bypass graft surgery, making it difficult to separate the effects of one intervention from the other.
3.2.2.1 Ischaemic cardiomyopathy
The POSEIDON trial, published in 2012, was the first study to compare autologous and allogeneic MSCs in patients with ischaemic HF (Table 1).37 In this randomized Phase I/II study, three doses of autologous or allogeneic BM-MSCs (20, 100, and 200 × 106 cells) were given transendocardially in 30 subjects. At 12 months, both allogeneic and autologous MSCs reduced scar size by 33% and improved the sphericity index, suggesting improved LV remodelling. Allogeneic cells, however, appeared to be more effective in that they significantly reduced LV end-diastolic volume (LVEDV) whereas autologous cells did not. POSEIDON was important because it was the first trial to support the concept that allogeneic MSCs may be superior to autologous MSCs. The limitations were the small sample size and the lack of a placebo control group.41
A significant advance in cell therapy for HF came with TAC-HFT, published in 2014, a Phase II randomized, double-blind, placebo-controlled trial that compared the effects of 100 × 106 autologous BM-MNCs, autologous 100 × 106 BM-MSCs, or placebo, given transendocardially, in 65 patients with ischaemic HF.42 At 12 months after treatment, there was no improvement in LV volumes or LVEF in either cell-treated group; however, quality of life (measured by the MLHFQ score) improved with both BM-MNCs and BM-MSCs. BM-MSCs (but not BM-MNCs) also produced a reduction in scar size (−19%) and an increase in the 6-min walk distance. Overall, the results of TAC-HFT suggest that administration of BM-MSCs is therapeutically more effective than that of BM-MNCs, possibly because of greater antifibrotic activity, leading to scar size reduction.
An important clinical trial of MSCs in chronic HF is MSC-HF, a randomized, double-blind, placebo-controlled, Phase II study in which autologous BM-MSCs were injected transendocardially in 60 patients with ischaemic HF; the 6-month results were published in 2015,54 and the 4-year follow-up in 2020.55 At 12 months after MSC administration, patients exhibited a highly significant improvement in the primary endpoint (change in LVESV) relative to placebo (−17 mL; P < 0.0002), which was associated with a highly significant improvement in LVEF (+6.2 units; P < 0.0001), myocardial mass (+9.8 g; P = 0.09), and quality of life (Figure 1). The long-term follow-up demonstrated that at four years the incidence of angina was significantly reduced in the MSC group.55 MSC-HF is notable because it met its primary endpoint.4 The results of this well-designed trial provide strong evidence supporting the utility of cell therapy in HF. It should also be noted that MSC-HF utilized autologous MSCs. As discussed above, allogeneic MSCs obtained from young, healthy donors may be more effective, a concept supported by the results of both POSEIDON37 and POSEIDON-DCM.72 Thus, it is possible that the beneficial effects reported in the MSC-HF trial with autologous MSCs could be even greater with the use of allogeneic MSCs.
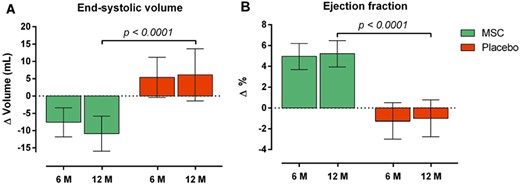
Effect of autologous BM-MSCs on LV end-systolic volume (A) and ejection fraction (B) in the MSC-HF trial. Reproduced with permission from ref.55
Since patients received different numbers of BM-MSCs, this trial provided information regarding the dose-response relationship.54,55 When patients were divided into tertiles based on the number of BM-MSCs injected, significantly greater reductions in LVESV and LVEF were observed with doses >83 × 106 million cells (upper tertile) compared with the lower tertile (<43 × 106 cells), suggesting a positive relationship between number of BM-MSCs administered and outcome. This conclusion is corroborated by the results of the TRIDENT study, published in 2017, which was a randomized, double-blind trial that compared transendocardial administration of 20 × 106 or 100 × 106 allogeneic BM-MSCs in 30 patients with ischaemic HF.49 After 12 months, both doses were associated with improvement in 6-min walk distance and reduction in scar size; however, only the higher dose of 100 × 106 cells improved LVEF (+3.7%), whereas the 20 × 106 cell dose produced no change, suggesting the therapeutic superiority of the higher dose. Taken together, the results of MSC-HF and TRIDENT support the use of doses of BM-MSCs in the 100–150 × 106 range when cells are given transendocardially. For MSC-HF, however, an alternative explanation is possible, namely, that the greater efficacy of higher cell doses may have reflected the greater proliferation and therapeutic efficacy of the cells.
The recently released results of CONCERT-HF (NCT02501811) [Table 1; see below section on c-kit-positive cardiac cells (CPCs) for details] show that administration of autologous BM-MSCs to patients with chronic ischaemic HF did not improve LV function or reduce scar size at 12 months but it did improve quality of life (measured by the MLHFQ score).60 These beneficial effects of BM-MSCs on quality of life despite no improvement in LV function are similar to those observed in TAC-HFT42 but differ from those in MSC-HF55; the reasons for this apparent discrepancy are unclear.
The largest trial of cell therapy for HF to date is, by far, DREAM-HF (NCT 02032004).62 Its results have recently been released in part by the sponsor.63 DREAM-HF was a randomized, double-blind, sham-controlled, Phase III study conducted in 55 sites across North America. A total of 565 patients with ischaemic or non-ischaemic HF were randomized; of these, 537 received either transendocardial injection of 150 × 106 allogeneic BM-MSCs (selected with anti-STRO-3 antibodies) (n = 261) or a sham-control catheterization procedure (no placebo) (n = 276) and were followed for a median of ∼30 months. The as-treated results show that the trial did not meet its primary endpoint, i.e. a reduction in recurrent HF-related hospitalizations. However, there were significant effects on other pre-specified endpoints. Patients treated with MSCs exhibited a 60% reduction in MI or stroke (P = 0.002) and a 30% reduction in overall major adverse cardiac events (MACE) (cardiac death, MI, or stroke) (P = 0.027) (Figure 2). In NYHA class II patients, MSC treatment was associated with a 60% reduction in cardiac death (P = 0.037). Covariate regression analyses suggested that elevated baseline levels of C-reactive protein, an important biomarker of systemic inflammation, predicted the effects of MSCs both on MACE in the entire patient cohort and on cardiac death in NYHA class II patients, which is consistent with the proposed anti-inflammatory mechanism of action of MSCs.62
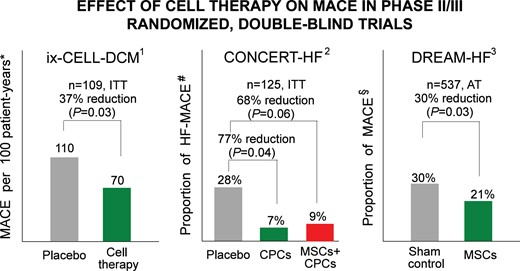
Randomized, double-blind, Phase II or III trials that have found improvement in a hard endpoint (reduction in MACE) in patients treated with cell therapy despite lack of improvement in LV function. *MACE: all-cause death, cardiovascular hospitalization, or HF exacerbation. #HF-MACE: all-cause death, HF hospitalization, or HF exacerbation. §MACE: cardiovascular death, MI, or stroke. AT, as-treated analysis; ITT, intention-to-treat analysis. 1Ref.161; 2Ref.92; 3Ref.94
The long-awaited results of DREAM-HF mark a pivotal point in the development of cell therapy. For the first time, a large, well-designed Phase III trial has demonstrated beneficial effects of cell therapy on hard clinical endpoints (cardiac death, MI, stroke) in HF patients. Of note, these effects occurred after a single dose of cells and in patients on maximal guideline-directed medical therapy for HF. Although HF hospitalizations (the primary endpoint) were not decreased significantly, the reduction in cardiac death, stroke, and MI (secondary endpoints) represents an important clinical outcome that should be tested as the primary endpoint in a new trial. If this new trial confirms the reduction in MACE observed in DREAM-HF, it would lead to FDA approval of MSCs for HF. On the other hand, several caveats must be kept in mind. At the time of this writing, the results of DREAM-HF have not been published or presented at scientific meetings, and only part of these results have been released by the sponsor. In particular, intention-to-treat results are not available. Whether secondary endpoints, such as LV volumes and function, NT-proBNP, and other clinical outcomes (e.g. functional capacity and quality of life) were improved by MSCs is not known. Nor is there a clear mechanism for a decrease in MACE without a reduction in HF-related hospitalizations. Nevertheless, the results of DREAM-HF are exciting and should rekindle interest in cell therapy among physicians, investigators, biomedical companies, and granting agencies. There is no precedent for a single dose of a therapy to produce a long-lasting improvement in clinical outcome over the subsequent 30 months.
3.2.2.2 Non-ischaemic cardiomyopathy
As mentioned, DREAM-HF included both ischaemic and non-ischaemic cardiomyopathy patients (Table 2). Several trials of BM-MSCs in non-ischaemic cardiomyopathy have been reported (Table 2). POSEIDON-DCM, published in 2017, was a randomized trial that compared the effects of autologous and allogeneic BM-MSCs, administered transendocardially, in 37 patients with idiopathic non-ischaemic cardiomyopathy.72 At 12 months after treatment, patients given allogeneic BM-MSCs, but not those given autologous BM-MSCs, exhibited a significant increase in LVEF (+8 EF units), which was associated with a greater improvement in the 6-min walking distance and incidence of MACE than in patients receiving autologous BM-MSCs. The increase in LVEF was not accompanied by a reduction in LVEDV, indicating that allogeneic BM-MSCs did not affect LV remodelling. Plasma tumour necrosis factor-alpha levels decreased with both autologous and allogeneic BM-MSCs, but the reduction was greater with the former. Similar to the POSEIDON study in ischaemic cardiomyopathy,37 POSEIDON-DCM supports the therapeutic superiority of allogeneic BM-MSCs over autologous BM-MSCs; however, because of the lack of a control group, conclusions regarding the therapeutic efficacy of BM-MSC therapy are not possible.72
In 2017, Butler et al.71 reported the results of a trial in which patients with non-ischaemic cardiomyopathy were randomized to i.v. BM-MSCs (n = 10) or placebo (n = 12) (Table 2). At 3 months, there was improvement in LV function, 6-min walking distance, and KCCQ score in treated but not in control patients, suggesting that i.v. administration of MSCs may improve clinical parameters in non-ischaemic cardiomyopathy. In addition, there was a reduction in circulating NK cells in treated patients, providing further evidence of systemic immunomodulatory effects of i.v. MSCs, consistent with the effects of i.v. MSCs in murine models of ischaemic cardiomyopathy.116
In the same year, Xiao et al.73 published the results of a randomized, double-blind, placebo-controlled comparison of BM-MSCs, BM-MNCs, and placebo, given intracoronarily, in 53 patients with dilated cardiomyopathy. At 12 months, administration of BM-MSCs, but not BM-MNCs, resulted in an increase in LVEF and NYHA class compared with placebo, again supporting the superiority of the former vs. the latter, in agreement with the TAC-HFT trial in ischaemic cardiomyopathy.42
The recently reported SENECA trial was a Phase I, randomized, placebo-controlled, double-blind, multicentre study of allogeneic BM-MSCs in cancer survivors with anthracycline-induced cardiomyopathy (Table 2).75,76 It was the first trial to use cell therapy in this population. Patients were randomized to transendocardial injection of allogeneic BM-MSCs (n = 14) or placebo (n = 17) and followed for 12 months. There was no safety signal and the protocol was feasible. Although this first-in-human study was small and not powered for efficacy, functional capacity (6-min walking distance) and MLHFQ score were significantly improved in cell-treated patients; other efficacy measures (LV function and volumes, scar size, and NT-proBNP) did not differ significantly.76 SENECA lays the groundwork for larger Phase II trials aimed at assessing the efficacy of cell therapy in patients with anthracycline-induced cardiomyopathy.
Taken together, the trials of BM-MSCs published to date in chronic HF suggest that these cells are safe, that they are superior to BM-MNCs, and that they may improve one or more endpoints including LV function, LV remodelling, quality of life, functional capacity, and/or MACE, particularly in patients with ischaemic cardiomyopathy. However, definitive conclusions must await the publication of DREAM-HF, the release of two ongoing trials of adipose-derived regenerative cells (ARDCs) (Table 3), and possibly further Phase III trials.
Trials . | Phase . | Placebo- controlled; randomized; double-blind . | Follow-up . | n . | Cell type, dose, and treatment groups . | Delivery method . | Participants . | Endpoint . |
---|---|---|---|---|---|---|---|---|
STEM VAD117 | IIa | Yes; yes; yes | 12 months | 30 | Three doses of allo BM-MSCs, 1.5 × 106 cells/kga given 1 month apart | IV | HF (ischaemic or non-ischaemic) requiring LVAD implantation and deemed stable on LVAD | Primary: uncontrolled infection, all-cause mortality. Secondary: RV systolic function, admission for RV failure, 6MWT, reduction in NK cells, change in NT-proBNP |
CardiAMP-HF118 Raval et al. | III | No (sham controlled); yes; yes | 12 months | 250 | 200 × 106 auto BM-MNCs | TO | LVEF 20–40% secondary to remote MI | Primary: 6MWT; secondary: survival, MACE, MLHFQ |
SCIENCE119 | II | Yes; yes; yes | 12 months | 133 | 100 × 106 adipose-derived allo MSCs vs. placebo | TO | Ischaemic cardiomyopathy, EF ≤45% on echo, CT or MRI | Primary: change in LVESV; Secondary: serious adverse events |
Allogenic Stem Cell Therapy in Heart Failure (CSCC_ASCII)120 | II | Yes; yes; yes | 12 months | 81 | 100 × 106 adipose-derived allo MSCs vs. placebo, 2:1 randomization | TO | Ischaemic cardiomyopathy, EF ≤45% | Primary: change in LVESV; secondary: incidence of treatment-emergent adverse events, LVEF, KCCQ, Seattle Angina Questionnaire, 6MWT |
REPEAT121 | II/III | No; yes; no | 5 years | 81 | Autologous BM-MNCs, single dose vs. two doses 4 months apart | IC | Ischaemic cardiomyopathy, EF ≤45% | Primary: mortality at 2 years; Secondary: morbidity efficacy endpoints (cardiac and CV mortality, HF hospitalization, ischaemic CE, CR, cardiac transplantation, VAD, ST, ICD, NYHA status, MLHFQ) and safety endpoints (BE, HE, LTA, new malignancies) |
Trials . | Phase . | Placebo- controlled; randomized; double-blind . | Follow-up . | n . | Cell type, dose, and treatment groups . | Delivery method . | Participants . | Endpoint . |
---|---|---|---|---|---|---|---|---|
STEM VAD117 | IIa | Yes; yes; yes | 12 months | 30 | Three doses of allo BM-MSCs, 1.5 × 106 cells/kga given 1 month apart | IV | HF (ischaemic or non-ischaemic) requiring LVAD implantation and deemed stable on LVAD | Primary: uncontrolled infection, all-cause mortality. Secondary: RV systolic function, admission for RV failure, 6MWT, reduction in NK cells, change in NT-proBNP |
CardiAMP-HF118 Raval et al. | III | No (sham controlled); yes; yes | 12 months | 250 | 200 × 106 auto BM-MNCs | TO | LVEF 20–40% secondary to remote MI | Primary: 6MWT; secondary: survival, MACE, MLHFQ |
SCIENCE119 | II | Yes; yes; yes | 12 months | 133 | 100 × 106 adipose-derived allo MSCs vs. placebo | TO | Ischaemic cardiomyopathy, EF ≤45% on echo, CT or MRI | Primary: change in LVESV; Secondary: serious adverse events |
Allogenic Stem Cell Therapy in Heart Failure (CSCC_ASCII)120 | II | Yes; yes; yes | 12 months | 81 | 100 × 106 adipose-derived allo MSCs vs. placebo, 2:1 randomization | TO | Ischaemic cardiomyopathy, EF ≤45% | Primary: change in LVESV; secondary: incidence of treatment-emergent adverse events, LVEF, KCCQ, Seattle Angina Questionnaire, 6MWT |
REPEAT121 | II/III | No; yes; no | 5 years | 81 | Autologous BM-MNCs, single dose vs. two doses 4 months apart | IC | Ischaemic cardiomyopathy, EF ≤45% | Primary: mortality at 2 years; Secondary: morbidity efficacy endpoints (cardiac and CV mortality, HF hospitalization, ischaemic CE, CR, cardiac transplantation, VAD, ST, ICD, NYHA status, MLHFQ) and safety endpoints (BE, HE, LTA, new malignancies) |
6MWT, 6-min walk test; allo, allogeneic; BE, bleeding events; BMMNCs, bone marrow mononuclear cells; BM-MSCs, bone marrow-derived mesenchymal stromal cells; CDCs, cardiosphere-derived cells; CE, cardiac events; CR, coronary revascularization; CT, computed tomography; CV, cardiovascular; Echo, echocardiography; EF, ejection fraction; HE, all in-hospital events during therapy; HF-MACE, heart failure major adverse cardiac events; IC, intracoronary; ICD, implantable cardioverter-defibrillator; IV, intravenous; KCCQ, Kansas City Cardiomyopathy Questionnaire; LTA, life-threatening arrhythmias; LV, left ventricle; LVEDVI, left ventricular end-diastolic volume index; LVEF, left ventricular ejection fraction; LVESVI, left ventricular end-systolic volume index; MACE, major adverse cardiac events; MLHFQ, Minnesota Living with Heart Failure Questionnaire; MPC, Mesenchymal Precursor Cells; n, number of patients; MRI, magnetic resonance imaging; NT-proBNP, N-terminal pro-brain natriuretic peptide; NYHA, New York Heart Association; RV, right ventricle; ST, new synchronization therapy; TCE, time-to-first terminal cardiac event; TE, transendocardial; VAD, assisted device implantation; VO2 max, maximal oxygen consumption.
Cells were cultured at 5% O2.
Trials . | Phase . | Placebo- controlled; randomized; double-blind . | Follow-up . | n . | Cell type, dose, and treatment groups . | Delivery method . | Participants . | Endpoint . |
---|---|---|---|---|---|---|---|---|
STEM VAD117 | IIa | Yes; yes; yes | 12 months | 30 | Three doses of allo BM-MSCs, 1.5 × 106 cells/kga given 1 month apart | IV | HF (ischaemic or non-ischaemic) requiring LVAD implantation and deemed stable on LVAD | Primary: uncontrolled infection, all-cause mortality. Secondary: RV systolic function, admission for RV failure, 6MWT, reduction in NK cells, change in NT-proBNP |
CardiAMP-HF118 Raval et al. | III | No (sham controlled); yes; yes | 12 months | 250 | 200 × 106 auto BM-MNCs | TO | LVEF 20–40% secondary to remote MI | Primary: 6MWT; secondary: survival, MACE, MLHFQ |
SCIENCE119 | II | Yes; yes; yes | 12 months | 133 | 100 × 106 adipose-derived allo MSCs vs. placebo | TO | Ischaemic cardiomyopathy, EF ≤45% on echo, CT or MRI | Primary: change in LVESV; Secondary: serious adverse events |
Allogenic Stem Cell Therapy in Heart Failure (CSCC_ASCII)120 | II | Yes; yes; yes | 12 months | 81 | 100 × 106 adipose-derived allo MSCs vs. placebo, 2:1 randomization | TO | Ischaemic cardiomyopathy, EF ≤45% | Primary: change in LVESV; secondary: incidence of treatment-emergent adverse events, LVEF, KCCQ, Seattle Angina Questionnaire, 6MWT |
REPEAT121 | II/III | No; yes; no | 5 years | 81 | Autologous BM-MNCs, single dose vs. two doses 4 months apart | IC | Ischaemic cardiomyopathy, EF ≤45% | Primary: mortality at 2 years; Secondary: morbidity efficacy endpoints (cardiac and CV mortality, HF hospitalization, ischaemic CE, CR, cardiac transplantation, VAD, ST, ICD, NYHA status, MLHFQ) and safety endpoints (BE, HE, LTA, new malignancies) |
Trials . | Phase . | Placebo- controlled; randomized; double-blind . | Follow-up . | n . | Cell type, dose, and treatment groups . | Delivery method . | Participants . | Endpoint . |
---|---|---|---|---|---|---|---|---|
STEM VAD117 | IIa | Yes; yes; yes | 12 months | 30 | Three doses of allo BM-MSCs, 1.5 × 106 cells/kga given 1 month apart | IV | HF (ischaemic or non-ischaemic) requiring LVAD implantation and deemed stable on LVAD | Primary: uncontrolled infection, all-cause mortality. Secondary: RV systolic function, admission for RV failure, 6MWT, reduction in NK cells, change in NT-proBNP |
CardiAMP-HF118 Raval et al. | III | No (sham controlled); yes; yes | 12 months | 250 | 200 × 106 auto BM-MNCs | TO | LVEF 20–40% secondary to remote MI | Primary: 6MWT; secondary: survival, MACE, MLHFQ |
SCIENCE119 | II | Yes; yes; yes | 12 months | 133 | 100 × 106 adipose-derived allo MSCs vs. placebo | TO | Ischaemic cardiomyopathy, EF ≤45% on echo, CT or MRI | Primary: change in LVESV; Secondary: serious adverse events |
Allogenic Stem Cell Therapy in Heart Failure (CSCC_ASCII)120 | II | Yes; yes; yes | 12 months | 81 | 100 × 106 adipose-derived allo MSCs vs. placebo, 2:1 randomization | TO | Ischaemic cardiomyopathy, EF ≤45% | Primary: change in LVESV; secondary: incidence of treatment-emergent adverse events, LVEF, KCCQ, Seattle Angina Questionnaire, 6MWT |
REPEAT121 | II/III | No; yes; no | 5 years | 81 | Autologous BM-MNCs, single dose vs. two doses 4 months apart | IC | Ischaemic cardiomyopathy, EF ≤45% | Primary: mortality at 2 years; Secondary: morbidity efficacy endpoints (cardiac and CV mortality, HF hospitalization, ischaemic CE, CR, cardiac transplantation, VAD, ST, ICD, NYHA status, MLHFQ) and safety endpoints (BE, HE, LTA, new malignancies) |
6MWT, 6-min walk test; allo, allogeneic; BE, bleeding events; BMMNCs, bone marrow mononuclear cells; BM-MSCs, bone marrow-derived mesenchymal stromal cells; CDCs, cardiosphere-derived cells; CE, cardiac events; CR, coronary revascularization; CT, computed tomography; CV, cardiovascular; Echo, echocardiography; EF, ejection fraction; HE, all in-hospital events during therapy; HF-MACE, heart failure major adverse cardiac events; IC, intracoronary; ICD, implantable cardioverter-defibrillator; IV, intravenous; KCCQ, Kansas City Cardiomyopathy Questionnaire; LTA, life-threatening arrhythmias; LV, left ventricle; LVEDVI, left ventricular end-diastolic volume index; LVEF, left ventricular ejection fraction; LVESVI, left ventricular end-systolic volume index; MACE, major adverse cardiac events; MLHFQ, Minnesota Living with Heart Failure Questionnaire; MPC, Mesenchymal Precursor Cells; n, number of patients; MRI, magnetic resonance imaging; NT-proBNP, N-terminal pro-brain natriuretic peptide; NYHA, New York Heart Association; RV, right ventricle; ST, new synchronization therapy; TCE, time-to-first terminal cardiac event; TE, transendocardial; VAD, assisted device implantation; VO2 max, maximal oxygen consumption.
Cells were cultured at 5% O2.
3.2.2.3 Ongoing trials in HF
Ongoing or recently completed and not yet published studies of BM-MSCs in HF (Table 3) include DREAM-HF62,63 (vide supra) and STEM VAD (NCT03925324), a randomized, placebo-controlled, double-blind study of allogeneic BM-MSCs in patients with HF requiring LV assist device implantation (Table 3).
3.2.3 ‘Cardiopoietic’ bone marrow-derived MSCs
‘Cardiopoietic’ cells are BM-MSCs that have been modified by exposing them to a specific cocktail to promote lineage specification towards cardiovasculogenesis and to enhance their therapeutic potential.122 The first study with these cells, the C-CURE trial, published in 2013, was a Phase II multicentre, randomized study of 48 patients with ischaemic cardiomyopathy39 (Table 1). It was an open-label study with two arms comparing cardiopoietic cells (delivered transendocardially) and standard care vs. standard care. At 6 months after treatment, patients treated with autologous cardiopoietic cells exhibited improved LVEF, LVEDV, LVESV, and functional capacity (6-min walking distance) compared to standard care. This study, however, was not double-blind or placebo-controlled; further, an as-treated analysis was used and LV function was assessed by echocardiography rather than by MRI, the gold standard.
The encouraging results of C-CURE led to a larger, Phase III, sham-controlled, double-blind study (CHART-1) that randomized 315 patients with ischaemic cardiomyopathy to transendocardial injection of autologous cardiopoietic cells (n = 157) or sham procedure with no cell injection (n = 158) (control patients received cardiac catheterization only)47 (Table 1). After 39 weeks, there was no difference in the primary endpoint, which was a hierarchical composite of multiple endpoints, including mortality, worsening HF, MLHFQ score, 6-min walking distance, LVESV, and LVEF (measured by echocardiography). However, subgroup analyses revealed a significant improvement in the composite outcome in patients with more dilated left ventricles at baseline (LVEDV >200 mL), which were 60% of the population, as well as in patients with baseline LV volumes greater than the median, suggesting that the subset of the population with more severe cardiac dilation may benefit from these cells. In addition, at the 1-year follow-up, the cell-treated group exhibited a significant reduction in both LVEDV and LVESV, which were secondary endpoints (LVEF did not differ significantly).
The limitations of CHART-1 include the use of echocardiography to assess LV function and the lack of a placebo-treated group. Taken together, however, the results of this trial may not be as ‘negative’ as implied by the failure to meet the primary endpoint. Although cardiopoietic cells did not benefit the population of HF patients as a whole, the results suggest that cardiopoietic cells may have salutary effects on LV remodelling and may be useful in patients with greater LV dilatation, a hypothesis that would have to be prospectively tested with another trial. This, unfortunately, is unlikely to be feasible in the present climate. CHART-1 is an excellent example of the difficulties encountered in developing new cell therapies and selecting the optimal target population of patients.
3.2.4 Umbilical cord-derived MSCs
MSCs derived from the UC, and especially from the gelatinous substance that constitutes the UC primitive connective tissue (Wharton’s jelly), are a promising cell type for the treatment of heart disease. These cells share the beneficial properties of BM-MSCs, including low immunogenic potential and immunosuppressive, anti-inflammatory, anti-fibrotic, and pro-angiogenic actions.26,123–129 Compared with BM-MSCs, however, UC-MSCs offer several advantages, including wide availability, easier isolation, higher proliferative capacity, less cellular ageing, and ability to be expanded ex vivo with high genomic stability and full clonogenic potential; furthermore, they do not require invasive harvesting procedures, and because of the abundance of donors and their higher proliferative rate, are less expensive to produce in large quantities.26,123–129 Importantly, there is evidence that UC-MSCs are more potent than BM-MSCs (since they are derived from a much younger organism). They secrete numerous pro-angiogenic factors [including vascular endothelial growth factor (VEGF), angiopoietin-1, hepatocyte growth factor (HGF), and transforming growth factor(TGF)-beta1130] and exert pro-angiogenic actions in vivo.131,132,In vitro studies have shown that, compared with BM-MSCs, UC-MSCs exhibit less senescence and superior clonogenicity, migration, lymphoproliferative suppression,133 and paracrine actions (e.g. they secrete much larger quantities of cytokines such as HGF).50 Various cell types are present in the UC; among these, the MSCs obtained from Wharton’s jelly appear to have the greatest therapeutic potential.129
The anti-inflammatory and immunosuppressive properties of UC-MSCs have been well documented, as detailed elsewhere.134 For example, in the RIMECARD trial (vide infra), UC-MSCs were shown to inhibit proinflammatory T cells in vitro.50 In a murine model of acute MI, i.v. infusion of UC-MSCs at 48 h after coronary ligation reduced pro-inflammatory M1-type macrophages and increased M2-type macrophages, an effect that appeared to be mediated by UC-MSC-derived interleukin-10.135
Regarding efficacy, many preclinical studies have documented the ability of UC-MSCs to improve LV function in various animal models of acute MI or chronic HF.136–144 In a swine model of acute MI, intramyocardial injection of UC-MSCs was reported to reduce apoptosis and fibrosis and to improve LV remodelling and function.143 Improvement in cardiac remodelling was also reported in rats that received intramyocardial injections of UC-MSCs after MI144 and in models of dilated (non-ischaemic) cardiomyopathy.145–147 In a swine model of chronic ischaemic cardiomyopathy, i.v. administration of UC-MSCs increased LVEF, fractional shortening, myocardial perfusion, angiogenesis, and collateral development, and reduced apoptosis and fibrosis.137 Anti-apoptotic and anti-fibrotic actions of UC-MSCs have also been documented in rodent models of chronic HF.125,126 Finally, UC-MSCs enhance angiogenesis in vitro and in vivo through up-regulation of various proangiogenic factors and chemokines, including VEGF, angiopoietin, and MCP-1 among others.125,126
In the clinical arena, only a handful of studies have evaluated UC-MSCs in patients with heart disease (Table 4). The most important is the RIMECARD trial,50 a Phase I, randomized, double-blind, and placebo-controlled study in which patients with ischaemic or non-ischaemic cardiomyopathy received an i.v. infusion of UC-MSCs (1 × 106 cells/kg) or placebo (n = 15 per group) and were followed for 1 year. None of the patients tested developed alloantibodies to the injected cells. In patients given UC-MSCs, there was a significant improvement in LVEF (assessed both by echocardiography and MRI) vs. baseline that became apparent at 3 months and was maintained at 6 and 12 months; no such improvement was observed in the placebo group. Throughout the follow-up period, the NYHA class, MLHFQ score, and KCCQ score all improved in the UC-MSC-treated group, but not in the control group. Studies in vitro showed that UC-MSCs and BM-MSCs inhibited T-cell proliferation to a similar extent, suggesting similar immunosuppressive properties. The secretomes of the two cell types were also similar, but UC-MSCs exhibited much higher expression of HGF and greater migration capacity.50 In conclusion, RIMECARD provided evidence that i.v. administration of UC-MSCs improves LV function, functional status, and quality of life in patients with HF of ischaemic or non-ischaemic origin.50
Trial . | Phase . | Clinical setting . | Placebo controlled; randomized; double-blind . | Follow-up . | n . | Cell dose and treatment groups . | Delivery method . | Endpoint evaluation . | LVEF . | LV volumes . | Scar size . | NYHA class . | Functional capacity . | QoL . |
---|---|---|---|---|---|---|---|---|---|---|---|---|---|---|
Zhao et al.148 | I/II | Ischaemic or non-ischaemic HF, EF < 35% | No; yes; ? | 6 months | 59 | UC-MSCs + GDMT vs. GDMT | IC | Echo | ↑ | ↓ | NA | NA | ↑ | NA |
Gao et al.149 | I/II | Acute STEMI | Yes; yes; yes | 18 months | 116 | 6 × 106 UC-MSCs (Wharton’s jelly) vs. placebo | IC | SPECT/Echo | ↑ | NA | ↓ | NA | NA | NA |
Musialek et al.150 a | I | AMI, LVEF < 45% | Not; not; not | 12 months | 10 | 30 × 106 UC-MSCs (Wharton’s jelly) | IC | Echo / MRI | NA | NA | NA | NA | NA | NA |
Li et a.l151 | I/II | Ischaemic HF with CTO, CCS II-IV angina, age >80 years | Not; not; not | 24 months | 15 | 3 × 106, 4 × 106, 5 × 106 UC-MSCs | IC | Echo/SPECT | All ↑ | NA | All ↓ | All ↑ | NA | NA |
Fang et al.152 | ? | ICM, EF < 40% | Not; not; not | 12 months | 3 | 5–10 × 106 UC-MSCs | IV | ? | 2 pts, 1 pt | EDV ↑ all pts, ESV ↑ 2 pts, ↓ 1 p | NA | 3 pts ↑ | 2 pts, 1 pt | NA |
RIMECARD50 Bartolucci et al . | I/II | ICM, EF <40% | Yes; yes; yes | 12 months | 30 | 1 × 106/kg UC-MSCs vs. placebo | IV | Echo / MRI | ↑ | ↑ EDV | NA | ↑ | ↑ | ↑ |
Can et al.153,154 | I/II | ICM, EF 25–45% | No (Sham); yes; no | 6 months | 79 | UC-MSCs (2 × 107) or BM-MNCs (20–25 × 107) + CABG vs. CABG | TO | MRI/SPECT | ? | ? | ? | ? | ? | ? |
Trial . | Phase . | Clinical setting . | Placebo controlled; randomized; double-blind . | Follow-up . | n . | Cell dose and treatment groups . | Delivery method . | Endpoint evaluation . | LVEF . | LV volumes . | Scar size . | NYHA class . | Functional capacity . | QoL . |
---|---|---|---|---|---|---|---|---|---|---|---|---|---|---|
Zhao et al.148 | I/II | Ischaemic or non-ischaemic HF, EF < 35% | No; yes; ? | 6 months | 59 | UC-MSCs + GDMT vs. GDMT | IC | Echo | ↑ | ↓ | NA | NA | ↑ | NA |
Gao et al.149 | I/II | Acute STEMI | Yes; yes; yes | 18 months | 116 | 6 × 106 UC-MSCs (Wharton’s jelly) vs. placebo | IC | SPECT/Echo | ↑ | NA | ↓ | NA | NA | NA |
Musialek et al.150 a | I | AMI, LVEF < 45% | Not; not; not | 12 months | 10 | 30 × 106 UC-MSCs (Wharton’s jelly) | IC | Echo / MRI | NA | NA | NA | NA | NA | NA |
Li et a.l151 | I/II | Ischaemic HF with CTO, CCS II-IV angina, age >80 years | Not; not; not | 24 months | 15 | 3 × 106, 4 × 106, 5 × 106 UC-MSCs | IC | Echo/SPECT | All ↑ | NA | All ↓ | All ↑ | NA | NA |
Fang et al.152 | ? | ICM, EF < 40% | Not; not; not | 12 months | 3 | 5–10 × 106 UC-MSCs | IV | ? | 2 pts, 1 pt | EDV ↑ all pts, ESV ↑ 2 pts, ↓ 1 p | NA | 3 pts ↑ | 2 pts, 1 pt | NA |
RIMECARD50 Bartolucci et al . | I/II | ICM, EF <40% | Yes; yes; yes | 12 months | 30 | 1 × 106/kg UC-MSCs vs. placebo | IV | Echo / MRI | ↑ | ↑ EDV | NA | ↑ | ↑ | ↑ |
Can et al.153,154 | I/II | ICM, EF 25–45% | No (Sham); yes; no | 6 months | 79 | UC-MSCs (2 × 107) or BM-MNCs (20–25 × 107) + CABG vs. CABG | TO | MRI/SPECT | ? | ? | ? | ? | ? | ? |
Trials are listed in chronological order of publication.
indicates increase; ↓ indicates decrease; AMI, acute myocardial infarction; CCS, Canadian cardiovascular scoring of angina pectoris; CTO, chronic coronary artery occlusion; Echo, echocardiogram; EDV, end-diastolic volume; EF, ejection fraction; ESV, end-systolic volume; GDMT, guideline-directed medical therapy; HF, heart failure; IC, intracoronary; ICM, ischaemic cardiomyopathy; IV, intravenous; SPECT, single-photon emission computed tomography; STEMI, ST-elevation myocardial infarction; LAD, left anterior descending; LV, left ventricle; MRI, magnetic resonance imaging; NA, not applicable; pt, patient; UC-MSC, umbilical cord-derived mesenchymal stromal cells.
This study looked at safety; Wharton’s jelly MSCs were found to be safe.
Trial . | Phase . | Clinical setting . | Placebo controlled; randomized; double-blind . | Follow-up . | n . | Cell dose and treatment groups . | Delivery method . | Endpoint evaluation . | LVEF . | LV volumes . | Scar size . | NYHA class . | Functional capacity . | QoL . |
---|---|---|---|---|---|---|---|---|---|---|---|---|---|---|
Zhao et al.148 | I/II | Ischaemic or non-ischaemic HF, EF < 35% | No; yes; ? | 6 months | 59 | UC-MSCs + GDMT vs. GDMT | IC | Echo | ↑ | ↓ | NA | NA | ↑ | NA |
Gao et al.149 | I/II | Acute STEMI | Yes; yes; yes | 18 months | 116 | 6 × 106 UC-MSCs (Wharton’s jelly) vs. placebo | IC | SPECT/Echo | ↑ | NA | ↓ | NA | NA | NA |
Musialek et al.150 a | I | AMI, LVEF < 45% | Not; not; not | 12 months | 10 | 30 × 106 UC-MSCs (Wharton’s jelly) | IC | Echo / MRI | NA | NA | NA | NA | NA | NA |
Li et a.l151 | I/II | Ischaemic HF with CTO, CCS II-IV angina, age >80 years | Not; not; not | 24 months | 15 | 3 × 106, 4 × 106, 5 × 106 UC-MSCs | IC | Echo/SPECT | All ↑ | NA | All ↓ | All ↑ | NA | NA |
Fang et al.152 | ? | ICM, EF < 40% | Not; not; not | 12 months | 3 | 5–10 × 106 UC-MSCs | IV | ? | 2 pts, 1 pt | EDV ↑ all pts, ESV ↑ 2 pts, ↓ 1 p | NA | 3 pts ↑ | 2 pts, 1 pt | NA |
RIMECARD50 Bartolucci et al . | I/II | ICM, EF <40% | Yes; yes; yes | 12 months | 30 | 1 × 106/kg UC-MSCs vs. placebo | IV | Echo / MRI | ↑ | ↑ EDV | NA | ↑ | ↑ | ↑ |
Can et al.153,154 | I/II | ICM, EF 25–45% | No (Sham); yes; no | 6 months | 79 | UC-MSCs (2 × 107) or BM-MNCs (20–25 × 107) + CABG vs. CABG | TO | MRI/SPECT | ? | ? | ? | ? | ? | ? |
Trial . | Phase . | Clinical setting . | Placebo controlled; randomized; double-blind . | Follow-up . | n . | Cell dose and treatment groups . | Delivery method . | Endpoint evaluation . | LVEF . | LV volumes . | Scar size . | NYHA class . | Functional capacity . | QoL . |
---|---|---|---|---|---|---|---|---|---|---|---|---|---|---|
Zhao et al.148 | I/II | Ischaemic or non-ischaemic HF, EF < 35% | No; yes; ? | 6 months | 59 | UC-MSCs + GDMT vs. GDMT | IC | Echo | ↑ | ↓ | NA | NA | ↑ | NA |
Gao et al.149 | I/II | Acute STEMI | Yes; yes; yes | 18 months | 116 | 6 × 106 UC-MSCs (Wharton’s jelly) vs. placebo | IC | SPECT/Echo | ↑ | NA | ↓ | NA | NA | NA |
Musialek et al.150 a | I | AMI, LVEF < 45% | Not; not; not | 12 months | 10 | 30 × 106 UC-MSCs (Wharton’s jelly) | IC | Echo / MRI | NA | NA | NA | NA | NA | NA |
Li et a.l151 | I/II | Ischaemic HF with CTO, CCS II-IV angina, age >80 years | Not; not; not | 24 months | 15 | 3 × 106, 4 × 106, 5 × 106 UC-MSCs | IC | Echo/SPECT | All ↑ | NA | All ↓ | All ↑ | NA | NA |
Fang et al.152 | ? | ICM, EF < 40% | Not; not; not | 12 months | 3 | 5–10 × 106 UC-MSCs | IV | ? | 2 pts, 1 pt | EDV ↑ all pts, ESV ↑ 2 pts, ↓ 1 p | NA | 3 pts ↑ | 2 pts, 1 pt | NA |
RIMECARD50 Bartolucci et al . | I/II | ICM, EF <40% | Yes; yes; yes | 12 months | 30 | 1 × 106/kg UC-MSCs vs. placebo | IV | Echo / MRI | ↑ | ↑ EDV | NA | ↑ | ↑ | ↑ |
Can et al.153,154 | I/II | ICM, EF 25–45% | No (Sham); yes; no | 6 months | 79 | UC-MSCs (2 × 107) or BM-MNCs (20–25 × 107) + CABG vs. CABG | TO | MRI/SPECT | ? | ? | ? | ? | ? | ? |
Trials are listed in chronological order of publication.
indicates increase; ↓ indicates decrease; AMI, acute myocardial infarction; CCS, Canadian cardiovascular scoring of angina pectoris; CTO, chronic coronary artery occlusion; Echo, echocardiogram; EDV, end-diastolic volume; EF, ejection fraction; ESV, end-systolic volume; GDMT, guideline-directed medical therapy; HF, heart failure; IC, intracoronary; ICM, ischaemic cardiomyopathy; IV, intravenous; SPECT, single-photon emission computed tomography; STEMI, ST-elevation myocardial infarction; LAD, left anterior descending; LV, left ventricle; MRI, magnetic resonance imaging; NA, not applicable; pt, patient; UC-MSC, umbilical cord-derived mesenchymal stromal cells.
This study looked at safety; Wharton’s jelly MSCs were found to be safe.
The remaining studies have used intracoronary infusion of UC-MSCs (Table 4). A randomized Phase I trial148 reported improvement in LVEF and 6-min walking distance at 6 months after intracoronary infusion of UC-MSCs in patients with chronic HF. In a randomized, double-blind study of 116 patients with ST-elevation myocardial infarction (STEMI), intracoronary infusion of UC-MSCs increased myocardial viability and LVEF 18 months later.149 Observational studies suggest improved LVEF after intracoronary infusion of UC-MSCs in patients with ischaemic HF (LVEF: 38.6 ± 5.1).151 Interestingly, many studies have reported beneficial effects and anti-inflammatory actions of UC-MSCs (mostly given i.v.) in non-cardiovascular settings such as multiple sclerosis, spinal cord injury, systemic lupus erythematosus, rheumatoid arthritis, ulcerative colitis, Crohn’s disease, and GVHD.83,155–165
In summary, UC-MSCs share the beneficial properties of BM-MSCs but offer many biological, financial, and logistic advantages over BM-MSCs. Extensive preclinical data, as well as initial clinical data from one study of i.v. infusion (RIMECARD) and several studies of i.c. infusion of UC-MSCs, are encouraging and warrant further investigation of these cells.
3.3 Adipose-derived regenerative cells and adipose-derived MSCs
The term ‘adipose-derived regenerative cells’ (ARDCs) has been used to indicate a heterogeneous population of cells, obtained from the vascular stromal fraction of adipose tissue, that includes endothelial cells, smooth muscle cells, pericytes, macrophages, and a high proportion of MSCs and CD34+ cells.166–169 ADRCs can be readily obtained by fat harvest (limited liposuction) with automated, same-day processing without requiring culture or expansion. Preclinical studies have suggested beneficial effects of ARDCs in animal models of acute170 and chronic23,169,171–173 ischaemic cardiomyopathy, providing a rationale for translational efforts.
The first clinical trial of ADRCs, published in 2014, was the PRECISE trial, a Phase I, randomized, placebo-controlled, double-blind study that examined the effects of transendocardial injection of autologous ADRCs cells in patients with chronic ischaemic cardiomyopathy43 (Table 1). Twenty-seven patients were randomized to three escalating doses of ADRCs or placebo, resulting in small group sizes (only six control patients). The study demonstrated safety and feasibility; although neither LV volumes nor LVEF was improved, functional capacity (peak VO2) was preserved in the cell-treated group whereas it declined significantly in the control group at 18 months. In addition, MRI showed improvement in wall motion in cell-treated patients at 6 months. The trial was limited by the small sample sizes, imbalances in age (treated patients were older), and lack of MRI data after 6 months; nevertheless, it suggested potential efficacy.
The ATHENA trials, published in 2017, were undertaken in follow-up to PRECISE with the goal of assessing the efficacy of ADRCs (Table 1). Unfortunately, they were terminated prematurely because of two cerebrovascular events that were deemed not related to the cell product itself but instead to the underlying disease and to the procedure. The ATHENA programme consisted of two parallel, randomized, double blind, placebo-controlled, phase II trials that studied transendocardial administration of ADRCs in 31 patients [40 × 106 cells, n = 28 (ATHENA) or 80 × 106 cells, n = 3 (ATHENA II)] with ‘no option’ chronic ischaemic cardiomyopathy (LVEF < 45%).46 At 12 months, there were no differences in LVEF or LV volumes; however, the treatment group exhibited an improvement in functional capacity, hospitalization rate, and MLHFQ score. Both PRECISE and ATHENA were small studies that used echocardiography to assess LV function and volumes. Larger trials using cardiac MRI will be necessary to assess the therapeutic utility of ADRCs. Further insights may be provided soon by two ongoing Phase II trials of adipose-derived MSCs: SCIENCE (NCT02673164119) and CSCC ASCII (NCT03092284), which are testing the effects of allogeneic adipose-derived MSCs in ischaemic HF (Table 3). A first-in-human study of these cells in 10 patients has shown safety and feasibility51 (Table 1).
3.4 CD34+ cells
Administration of G-CSF causes the BM to release CD34+ stem cells, which can be harvested in the peripheral blood. In 2011, Vrtovec et al.67 reported the results of a pilot open-label study in which G-CSF-mobilized CD34+ cells were infused intracoronarily in 55 patients with non-ischaemic cardiomyopathy (Table 2). After 1 year, CD34+ cell infusion was associated with a significant improvement in LVEF, functional capacity, NT-proBNP levels, and overall mortality compared with the control patients who received no treatment. In a subsequent larger study (published in 2013) that enrolled 110 patients, Vrtovec et al.68 found that at 5 years after intracoronary infusion of G-CSF-mobilized CD34+ cells, there was still a significant improvement in LVEF, functional capacity, NT-proBNP levels, and overall mortality in the cell-treated group (Table 2). An additional study, published in 2013, that compared transendocardial and intracoronary injection routes in 40 patients with non-ischaemic cardiomyopathy demonstrated that direct transendocardial CD34+ cell injection led to greater retention of cells and greater improvement in LVEF, functional capacity, and NT-proBNP levels69 (Table 2). Taken together, these results are encouraging and warrant further investigation; however, the three studies67–69 are limited by the fact that they were performed at a single centre, used echocardiography to assess LV function, and were not double-blind or placebo-controlled. More rigorous trials of G-CSF-mobilized CD34+ cells are in order to assess the therapeutic efficacy of this product. On the other hand, transendocardial injection of CD34+ cells has shown great promise in treating refractory angina.174
3.5 c-kit-positive cardiac cells
It is not possible to review the CONCERT-HF trial of CPCs without discussing the recent controversy regarding CPCs. Briefly, one prominent basic research laboratory at Harvard published numerous studies claiming that cells isolated from the adult heart on the basis of c-kit expression exhibited the properties of genuine stem cells, i.e. they were self-renewing, clonogenic, and able to differentiate into smooth muscle cells, endothelial cells and cardiomyocytes and to regenerate large swaths of dead myocardium.175 However, many of these papers were subsequently retracted when fraudulent data manipulation was discovered.5
One of the casualties of the misconduct in the Harvard laboratory was the SCIPIO trial; the paper reporting the results of this Phase I study was retracted because images (generated at Harvard) of the phenotype of CPCs were found to have been manipulated.176 SCIPIO was a collaboration between a basic research group at Harvard and a clinical group in Louisville. As the Lancet editors made clear, the retraction of the SCIPIO paper was caused exclusively by issues with the data generated at Harvard, not with those generated in Louisville. In the retraction notice, the editors state: ‘Although we do not have any reservations about the clinical work in Louisville that used the preparations from Anversa’s laboratory in good faith, the lack of reliability regarding the laboratory work at Harvard means that we are now retracting this paper’.176 Because of the uncertainty regarding the phenotype of the cell product used, the clinical results of SCIPIO cannot be properly interpreted. However, it would be inappropriate to dismiss all the work done on CPCs heretofore because of one laboratory’s misconduct. The falsification of data by the Harvard group is a tragedy, but it should not lead to the conclusion that CPCs have no therapeutic potential. In fact, a review of the literature shows that the preclinical data on CPCs are quite consistent: at least 50 studies from 26 laboratories independent of the Harvard group have reported beneficial effects of CPCs in various animal models of heart disease.6 Our group has shown that CPCs do not engraft in the heart, do not differentiate into new cardiac myocytes, do not regenerate dead myocardium, and thus work via paracrine mechanisms.7,11–17,23–25,177 Although the mechanism of action of CPCs is unclear7,11–17,23–25,177 (as is the case for all cell types used for cardiac repair), the aforementioned large body of preclinical evidence provided a robust rationale for the recently completed CONCERT-HF trial.60,61
CONCERT-HF was a Phase II, randomized, placebo-controlled, double-blind, multicentre study of autologous BM-MSCs, CPCs, or both, in patients with ischaemic HF.60,61 A total of 125 patients with chronic ischaemic HF receiving maximal guideline-directed therapy were randomized (1:1:1:1) to receive transendocardial injections of 5 × 106 autologous CPCs (n = 31), 150 × 106 autologous BM-MSCs (n = 29), their combination (n = 33), or placebo (n = 32). At 12 months, there were no differences among groups with respect to LV function, scar size, functional capacity (assessed as maximal O2 consumption and 6-min walking distance), or NT-proBNP levels. However, the proportion of patients with HF-related major adverse cardiac events (HF-MACE) was significantly different, being highest in the placebo group (28.1%) and lowest in the CPCs alone group (6.5%; P = 0.043 vs. placebo) (Figure 2). In the CPCs + MSCs group, HF-MACE occurred in 9.1% of patients (P = 0.061 vs. placebo). The differences in HF-MACE were driven primarily by hospitalization for HF. As discussed above in the section on BM-MSCs, CONCERT-HF also found an improvement in quality of life in patients treated with MSCs, alone or in combination with CPCs.
CONCERT-HF was arguably one of the most rigorous cell therapy trials conducted heretofore; it was also the first trial that used CPCs manufactured according to GMP standards. Taken together, the results suggest that in patients with chronic ischaemic HF on maximal guideline-driven therapy, a single administration of CPCs or MSCs has measurable beneficial effects over the ensuing 12 months, namely, a reduction in hospitalization for HF (with CPCs) and an improvement in quality of life (with MSCs). The improvement in clinical outcomes without an improvement in LV function or reduction in scar size is consistent with several previous studies (e.g. ixCELL-DCM and TAC-HFT) indicating that in patients with chronic ischaemic HF, cell therapy can effect significant clinical changes without concomitant changes in LVEF40,42,45,178 (vide infra). Whether these beneficial effects are related to anti-inflammatory, immunomodulatory, antifibrotic, proangiogenic, endothelial protective, or other as-yet unknown actions of the transplanted cells remains to be elucidated.
3.6 Cardiosphere-derived cells
Cardiosphere-derived cells (CDCs) were first described by Messina et al. in 2004,179 They can be obtained from the culture outgrowth of human myocardial biopsies and consist of a heterogeneous mixture of different cell types that includes, among others, MSCs, c-kit+ cells, endothelial cells, as well as other less well-defined phenotypes.180 The first trial of CDCs, published in 2012, was CADUCEUS, a single-centre, open-label, Phase I study of 31 patients that received intracoronary infusion of autologous CDCs or standard of care 1.5–3 months after MI38 (Table 1). The investigators reported a reduction in scar size at 12 months which, however, was not associated with improvement in LVEF or LV volumes. In follow-up to this trial, these investigators undertook the ALLSTAR trial, a multicentre, randomized, double-blind, placebo-controlled, Phase II study in which 142 patients with LV dysfunction secondary to an MI in the previous 1–12 months (mean, 4.6 months) were randomized 2:1 to receive an intracoronary infusion of allogeneic CDCs or placebo56 (Table 1). The primary endpoint was scar size, as assessed by MRI, at 12 months after treatment. The trial was stopped by the sponsor after an interim data analysis of the 6-month data revealed evidence of futility to meet the primary endpoint.57 The 6-month results showed no difference in scar size between the CDC and placebo groups and a small reduction in LVEDV (−4.5 mL), LVESV (−4.8 mL), and NT-proBNP (−303 pg/mL) in the CDC relative to the placebo group.57 Thus, this study did not confirm the scar size reduction reported in CADUCEUS.38 ALLSTAR suffers from several limitations. It did not assess its primary endpoint because the study was stopped before completion and so the decision to report the 6-month results instead of the 12-month results was made post hoc. The patient population was heterogeneous [both recent (1–2 months) and old MIs were included] and at relatively low risk (average LV EF was 40% and both STEMI and non-STEMI patients were included). Finally, clinical data (e.g. medications, presence of multivessel disease, comorbidities, diastolic dysfunction, and body mass index) were not collected consistently, and information on the type of MI was missing in 58 of the 142 patients.57 The combination of CADUCEUS and ALLSTAR is an example of how single-centre, unblinded studies can generate results that are not confirmed in subsequent multicentre, double-blind studies.
DYNAMIC was a pilot, open-label study of 14 patients with dilated cardiomyopathy (ischaemic or non-ischaemic) who received intracoronary infusions of four escalating doses of allogeneic CDCs in all three coronary arteries58 (Table 1). Although LV EF and quality of life scores improved at 6 and 12 months, the lack of a placebo group and blinding makes these results uninterpretable. Apart from HOPE-2 (NCT03406780), which assesses the effects of CDCs in Duchenne muscular dystrophy, with LV wall thickening as a secondary endpoint, no ongoing study of CDCs in HF is registered in the ClinicalTrials.gov website. It appears that investigation of CDCs in HF is all but abandoned.
3.7 Cell combinations
In principle, combining different cell types may be advantageous because the actions of one type could be augmented by different actions of another type. Several of the products discussed above (unfractionated cells from BM or adipose tissue, CDCs) could technically be categorized as cell combinations because they contain multiple cell types. In this section, we will review cell combinations that have been prepared by investigators for therapeutic use.
The largest clinical trial of a cell combinations for HF reported to date is ixCELL-DCM, published in 2016, a randomized, double-blind, placebo-controlled, Phase IIb study that examined the effects of ixmyelocel-T, a specific fraction of autologous BM-MNCs that contains both MSCs and macrophages45 (Table 1). The precursors to ixCELL-DCM were two small Phase IIa trials [IMPACT-DCM (n = 39) and Catheter-DCM (n = 22)] that compared ixmyelocel-T with standard of care in an unblinded fashion and found improved clinical outcome (reduction in MACE and improved NYHA class and MLHFQ score), but only in patients with ischaemic HF40 (Table 1). ixCELL-DCM followed up on these studies using a larger population and more rigorous methods. A total of 126 patients with ischaemic HF received transendocardial injection of ixmyelocel-T or placebo. The primary endpoint was the 12-month incidence of MACE (death, cardiac hospitalization, acute decompensated HF). Although there were no differences in LVEF or LV volumes (assessed by echocardiography), cell-treated patients exhibited a statistically significant 37% reduction in MACE (Figure 2). ixCELL-DCM is important not only because it is one of the few Phase II studies of cell therapy in HF that has met its primary endpoint,4 but also because it supports the notion that cell therapy can beneficially affect hard clinical endpoints (as opposed to imaging endpoints)—an outcome that has been recently observed also in CONCERT-HF60 and DREAM-HF (Figure 2).63
Preclinical studies in pigs support the concept of a synergistic interaction between BM-MSCs and CPCs, whereby the combination of these cell types is more effective than either alone at improving LV function in models of chronic ischaemic cardiomyopathy.181–183 As discussed above, CONCERT-HF tested whether the combination of autologous BM-MSCs and CPCs was superior to either cell type alone.61 Although no treatment improved LV function or scar size, patients receiving CPCs alone exhibited a reduction in HF-MACE whereas those receiving MSCs alone exhibited improved quality of life; patients treated with CPCs + MSCs exhibited the best overall clinical outcome, i.e. improved quality of life and reduced HF-MACE.60 The results of CONCERT-HF suggest that combining two different cell products results in a better outcome than either product alone, providing a rationale for further studies of combinatorial cell therapy.
3.8 Embryonic stem cells
Unlike all of the adult cell types being examined as potential cardiac therapies, human embryonic stem cells (hESCs) possess the unquestionable ability to differentiate into contracting adult cardiomyocytes. For this reason, extensive efforts have been made over the past two decades to harness hESCs to regenerate dead or injured myocardium. However, it is now apparent that transplantation of hESCs or hESC-derived cells is plagued by many formidable problems that will preclude its clinical application, including graft rejection requiring life-long immunosuppression, life-threatening arrhythmias,184,185 genomic instability, and potential risk of teratomas (reviewed in ref.28) Although the probability of tumours can be reduced by inducing hESC differentiation, it is unlikely to be completely eliminated, particularly when billions of cells are injected (as was done in primate studies184,185) Even more importantly, the most compelling reason for using hESC-derived cells (i.e. their potential to regenerate working cardiac muscle) has now evaporated, because many preclinical studies have documented that hESC-derived cells disappear rapidly after transplantation in the heart (e.g. Zhu et al.27; reviewed in ref.28) To date, there is no evidence of long-term (i.e. longer than 2–3 months) engraftment, let alone regeneration, after transplantation of hESCs in the heart. Instead, recent evidence has shown that hESC-derived cells act via paracrine mechanisms186—just like all of the adult cell types discussed in this review.
Many studies have reported that transplantation of hESCs or hESC -derived myocytes improves LV function in animal models of HF (reviewed in ref.28) To date, the only clinical experience with these cells in the cardiovascular field is the ESCORT trial,52,187 an uncontrolled, open-label, phase I study in which a fibrin scaffold containing hESC-derived progenitor cells was implanted on the epicardium of six patients with ischaemic HF that underwent coronary artery bypass surgery; no control patients were enrolled (Table 1). All patients were treated with immunosuppressive drugs to prevent graft rejection. In three patients there were clinically silent allorejection reactions, demonstrated by the presence of alloantibodies at low titer; these reactions resolved over time, most likely due to immunosuppression. Because of the absence of a control group, the small sample size, and the fact that patients received two interventions (revascularization and stem cells) at the same time, no conclusions regarding safety or efficacy can be made from ESCORT. Ultimately, this study was stopped because of the mounting evidence that transplanted cells most likely work via paracrine mechanism, not by engrafting and forming new contractile units.186
In summary, despite sanguine claims in the scientific literature that presented hESCs as a therapeutic breakthrough188–195 and despite much hype in the media, it is implausible that hESC-derived cells will become a therapeutic option for HF or any cardiovascular condition.28 The available evidence shows that hESC-derived cells do not engraft and thus do not regenerate myocardium in experimental animals. Furthermore, hESC-derived cell transplantation is associated with a host of serious side effects that are not observed with transplantation of adult cells. No controlled clinical trial of hESC-derived cells in cardiovascular disease has been performed or even initiated, whereas adult cells have been used in thousands of patients, with no significant adverse effects and with results that were sufficiently encouraging to warrant Phase II and III trials. Finally, the need for hESCs is obviated by the availability of induced pluripotent stem cells, which have pluripotency similar to hESCs but do not require lifelong immunosuppression. Given these facts, and given the serious ethical concerns associated with the use of hESCs, the rationale for continuing to pursue hESC-derived therapies for heart disease is unclear.
3.9 Repeated cell therapy
To date, almost all clinical trials of cell therapy in cardiovascular medicine have used one dose of cells. However, as discussed above, cells disappear almost completely within days or weeks of transplantation,11–22 and so it is difficult to rationalize why one cell dose should be sufficient to achieve a sustained therapeutic response, particularly in a condition, such as HF, that develops gradually over many years. Apart from surgical and interventional procedures, there is no example of a therapy that produces long-lasting improvement in HF when administered only once. Given that the retention of cells in the heart after transplantation is short-lived, it stands to reason that greater benefit should be accrued by additional treatments. Preclinical studies have shown that repeated administrations of cells have additive effects on cardiac function in animal models of ischaemic cardiomyopathy16,17,19,196,197; however, in the clinical arena, this paradigm has not been tested thoroughly in patients with HF, probably because of the invasive nature of the delivery techniques used (performing two or three intracoronary or transendocardial injections would be difficult, particularly if a placebo control is contemplated).
Thus, few clinical studies of repeated cell dosing have been reported, and their design has been limited. Most of these trials have been conducted in ischaemic cardiomyopathy (Table 5). The DanCell-CHF trial, published in 2008, failed to demonstrate significant improvement in LV function after two intracoronary injections of BM-MNCs administered 4 months apart in patients with ischaemic HF.198 In contrast to these findings, in a study of 39 patients with STEMI published in 2009, Yao et al.199 reported that two intracoronary infusions of BM-MNCs at 3–7 days and again 3 months after MI were associated with greater improvement in LV function and reduction of myocardial scar size compared with single-cell administration. Similarly, in 2011, Gu et al.200 reported that in 45 patients with ischaemic HF, a repeated intracoronary infusion of peripheral blood mononuclear cells (containing ≥ 1 million CD34+ cells) at 6 months resulted in a more pronounced improvement in LVEF when compared with single-cell dosing. In an observational study lacking a control group, Mann et al.201 reported in 2015 that in 23 patients with refractory angina pectoris, a second transendocardial injection of autologous BM-MNCs performed an average of 4.6 years after the first resulted in improved myocardial perfusion and angina symptoms for at least 1 year, with an effect size comparable to the first injection; the effects on LVEF could not be properly evaluated because LVEF was normal before the second injection. In 2016, a registry analysis suggested that a second intracoronary infusion of BM-MNCs 3–6 months after the first resulted in lower-than-expected mortality in patients with ischaemic HF,202 providing a rationale for the ongoing REPEAT trial (NCT01693042) (Table 5).
Clinical trials of repeated cell therapy in ischaemic or non-ischaemic cardiomyopathy
Trials . | Phase . | Clinical setting . | Placebo controlled; randomized; double-blind . | Follow-up . | n . | Cell type, dose, and treatment groups . | Delivery method . | Endpoint evaluation . | LVEF . | LV volumes . | Scar size . | NYHA class . | Functional capacity . | QoL . |
---|---|---|---|---|---|---|---|---|---|---|---|---|---|---|
DanCell-CHF Diederichsen et al.198 | II | Ischaemic HF | Not; not; not | 12 months | 32 | One group: 647 ± 382 × 106 (1st dose) and 889 ± 361 × 106 (2nd dose) BM-MNCs, 4 months apart; variables compared before and after treatment | IC | Echo | NS | NS | NA | ↑ | NS | NA |
Yao et al.199 | ? | First STEMI with LVEF 20–39% | Yes; yes; ? | 12 months | 39 | Three groups: single dose 1.9 ± 1.2 × 108; two doses of 2.0 ± 1.4 × 108 and 2.1 ± 1.7 × 108 BM-MNCs 3 months apart; one dose of placebo | IC | MRI/SPECT | 2 doses > 1 dose > control | 2 doses > 1 dose > control | 2 doses > 1 dose > control | NA | NA | NA |
Gu et al.200 | I | Ischaemic HF | Not; ?; not | 12 months | 45 | Control (no intervention) vs. single dose SC G-CSF and single dose (1.5–2 × 108) IV MNCs containing a minimum of 1 × 106 CD34+cells vs. single dose G-CSF and two doses of IV MNCs 6 months apart | IC | Echo/SPECT | 2 doses > 1 dose > control | NA | LVEDV: 2 doses > 1 dose > control | 2 doses > 1 dose or control | NA | NA |
Mann et al.201 a | NA | Refractory, no-option angina | Not; not; not | 12 months | 23 | One group: 1st dose 93.5 ± 20.1 × 106 BM-MNCs (with 2.0 ± 1.4% CD34+ cells); 2nd dose 98.7 ± 6.3 × 106 BM-MNCs (with 1.8 ± 1.2% CD34+ cells), 4.6 ± 2.5 years apart | TO | MRI/SPECT | NA | NA | NA | NA | ↑ | ↑ |
Assmus et al.202b | NA | Ischaemic HF | Not; not; not | 36 months | 297 | Two doses of 190 + 110 × 106 BM-MNCs at 3–6 months vs. one dose | IC | SHFM score | NA | NA | NA | NA | NA | NA |
Vrtovec et al.74 | II/III | Non-ischaemic HF | No; yes; yes | 12 months | 60 | Two doses 6 months apart vs. single dose of 80 × 106 G-CSF-mobilized CD34+ cells | TO | Echo | NS | NS | NA | NA | NS | NA |
REPEAT121c | II/III | Ischaemic cardiomyopathy, EF ≤45% | No; yes; no | 5 years | 81 | Autologous BM-MNCs, one vs. two doses 4 months apart | IC | Follow-up | NA | NA | NA | NA | NA | NA |
Trials . | Phase . | Clinical setting . | Placebo controlled; randomized; double-blind . | Follow-up . | n . | Cell type, dose, and treatment groups . | Delivery method . | Endpoint evaluation . | LVEF . | LV volumes . | Scar size . | NYHA class . | Functional capacity . | QoL . |
---|---|---|---|---|---|---|---|---|---|---|---|---|---|---|
DanCell-CHF Diederichsen et al.198 | II | Ischaemic HF | Not; not; not | 12 months | 32 | One group: 647 ± 382 × 106 (1st dose) and 889 ± 361 × 106 (2nd dose) BM-MNCs, 4 months apart; variables compared before and after treatment | IC | Echo | NS | NS | NA | ↑ | NS | NA |
Yao et al.199 | ? | First STEMI with LVEF 20–39% | Yes; yes; ? | 12 months | 39 | Three groups: single dose 1.9 ± 1.2 × 108; two doses of 2.0 ± 1.4 × 108 and 2.1 ± 1.7 × 108 BM-MNCs 3 months apart; one dose of placebo | IC | MRI/SPECT | 2 doses > 1 dose > control | 2 doses > 1 dose > control | 2 doses > 1 dose > control | NA | NA | NA |
Gu et al.200 | I | Ischaemic HF | Not; ?; not | 12 months | 45 | Control (no intervention) vs. single dose SC G-CSF and single dose (1.5–2 × 108) IV MNCs containing a minimum of 1 × 106 CD34+cells vs. single dose G-CSF and two doses of IV MNCs 6 months apart | IC | Echo/SPECT | 2 doses > 1 dose > control | NA | LVEDV: 2 doses > 1 dose > control | 2 doses > 1 dose or control | NA | NA |
Mann et al.201 a | NA | Refractory, no-option angina | Not; not; not | 12 months | 23 | One group: 1st dose 93.5 ± 20.1 × 106 BM-MNCs (with 2.0 ± 1.4% CD34+ cells); 2nd dose 98.7 ± 6.3 × 106 BM-MNCs (with 1.8 ± 1.2% CD34+ cells), 4.6 ± 2.5 years apart | TO | MRI/SPECT | NA | NA | NA | NA | ↑ | ↑ |
Assmus et al.202b | NA | Ischaemic HF | Not; not; not | 36 months | 297 | Two doses of 190 + 110 × 106 BM-MNCs at 3–6 months vs. one dose | IC | SHFM score | NA | NA | NA | NA | NA | NA |
Vrtovec et al.74 | II/III | Non-ischaemic HF | No; yes; yes | 12 months | 60 | Two doses 6 months apart vs. single dose of 80 × 106 G-CSF-mobilized CD34+ cells | TO | Echo | NS | NS | NA | NA | NS | NA |
REPEAT121c | II/III | Ischaemic cardiomyopathy, EF ≤45% | No; yes; no | 5 years | 81 | Autologous BM-MNCs, one vs. two doses 4 months apart | IC | Follow-up | NA | NA | NA | NA | NA | NA |
Trials are listed in chronological order of publication.
indicates increase; ↓, decrease; BMMNCs, bone marrow mononuclear cells; Echo, echocardiography; EDV, end-diastolic volume; EF, ejection fraction; ESV, end-systolic volume; G-CSF, Granulocyte-colony stimulating factor; HF, heart failure; IC, intracoronary; IV, intravenous; LV, left ventricle; LVEF, left ventricular ejection fraction; MRI, magnetic resonance imaging; n, number of patients; NA, not assessed; NS, not significant; NYHA, New York Heart Association; QoL, quality of life; SHFM, Seattle Heart Failure model; SPECT, single-photon emission tomography; STEMI, ST-elevation myocardial infarction; TE, transendocardial.
Improvement in myocardial perfusion (measured by SPECT), angina, and quality of life score was noted.
Repeated intracoronary infusion of autologous BMMNCs was associated with significantly better 2-year survival compared with a single BMMNC infusion. At the 3-year follow-up, the trend persisted but the mortality reduction was no longer statistically significant between single and repeated treatment. Additionally, mortality was significantly lower at the 2-year follow-up compared with the mortality estimated using the Seattle Heart Failure Model (SHFM) in patients receiving repeated BMMNC infusions.
Study will be completed in 2022. Primary endpoint: mortality at 2 years; secondary endpoint: Morbidity [cardiac and cardiovascular mortality, HF hospitalization, ischaemic cardiac events, coronary revascularization, cardiac transplantation, assisted device implantation, new synchronization therapy, ICD implantation, NYHA status, MLHFQ, bleeding events, all in-hospital events (during hospitalization for BMC therapy), life-threatening arrhythmias, new malignancies].
Clinical trials of repeated cell therapy in ischaemic or non-ischaemic cardiomyopathy
Trials . | Phase . | Clinical setting . | Placebo controlled; randomized; double-blind . | Follow-up . | n . | Cell type, dose, and treatment groups . | Delivery method . | Endpoint evaluation . | LVEF . | LV volumes . | Scar size . | NYHA class . | Functional capacity . | QoL . |
---|---|---|---|---|---|---|---|---|---|---|---|---|---|---|
DanCell-CHF Diederichsen et al.198 | II | Ischaemic HF | Not; not; not | 12 months | 32 | One group: 647 ± 382 × 106 (1st dose) and 889 ± 361 × 106 (2nd dose) BM-MNCs, 4 months apart; variables compared before and after treatment | IC | Echo | NS | NS | NA | ↑ | NS | NA |
Yao et al.199 | ? | First STEMI with LVEF 20–39% | Yes; yes; ? | 12 months | 39 | Three groups: single dose 1.9 ± 1.2 × 108; two doses of 2.0 ± 1.4 × 108 and 2.1 ± 1.7 × 108 BM-MNCs 3 months apart; one dose of placebo | IC | MRI/SPECT | 2 doses > 1 dose > control | 2 doses > 1 dose > control | 2 doses > 1 dose > control | NA | NA | NA |
Gu et al.200 | I | Ischaemic HF | Not; ?; not | 12 months | 45 | Control (no intervention) vs. single dose SC G-CSF and single dose (1.5–2 × 108) IV MNCs containing a minimum of 1 × 106 CD34+cells vs. single dose G-CSF and two doses of IV MNCs 6 months apart | IC | Echo/SPECT | 2 doses > 1 dose > control | NA | LVEDV: 2 doses > 1 dose > control | 2 doses > 1 dose or control | NA | NA |
Mann et al.201 a | NA | Refractory, no-option angina | Not; not; not | 12 months | 23 | One group: 1st dose 93.5 ± 20.1 × 106 BM-MNCs (with 2.0 ± 1.4% CD34+ cells); 2nd dose 98.7 ± 6.3 × 106 BM-MNCs (with 1.8 ± 1.2% CD34+ cells), 4.6 ± 2.5 years apart | TO | MRI/SPECT | NA | NA | NA | NA | ↑ | ↑ |
Assmus et al.202b | NA | Ischaemic HF | Not; not; not | 36 months | 297 | Two doses of 190 + 110 × 106 BM-MNCs at 3–6 months vs. one dose | IC | SHFM score | NA | NA | NA | NA | NA | NA |
Vrtovec et al.74 | II/III | Non-ischaemic HF | No; yes; yes | 12 months | 60 | Two doses 6 months apart vs. single dose of 80 × 106 G-CSF-mobilized CD34+ cells | TO | Echo | NS | NS | NA | NA | NS | NA |
REPEAT121c | II/III | Ischaemic cardiomyopathy, EF ≤45% | No; yes; no | 5 years | 81 | Autologous BM-MNCs, one vs. two doses 4 months apart | IC | Follow-up | NA | NA | NA | NA | NA | NA |
Trials . | Phase . | Clinical setting . | Placebo controlled; randomized; double-blind . | Follow-up . | n . | Cell type, dose, and treatment groups . | Delivery method . | Endpoint evaluation . | LVEF . | LV volumes . | Scar size . | NYHA class . | Functional capacity . | QoL . |
---|---|---|---|---|---|---|---|---|---|---|---|---|---|---|
DanCell-CHF Diederichsen et al.198 | II | Ischaemic HF | Not; not; not | 12 months | 32 | One group: 647 ± 382 × 106 (1st dose) and 889 ± 361 × 106 (2nd dose) BM-MNCs, 4 months apart; variables compared before and after treatment | IC | Echo | NS | NS | NA | ↑ | NS | NA |
Yao et al.199 | ? | First STEMI with LVEF 20–39% | Yes; yes; ? | 12 months | 39 | Three groups: single dose 1.9 ± 1.2 × 108; two doses of 2.0 ± 1.4 × 108 and 2.1 ± 1.7 × 108 BM-MNCs 3 months apart; one dose of placebo | IC | MRI/SPECT | 2 doses > 1 dose > control | 2 doses > 1 dose > control | 2 doses > 1 dose > control | NA | NA | NA |
Gu et al.200 | I | Ischaemic HF | Not; ?; not | 12 months | 45 | Control (no intervention) vs. single dose SC G-CSF and single dose (1.5–2 × 108) IV MNCs containing a minimum of 1 × 106 CD34+cells vs. single dose G-CSF and two doses of IV MNCs 6 months apart | IC | Echo/SPECT | 2 doses > 1 dose > control | NA | LVEDV: 2 doses > 1 dose > control | 2 doses > 1 dose or control | NA | NA |
Mann et al.201 a | NA | Refractory, no-option angina | Not; not; not | 12 months | 23 | One group: 1st dose 93.5 ± 20.1 × 106 BM-MNCs (with 2.0 ± 1.4% CD34+ cells); 2nd dose 98.7 ± 6.3 × 106 BM-MNCs (with 1.8 ± 1.2% CD34+ cells), 4.6 ± 2.5 years apart | TO | MRI/SPECT | NA | NA | NA | NA | ↑ | ↑ |
Assmus et al.202b | NA | Ischaemic HF | Not; not; not | 36 months | 297 | Two doses of 190 + 110 × 106 BM-MNCs at 3–6 months vs. one dose | IC | SHFM score | NA | NA | NA | NA | NA | NA |
Vrtovec et al.74 | II/III | Non-ischaemic HF | No; yes; yes | 12 months | 60 | Two doses 6 months apart vs. single dose of 80 × 106 G-CSF-mobilized CD34+ cells | TO | Echo | NS | NS | NA | NA | NS | NA |
REPEAT121c | II/III | Ischaemic cardiomyopathy, EF ≤45% | No; yes; no | 5 years | 81 | Autologous BM-MNCs, one vs. two doses 4 months apart | IC | Follow-up | NA | NA | NA | NA | NA | NA |
Trials are listed in chronological order of publication.
indicates increase; ↓, decrease; BMMNCs, bone marrow mononuclear cells; Echo, echocardiography; EDV, end-diastolic volume; EF, ejection fraction; ESV, end-systolic volume; G-CSF, Granulocyte-colony stimulating factor; HF, heart failure; IC, intracoronary; IV, intravenous; LV, left ventricle; LVEF, left ventricular ejection fraction; MRI, magnetic resonance imaging; n, number of patients; NA, not assessed; NS, not significant; NYHA, New York Heart Association; QoL, quality of life; SHFM, Seattle Heart Failure model; SPECT, single-photon emission tomography; STEMI, ST-elevation myocardial infarction; TE, transendocardial.
Improvement in myocardial perfusion (measured by SPECT), angina, and quality of life score was noted.
Repeated intracoronary infusion of autologous BMMNCs was associated with significantly better 2-year survival compared with a single BMMNC infusion. At the 3-year follow-up, the trend persisted but the mortality reduction was no longer statistically significant between single and repeated treatment. Additionally, mortality was significantly lower at the 2-year follow-up compared with the mortality estimated using the Seattle Heart Failure Model (SHFM) in patients receiving repeated BMMNC infusions.
Study will be completed in 2022. Primary endpoint: mortality at 2 years; secondary endpoint: Morbidity [cardiac and cardiovascular mortality, HF hospitalization, ischaemic cardiac events, coronary revascularization, cardiac transplantation, assisted device implantation, new synchronization therapy, ICD implantation, NYHA status, MLHFQ, bleeding events, all in-hospital events (during hospitalization for BMC therapy), life-threatening arrhythmias, new malignancies].
The only study of repeated cell therapy in non-ischaemic cardiomyopathy is REMEDIUM, published in 2018, a Phase II-III randomized, double-blind trial that compared the effects of one or two transendocardial injections of CD34+ cells (given 6 months apart) in 60 patients with non-ischaemic cardiomyopathy.74 Patients received BM stimulation with G-CSF, following which 80 × 106 CD34+ cells were collected by apheresis and injected transendocardially. At 1 year after the first dose (6 months after the second dose), the improvement in LVEF, LV volumes, exercise tolerance (6-min walking distance), and NT-proBNP did not differ between patients given a second cell dose and those given a single dose. However, the characteristics of the patients before the second injection were different from those before the first, making comparisons difficult. For example, LVEF was significantly higher before the second injection (39.1%) than before the first (31.2%); this is relevant because meta-analyses suggest that a lower LVEF is associated with a better response to cell therapy.203 Indeed, subgroup analysis of responders (patients in whom LVEF increased at least 5%) vs. non-responders revealed that LVEF prior to the second cell injection was significantly lower in the 10 patients who responded to the second injection than in the 20 who did not (34.9% vs. 40.0%; P = 0.02), suggesting that there may be a ceiling effect for cell therapy and that in most patients the LVEF before the second dose was not low enough to improve after that dose. In addition, responders exhibited an increase in myocardial viability after the first dose (as assessed by unipolar voltage mapping with the NOGA system) whereas non-responders did not.74 Since the response to transendocardial CD34+ cell injection in HF depends on the LV scar burden,204 it is possible that the effects of the first dose altered the effects of the second dose.
In summary, the potential therapeutic utility of repeated cell therapy remains to be adequately assessed. The available clinical data are scarce and inconsistent. The studies reviewed above74,198–202 were limited by the use of echocardiography to assess LV function, the lack of a true control group, and the open-label design; except for one study in patients acute MI,199 in all of the other trials the single-dose group did not receive a second (placebo) infusion. To date, no study has evaluated the effects of repeated cell therapy in patients with HF in a randomized, blinded, placebo-controlled fashion, and none has tested >2 doses of cells. Overcoming these limitations will be arduous. The invasive delivery techniques currently used (intracoronary or transendocardial injection) make it very difficult to examine >2 doses and to conduct placebo-controlled, double-blinded studies. If intravenous administration of cells proves effective, it would make it possible to test >2 doses with a double-blind design.
3.10 Moving away from using LV function as the primary endpoint
Based on the results of animal data,23 most clinical trials of cell therapy in HF have used LV function as the primary endpoint (Tables 1–5). Mounting evidence, however, suggests that cell therapy can impart beneficial effects to HF patients without necessarily augmenting common indices of LV function (Figure 2). For example, among placebo-controlled, double-blind, randomized Phase II trials in ischaemic HF, some have reported an improvement in LV function with cell treatment (e.g. MSC-HF54,55) but others have not: patients receiving cell therapy exhibited improved functional capacity and quality of life in TAC-HF,42 improved quality of life in ATHENA46 and CONCERT-HF,60 and reduced mortality and cardiovascular hospitalization in ixCELL-DCM45 and CONCERT-HF,60 yet none of these studies detected a change in LV function (Figure 2; Table 1). DREAM-HF (NCT 02032004),62 the largest study of cell therapy in HF, has also reported a reduction in MACE (vide supra).63 As discussed earlier, HF is a systemic inflammatory disease29–31 and MSCs have anti-inflammatory properties8,88,92–94; therefore, it is possible that MSC therapy could alleviate the outcome of HF via systemic actions that do not necessarily result in augmented LV function.25 Because of this, and because clinical outcome is more relevant to patients than surrogate endpoints such as LVEF or LVESV, there has been a shift away from LV function as the primary endpoint for cell therapy trials in HF.62
4. Conclusions
The utility of cell therapy in HF remains to be determined. Many Phase I and II trials have been conducted but pivotal Phase III trials have not been published. The available evidence can be summarized as follows (Table 6):
Current status of various cell types used for the treatment of heart failure
. | Safety . | Efficacy . | Ongoing Phase III trials . | Notes . | |
---|---|---|---|---|---|
Ischaemic cardiomyopathy . | Non-ischaemic cardiomyopathy . | ||||
BM-MNCs | + | – | – | – | Abandoned |
BM-MSCs | + | ++ | ++ | * | |
Cardiopoietic BM-MSCs | + | – | – | – | |
CPCs | + | + | No data | – | Only one study (CONCERT-HF) |
UC-MSCs | + | + | + | – | |
ADRCs | + | + | No data | – | Phase II trials ongoing |
CD34+ cells | + | No data | + | – | Phase II trials have shown efficacy for refractory angina |
CDCs | + | – | No data | Abandoned | |
ixmyelocel-T | + | ++ | No data | – | |
ESCs | No data | No data | No data | – | Serious safety concerns; need for immunosuppressive therapy |
. | Safety . | Efficacy . | Ongoing Phase III trials . | Notes . | |
---|---|---|---|---|---|
Ischaemic cardiomyopathy . | Non-ischaemic cardiomyopathy . | ||||
BM-MNCs | + | – | – | – | Abandoned |
BM-MSCs | + | ++ | ++ | * | |
Cardiopoietic BM-MSCs | + | – | – | – | |
CPCs | + | + | No data | – | Only one study (CONCERT-HF) |
UC-MSCs | + | + | + | – | |
ADRCs | + | + | No data | – | Phase II trials ongoing |
CD34+ cells | + | No data | + | – | Phase II trials have shown efficacy for refractory angina |
CDCs | + | – | No data | Abandoned | |
ixmyelocel-T | + | ++ | No data | – | |
ESCs | No data | No data | No data | – | Serious safety concerns; need for immunosuppressive therapy |
, Trials have failed to show efficacy.
Phase I-II trials have shown efficacy.
Two or more Phase I-II trials have shown efficacy.
DREAM-HF completed but not yet published.
ADRCs, adipose-derived regenerative cells; BM-MNCs, bone marrow-derived mononuclear cells; BM-MSCs, bone marrow-derived mesenchymal stem/stromal cells; CDCs, cardiosphere-derived cells; CPCs, c-kit-positive cardiac cells; ESCs, embryonic stem cells; UC-MSCs, umbilical cord mesenchymal stem cells.
Current status of various cell types used for the treatment of heart failure
. | Safety . | Efficacy . | Ongoing Phase III trials . | Notes . | |
---|---|---|---|---|---|
Ischaemic cardiomyopathy . | Non-ischaemic cardiomyopathy . | ||||
BM-MNCs | + | – | – | – | Abandoned |
BM-MSCs | + | ++ | ++ | * | |
Cardiopoietic BM-MSCs | + | – | – | – | |
CPCs | + | + | No data | – | Only one study (CONCERT-HF) |
UC-MSCs | + | + | + | – | |
ADRCs | + | + | No data | – | Phase II trials ongoing |
CD34+ cells | + | No data | + | – | Phase II trials have shown efficacy for refractory angina |
CDCs | + | – | No data | Abandoned | |
ixmyelocel-T | + | ++ | No data | – | |
ESCs | No data | No data | No data | – | Serious safety concerns; need for immunosuppressive therapy |
. | Safety . | Efficacy . | Ongoing Phase III trials . | Notes . | |
---|---|---|---|---|---|
Ischaemic cardiomyopathy . | Non-ischaemic cardiomyopathy . | ||||
BM-MNCs | + | – | – | – | Abandoned |
BM-MSCs | + | ++ | ++ | * | |
Cardiopoietic BM-MSCs | + | – | – | – | |
CPCs | + | + | No data | – | Only one study (CONCERT-HF) |
UC-MSCs | + | + | + | – | |
ADRCs | + | + | No data | – | Phase II trials ongoing |
CD34+ cells | + | No data | + | – | Phase II trials have shown efficacy for refractory angina |
CDCs | + | – | No data | Abandoned | |
ixmyelocel-T | + | ++ | No data | – | |
ESCs | No data | No data | No data | – | Serious safety concerns; need for immunosuppressive therapy |
, Trials have failed to show efficacy.
Phase I-II trials have shown efficacy.
Two or more Phase I-II trials have shown efficacy.
DREAM-HF completed but not yet published.
ADRCs, adipose-derived regenerative cells; BM-MNCs, bone marrow-derived mononuclear cells; BM-MSCs, bone marrow-derived mesenchymal stem/stromal cells; CDCs, cardiosphere-derived cells; CPCs, c-kit-positive cardiac cells; ESCs, embryonic stem cells; UC-MSCs, umbilical cord mesenchymal stem cells.
In contrast to acute MI (where trials have been consistently negative for more than a decade),205 in the setting of HF the results of cell therapy trials are more encouraging, both in the setting of ischaemic and non-ischaemic cardiomyopathy (Table 6).
Contrary to misleading assertions by anonymous editorialists,32 several well-designed Phase II trials (e.g. MSC-HF,54,55 ix CELL-DCM,45 REGENERATE-DCM).9 have met their primary endpoint and demonstrated an efficacy signal in HF4 (Tables 1 and 2). CONCERT-HF60 and DREAM-HF63 also support efficacy. This is remarkable, considering that in these studies only one dose of cells was used and the outcome measured a year later. That an efficacy signal was seen long after treatment provides a rationale for larger, rigorous trials.
The best endpoint in HF trials remains unclear, as different studies have reported improvement in different endpoints, such as LV function,9,54,55 MACE,45,60,63 and quality of life.9,54,55,60,76 Differences in cell products and patient populations may account for these differences.
What is needed now is rigorous, well-designed Phase III trials to conclusively address the issue of efficacy and identify the appropriate endpoint.
Importantly, no concerns have emerged regarding the safety of adult cell therapy in HF (Table 6).
Amongst all the various cell types being tested, MSCs show the greatest promise as a treatment for HF. Although not yet tested clinically, induced pluripotent stem cells may turn out to be useful. In contrast, BM-MNCs, CDCs, and embryonic stem cells are not likely to become a clinical therapy for HF (Table 6).
Effective cell therapies will most likely be allogeneic.
New approaches, such as multiple doses and intravenous delivery, may revolutionize the field.
As is the case for most new therapies, the development of cell therapy for HF has been slow, difficult, and punctuated by setbacks. The most important things to move forward are to have an open mind, avoid preconceived notions, and let ourselves be guided by the evidence.
Funding
This work was supported by the National Institutes of Health (P01 HL078825 and UM1 HL113530).
Conflict of interest: none declared.
References
Capricor_Inc. Dilated cardiomYopathy iNtervention With Allogeneic MyocardIally-regenerative Cells (DYNAMIC) (DYNAMIC). ClinicalTrialsgov identifier (NCT number): NCT02293603.
,
Mesoblast the regenerative medicine company, Rexlemestrocel-L for Cardiovascular Diseases - Heart Failure Phase 3 Trial Top Line Results. http://investorsmedia.mesoblast.com/static-files/4e34db52-9d78-414f-904b-5b733aee8b6a.
Rebecca_Torguson. Serial Infusions of Allogeneic Mesenchymal Stem Cells in Cardiomyopathy Patients with Left Ventricular Assist Device (STEM-VAD). ClinicalTrialsgov identifier (NCT number): NCT03925324.
JKastrup. Allogeneic Stem Cell Therapy in Heart Failure (CSCC_ASCII). ClinicalTrialsgov identifier (NCT number): NCT03092284.
Johann_Wolfgang_Goethe_University_Hospital. Compare the Effects of Single Versus Repeated Intracoronary Application of Autologous Bone Marrow-derived Mononuclear Cells on Mortality in Patients with Chronic Post-infarction Heart Failure (REPEAT). ClinicalTrialsgov identifier (NCT number): NCT01693042.
The Lancet Editors.