-
PDF
- Split View
-
Views
-
Cite
Cite
Sam Ng, Sylvie Moritz-Gasser, Anne-Laure Lemaitre, Hugues Duffau, Guillaume Herbet, Multivariate mapping of low-resilient neurocognitive systems within and around low-grade gliomas, Brain, Volume 147, Issue 8, August 2024, Pages 2718–2731, https://doi.org/10.1093/brain/awae130
- Share Icon Share
Abstract
Accumulating evidence suggests that the brain exhibits a remarkable capacity for functional compensation in response to neurological damage, a resilience potential that is deeply rooted in the malleable features of its underlying anatomofunctional architecture. This propensity is particularly exemplified by diffuse low-grade glioma, a subtype of primary brain tumour. However, functional plasticity is not boundless, and surgical resections directed at structures with limited neuroplasticity can lead to incapacitating impairments. Yet, maximizing diffuse low-grade glioma resections offers substantial oncological benefits, especially when the resection extends beyond the tumour margins (i.e. supra-tumour or supratotal resection). In this context, the primary objective of this study was to identify which cerebral structures were associated with less favourable cognitive outcomes after surgery, while accounting for intra-tumour and supra-tumour features of the surgical resections.
To achieve this objective, we leveraged a unique cohort of 400 patients with diffuse low-grade glioma who underwent surgery with awake cognitive mapping. Patients benefitted from a neuropsychological assessment consisting of 18 subtests administered before and 3 months after surgery. We analysed changes in performance and applied topography-focused and disconnection-focused multivariate lesion–symptom mapping using support vector regressions, in an attempt to capture resected cortico-subcortical structures less amenable to full cognitive compensation.
The observed changes in performance were of a limited magnitude, suggesting an overall recovery (13 of 18 tasks recovered fully despite a mean resection extent of 92.4%). Nevertheless, lesion–symptom mapping analyses revealed that a lack of recovery in picture naming was linked to damage in the left inferior temporal gyrus and inferior longitudinal fasciculus. Likewise, for semantic fluency abilities, an association was established with damage to the left precuneus/posterior cingulate. For phonological fluency abilities, the left dorsomedial frontal cortex and the frontal aslant tract were implicated. Moreover, difficulties in spatial exploration were associated with injury to the right dorsomedial prefrontal cortex and its underlying connectivity. An exploratory analysis suggested that supra-tumour resections were associated with a less pronounced recovery following specific resection patterns, such as supra-tumour resections of the left uncinate fasciculus (picture naming), the left corticostriatal tract and the anterior corpus callosum (phonological fluency), the hippocampus and parahippocampus (episodic memory) and the right frontal–mesial areas (visuospatial exploration).
Collectively, these patterns of results shed new light on both low-resilient neural systems and the prediction of cognitive recovery following glioma surgery. Furthermore, they indicate that supra-tumour resections were only occasionally less well tolerated from a cognitive viewpoint. In doing so, they have deep implications for surgical planning and rehabilitation strategies.
Introduction
The cerebral architecture is fundamentally characterized by the intricate interplay of distributed, parallel and gradually specialized networks, dynamically orchestrated to enable the emergence of a wide array of complex behaviours.1-3 The capacity of these systems to adapt and restore functions after cerebral damage is contingent not only upon the nature of the lesion itself4,5 but also upon the topological distribution of the lesion.6-8 Chronic brain lesions, notably diffuse low-grade gliomas (DLGGs), prominently exemplify this propensity, because they initiate widespread compensatory adaptations through various neuroplasticity strategies. These primary brain tumours, characterized by slow-growth kinetics and shared histomolecular signatures,9 have been observed to integrate into surrounding neural circuits through cellular, chemical and electrical coupling,10,11 resulting in reorganizations of the functional architecture.12 Such malleability is inherently constrained at the topological scale because essential structures, such as white matter tracts, are necessary to facilitate long-range electrical interactions that underlie functional remodelling.7,13 Furthermore, certain critical systems exhibit restricted intrinsic plasticity capabilities owing to their heightened cytoarchitectural specialization, as seen in unimodal areas. This observation reinforces the perspective that glioma-induced neuroplastic potential is intrinsically routed in the underlying brain anatomy.14
In the clinical management of DLGGs, surgery plays a pivotal role in controlling tumour expansion.15,16 Extensive tissue resections, often including the peri-tumour zone (referred to as supra-tumour resections, i.e. surgical removal of brain tissue that extends beyond margins identified with MRI) have been demonstrated to improve long-term survival significantly, especially in the event of supratotal resection (i.e. complete removal of the tumour, associated with an additional margin of supra-tumour resection).17,18 Yet, the decision for surgical intervention always involves a dilemma: should surgical resections of tumour and supra-tumour tissue be prioritized owing to the survival benefits they offer? Or should neurosurgeons prioritize preservation of cognitive functions, with the goal of fully maintaining post-surgical quality of life, especially for patients seeking to resume active professional and social lives? Intraoperative direct electrostimulation (DES) mapping in awake conditions has been established as the most efficient approach to finding an optimal trade-off between these two dual priorities (i.e. ‘onco-functional balance’19). This procedure allows real-time, in vivo mapping of essential cerebral functions, including motor and language functions,20 among many others,21 thereby enabling assessment of the degree to which the intra-tumour and peri-tumour tissue has been functionally reorganized. Consequently, it provides the opportunity to optimize resection boundaries without the risk of inducing debilitating disorders following surgery, thus allowing >86% of cognitive preservation, as recently demonstrated in a longitudinal cohort study.21
In this context, however, the existing body of literature falls short of addressing some important issues. First, the link between cognitive outcomes and the topological distribution of surgical resections in DLGG patients has not been formally established through large cohort studies by means of longitudinal behaviour measures. This is a prerequisite for anticipating the likelihood of cognitive recovery after surgery, for building patient-specific intraoperative mapping and post-surgery rehabilitation strategies and for developing new, evidence-based intraoperative cognitive tasks, especially given that 14% of patients can suffer from cognitive worsening.21 Second, the possible disparity in plasticity potential between intra-tumour tissue and peri-tumour tissue has never been investigated thoroughly. A characterization of the ability of brain circuits to compensate functionally for resection in the peri-tumour zones might help to orient current surgical strategies (e.g. the use of non-invaded brain corridors to access deep-sited tumours)22 and discuss the feasibility and risks of supra-tumour resections, considering both neuroanatomical and functional perspectives.
Here, we addressed these gaps by leveraging an unprecedented cohort comprising 400 patients with DLGGs who underwent surgery assisted by intraoperative awake mapping. These patients also benefitted from longitudinal neuropsychological assessments at two critical time points: prior to surgery and 3 months post-surgery. These assessments encompassed 18 cognitive subtasks. Our methodology involved two primary lines of analysis, each with distinct objectives. In the first one, we used resection-focused and disconnection-focused multivariate lesion–symptom analyses, using support vector regressions. This approach, which is a machine learning-based evolution of conventional mass-univariate lesion mapping techniques,23 considers the interdependent nature of voxels within a given lesion or disconnection map. It has recently gained prominence in the field, outperforming previous univariate methods, and has found application in diverse domains, such as stroke,24-28 epilepsy29 and gliomas.30 In a second line of analysis, our aim was to assess the influence of the extent of intra- and supra-tumour resected voxels on cognitive variations. We conducted a parallel analysis to investigate the role of disconnective disruptions, using indirect disconnection measures based on a population-averaged diffusion dataset. These combined approaches were used to unveil the vulnerable cerebral circuits that adversely modulate cognitive recovery in DLGG patients undergoing surgery, with a particular interest in identifying specific contributions of intra-tumour and supra-tumour resections/disconnections to cognitive outcomes.
Materials and methods
Study population
Data processed in this study were acquired in a clinical context, and all procedures outlined later (including neurosurgical procedures, neuroimaging acquisition and neuropsychological examinations) adhere to the standard of care in our medical department. The collection of data and the retrospective analysis were approved by our Institutional Ethics Commission (collection NEUROLOGIE DC-2013-2027) and an independent Institutional Review Board (National French College of Neurosurgery no. 00011687-2021/18). All patients gave their informed consent.
Eligible participants for this study included adult patients with histopathologically confirmed DLGG who underwent surgical resection with awake cognitive mapping at Montpellier University Medical Centre (Montpellier, France) between September 2012 and January 2023. The inclusion criteria encompassed: (i) a verified DLGG diagnosis through histomolecular analysis; (ii) surgical resection assisted by awake intraoperative functional mapping using DES; and (iii) completion of pre- and 3-month postoperative cognitive assessments. Exclusion criteria comprised: (i) a history of prior brain radiotherapy or surgery; (ii) pre-existing neurological or neurodevelopmental diseases (e.g. stroke); and (iii) non-French-speaking patients.
Surgical procedure
All patients underwent resection surgery with intraoperative awake cognitive mapping using DES, following an asleep–awake–asleep protocol that has been thoroughly described elsewhere.31,32 In short, DES mapping was conducted during the awake phase of the procedure, subsequent to craniotomy and dura opening. A bipolar probe (NIMBUS stimulator, Newmedic, 5 mm inter-tip spacing) was used, delivering biphasic current (60 Hz, 1 ms pulse width, amplitude ranging from 1.50 to 3.50 mA). Cortical and subcortical functional responses were used as resection boundaries, following the principle of functionally guided tumour resection. In other words, the extension of cortical and white matter resection was halted whenever DES-induced transient disturbances in motor, language or cognition occurred at each edge of the resection cavity. To be established as a ‘definite boundary’, these DES-induced disturbances had to be replicated at least three times in a non-consecutive manner. Intraoperative monitoring included upper/lower limb movements, counting, picture naming (DO), semantic association (Pyramid and Palm Trees Test), line bisection, reading, emotion recognition, self-evaluation and dual or triple combination of tasks.32 It was achieved by a senior speech therapist or neuropsychologist who maintained blindness to the application of DES.
Behavioural analyses
Patients underwent neuropsychological assessments and language examinations at standardized end points, specifically the day before surgery and 3 months post-surgery. These evaluations were administered by the same neuropsychologist and/or speech therapist. Detailed information about the 18 cognitive tasks is provided in the Supplementary materials.
Preoperative and 3-month postoperative raw and z-transformed (according to French normative data, taking into consideration age, sex and educational level) cognitive measures were subjected to statistical comparison using non-parametric Wilcoxon matched-pairs statistics to assess within-subject differences. Additionally, the proportion of patients exhibiting a deficit, defined as z ≤ 1.65 (representing the lower 5% of the reference population), was computed in pre- and postoperative datasets.
Imaging acquisition, normalization and lesion drawing
T1-weighted and fluid-attenuated inversion recovery (FLAIR) MRIs were acquired systematically on the day before surgery and 3 months post-surgery. Further details regarding neuroimaging acquisition can be found in the Supplementary material. To mitigate potential bias arising from abnormal lesion-related neuroimaging signals, all images were co-registered to the Montreal Neurological Institute (MNI) space using enantiomorphic normalization33 with the Clinical toolbox (https://www.nitrc.org/projects/clinicaltbx) and SPM12 (https://www.fil.ion.ucl.ac.uk/spm/software/spm12) implemented in the MATLAB environment (Release 2022a, The MathWorks Inc., Natick, MA, USA). Subsequent to image normalization, the tumour infiltration map was delineated manually using preoperative FLAIR images using MRIcron software (http://www.mccauslandcenter.sc.edu/mricro/mricron). The resection cavity map was generated using the same approach, using 3-month postoperative T1-weighted images. The resulting three-dimensional volumes of interest were then used to compute binary operations in the FMRIB Software Library program (FSL, v.6.0; https://fsl.fmrib.ox.ac.uk/fsl). In summary, the dataset for each patient yielded the following binary masks (voxel resolution 1 mm isometric): (i) the tumour infiltration mask; (ii) the resection cavity mask; (iii) the intra-tumour resection mask (i.e. overlapping voxels within the resection and infiltration masks); and (iv) the supra-tumour resection mask (i.e. voxels within the resection mask but outside the tumour infiltration mask). A graphical illustration of this workflow and representative examples of lesion segmentations are provided in Supplementary Fig. 1.
Disconnection maps and tract disconnection index
To measure the severity with which the resection surgery damaged the main white matter tracts, we used the lesion quantification toolkit (LQT),34 which is a recent toolbox implemented in MATLAB environment. The resection cavity masks, the intra-tumour resection masks and the supra-tumour resection masks were embedded into the human connectome project (HCP) 842 tractography atlas35 to quantify the extent of disconnection experienced by each of the 70 canonical white matter tracts. Two sets of data were generated to gauge the relationships between structural disconnections and cognitive measurements: (i) the TDI (tract density imaging) map, a whole-brain, severity-based disconnection map, in which surgery-related reduction in white matter streamline density is expressed at each voxel with reference to the population-averaged HCP white matter atlas; and (ii) the tract disconnection index, i.e. the percentage of streamlines intersecting the lesion for each analysed white matter tract.
Multivariate topography-focused and disconnection-focused lesion–symptom mapping
The relationship between resection cavity masks and cognitive outcomes (i.e. the cognitive variation between preoperative and at 3-month postoperative status) was explored through support vector regression-based lesion–symptom mapping (SVR-LSM).36 Radial basis function-based SVR-LSM analyses were conducted with the DeMarco and Turkeltaub toolbox,37 implemented in MATLAB. A fine-tuning of SVR-LSM model hyperparameters was performed by means of a 5-fold cross-validation procedure in tandem with a grid search approach. This optimization strategy entailed scrutinizing cost function (i.e. penalizing/regularization parameter) and gamma (radial basis function parameter) within recommended ranges (C range: 1–50, γ range: 0.1–10), resulting in the evaluation of 66 models. More specifically, pairs of hyperparameters were selected carefully to optimize the balance between prediction accuracy and reproducibility of SVR-LSM maps. Importantly, datasets for which models did not meet the dual minimum requirement of r < 0.20 for accuracy and r < 0.80 for reproducibility were excluded from further processing. The potential impact of resection volumes on output statistical maps was controlled systematically by using the DTLVC option (direct total lesion volume control), as originally proposed by Zhang et al.36 Unwanted variance associated with age and educational attainment was factored out systematically using nuisance models. The resulting SVR β-maps were subjected to a 10000-permutation procedure to derive P-maps. These statistical maps were corrected for multiple comparisons by two approaches, both of which are commonly adopted in the current literature. The conservative approach consisted in applying a continuous permutation-based familywise error correction with P < 0.05 and volume (V) = 10 mm3. This enabled us to identify a first subset of regions of interest (mentioned hereafter as ROIa). The second, more lenient, approach taken to threshold the permutation-derived P-maps using an uncorrected voxelwise P < 0.001 and clusterwise P < 0.05, resulting in a second, more inclusive, subset of regions of interest (hereafter referred to as ROIb).
It is noteworthy that an identical methodological procedure was applied using preoperative masks and preoperative levels of cognitive performance. This was undertaken to investigate whether lesion–symptom relationships could already be observed before the surgical procedure.
In a second set of analyses, the identical analysis pipeline was performed on continuous TDI maps [i.e. SVR disconnection–lesion symptom mapping (SVR-DSM)] to assess the voxelwise relationship between disconnection severity and cognitive outcomes. We thus derived two subsets of areas of disconnections of interest (DISCOa, i.e. a more conservative one, and DISCOb, i.e. a more lenient one).
Effects of intra-tumour and supra-tumour resection
To gauge the individual contributions of intra-tumour and supra-tumour resections (i.e. resections beyond FLAIR-defined tumour margins), we used two additional methodological frameworks.
First, we generated supra-tumour resection probability maps by computing the ratio of intra-tumour resections to supra-tumour resections in each voxel. More specifically, the supra-tumour resection probability value in a voxel, x, was defined as the ratio of the number of supra-tumour resections in x to the total number of resections (both intra- and supra-tumour resections) in x. Additional methodological details are available in the Supplementary material.
Second, to test the hypothesis that supra-tumour resections might contribute differently to behavioural changes compared with intra-tumour resections, we computed non-parametric Spearman correlations between the total number of resected voxels (brain-wide), the number of resected voxels within ROIa, and the number of resected voxels within ROIb on the one hand and cognitive measurements on the other hand. The same analysis was conducted with supra-tumour and intra-tumour resected voxels. These correlation analyses were performed uniquely with cognitive tasks associated with significant SVR-LSM results. It is important to note that residualized cognitive measures, obtained through multiple linear regressions, were used to account for age and educational level (similar to SVR-LSM analyses). To address the issue of multiple correlation analyses, a false discovery rate (FDR) correction was applied, setting the threshold at q = 0.05.
Effects of intra-tumour and supra-tumour disconnection
The tract disconnection index, reflecting the percentage of fibres intersecting the resection map based on the HCP-derived white matter tracts, was used to determine the magnitude of surgically induced disconnections. To mitigate the risk of inflating the type I error and unwarranted multiple comparisons owing to a priori selection biases, we selectively retained the 15 white matter tracts with the highest average disconnection indices. This included the uncinate fasciculus (UF), the inferior fronto-occipital fasciculus (IFOF), the inferior longitudinal fasciculus (ILF) and the extreme capsule (EMC), the anterior commissure (AC), the frontal aslant tract (FAT) and the cingulate (Cing), the mid-anterior (CCmidant), the anterior (CCant) and the posterior parts (CCpost) of the corpus callosum, the arcuate fasciculus (AF), the corticostriatal tracts (CS), the frontopontine tracts (FPT), the middle longitudinal fasciculus (MdLF) and the superior longitudinal fasciculus (SLF). We next proceeded to non-parametric Spearman correlations between disconnection estimates (from both intra- and supra-tumour masks) and behavioural measures. According to prior analyses (see earlier), these analyses were performed uniquely for each cognitive measurement associated with significant SVR-DSM results. Residualized cognitive measures, obtained through multiple linear regressions, were used to account for age and educational level (similar to SVR-LSM analyses). An FDR correction was applied. Furthermore, we conducted a direct comparison of correlation coefficients associated with intra-tumour resection versus supra-tumour resection using the ‘cocor’38 package implemented in the R environment (https://www.r-project.org/). The correlations selected for comparison were treated as dependent and overlapping (i.e. sharing a common variable, here the cognitive performance). In particular, we followed the procedures described by Zou39 and Hittner et al.40 To ensure the behavioural relevance of our analyses, we compared uniquely the correlations associated with cognitive worsening (in either their intra- and/or supra-tumour counterparts). An FDR correction for multiple comparisons was applied (q = 0.05).
Results
Clinical data
Participants and oncological data
Overall, 400 patients (174 females) were included in the study (right tumours: 166 patients; left tumours: 234 patients), with a mean age of 39.36 ± 1.38 years at the time of surgery and an average educational attainment of 14.58 ± 2.95 years. One hundred and fifty-three tumours (38.3%) were isocitrate dehydrogenase (IDH)-mutated 1p19q co-deleted oligodendrogliomas, 221 (55.3%) were IDH-mutated astrocytomas, 1 (0.3%) was an oligodendroglioma, not otherwise specified (NOS), and 25 (6.3%) were astrocytomas, NOS, according to the 2016 World Health Organization classification. All patients received systematic antiepileptic drug therapy between pre- and post-surgical cognitive assessment. Additional information on the impact of postoperative seizures on cognitive measures is included in Supplementary Figs 2–4 and Supplementary Table 1. In brief, statistical analyses revealed no group difference regarding the proportion of supra-tumour resected voxels when considering the epileptic status, with the notable exception of patients with iterative postoperative epilepsy (n = 10), who generally displayed a lower proportion of supra-tumour resected voxels in comparison to patients without postoperative epilepsy (n = 365, mean rank difference: 93.14, P-adjusted = 0.024, Dunn’s test). Importantly, no statistical difference emerged regarding cognitive outcomes in the subset of patients who presented with postoperative seizure between the presurgical and the 3-month post-surgical cognitive assessment [n = 35 (8.8%)].
Longitudinal behavioural analyses
The longitudinal behavioural data, presented as z-scores after appropriate alignments to French normative data, are shown in Table 1. Differences between the preoperative and the 3-month postoperative assessments were examined systematically using non-parametric, two-tailed Wilcoxon tests without correction for multiple analyses. A noteworthy decline in z-scores was noted for five tasks, including picture naming (W = −4481, P < 0.001), semantic fluency (W = −17197, P < 0.001), Stroop naming (W = −3843, P 0.033), Stroop reading (W = −8900, P < 0.001) and verbal episodic memory total recall (W = −4179, P < 0.001). There was a significant improvement in z-scores in Stroop interference minus denomination (I-D) (W = 4772, P = 0.007). For completeness, among 365 patients with preoperative professional activity, 318 (87.1%) resumed work after surgery. No patients presented with a motor deficit or a severe aphasia 3 months post-surgery. Among patients who met the potential eligibility criteria preoperatively, there were no post-surgical deaths. Raw score comparisons, the rate of patients with cognitive deficits (z-score < −1.65) and additional violin plots are provided in Supplementary Tables 2 and 3 and Supplementary Fig. 5, respectively.
Task . | n . | z-Score (pre) . | z-Score (post) . | Median of difference . | W . | P-value . |
---|---|---|---|---|---|---|
Picture naminga | 223 | 0.11 ± 1.2 | −0.71 ± 3.3 | 0.00 | −4481 | <0.001 |
Semantic fluency | 344 | −0.35 ± 1.1 | −0.61 ± 1.2 | −0.24 | −17197 | <0.001 |
Phonological fluency | 343 | −0.33 ± 1.6 | −0.42 ± 1.3 | −0.15 | −5030 | 0.124 |
TMT-A | 232 | 0.43 ± 0.8 | 0.34 ± 0.9 | 0.00 | −1676 | 0.349 |
TMT-B | 233 | 0.14 ± 0.9 | 0.06 ± 1.4 | 0.05 | 678 | 0.734 |
TMT-B − TMT-A | 232 | −0.16 ± 0.9 | −0.21 ± 1.3 | 0.10 | 1778 | 0.370 |
Stroop naming | 232 | −0.32 ± 1.2 | −0.62 ± 1.9 | −0.10 | −3843 | 0.033 |
Stroop reading | 231 | −0.44 ± 1.5 | −0.88 ± 2.0 | −0.33 | −8900 | <0.001 |
Stroop I | 228 | −0.25 ± 1.4 | −0.27 ± 1.6 | 0.11 | 3203 | 0.071 |
Stroop I-D | 227 | −0.21 ± 1.4 | −0.10 ± 1.4 | 0.15 | 4772 | 0.007 |
PPTTa | 219 | −0.47 ± 1.5 | −0.27 ± 1.5 | 0.00 | 2001 | 0.066 |
Forward span | 208 | −0.06 ± 1.1 | 0.05 ± 1.1 | 0.00 | 984 | 0.221 |
Backward span | 208 | 0.09 ± 1.1 | 0.11 ± 1.1 | 0.00 | 563 | 0.542 |
Free recall (RL-RI) | 165 | −0.51 ± 0.9 | −0.70 ± 1.4 | 0.01 | −107 | 0.929 |
Total recall (RL-RI) | 165 | −0.37 ± 0.5 | −0.72 ± 0.8 | −0.11 | −4179 | <0.001 |
Bell test (omissions)b | 155 | −0.05 ± 1.2 | 0.03 ± 1.1 | 0.00 | 577 | 0.405 |
Rey/Taylor (immediate) | 75 | 0.18 ± 1.1 | −0.10 ± 1.2 | −0.23 | −580 | 0.127 |
Rey/Taylor (delayed) | 73 | 0.22 ± 1.1 | −0.09 ± 1.2 | −0.25 | −455 | 0.213 |
Task . | n . | z-Score (pre) . | z-Score (post) . | Median of difference . | W . | P-value . |
---|---|---|---|---|---|---|
Picture naminga | 223 | 0.11 ± 1.2 | −0.71 ± 3.3 | 0.00 | −4481 | <0.001 |
Semantic fluency | 344 | −0.35 ± 1.1 | −0.61 ± 1.2 | −0.24 | −17197 | <0.001 |
Phonological fluency | 343 | −0.33 ± 1.6 | −0.42 ± 1.3 | −0.15 | −5030 | 0.124 |
TMT-A | 232 | 0.43 ± 0.8 | 0.34 ± 0.9 | 0.00 | −1676 | 0.349 |
TMT-B | 233 | 0.14 ± 0.9 | 0.06 ± 1.4 | 0.05 | 678 | 0.734 |
TMT-B − TMT-A | 232 | −0.16 ± 0.9 | −0.21 ± 1.3 | 0.10 | 1778 | 0.370 |
Stroop naming | 232 | −0.32 ± 1.2 | −0.62 ± 1.9 | −0.10 | −3843 | 0.033 |
Stroop reading | 231 | −0.44 ± 1.5 | −0.88 ± 2.0 | −0.33 | −8900 | <0.001 |
Stroop I | 228 | −0.25 ± 1.4 | −0.27 ± 1.6 | 0.11 | 3203 | 0.071 |
Stroop I-D | 227 | −0.21 ± 1.4 | −0.10 ± 1.4 | 0.15 | 4772 | 0.007 |
PPTTa | 219 | −0.47 ± 1.5 | −0.27 ± 1.5 | 0.00 | 2001 | 0.066 |
Forward span | 208 | −0.06 ± 1.1 | 0.05 ± 1.1 | 0.00 | 984 | 0.221 |
Backward span | 208 | 0.09 ± 1.1 | 0.11 ± 1.1 | 0.00 | 563 | 0.542 |
Free recall (RL-RI) | 165 | −0.51 ± 0.9 | −0.70 ± 1.4 | 0.01 | −107 | 0.929 |
Total recall (RL-RI) | 165 | −0.37 ± 0.5 | −0.72 ± 0.8 | −0.11 | −4179 | <0.001 |
Bell test (omissions)b | 155 | −0.05 ± 1.2 | 0.03 ± 1.1 | 0.00 | 577 | 0.405 |
Rey/Taylor (immediate) | 75 | 0.18 ± 1.1 | −0.10 ± 1.2 | −0.23 | −580 | 0.127 |
Rey/Taylor (delayed) | 73 | 0.22 ± 1.1 | −0.09 ± 1.2 | −0.25 | −455 | 0.213 |
The raw scores for all participants were appropriately aligned to published French normative data (adjusted according to educational level, age and sex) and subsequently converted into z-scores. Wilcoxon matched-pairs signed rank test was used, with a two-tailed P-value, uncorrected. PPTT = Pyramid and Palm Trees Test; RL-RI = rappel libre–rappel indicé; TMT-A/B = Trail Making Test parts A/B.
aOnly patients with left-sided tumours.
bOnly patients with right-sided tumours.
Task . | n . | z-Score (pre) . | z-Score (post) . | Median of difference . | W . | P-value . |
---|---|---|---|---|---|---|
Picture naminga | 223 | 0.11 ± 1.2 | −0.71 ± 3.3 | 0.00 | −4481 | <0.001 |
Semantic fluency | 344 | −0.35 ± 1.1 | −0.61 ± 1.2 | −0.24 | −17197 | <0.001 |
Phonological fluency | 343 | −0.33 ± 1.6 | −0.42 ± 1.3 | −0.15 | −5030 | 0.124 |
TMT-A | 232 | 0.43 ± 0.8 | 0.34 ± 0.9 | 0.00 | −1676 | 0.349 |
TMT-B | 233 | 0.14 ± 0.9 | 0.06 ± 1.4 | 0.05 | 678 | 0.734 |
TMT-B − TMT-A | 232 | −0.16 ± 0.9 | −0.21 ± 1.3 | 0.10 | 1778 | 0.370 |
Stroop naming | 232 | −0.32 ± 1.2 | −0.62 ± 1.9 | −0.10 | −3843 | 0.033 |
Stroop reading | 231 | −0.44 ± 1.5 | −0.88 ± 2.0 | −0.33 | −8900 | <0.001 |
Stroop I | 228 | −0.25 ± 1.4 | −0.27 ± 1.6 | 0.11 | 3203 | 0.071 |
Stroop I-D | 227 | −0.21 ± 1.4 | −0.10 ± 1.4 | 0.15 | 4772 | 0.007 |
PPTTa | 219 | −0.47 ± 1.5 | −0.27 ± 1.5 | 0.00 | 2001 | 0.066 |
Forward span | 208 | −0.06 ± 1.1 | 0.05 ± 1.1 | 0.00 | 984 | 0.221 |
Backward span | 208 | 0.09 ± 1.1 | 0.11 ± 1.1 | 0.00 | 563 | 0.542 |
Free recall (RL-RI) | 165 | −0.51 ± 0.9 | −0.70 ± 1.4 | 0.01 | −107 | 0.929 |
Total recall (RL-RI) | 165 | −0.37 ± 0.5 | −0.72 ± 0.8 | −0.11 | −4179 | <0.001 |
Bell test (omissions)b | 155 | −0.05 ± 1.2 | 0.03 ± 1.1 | 0.00 | 577 | 0.405 |
Rey/Taylor (immediate) | 75 | 0.18 ± 1.1 | −0.10 ± 1.2 | −0.23 | −580 | 0.127 |
Rey/Taylor (delayed) | 73 | 0.22 ± 1.1 | −0.09 ± 1.2 | −0.25 | −455 | 0.213 |
Task . | n . | z-Score (pre) . | z-Score (post) . | Median of difference . | W . | P-value . |
---|---|---|---|---|---|---|
Picture naminga | 223 | 0.11 ± 1.2 | −0.71 ± 3.3 | 0.00 | −4481 | <0.001 |
Semantic fluency | 344 | −0.35 ± 1.1 | −0.61 ± 1.2 | −0.24 | −17197 | <0.001 |
Phonological fluency | 343 | −0.33 ± 1.6 | −0.42 ± 1.3 | −0.15 | −5030 | 0.124 |
TMT-A | 232 | 0.43 ± 0.8 | 0.34 ± 0.9 | 0.00 | −1676 | 0.349 |
TMT-B | 233 | 0.14 ± 0.9 | 0.06 ± 1.4 | 0.05 | 678 | 0.734 |
TMT-B − TMT-A | 232 | −0.16 ± 0.9 | −0.21 ± 1.3 | 0.10 | 1778 | 0.370 |
Stroop naming | 232 | −0.32 ± 1.2 | −0.62 ± 1.9 | −0.10 | −3843 | 0.033 |
Stroop reading | 231 | −0.44 ± 1.5 | −0.88 ± 2.0 | −0.33 | −8900 | <0.001 |
Stroop I | 228 | −0.25 ± 1.4 | −0.27 ± 1.6 | 0.11 | 3203 | 0.071 |
Stroop I-D | 227 | −0.21 ± 1.4 | −0.10 ± 1.4 | 0.15 | 4772 | 0.007 |
PPTTa | 219 | −0.47 ± 1.5 | −0.27 ± 1.5 | 0.00 | 2001 | 0.066 |
Forward span | 208 | −0.06 ± 1.1 | 0.05 ± 1.1 | 0.00 | 984 | 0.221 |
Backward span | 208 | 0.09 ± 1.1 | 0.11 ± 1.1 | 0.00 | 563 | 0.542 |
Free recall (RL-RI) | 165 | −0.51 ± 0.9 | −0.70 ± 1.4 | 0.01 | −107 | 0.929 |
Total recall (RL-RI) | 165 | −0.37 ± 0.5 | −0.72 ± 0.8 | −0.11 | −4179 | <0.001 |
Bell test (omissions)b | 155 | −0.05 ± 1.2 | 0.03 ± 1.1 | 0.00 | 577 | 0.405 |
Rey/Taylor (immediate) | 75 | 0.18 ± 1.1 | −0.10 ± 1.2 | −0.23 | −580 | 0.127 |
Rey/Taylor (delayed) | 73 | 0.22 ± 1.1 | −0.09 ± 1.2 | −0.25 | −455 | 0.213 |
The raw scores for all participants were appropriately aligned to published French normative data (adjusted according to educational level, age and sex) and subsequently converted into z-scores. Wilcoxon matched-pairs signed rank test was used, with a two-tailed P-value, uncorrected. PPTT = Pyramid and Palm Trees Test; RL-RI = rappel libre–rappel indicé; TMT-A/B = Trail Making Test parts A/B.
aOnly patients with left-sided tumours.
bOnly patients with right-sided tumours.
Resection distributions
The spatial distribution of resection cavities, supra-tumour resections and intra-tumour resections is rendered onto the standard Montreal Neurological Institute (MNI)-152 brain template in Fig. 1. The mean preoperative average tumour volume was 56.9 ± 46.4 cm3 (1.5–225). The mean extent of resection was 92.4% ± 8.8% (37–100), leading to a mean residual tumour volume of 5.84 ± 8.9 cm3 (0.0–70.0). Forty (10.0%) patients underwent a supratotal resection (i.e. complete removal of the tumour and an additional supra-tumour margin of resection), 86 (21.5%) had a total resection (no residual tumour volume), 192 (48.0%) had a residual tumoral volume of ≤10 cm3, and 77 (19.3%) had a residual tumoral volume of >10 cm3.
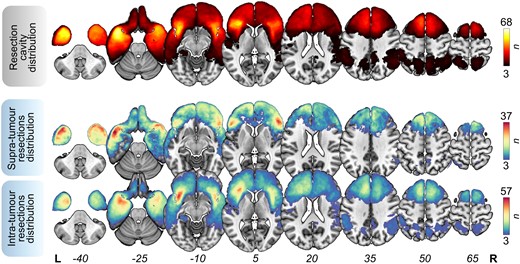
Resection overlap maps. Overlap maps of resection cavities (top), supra-tumour resections (middle) and intra-tumour resections (bottom). These maps were thresholded in such a way that only voxels affected in at least three patients are shown. Bars indicate the number of overlapping lesions. n = 400 patients (left = 234, right = 166). L = left; R = right.
Three hundred and eighty-one (95.3%) subjects presented with ≥1 cm3 of supra-tumour resection, and 311 (77.8%) subjects presented with ≥5 cm3 of supra-tumour resection. supra-tumour resection probability maps are illustrated in Supplementary Fig. 6.
Lesion–symptom mapping
Results of SVR-LSM analyses (topography focused)
All details regarding grid search-driven optimal selection of model hyperparameters (γ and C) and the outcomes of subsequent SVR-LSM analyses, including the number of suprathresholded voxels, are given in Supplementary Figs 7 and 8 and Supplementary Table 4. The addition of supra-tumour masks to intra-tumour masks consistently resulted in higher predictive accuracy values, except in the case of trail making test part A (TMT-A) (Supplementary Fig. 9). First, significant relationships between tumour infiltration masks and preoperative cognitive performances were observed only for verbal episodic memory, including free recall and total recall; these results are detailed fully in Supplementary Fig. 10. Second, brain-function relationships between cavity maps and longitudinal cognitive variations were identified across a total of eight tasks, irrespective of the correction method used (i.e. lenient versus conservative). Picture naming performance was associated with mid-to-posterior part of the left middle temporal (MTG), inferior temporal (ITG) and fusiform (Fus) gyri, extending to the parahippocampal gyrus (ParaHG) (Fig. 2A, ROIa = 13142 voxels; ROIb = 23929 voxels; raccuracy = 0.39; rreproducibility = 0.94); semantic fluency performance with the left posterior precuneus and superior frontal sulcus (Fig. 2B, ROIa = 849; ROIb = 9602; raccuracy = 0.24; rreproducibility = 0.86); phonological fluency performance with the left dorsomesial prefrontal cortex and the left middle frontal gyrus (MFG) (Fig. 2C, ROIa = 5833; ROIb = 17791; raccuracy = 0.24; rreproducibility = 0.90); Stroop naming performance with the left MFG and superior frontal sulcus (Fig. 2D, ROIa = 1074; ROIb = 5815; raccuracy = 0.28; rreproducibility = 0.84); free recall and total recall performances (verbal episodic memory) with the left hippocampus and the left ParaHG (Fig. 2E, ROIa = 1887; ROIb = 5469; raccuracy = 0.35; rreproducibility = 0.84; Fig. 2F, ROIa = 4733; ROIb = 8806; raccuracy = 0.43; rreproducibility = 0.87); Bell test performance with the right dorsomedial prefrontal cortex extending to the superior frontal gyrus (SFG) (Fig. 2G, ROIa = 2349; ROIb = 6410; raccuracy = 0.26; rreproducibility = 0.91); and TMT-A performance with the right superior parietal lobule (SPL) (Fig. 2H, ROIa = 363; ROIb = 165; raccuracy = 0.20; rreproducibility = 0.81).
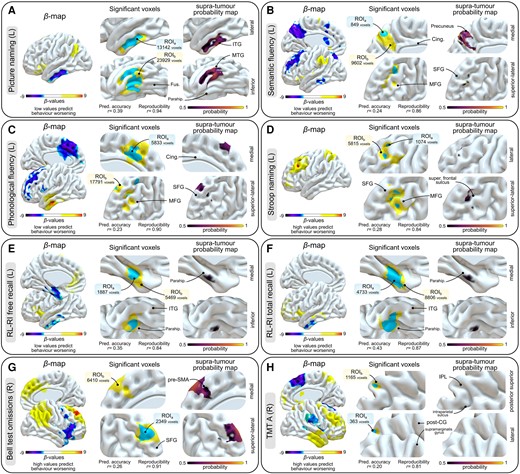
Support vector regression-based lesion–symptom mapping results. (A) Picture naming task (left-sided tumours, n = 223). (B) Semantic fluency task (left-sided tumours, n = 223). (C) Phonological fluency task (left-sided tumours, n = 220). (D) Stroop naming task (left-sided tumours, n = 114). (E) RL-RI free recall task (left-sided tumours, n = 93). (F) RL-RI total recall task (left-sided tumours, n = 93). (G) Bell test (right-sided tumours, n = 155). (H) TMT-A task (right-sided tumours, n = 122). In each panel, raw β-maps are on the left, significant voxels are in the middle (ROIa are displayed in blue, ROIb in yellow, with the used hyperparameters displayed underneath), and supra-tumour probability maps within suprathresholded voxels are on the right. Statistical P-maps are derived from a 10 000 permutation-based procedure. ROIa were obtained after continuous permutation-based familywise error correction set at P < 0.05 (V = 10), i.e. the conservative approach. ROIb were obtained using P < 0.001 uncorrected voxelwise and P < 0.05 clusterwise, i.e. the lenient approach. Importantly, no voxels with positive β-values were found to survive statistical thresholding when negative β-values predicted behaviour worsening (A–C, E and F) and, conversely, no voxels with negative β-values were found to survive statistical thresholding when positive β-values predicted behaviour worsening (D, G and H). Cing = cingulate; IPL = inferior parietal lobule; ITG = inferior temporal gyrus; L = left-sided tumours; MFG = middle frontal gyrus; MTG = middle temporal gyrus; parahip = parahippocampal gyrus; post-CG = post-central gyrus; R = right-sided tumours; RL-RI = rappel libre–rappel indicé; ROI = region of interest; SFG = superior frontal gyrus; TMT = Trail Making Test.
To gauge the weight of supra-tumour resections in the observed associations, we performed intersections between the supra-tumour probability maps on the one hand and ROIa and ROIb on the other hand. The outcomes of these analyses are presented on the left side of each panel in Fig. 2. The probability of observing supra-tumour resection in significant SVR-LSM territories was high in the left posterior ITG and MTG (Fig. 2A) for picture naming, in the left posterior precuneus (Fig. 2B) for semantic fluency and in the right SFG for visuospatial exploration (Fig. 2G).
Relationships between longitudinal behavioural measures and the quantity of resected voxels
To scrutinize the hypothesis suggesting a more pronounced contribution of supra-tumour resections to behavioural post-surgical decline compared with intra-tumour resections, we conducted a series of non-parametric correlations. These correlations encompassed cognitive measurements, the total number of resected voxels (brain-wide) and the number of intra/supra-tumour resected voxels within ROIa and ROIb (Fig. 3). Irrespective of significant areas revealed by SVR-LSM analyses (brain-wide analyses), no correlations emerged, with the notable exception of semantic fluency scores, which were modestly associated with the number of supra-tumour resected voxels in the left hemisphere [r(223) = 0.19, PFDR = 0.029].
![Correlograms showing the relationship between cognitive measurements and the quantity of resected voxels. *Significant non-parametric correlations after false discovery rate correction for multiple comparisons (q = 0.05). Colours reflect the strength of Spearman’s r. Note that values of Spearman’s r were thresholded to zero in the event of a negative r-value. No negative correlation result reached statistical significance. The number of patients included in each analysis is indicated. Across significant correlations, direct comparison of correlation coefficients associated with intra-tumour resection versus supra-tumour resection indicated larger contributions of supra-tumour resected voxels to cognitive declines in free recall (ROIb), Bell test (ROIa) and TMT-A (ROIa and ROIb). Significant differences between intra-tumour versus supra-tumour resection correlation coefficients are circled in red [a false discovery rate correction for multiple comparisons (q = 0.05) was applied]. RL-RI = rappel libre–rappel indicé; ROI = region of interest; TMT = Trail Making Test.](https://oup.silverchair-cdn.com/oup/backfile/Content_public/Journal/brain/147/8/10.1093_brain_awae130/1/m_awae130f3.jpeg?Expires=1748847450&Signature=uC~rfPn1EknOn2bm-roVfzdtGOZfQtjBwm25Ir-LNIzkudrcLG5wcM5VkWTnBgPZmZYu5kK9-Ylv8N9EAAfhrGtcgnAvk1Vgwsm5YST9sX05rVyKII6Cx7YryQ7KLP384etbDJs5-JzW9VBa2xHiG7ZHw-x2zbcWUkIfJ2V4UMuGPhpd00G48ClJIAfclZYoRsjvk4L~FZa2AbgPgoEeMSpo~RTnU6mjfVb4VwX4ZgtUlyTQNVWwh40bPIsad73YC12plTxYhJ5cTltV0WDqm3dw61DdP1vyGUFMKm3tHrbrmEEYdCB9csEw~x8dgBqVxhwiAfWs7uZHh3PP7LKiJw__&Key-Pair-Id=APKAIE5G5CRDK6RD3PGA)
Correlograms showing the relationship between cognitive measurements and the quantity of resected voxels. *Significant non-parametric correlations after false discovery rate correction for multiple comparisons (q = 0.05). Colours reflect the strength of Spearman’s r. Note that values of Spearman’s r were thresholded to zero in the event of a negative r-value. No negative correlation result reached statistical significance. The number of patients included in each analysis is indicated. Across significant correlations, direct comparison of correlation coefficients associated with intra-tumour resection versus supra-tumour resection indicated larger contributions of supra-tumour resected voxels to cognitive declines in free recall (ROIb), Bell test (ROIa) and TMT-A (ROIa and ROIb). Significant differences between intra-tumour versus supra-tumour resection correlation coefficients are circled in red [a false discovery rate correction for multiple comparisons (q = 0.05) was applied]. RL-RI = rappel libre–rappel indicé; ROI = region of interest; TMT = Trail Making Test.
Regarding the correlations within ROIa, comparable associations in terms of strength were identified between picture naming and the quantity of both intra-tumour [r(82) = 0.34, PFDR = 0.017] and supra-tumour resected voxels [r(82) = 0.33, PFDR = 0.015]. Direct comparisons of correlation coefficients associated with intra-tumour resection versus supra-tumour resection are detailed in Supplementary Table 5. A distinct pattern of results emerged for Bell test [r(41) = 0.50, PFDR = 0.015, right hemisphere] and TMT-A [r(42) = 0.53, PFDR = 0.008, right hemisphere], displaying significant associations with the quantity of supra-tumour resected voxels [95% confidence interval (CI): 0.131 to 1.071, PFDR = 0.045, and 95% CI: 0.410 to 1.298, PFDR = 0.004, respectively]. No correlations were discerned for the remaining cognitive tasks when considering the amount of both intra- and supra-tumour resected voxels, although it is noticeable that supra-tumour resected voxels within ROIa were correlated with deficits in total recall [r(30) = 0.54, PFDR = 0.017, left hemisphere].
As expected, a similar pattern emerged when examining ROIb. Notably, correlations persisted between picture naming and the quantity of both intra-tumour [r(89) = 0.33, PFDR = 0.015, left hemisphere] and supra-tumour resected voxels [r(89) = 0.33, PFDR = 0.015, left hemisphere]. Likewise, a distinct pattern remained for the TMT-A [r(63) = 0.57, PFDR = 0.005, right hemisphere], whereby the contributions of supra-tumour resected voxels to cognitive decline were higher (95% CI: 0.397 to 1.034, PFDR = 0.0009]. This dissociation was observed for free recall [r(33) = 0.47, PFDR = 0.029, left hemisphere] in favour of supra-tumour resected voxels (95% CI: 0.043 to 0.675, PFDR = 0.045).
Results of SVR-DSM analyses (disconnection focused)
All specifics pertaining to the grid search-driven optimal selection of model hyperparameters, in addition to the outcomes of subsequent SVR-DSM analyses, including the count of suprathresholded voxels, are outlined comprehensively in Supplementary Fig. 11 and Supplementary Table 6. The results of both SVR-DSM and tract-by-tract correlation analyses are presented visually in Fig. 4. Again, we observed that the addition of supra-tumour disconnections to intra-tumour disconnections consistently resulted in higher predictive accuracy values (Supplementary Fig. 12). In summary, notable outcomes were observed for the same tasks as those identified in topography-focused SVR-LSM analyses, except for TMT-A (right hemisphere) and Stroop inhibition (left hemisphere).
![Support vector regression-based disconnection–symptom mapping (SVR-DSM) and tract-by-tract correlation results. (A) Picture naming task (left-sided tumours, n = 223). (B) Semantic fluency task (left-sided tumours, n = 223). (C) Phonological fluency task (left-sided tumours, n = 220). (D) RL-RI free recall task (left-sided tumours, n = 93). (E) RL-RI total recall task (left-sided tumours, n = 93). (F) Stroop naming task (left-sided tumours, n = 114). (G) Stroop inhibition task (left-sided tumours, n = 112). (H) Bell test (right-sided tumours, n = 155). In each panel, SVR-DSM suprathresholded voxels are placed on the left side (DISCOa is illustrated in red and DISCOb in yellow), and correlation analyses between longitudinal behaviour measures and the number of disconnected fibres are presented on the right side. Statistical P-maps are derived from a 10 000 permutation-based procedure. DISCOa were obtained after continuous permutation-based familywise error correction set at P < 0.05 (V = 10), i.e. the conservative approach. DISCOb were obtained using P < 0.001 uncorrected voxelwise and P < 0.05 clusterwise, i.e. the lenient approach. *Significant non-parametric correlations after false discovery rate correction for multiple comparisons (q = 0.05). Colours reflect the strength of Spearman’s r (correlations with behaviour worsening are presented in yellow–red, and anti-correlations with behaviour worsening are presented in yellow–green). Across significant correlations, direct comparisons of correlation coefficients associated with intra-tumour disconnection versus supra-tumour disconnection indicate higher contributions of supra-tumour disconnections to cognitive declines in picture naming (UF, uncinate fasciculus) and phonological fluency (CS, corticostriatal tracts; and CCant, anterior corpus callosum). Conversely, higher contributions of intra-tumour disconnections were found in the Stroop naming task (SLF, superior longitudinal fasciculus). Significant differences between intra-tumour versus supra-tumour disconnection correlation coefficients are circled in red [a false discovery rate correction for multiple comparisons (q = 0.05) was applied]. AC = anterior commissure; AF = arcuate fasciculus; CCmidant = mid-anterior corpus callosum; CCpost = posterior corpus callosum; Cing = cingulum; EMC = extreme capsule; FAT = frontal aslant tract; FPT = frontopontine tracts; IFOF = inferior fronto-occipital fasciculus; ILF = inferior longitudinal fasciculus; MdLF = middle longitudinal fasciculus.](https://oup.silverchair-cdn.com/oup/backfile/Content_public/Journal/brain/147/8/10.1093_brain_awae130/1/m_awae130f4.jpeg?Expires=1748847450&Signature=sH7GJEC1Zql91Aw7r5p0qHSf7AQcAPXFT1rvNfcGkjhdnG58Ifz-4sts3cVhlEpb7TcyX0BtIGqj6y0V2Jxr7KtWkwJ6iV5dvRp36tbUvg36lZJwI3o0x6WmTLW~mpAx8~woEnO-BuV7ZJEpbCj2TqrP34sQ9eCRxMJicAYf-KZmjTQRfT9GVw0EpbpUkD10nw-oucwlxF0HvUDF5gH9w4v7oCGPi1W2h9VcczJh0zfvLM9EsBpQzNOP~nd74OwLj6D~-TG64qE-EsCHf~RHxI3mw4Asb3z5TMbaK~w-nh2kBlvG3koy3Qwzwjb~8nBCNDUkzGG7kuEkUkNopELEGQ__&Key-Pair-Id=APKAIE5G5CRDK6RD3PGA)
Support vector regression-based disconnection–symptom mapping (SVR-DSM) and tract-by-tract correlation results. (A) Picture naming task (left-sided tumours, n = 223). (B) Semantic fluency task (left-sided tumours, n = 223). (C) Phonological fluency task (left-sided tumours, n = 220). (D) RL-RI free recall task (left-sided tumours, n = 93). (E) RL-RI total recall task (left-sided tumours, n = 93). (F) Stroop naming task (left-sided tumours, n = 114). (G) Stroop inhibition task (left-sided tumours, n = 112). (H) Bell test (right-sided tumours, n = 155). In each panel, SVR-DSM suprathresholded voxels are placed on the left side (DISCOa is illustrated in red and DISCOb in yellow), and correlation analyses between longitudinal behaviour measures and the number of disconnected fibres are presented on the right side. Statistical P-maps are derived from a 10 000 permutation-based procedure. DISCOa were obtained after continuous permutation-based familywise error correction set at P < 0.05 (V = 10), i.e. the conservative approach. DISCOb were obtained using P < 0.001 uncorrected voxelwise and P < 0.05 clusterwise, i.e. the lenient approach. *Significant non-parametric correlations after false discovery rate correction for multiple comparisons (q = 0.05). Colours reflect the strength of Spearman’s r (correlations with behaviour worsening are presented in yellow–red, and anti-correlations with behaviour worsening are presented in yellow–green). Across significant correlations, direct comparisons of correlation coefficients associated with intra-tumour disconnection versus supra-tumour disconnection indicate higher contributions of supra-tumour disconnections to cognitive declines in picture naming (UF, uncinate fasciculus) and phonological fluency (CS, corticostriatal tracts; and CCant, anterior corpus callosum). Conversely, higher contributions of intra-tumour disconnections were found in the Stroop naming task (SLF, superior longitudinal fasciculus). Significant differences between intra-tumour versus supra-tumour disconnection correlation coefficients are circled in red [a false discovery rate correction for multiple comparisons (q = 0.05) was applied]. AC = anterior commissure; AF = arcuate fasciculus; CCmidant = mid-anterior corpus callosum; CCpost = posterior corpus callosum; Cing = cingulum; EMC = extreme capsule; FAT = frontal aslant tract; FPT = frontopontine tracts; IFOF = inferior fronto-occipital fasciculus; ILF = inferior longitudinal fasciculus; MdLF = middle longitudinal fasciculus.
Both types of analyses consistently provided converging evidence, highlighting that a lack of recovery in picture naming was primarily associated with damage to the left ILF and AF, CCpost and AC (Fig. 4A). Likewise, a lack of recovery in semantic fluency was linked to damage in the left posterior cingulum (Fig. 4B), and a deficit in phonological fluency was associated with damage to the left FAT, FPT, CS, CCmidant and CCant (Fig. 4C). Furthermore, a lack of recovery in verbal episodic memory (free recall) was correlated with damage to the temporal portion of the left cingulum (Fig. 4D). Such a pattern was also observed for verbal episodic memory (total recall; Fig. 4E). Stroop naming deficits were associated mainly with damage to the left SLF and left CS (Fig. 4F), whereas Stroop inhibition deficits were correlated with damage to the left FAT and CCmidant (Fig. 4G). A lack of recovery in spatial exploration (Bell test) was tied to damage in the right medial tracts, particularly the cingulum and the SLF (Fig. 4H).
Correlations stemming from disconnections associated with either intra-tumour or supra-tumour resection generally produced parallel findings (Supplementary Table 7). However, variations were observed in specific instances: in the case of picture naming, supra-tumour disconnections of the left UF [r(223) = 0.21, PFDR = 0.024; Fig. 4A] were more prone to induce declines in performance than intra-tumour disconnections (95% CI: 0.028 to 0.178, PFDR = 0.036); for phonological fluency, the left CS and the CCant [r(220) = 0.34, PFDR = 0.002 and r(220) = 0.30, PFDR = 0.002, respectively; Fig. 4C] exhibited a greater association with disconnections tied to supra-tumour rather than intra-tumour resections (95% CI: 0.025 to 0.158, PFDR = 0.036 and 95% CI: 0.029–0.103, PFDR = 0.008, respectively]. Lastly, in Stroop naming, the left SLF was the only tract where intra-tumour disconnections elicited more decline in performance than supra-tumour disconnections (95% CI: −0.346 to −0.057, PFDR = 0.036; Fig. 4F). It is worth noting that SVR-DSM and tract-by-tract correlation analyses focused on the TMT-A (left hemisphere) did not yield significant disconnection-focused results (Supplementary Fig. 13).
Discussion
In this study, our focus was on discerning the surgically disrupted cerebral circuits that restricted the potential for full cognitive recovery, in a cohort of patients diagnosed with a brain condition established to induce major neuroplastic adaptations.41 To attain this goal, we conducted topography-focused and disconnection-focused multivariate lesion–symptom analyses using support vector regressions. Additionally, we gauged the respective weight of intra- and supra-tumour resections in anatomofunctional correlations, introducing a new dimension in our understanding of cognitive outcomes in resective neurosurgery. The strengths of the study were manifold: (i) a substantial sample size (n = 400), ensuring statistical robustness; (ii) the homogeneity of the patient sample, composed exclusively of patients with low-grade gliomas, thereby mitigating limitations related to tumour characteristics (e.g. mass effect and tissue deformations in higher-grade gliomas) and to additional neurooncological treatments, such as radiotherapy (a risk for cognitive decline42); and (iii) the longitudinal design, allowing for a focused examination of the specific effects of resections on behavioural measurements. In summary, cognitive measurements reverted to their baseline level 3 months after surgery in 13 of 18 subtests, suggesting that mapping-guided surgical resections have only a limited negative impact on overall cognitive functioning. Nevertheless, our analyses unveiled correlations between cortical–subcortical structures and incompletely compensated functions, implying constraints on the reorganization potential of specific neural circuits. Finally, although the analyses dissociating the effects of intra-tumour versus supra-tumour resections produced similar findings overall, distinct patterns emerged, suggesting that supra-tumour resections were occasionally less well tolerated from a cognitive viewpoint.
Lesion mapping techniques remain indispensable for delineating the causal role of cerebral structures within a specific functional network.43,44 In investigations focused on brain conditions associated with efficient compensatory mechanisms, such as DLGG, anatomofunctional correlations are of paramount importance. They provide an understanding of the ability of brain circuits to compensate for an acute and sharp cerebral injury (surgical resection) within areas preliminarily remodelled to varying degrees owing to prior chronic tumour infiltration. Stated another way, they allow for the identification of critical structures that maintain cognitive eloquence despite undergoing neuroplastic changes. Therefore, LSM techniques in DLGG patients are well suited to probing the neuroplasticity potential of structures involved in a wide array of cognitive functions, but admittedly, they fall short of identifying all structures involved in normal physiological circumstances. This limitation has consequences for accurate interpretation of the findings reported here, which might diverge from the prevailing neuropsychological literature primarily rooted in sudden neurological diseases, particularly stroke injuries.
First and foremost, it is important to note that optimization procedures for preoperative SVR-LSM analyses consistently faced challenges in identifying hyperparameter pairs driving satisfactory prediction accuracy and reproducibility. This implies that the preoperative pathological variance was not enough for appropriate modelling in the large majority of cases, which is consistent with the low rate of preoperative deficits. This overarching pattern of results aligns with the viewpoint that the slow growth kinetics of DLGGs allow for the instantiation of efficient neural compensation, thereby drastically limiting the occurrence of cognitive deficits. In the same vein, the postoperative performances associated with only five cognitive tasks did not return to their baseline levels, indicating the effectiveness of both preoperative neuroplasticity compensations and intraoperative cognitive mapping. Collectively, these results demonstrate that mapping-aided surgical resection has a limited negative impact on cognitive functions at the 3-month post-surgery juncture, which is a time point at which recovery is still ongoing.
SVR-LSM performed on both resection cavities and white matter disconnectomes highlighted significant structure–function relationships. In the domain of language, limited recovery in lexical retrieval (as assessed with a picture naming task) was mainly associated with damage to both the left mid-to-posterior ITG and the inferior temporal sulcus (slightly extending to the mid-to-posterior MTG), the fusiform gyrus and, to a lesser extent, the parahippocampal gyrus. Unsurprisingly, associations were also identified with the white matter fibres running deep within and projecting to these cortical areas, mostly the left ILF and the posterior CC. This is consistent with previous findings from DLGG patients, which underscored the left posterior ITG/FG as a high-centrality cortical hub in the lexical retrieval network45 and damage of the ILF as particularly detrimental in the lexical access of both common names and proper names.46 This pattern reinforces the evidence that the ventral temporal networks play a crucial role in processing visual input-based language, a perspective that has previously been underestimated, given that stroke injuries only occasionally disrupt the white matter connections running basally. It should be acknowledged that the emergence of anatomical–clinical correlations might be viewed as somewhat surprising, given the systematic language mapping that was conducted. This observation has, however, to be considered in the context of our recently developed understanding of both the role of the left ILF in language and its mechanisms of tumour-induced rerouting. Confirmed evidence supports the occurrence of functional compensation within this occipitotemporal tract, but only in specific pathophysiological circumstances. This phenomenon is particularly notable when the anterior temporal structures, exclusive recipients of ILF projections anteriorly, relinquish their functions owing to tumour invasion.47,48 This significant finding has led to a recent refinement in the surgical mapping of the left ventral connections that were previously overlooked.
Semantic fluency and phonological fluency performances were found to be associated with both shared and distinct cortical–subcortical structures. In the case of semantic fluency, significant structures included the posterior part of the left precuneus along with the cingulum and, to a lesser extent, the left superior frontal sulcus. Conversely, for phonological fluency, only the left superior frontal sulcus, extending to both the superior and middle frontal gyri, was identified, along with white matter tracts projecting into these areas, such as the frontal aslant and the corticostriatal tract. Interestingly, the left precuneus, traditionally overlooked in lesion mapping studies related to semantics owing to limited coverage of stroke injuries in this region, has nonetheless emerged as a central semantic hub in quantitative meta-analyses of functional MRI.49 In this context, our findings offer behavioural evidence that this medial parietal area engages in aspects of semantic processing, albeit without precisely pinpointing the specific process that might be disrupted, given the multicomponent nature of the task.50 Considering the involvement of both the precuneus51 and the cingulum52 in cognitive control, it is conceivable that they contribute to the search strategy within the semantic store.
Additional and classic associations were identified with verbal episodic memory performances, specifically involving the left hippocampal and the parahippocampal gyrus. Complaints related to episodic memory constitute one of the most prevalent subjective concerns reported by patients undergoing brain surgery.53 In addition, episodic memory was reported as the most frequent cognitive dysfunction in the preoperative setting in DLGG patients,54 which is consistent with the fact that we identified significant anatomofunctional relationships before surgery. Epileptic discharges, commonly targeting the temporomesial structures and their underlying white matter connectivity, are acknowledged for their adverse effects on episodic memory. This impact is particularly noteworthy in patients with preoperatively intractable epilepsy, for whom quality of life is affected. In this context, the goal of neurosurgery extends beyond its oncological benefits to maximize opportunities for postoperative epilepsy control in patients. This can involve guiding the surgical procedure beyond fully functionally compensated structures to target and remove the areas generating epileptic discharges.55,56 As a consequence, deficits in verbal episodic memory can emerge following left hippocampectomy, similar to those observed in standard epilepsy surgery. The correlation obtained between the number of supra-tumour resected voxels and memory performances is a good illustration of this. Unfortunately, accurate intraoperative mapping of this process in awake tumour surgery is challenging because of constraints related to direct electrostimulation procedures. From a clinical standpoint, this entails determining, with patients, the best balance between controlling epilepsy and the likelihood of post-surgical deficits, while considering highly personalized strategies for cognitive rehabilitation.
Damage to the right dorsomedial prefrontal cortex and the underlying cingulum, in addition to the right SLF, was associated with a lack of full recovery in non-lateralized visual exploration. This result aligns, in part, with the findings of a recent study, which demonstrated early left visuomotor exploratory neglect following surgical removal of the same structures, with, however, a full recovery being observed 3 months later.30 It is also consistent with the established view that the right frontoparietal connectivity is of vital importance for visuospatial cognition.57,58 Importantly, our results showed that supra-tumour resections were particularly prone to causing this decline in performance. Collectively, this pattern of findings suggests that awake mapping-assisted neurosurgery does not induce long-term spatial neglect, as previously demonstrated.30 It might, nevertheless, lead to difficulties in optimally orienting attention in the visual scene, irrespective of the visual field, especially when the procedure extends beyond the tumour boundaries. Currently, spatial attention is commonly assessed intraoperatively with a line bisection task. However, this task captures only the perceptive aspects of visuospatial attention and not its voluntary component.59,60 Our results thus suggest that visual exploration tasks tapping into the voluntary deployment of attention might be valuable, particularly when the resection involves the mesial portion of the frontal lobe. Importantly, from a neurosurgical perspective, our findings challenge the classical conception that right prefrontal areas can be resected safely, without functional consequences.61
As mentioned earlier, the fine-grained analyses that aimed to differentiate the behavioural variations associated with intra-tumour versus supra-tumour resections generally yielded comparable results, with some noteworthy exceptions, some of which have been discussed earlier. It is worth noting that despite the extensive coverage of supra-tumour resections within the left and right inferior frontal gyrus (areas that are frequently resected to access insular tumours because of the added safety value of this trans-opercular approach over trans-sylvian approaches22), no anatomofunctional correlations were observed in the cognitive tasks under scrutiny. Conversely, supra-tumour resections within both the left ITG and MTG, in addition to the left UF, were probabilistically linked to a diminished likelihood of full recovery in lexical retrieval. Likewise, supra-tumour resections in the left precuneus were associated with a higher likelihood of incomplete recovery in semantic fluency ability. These findings suggest that, for specific functions, increased attention might be warranted when intervening in specific areas surrounding the glioma. In practical terms, this implies that new efforts should be made to develop sensitive neuropsychological tasks capable of optimally guiding the neurosurgical procedure in at-risk structures, without compromising the delicate balance between maximizing the oncological benefits of supratotal resection and preserving functions and quality of life. This refinement in surgical approaches should be pursued concurrently with advancements in understanding the mechanistic aspects of tumour-induced neuroplasticity. For instance, gaining a precise understanding of how neuroplasticity affects peri-tumour areas would be particularly valuable in elucidating why only specific task performances are impaired by supra-tumour resections. Currently, there is evidence that glioma-induced neuroplasticity might induce remote and distributed cortical remodellings, which occur beyond peri-tumoural areas, including the contralateral hemisphere.4,62,63
Limitations
This study has a number of limitations. First, we conducted our cognitive assessments at two time points: before and 3 months after surgery. Although it is generally accepted that a significant portion of recovery occurs during the first 3 months of convalescence, the process of functional compensation can continue for several months thereafter. This implies that the statistical associations identified in this study might diminish in strength over time. Second, patients systematically received antiepileptic drugs for 3 months after surgery, which might potentially have influenced the variations in cognition observed between the two assessments. Third, certain tasks were less frequently administered to patients, such as language tasks for those with right-sided tumours, potentially introducing a selection bias. Fourth, it should be acknowledged that certain brain–behaviour relationships might not have been captured by our current disconnection analyses, because the study did not incorporate a couple of white matter tracts that are now available in the latest version of the HCP white matter atlas. This includes the parietal aslant tract and the subdivisions of the SLF. Fifth, we assessed the prediction accuracy of the generated lesion–symptom models through a 5-fold cross-validation procedure. This assessment was conducted independently, focusing either on lesion topographies or on structural disconnections. A yet-unexplored dimension in this study revolves around the potential improvement in predictive accuracy for cognitive outcomes through the integration of both types of neuroanatomical predictors. Although a handful of recent studies have successfully implemented methodological approaches, such as ridge regressions with dimensionality reduction of anatomical data,64 to assess precisely the added impact of incorporating disconnection data alongside topographic information in predicting cognitive variance, determining such effects in the context of non-linear radial basis function-derived SVR models would require an extensive validation study that surpasses the scope of the present research. Sixth, our conclusions are drawn from a single-cohort study, which constitutes a limitation owing to the lack of an external replication cohort. Finally, some of the statistical analyses were exploratory in nature, especially the correlation approaches between cognitive scores on the one hand and intra- or supra-tumour resections on the other hand. The addition of a substantial number of patients to the database might enable, in the future, the use of more complex statistical designs, providing a more precise estimation of the behavioural variance associated with each of these resection categories.
Conclusion
In summary, our results yield valuable insights into both the topological organization of critical neural systems and the prediction of cognitive recovery after resection surgery in patients with diffuse low-grade gliomas. These findings carry implications for various facets of neurosurgical practice, potentially reshaping our approach to DLGG treatment (e.g. refining the balance between the oncological benefits of supra-tumour resections versus the preservation of cognitive functions at the individual level). The implications of the study extend to the development of tailored rehabilitation strategies, aiming to optimize the trajectory of postoperative cognitive recovery. Moreover, the associations and patterns uncovered in our study might serve as pivotal signposts for future research endeavours, providing concrete targets for neuromodulation protocols. This opens up exciting possibilities for exploring interventions that could significantly enhance cognitive outcomes after resection surgery, paving the way for innovative approaches to enhance patient well-being and quality of life.
Data availability
Data are available upon request.
Funding
No funding was received towards this work.
Competing interests
The authors report no competing interests.
Supplementary material
Supplementary material is available at Brain online.