-
PDF
- Split View
-
Views
-
Cite
Cite
Nico J. Diederich, Glenn Stebbins, Christine Schiltz, Christopher G. Goetz, Are patients with Parkinson’s disease blind to blindsight?, Brain, Volume 137, Issue 6, June 2014, Pages 1838–1849, https://doi.org/10.1093/brain/awu094
- Share Icon Share
Abstract
In Parkinson’s disease, visual dysfunction is prominent. Visual hallucinations can be a major hallmark of late stage disease, but numerous visual deficits also occur in early stage Parkinson’s disease. Specific retinopathy, deficits in the primary visual pathway and the secondary ventral and dorsal pathways, as well as dysfunction of the attention pathways have all been posited as causes of hallucinations in Parkinson’s disease. We present data from patients with Parkinson’s disease that contrast with a known neuro-ophthalmological syndrome, termed ‘blindsight’. In this syndrome, there is an absence of conscious object identification, but preserved ‘guess’ of the location of a stimulus, preserved reflexive saccades and motion perception and preserved autonomical and expressive reactions to negative emotional facial expressions. We propose that patients with Parkinson’s disease have the converse of blindsight, being ‘blind to blindsight’. As such they preserve conscious vision, but show erroneous ‘guess’ localization of visual stimuli, poor saccades and motion perception, and poor emotional face perception with blunted autonomic reaction. Although a large data set on these deficits in Parkinson’s disease has been accumulated, consolidation into one specific syndrome has not been proposed. Focusing on neuropathological and physiological data from two phylogenetically old and subconscious pathways, the retino-colliculo-thalamo-amygdala and the retino-geniculo-extrastriate pathways, we propose that aberrant function of these systems, including pathologically inhibited superior colliculus activity, deficient corollary discharges to the frontal eye fields, dysfunctional pulvinar, claustrum and amygdaloid subnuclei of the amygdala, the latter progressively burdened with Lewy bodies, underlie this syndrome. These network impairments are further corroborated by the concept of the ‘silent amygdala’. Functionally being ‘blind to blindsight’ may facilitate the highly distinctive ‘presence’ or ‘passage’ hallucinations of Parkinson’s disease and can help to explain handicaps in driving capacities and dysfunctional ‘theory of mind’. We propose this synthesis to prompt refined neuropathological and neuroimaging studies on the pivotal nuclei in these pathways in order to better understand the networks underpinning this newly conceptualized syndrome in Parkinson’s disease.
Introduction
Numerous visual complaints and deficits have been reported in patients with Parkinson’s disease, ranging from blurred vision to impairments of contrast and colour discrimination, reduced recognition of facial expression, impaired motion detection and all kinds of visual illusions and hallucinations. These deficits have been thought to be due to circumscribed Parkinson’s disease-related lesions, such as specific retinopathy, burdens of Lewy body in the striate or extrastriate visual cortex, and medication-induced effects (Archibald et al., 2009; Bodis-Wollner, 2009; Diederich et al., 2009). It has been suggested that the deficits affect both the ventral and the dorsal visual pathways. However, no further conceptualization has been proposed. The present essay presents clinical and neuropathological arguments in support of selective impairment of the visual capacities of patients with Parkinson’s disease, controlled by neural networks previously studied to explain ‘blindsight’ syndrome. It postulates that patients with Parkinson’s disease are ‘blind to blindsight’. Indeed, contrasting with (relatively) intact neocortical visual pathways, patients with Parkinson’s disease show dysfunctional blindsight visual pathways, including dysfunctional saccades, deficient motion perception and impaired emotional face perception. From this perspective, Parkinson’s disease might actually be a unique opportunity to study isolated ‘pure’ conscious vision mediated by the circuit involving the lateral geniculate nucleus, the primary visual cortex and the extrastriate visual areas. Importantly, blindsight vision is also used by normal viewers, but difficult to study (Hesselmann et al., 2011), because in the conscious sighted persons features of blindsight vision are masked by features of conscious vision, with the predominant pathway connecting the lateral geniculate nucleus to primary visual cortex, and from there to the extrastriate higher visual areas.
The blindsight syndrome
Historical perspective
Weiskrantz coined the term ‘blindsight’ after extensive studies of the retained visual capacities in a patient having had bilateral occipital damage (Weiskrantz, 1986). Based on his findings, blindsight has been considered to be a phenomenon in which people, who are perceptually blind in a certain area of their visual field, nevertheless demonstrate intact responses to visual stimuli in that field, thus suggesting a residual network of functions left undisturbed by the cortical lesion (Weiskrantz, 1974, 1996; Stoerig and Cowey, 1997). A more concise definition by Barbur (2004) states that ‘blindsight describes the ability to make accurate use of visual information in the absence of conscious visual perception’. The components of the blindsight syndrome defined in this purely clinical way are variable, as affected patients have cortical lesions differing in size and location. However, in patients with hemispherectomy, the archetypal syndrome has been highly consistent, thus allowing the development of a precise framework for anatomical and physiological characterization of the blindsight syndrome.
Classical blindsight syndrome
Several visual properties characterize the blindsight syndrome. First, patients who perceive themselves as blind and therefore are unable to consciously see visual stimuli, are still able to accurately guess or localize by hand or eye-movement the location and, rarely, even the identity of stimuli presented in their blind field (Stoerig and Cowey, 1997). Second, reflexive saccades and smooth pursuit occur when a tracking object is presented in the blind visual field, despite the perceived inability to see it. Thus their ‘eyes move with a moving visual scene’ (Stoerig and Cowey, 1997).
New addition: affective blindsight
Blindsight patients appropriately respond, both expressively and by autonomic testing to emotional facial expressions, especially those expressing fear or danger (‘affective blindsight’). This latter blindsight feature, although not described by the original authors, has recently been outlined by Tamietto and de Gelder (2010) and Tamietto et al. (2010), who emphasized that there is overlapping of subcortical pathways for vision and emotion and that rapid, subconscious appreciation of emotional face expression is also used by the visually intact subject (De Gelder et al., 2005; Tamietto et al., 2009; Tamietto and de Gelder, 2010). In this essay, we include affective blindsight as the third element of a blindsight triad.
Blindsight variants
Several authors (Danckert and Rossetti, 2005; Ptito and Leh, 2007) have classified blindsight vision into: (i) action blindsight: the residual capacity for adequate oculomotor behaviour (pointing or grasping of blindfield stimuli); (ii) attention blindsight: the residual capacity of covert spatial orienting and motion detection; and (iii) agnosopia: the persistent ability to correctly guess perceptual characteristics (wavelength, texture, form).
The (correct) above-chance discrimination of certain visual features, in the absence of any conscious awareness, has also been termed ‘blindsight type 1’, whereas in the ‘softer variant’ of ‘blindsight type 2’, the patient has a vague feeling that something has occurred (Weiskrantz, 1996).
Pathophysiology
Achromatic or only S-cone independent vision?
The blindsight triad is usually mediated by achromatic stimuli so that there is no perceptual preference of a single visual wave-length band. However, animal work on lower order mammals and reptilians suggests that selective wave-length bands may be an inherent part of blindsight. Thus, in frogs, which rely on a dichromatic visual system, tectal fibres are specifically driven by red-sensitive receptors (Maximov et al., 1985). Recent research on blindsight in humans supports the retention of this reptilian-like colour encoding program, with the human superior colliculus being only insensitive to S-cone (blue-yellow) colour inputs, but still sensitive to other wave-length colours (Leh et al., 2006b, 2010). Also, in patients with hemi-field blindsight, the pupils continue to react selectively to red stimuli, even when the stimulus is isoluminant and entirely restricted to the blind hemi-field (Barbur et al., 1999).
Two separate pathways
Blindsight functions are processed by two pathways, with neither targeting the primary visual cortex area that plays a critical and pivotal role in visual awareness (Figs 1 and 2). The first pathway, termed the retino-colliculo-thalamo-amygdala (RCTA) pathway, targets the amygdala, running through the superior colliculus and thalamic relay stations. The second pathway, named the retino-geniculo-extrastriate (RGES) pathway, connects the retina through the lateral geniculate nucleus to the extrastriate ipsilateral visual cortex, namely the motion area V5/MT in the medial temporal lobe. It constitutes the unconscious part of the dorsal ‘where’ stream, whereas the conscious counterpart passes through the primary visual cortex before reaching the V5/MT area. For the purpose of this essay, we use the term ‘blindsight pathways’ for both blindsight-mediating circuits. Although initially the exact anatomy of the involved pathways could only be hypothesized based on lesion studies, functional MRI and diffusion tensor imaging (Leh et al. 2006a, 2010; Schmid et al., 2010; Hesselmann et al., 2011) have recently been used to precisely delineate the pathways underpinning the unconscious visual discrimation capacities preserved in blindsight. Diffusion tensor imaging and transcranial magnetic stimulation in patients with blindsight have also shown that after several decades such patients demonstrate some neuroplasticity by ‘reopening’ direct transcallosal pathways between both V5/MT areas, thus bypassing the usual visual pathways. In unaffected controls these latter pathways seem to be ‘vestigial’ at best (Bridge et al., 2008; Ress, 2008; Silvano et al., 2009).
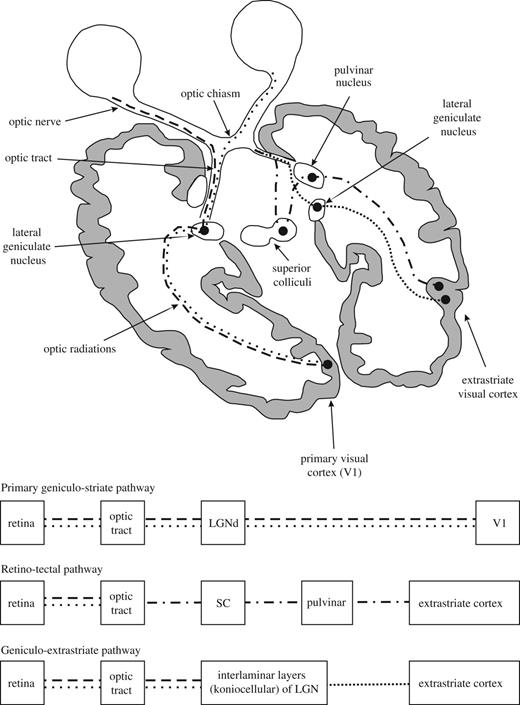
Schematic representation of the visual pathways. Beside the conscious geniculo-striate pathway (dashed lines), there are two subconscious visual pathways that do not pass through V1: the RCTA (dashed/dotted lines) and the RGES (closely spaced dotted lines). Of note, the discussed extension of the RCTA to the amygdala is not represented in the figure. For further explanations see text from Danckert and Rossetti (2005); with permission. LGN = lateral geniculate nucleus; SC = superior colliculus.
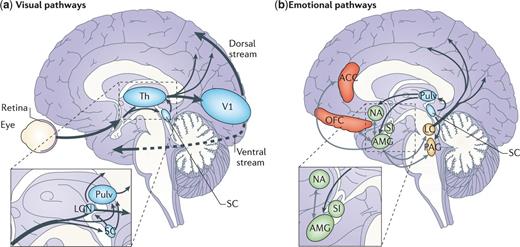
Cortical and subcortical pathways for vision and emotion. (a) The primary visual pathway (shown by thick arrows) originates from the retina and projects to the primary V1 in the occipital lobe via an intermediate station in LGN of Th. From V1, visual information reaches the extrastriate cortex along the ventral (occipitotemporal) and the dorsal (occipitoparietal) stream. However, a minority of fibres originating from the retina take a secondary route (shown by thin arrows) and reach both SC and Pulv. These two subcortical sites are connected and also send direct projections to the extrastriate visual cortex, bypassing V1. Another V1-independent visual pathway consists of the direct projections between the superior colliculus and LGN that, in turn, send efferents to extrastriate cortices in the dorsal stream. (b) The ‘emotion system' includes several cortical and subcortical areas. Among the subcortical structures are AMG and SI (shown in green), which are buried deeply in the temporal lobe and in the basal forebrain, respectively, NA in the basal ganglia (shown in green) and brainstem nuclei (shown in yellow), such as PAG and LC. Among cortical areas (shown in red) are OFC and ACC. The visual and emotional systems are extensively interconnected, especially at the subcortical level, where the superior colliculus is connected to the amygdala via the pulvinar. Direct connections also exist between subcortical and cortical emotion regions (for example, between the amygdala and OFC or ACC), between subcortical structures for emotions and cortical visual areas (for example, between the amygdala and temporal cortex) (not shown) and between brainstem nuclei and the cortex via diffuse projections (shown only from the LC). Grey arrows indicate connections within the emotion system. ACC = anterior cingulate cortex; AMG = amygdala; LC = Locus coeruleus; LGN = lateral geniculate nucleus; NA = nucleus accumbens; OFC = orbitofrontal cortex; PAG = periaqueductal grey; Pulv = pulvinar; SC = superior colliculus; SI = substantia innominata; Th = thalamus; V1 = primary visual cortex.
Nuclei involved
In blindsight pathways, four nuclei are all directly implicated.
Superior colliculus
The superior colliculus is not only responsible for subconscious guidance of eye movements, it also informs several brain regions about the on-going eye movement. It has a layered structure. Whereas the top layers receive primary inputs from the retina and are organized in a topographical manner, the deeper layers contain cells with eye movement properties and induce saccadic eye movements (Schiller et al., 1972; Wurtz and Goldberg, 1972). The superior colliculus also receives numerous feedback stimuli from the basal ganglia and the frontal visual eye fields, among others (McHaffie et al., 2005). Corollary discharges from the superior colliculus to different brain regions are essential to guarantee the stability of the visual field. Thus, in monkeys, pathways running from the superior colliculus (i) through the medial dorsal nucleus of the thalamus to the frontal eye fields; and (ii) through the pulvinar nucleus of the thalamus to parietal and occipital cortex, have been identified. By providing information about the impending saccade to these regions, these corollary discharges contribute to visual stability (Sommer and Wurtz, 2008; Wurtz et al., 2011). Conversely, feedback pathways from the frontal eye fields to the superior colliculus are involved in the execution and correction of the saccades, once the decision has been taken by the superior colliculus ‘about where to look and where not to look’ (Schiller et al., 2001, 2005). Recordings from the superior colliculus in monkeys adequately predict if a Go or No-Go decision will be executed (Hasegawa et al., 2006). Usually, the basal ganglia tonically inhibit the superior colliculus, but disinhibition is required for saccade initiation. The closed-loop interaction between both regions handles visual information selectively, using the following visual parameters: achromatic or S-cone (blue–yellow) independent stimuli, motion stimuli, differential consideration of the upper and lower visual field (Comoli et al., 2003; McHaffie et al., 2005; Coizet et al., 2009; Schulz et al., 2009; Leh et al., 2010). By processing and selecting the biological salience of targets, the superior colliculus induces presaccadic short-latency activations of striatal spiny neurons (Schulz et al., 2009). Furthermore, for the effective function of the superior colliculus, the two-way connections with the frontal eye fields are a prerequisite in that the superior colliculus activates the frontal eye fields in the preparatory phase of visual perception, and the frontal eye fields send an excitatory signal to the superior colliculus when initiating a gaze shift to a target (Dommett et al., 2005; McHaffie et al., 2005). Dysfunctional interplay within these loops underlies saccade abnormalities in Parkinson’s disease (see below). In summary it can be argued that the superior colliculus functions as a pivotal ‘gate keeper’ to and from the basal ganglia and the frontal eye fields.
Ventral intralaminar layers of the lateral geniculate nucleus
The ventral intralaminar layers of the lateral geniculate nucleus are pivotal relay stations of the RGES pathway, running from the retina to the extrastriate V5/MT area, which is responsible for motion detection (Schmid et al., 2010). These ventral layers have to be differentiated from the dorsal layers functioning as a relay station to the primary visual cortex.
Pulvinar
The pulvinar mediates information from the superior colliculus to both the striatum and the amygdala, thus it is also implicated in processing behaviourally salient visual targets (Day-Brown et al., 2010).
Amygdala
The amygdala is the end station of the RCTA but also part of the phylogenetically old fear system. It plays an essential role in emotional arousal (Masaoka et al., 2003).
Evolutionary aspects
The blindsight pathways are highly functional in fish, amphibians, and reptiles and are operational in human newborns (Braddick et al., 1992). Essential organizational characteristics of these phylogenetically old loops are well preserved in the primate brain, with the superior colliculus being considered to be the mammalian homologue of the optic tectum of the reptiles (McHaffie et al., 2005). Of note, blindsighted monkeys hardly show any impairment in routine tasks (Cowey and Stoerig, 1995, 1997). Blindsight mediated by the RCTA handles vitally essential visual information. Through the covert circuitry to the amygdala it allows ‘expedient detection’ and ‘faster processing’ of fear-related cues, which ‘could aid speedy, survival-enhancing responses to danger’ (Vuilleumier et al., 2003). Indeed, the latencies of this essentially magnocellular pathway, highly sensitive to low spatial frequencies, are shorter than those of the retino-geniculate-primary visual cortex pathway mediating conscious vision. Evidently, in healthy humans this alarm-system prerogative of the RCTA is difficult to prove and with current technology, its functions are impossible to disentangle from superimposed, conscious, forebrain-induced visual responses (Goodale and Milner, 1992; Crick and Koch, 1995).
The blind to blindsight syndrome in Parkinson’s disease
For a comparison of blindsight vision and blind to blindsight syndrome, see Table 1.
Function/property . | Patients with blindsight . | Patients with Parkinson’s disease with blind to blindsight . | Pathway . | Nuclei/regions involved . |
---|---|---|---|---|
Motion perception | Correct ‘guess’ (without conscious vision) of moving objects in the peripheral field |
| RGES |
|
Reflexive saccades and pursuit | Correct reflexive saccades and smooth pursuit when tracking objects are presented in the blind hemi-field |
| RGES | Idem plus frontal eye fields |
Emotional face recognition and reaction to it | Correct expressive and autonomic reaction to (negative) facial emotions |
| RCTA |
|
Function/property . | Patients with blindsight . | Patients with Parkinson’s disease with blind to blindsight . | Pathway . | Nuclei/regions involved . |
---|---|---|---|---|
Motion perception | Correct ‘guess’ (without conscious vision) of moving objects in the peripheral field |
| RGES |
|
Reflexive saccades and pursuit | Correct reflexive saccades and smooth pursuit when tracking objects are presented in the blind hemi-field |
| RGES | Idem plus frontal eye fields |
Emotional face recognition and reaction to it | Correct expressive and autonomic reaction to (negative) facial emotions |
| RCTA |
|
Function/property . | Patients with blindsight . | Patients with Parkinson’s disease with blind to blindsight . | Pathway . | Nuclei/regions involved . |
---|---|---|---|---|
Motion perception | Correct ‘guess’ (without conscious vision) of moving objects in the peripheral field |
| RGES |
|
Reflexive saccades and pursuit | Correct reflexive saccades and smooth pursuit when tracking objects are presented in the blind hemi-field |
| RGES | Idem plus frontal eye fields |
Emotional face recognition and reaction to it | Correct expressive and autonomic reaction to (negative) facial emotions |
| RCTA |
|
Function/property . | Patients with blindsight . | Patients with Parkinson’s disease with blind to blindsight . | Pathway . | Nuclei/regions involved . |
---|---|---|---|---|
Motion perception | Correct ‘guess’ (without conscious vision) of moving objects in the peripheral field |
| RGES |
|
Reflexive saccades and pursuit | Correct reflexive saccades and smooth pursuit when tracking objects are presented in the blind hemi-field |
| RGES | Idem plus frontal eye fields |
Emotional face recognition and reaction to it | Correct expressive and autonomic reaction to (negative) facial emotions |
| RCTA |
|
Inappropriate guessing of stimuli in the peripheral visual field
Blindsighted patients adequately ‘guess’ without consciously perceiving objects, especially moving ones, in their peripheral visual field. In contrast, patients with Parkinson’s disease are particularly prone to inaccurate guessing and to misinterpretation of peripherally located visual stimuli, experiencing illusions and hallucinations in the peripheral visual field. As such, they often erroneously guess that there may be moving beings in their lateral visual field (sensation de passage) or static beings in their surroundings (sensations de presence) (Fénelon et al., 2000). In a first study on 116 consecutive patients with Parkinson’s disease specifically addressing minor psychotic symptoms (sense of presence, visual illusions, or passage hallucinations) (Fénelon et al., 2010), these symptoms were present in 45% of the patients. Thirty-three patients reported sense of presence hallucinations and 16 passage hallucinations. In the largest published cohort to date (n = 250 non-demented patients with Parkinson’s disease) (Mack et al., 2012), minor psychotic phenomena were seen in 51 patients (20.4%). These were isolated symptoms in 31 patients (12.4%). Passage hallucinations (45 patients) were twice as frequent as presence hallucinations. Other studies with no clear distinction of the different phenomena have prevalence rates up to 72% (Williams et al., 2008). Further exploration of the feeling of presence clearly confirms the projection of the sensation into the extrapersonal space and also demonstrates the frequent co-occurrence of illusions as well as elementary or complex visual hallucinations, thus sustaining its hallucinatory nature (Fénelon et al., 2011). These phenomena have been attributed to side-effects of the dopaminergic treatment in the context of advancing Parkinson’s disease (Bulens et al., 1986). It is possible that excessive dopaminergic stimulation (possibly already at the retinal level) causes inappropriate signalling of the RGES pathway, thus giving rise to erroneous perception of motion in the peripheral visual field. To confirm or reject this information, foveation of the peripheral perception is required, but in Parkinson’s disease, the obligate pursuit saccade is difficult to initiate (see below). Disturbance of the receptive visual field may further enhance the erroneous, subconscious predilection for perception of stimuli in the peripheral visual field. Indeed, during daytime, patients with Parkinson’s disease experience decreased surround inhibition of their receptive visual fields, which mimics the visual properties of light sensitive, wide-angle night vision, enlarging the visual region of interest to include the peripheral visual fields (Bodis-Wollner and Tzelepi, 1998).
Inadequate saccades and deficient motion perception
Blindsighted patients unconsciously detect moving objects and follow them with adequate pursuit movements, or even grasp them. In contrast, patients with Parkinson’s disease have difficulty in rapidly detecting moving objects in their lateral visual field and in visually following them (Trick et al., 1994). Indeed visually triggered saccades are hypometric in Parkinson’s disease and the target is often only reached after several saccades (fragmentation of gaze shifts) (Terao et al., 2013). This excessive hypometria occurs independently of the adaptation direction (centropedal or centrifugal) (MacAskill et al., 2002). Memory-guided saccades are less accurate and difficult to initiate. In the classical model on Parkinson’s disease pathophysiology by Alexander and Crutcher (1990), this impaired initiation is due to increased activity of the inhibitory indirect dopaminergic pathway. As volitional saccades, memory-guided saccades rely on a correct internal representation of the movement pattern and are accessed to recheck a visual stimulus (Terao et al., 2013). Dopaminergic treatment facilitates planed eye movements and also suppresses paradoxical fast ‘reflexive’ saccades, although the contrary, coined superior colliculus dyskinesia, has also been reported (Cubizolle et al., 2013). These findings support the concept that the superior colliculus, pivotal for ‘blindsight’ and instrumental for visuomotor coordination, is the dysfunctional ‘bottleneck of saccades’ in Parkinson’s disease (Terao et al., 2013): visually guided saccades are impaired due to increased inhibition of the superior colliculus through excessive basal ganglia output and memory-guided saccades are impaired due to dysfunctional frontal cortex—superior colliculus circuitries. Paradoxically, hypermetric reflexive saccades can also occur in Parkinson’s disease, when the inhibition of the superior colliculus becomes ‘leaky’ (Sauleau et al., 2008; Terao et al., 2011, 2013). It can be concluded that, independent of the trigger mechanism (internal or external), saccades are dysfunctional in Parkinson’s disease, due to hypometria (mostly) or hypermetria, delayed initiation, or even inadvertent elicitation. In conjunction with prolongation of fixation, a disease-specific impact on the networks directing visual exploration has been postulated (Archibald et al., 2013).
To highlight the comprehensive dysfunction in place, Bodis-Wollner (2008) and Javaid et al. (2010, 2012) successfully applied the concept of pre-emptive perception to visual impairment, and in particular, to saccade impairment in Parkinson’s disease. Even before definitely knowing what the goal of a perception will be, pre-emptive perception covers all mechanisms in place by which the voluntary perception of this eventual target is, first prepared and, later, monitored during its execution. Patients with Parkinson’s disease show impairment both at the level of pre-attentional visual processing (Lieb et al., 1999), and later at the level of saccade execution.
Beyond the superior colliculus, functional MRI demonstrates hypoactivation of the frontal eye fields in patients with Parkinson’s disease during the preparatory phase of voluntary saccades (Rieger et al., 2008). Furthermore, missing intrasaccadic gamma synchronization leads to a lack of modulatory coupling between frontal and posterior mechanisms in patients with Parkinson’s disease (Javaid et al., 2012). Thus, ‘a frontal neuronal circuitry, underlying saccade direction selection is deficient’ (Rieger et al., 2008). However, underlying pathophysiology refers again to the superior colliculus as it connects both anterior oculo-motor and occipital visual regions by a corollary discharge when an eye movement is initiated (Sommer and Wurtz, 2008; Javaid et al., 2012). The disease-inherent hypoactivation of the frontal eye fields can be reversed by adequate dopaminergic treatment, at least in monkeys with MPTP-induced parkinsonism (Ho et al., 1988). Hyperactivation of the frontal eye fields, as elucidated in a kinematic paradigm in patients with Parkinson’s disease with visual hallucinations (Stebbins et al., 2004) probably results from inadequate dopaminergic overstimulation.
The excessive inhibition of the superior colliculus is directly due to the overactive substantia nigra pars reticulate. In non-human primates electrical stimulation of the substantia nigra pars reticulata replicates this impairment, with reduction in velocity and amplitude of both visually or memory-triggered saccades by this procedure (Basso and Liu, 2007). Conversely, reduction of the substantia nigra pars reticulata inhibition, as achieved by deep brain stimulation of the nucleus subthalamicus has the reverse effect, as shown in several studies (Rivaud-Péchaux et al., 2000; Yugeta et al., 2010; Nilsson et al., 2013). In the largest study (Yugeta et al., 2010), 32 medicated patients with Parkinson’s disease were studied in the deep brain stimulation-on condition; the delay for saccade initiation was shorter for both visually and memory guided saccades, and the amplitude was shorter for visually guided saccades. In a smaller study a similar favourable impact of deep brain stimulation treatment on saccade amplitude accuracy was observed after complete withdrawal of the medication (Nilsson et al., 2013). These studies suggest that, indeed, dysfunctional activation of the superior colliculus underlies saccadic abnormalities in Parkinson’s disease.
Deficient negative emotional face perception and blunted autonomic reaction
Blindsighted patients appropriately respond, both expressively and by autonomic testing, to emotional facial expressions, especially those expressing fear or danger. In contrast, patients with Parkinson’s disease are impaired in these same facial emotion recognition performances. In a pioneer study, Sprengelmeyer et al. (2003) showed that there is evidence of impaired recognition of facial expressions in both medicated and unmedicated patients with Parkinson’s disease. This impairment concerned the recognition of the facial emotion expressions disgust, fear and anger. Other authors have confirmed the trend of impaired recognition of especially negative emotional face expressions and pointed out that, in comparison to controls, patients with Parkinson’s disease use different perceptual processes and cognitive strategies during visual exploration of emotional facial expressions (Pell and Leonard, 2005; Suzuki et al., 2006; Assogna et al., 2008; Clark et al., 2008, 2010). This deficient perception is not related to overall disease severity or to depression (Lawrence et al., 2007). Part of the underlying visual deficit that leads to poor emotional recognition may relate to reduced low contrast discrimination, a well-established deficit experienced by patients with Parkinson’s disease (Bulens et al., 1986; Diederich et al., 2002; Bodis-Wollner, 2008). Indeed, it has been shown that contrasts containing low spatial frequencies are crucial for immediate appreciation of any (dangerous) change of the outside world and for the rapid processing of emotional face expressions (Vuilleumier et al., 2003). However, surprisingly, these deficits do not manifest themselves similarly, but only sequentially, with reduced performances of low contrast discrimination appearing first and reduced facial emotion recognition appearing later (Hipp et al., 2014). Notably, Sprengelmeyer (2003), while also reporting lower performances in contrast discrimination in patients with Parkinson’s disease, did not discuss the potential pathophysiological link with facial emotion recognition, reported by Vuilleumier (2003) in healthy subjects. We propose that subjects with Parkinson’s disease lose the ability to profit from this ‘early warning system’ and consequently show abnormal or at least delayed responses to facial emotions, while in contrast, ‘affective blindsight’ is fully operational in patients with ‘blindsight’ vision.
As mentioned above, the superior colliculus is sensitive to both achromatic and S-cone-isolating stimuli and ‘blindsight’ vision can partially read red stimuli (Barbur et al., 2004; Leh et al., 2006b). Patients with Parkinson’s disease show prominent reduced colour discrimination (Büttner et al., 1995; Diederich et al., 2002; Sartucci et al., 2003), even in early and unmedicated stages of the disease. The luminance pattern electroretinogram of the tritan axis (blue/yellow spectrum) may show the earliest changes (Sartucci et al., 2003; Silva et al., 2005), but involvement of this colour axis is considered to be an independent ageing phenomenon, thus irrelevant for the RCTA functioning. In contrast, the more pronounced deficits along the protan/deutan axis (red/green spectrum) compromise the RCTA signalling and thus ‘blindsight’ vision. Dysfunction of the RCTA reduces the most efficient ‘expedient detection’ of negative face expressions, forcing the patient with Parkinson’s disease to rely on a greater number of fixations when scanning a face of interest (Clark et al., 2010). This more tedious procedure potentially leads to errors or delayed responses.
Again in contrast to patients with blindsight, patients with Parkinson’s disease also show reduced autonomic reactions when viewing emotionally loaded images. A recent physiological study demonstrated a reduced amplitude of the emotionally driven centro-parietal late positive potential in patients with Parkinson’s disease viewing unpleasant images (Dietz et al., 2013). This study has echoed reports showing blunted eyeblink startle reactions in patients with Parkinson’s disease exposed to photos with negative emotional face content: fear, anger, disgust; or photos showing cruel scenes (e.g. mutilations) (Bowers et al., 2006; Miller et al., 2009). In contrast to control subjects, patients with Parkinson’s disease did not produce more marked responses to images with higher emotional valence than to images with lower emotional valence. Reduced sympathetic skin conductance in patients with Parkinson’s disease viewing faces with negative emotional expressions could possibly only reflect a peripheral deficit, as Lewy body pathology also occurs in sympathetic ganglia of the skin (Bowers et al., 2008). Finally, pupillometry has been proposed as a ‘window to the preconscious’ (Laeng et al., 2012) and used for studying the autonomic reactions to visual stimuli. A small study did not observe any difference in pupillary dynamics in response to emotional expressions between patients with Parkinson’s disease and healthy controls, all viewing emotional face expressions (Dietz et al., 2011). The results are difficult to interpret, because the patients with Parkinson’s disease were tested while ON dopaminergic medication and half of them were also taking antidepressants. Pharmacologically, SNRI (serotonin–norepinephrine reuptake inhibitors) may have partially compensated for disease-inherent noradrenergic deficits in pupillary dynamics, usually mediated by the locus coeruleus (Laeng et al., 2012). Furthermore, rapid as well as ample, pupillary responses cannot be expected in patients with Parkinson’s disease as variations in pupillary diameter in general are reduced and slower than in controls (Micieli et al., 1991). When trying to globally explain these diminished autonomic reactions, authors have hypothesized on autonomic hypoarousal due to amygdala dysfunction in Parkinson’s disease, secondary to dysfunctional RCTA pathway (Miller et al., 2009). This pathway dysfunction can only be posited at this point, and specific experiments must be designed to examine the issue.
Taken together, these data suggest impairment in patients with Parkinson’s disease of several visual parameters that are generally preserved within the blindsight visual system, namely detection of motion in the lateral field, initiation and execution of appropriately targeted saccades, ‘first glimpse’ rapid visual appreciation of negative (vitally important?) facial expressions.
Supportive neuropathology and neuroimaging
We are unaware of any functional MRI study directly exploring pathways and nuclei specifically involved in blindsight in patients with Parkinson’s disease. Voxel-based morphometric studies have only reported a correlation between facial emotion recognition of sadness and the grey matter volume of the amygdala in Parkinson’s disease. Correlations between the recognition of disgust and the grey matter volume of the anterior cingulate cortex have also been reported (Ibarretxe-Bilbao et al., 2009; Baggio et al., 2012). As mentioned above, neurophysiological data implicate that in Parkinson’s disease the superior colliculus is constantly inhibited by the basal ganglia, while for adequate visual guidance, intermittent disinhibition of the superior colliculus would be required. Beyond this pathophysiological argument for secondary involvement of the superior colliculus in Parkinson’s disease, there are histochemical data documenting excessive inhibition of the superior colliculus in patients with Parkinson’s disease. Relevant studies have examined autopsies of patients with Parkinson’s disease, using the marker for messenger RNA coding of GAD1. The substantial reduction in GAD1 messenger RNA expression documented in superior colliculus is probably due to the assumed hyperactivity of the inhibitory nigrotectal pathway (Vila et al., 1996).
Extensive neuropathological autopsy data have been reported in patients with Parkinson’s disease with visual hallucinations. In a seminal autopsy study on patients with Parkinson’s disease with hallucinations and with particular emphasis on the amygdala, Harding et al. (2002) documented that the different amygdala subnuclei do not show uniform burden of Lewy body, as more selective involvement with cellular loss and high burden of Lewy body was seen in the subnucleus called the cortical amygdaloid nucleus. Other studies have confirmed higher Lewy body burden in the basal and lateral amygdaloid nuclei, but also in the claustrum and the pulvinar (Braak et al., 1994, 2003; Iseki et al., 2007). In an autopsy study focusing on patients with Lewy body dementia and visual hallucinations, 15 of 19 patients showed significant neuronal cell loss, most marked in the lateral and basal amygdaloid nucleus (Yamamoto et al., 2006). The same investigators described marked Lewy body pathology in the pulvinar and claustrum, as well as the insular and inferior temporal cortices. They reported mild cell loss in the lateral geniculate nucleus and suggested that dysfunction of both the visuo-claustral and the visuo-amygdaloid pathway may contribute to visual misidentification syndromes in Parkinson’s disease (Yamamoto et al., 2007). Braak et al. (2003) and Halliday (2009) did not report Lewy body in the lateral geniculate nucleus, although thalamic nuclei are prominently affected by the disease process. Based on neurophysiological and neuroimaging studies, the concept of the ‘silent amygdala’ has been coined to explain conjoint odour misidentification, autonomic disturbances, and visual hallucinations in patients with Parkinson’s disease (Yoshimura et al., 2005). Indeed, the amygdala plays an essential role for adequate appreciation of and body response to the emotional salience of various perceptions. In summary neuropathological and neuroradiological literature provides ample evidence for blindsight pathways’ involvement in Parkinson’s disease without, however, specifically referring to blindsight as an underlying concept. Again, these findings contrast with selective preservation of these unconscious pathways in patients with blindsight.
Discussion
The proposal of ‘blind to blindsight’ in Parkinson’s disease is in line with the recently proposed model by Redgrave et al. (2010a, b) on the pivotal role of the basal ganglia in ‘habitual and goal-directed’ actions. As proposed by these authors, the predominant dopamine loss in the posterior putamen forces patients with Parkinson’s disease to rely on goal-directed, inherently slower modes of action. Extended to vision, this model means that rapid blindsight-mediated visual functions, as outlined above, have to be replaced by the longer-latency performances of the neocortical visual pathway. We highlight here a few parkinsonian features, beyond the hallucinations of ‘passage’ and ‘presence’ already explicitly discussed above, that may result from this blind to blindsight syndrome and contribute to parkinsonian disability and diminished quality of life. As alluded to above, the unconscious emotional perception of faces contributes to rapid and intense body reactions, including autonomic responses, respiration and facial movements; all important to social interactions and communication (Frith, 2009). It is possible that the marked hypomimia of patients with Parkinson’s disease not only reflects bradykinesia of facial muscles, but also mirrors deficient emotional reactivity mediated by the RTCA. ‘Theory of mind’, or the ability to reason about other people’s mental states to explain and predict their behaviour (Fletcher et al.,1995), both in its affective and cognitive components, is altered even in early Parkinson’s disease stages and may not only be due to disturbed fronto-subcortical circuitries, but also to reduced subconscious appreciation of emotional facial expressions in direct face-to-face interactions (Bodden et al., 2010; Roca et al., 2010; Narme et al., 2013). The encountered vehicle driving difficulties of patients with Parkinson’s disease may not only be mediated by reduced vigilance spans, prolonged reaction times or deficient decision making, but may also be due to reduced rapid screening of the peripheral visual field by automatic exploratory saccades, respectively by reduced motion detection due to malfunction of collicular oculo-motor pathways. Patients with Parkinson’s disease show deficiencies in exploring the visual field and score worse than age-matched controls on measures of visual processing speed, attention and motion perception. They are particularly handicapped when driving under low-contrast visibility conditions (Uc et al., 2006, 2007, 2009).
Limitations
The present essay reviews knowledge on visual and visually-related neurobehavioral symptoms in Parkinson’s disease. We emphasize that not all visual and ocular deficits encountered in patients with Parkinson’s disease can be classified within the new concept. Whereas impaired saccadic and smooth pursuit can be explained within this paradigm, other restrictions of oculomotor movements, such as loss of the convergence reflex and limitation of the upward gaze (Jankovic, 2003), do not specifically fit into the present concept. Furthermore, the ‘blind to blindsight’ hypothesis focuses on unconscious visual networks, whereas numerous studies have shown involvement of conscious neocortical visual pathways, especially in more advanced disease stages. These studies have focused on freezing of gait and hallucinations, and in such studies, we consider the concept of segregated visual pathways as suggested by Goodale and Milner (1992) to be highly pertinent. As predicted by that framework, patients with freezing of gait show involvement of the dorsal visual pathways (Lord et al., 2012), whereas marked atrophy of numerous regions involved in both the ventral ‘what’ and the dorsal ‘where’ pathways is visible in patients with hallucinations (Goldman et al., 2014). Atrophy may not only be the consequence of local Lewy body pathology as predicted by Braak’s hypothesis on ascending degeneration in Parkinson’s disease, but also a consequence of pathological deactivation, during or before visual perception (Meppelink et al., 2009) and, more fundamentally, as part of malfunctioning of the default mode network (van Eimeren et al., 2009; Onofrj et al., 2013).
Nonetheless, because so many studies have been conducted without a specific and consolidating hypothesis on visual dysfunction in patients with Parkinson’s disease, we offer this ‘blind to blindsight’ as a new conceptual framework to integrate many types of visual impairments in Parkinson’s disease. Blindsight has been posited as a function of ‘phylogenetically old parts of the brain’ (Widakowich, 2001). Patients with Parkinson’s disease may suffer from other symptoms due to dysfunction of archaic networks, such as loss of gait automatization and hyposmia (Diederich and Parent, 2012). It is possible that secondary neuronal plasticity and network reorganization compensate some of the encountered deficits due to deficient blindsight; these compensatory changes, while reducing the clinical repercussions of the syndrome, would also echo the compensatory changes operative in patients with blindsight (Stoerig and Cowey, 1997; Bridge et al., 2008). The authors also agree with Tamietto and de Gelder (2010) that conscious and non-conscious pathways may be anatomically distinct, but not functionally independent, although we have conceptually done so to unravel the ‘blindsight’ deficit in patients with Parkinson’s disease. As with any introductory model, criticisms of simplicity can be lodged, and the authors accept this very legitimate criticism. Nonetheless, from this model, testable hypotheses can be developed logically, with experimental paradigms to confirm or refute these tenets.
Future perspectives and testable hypotheses
Neuropathological studies focusing on the pivotal areas involved in ‘blindsight’ vision, namely the superior colliculus, lateral geniculate nucleus and pulvinar have to be encouraged in patients with Parkinson’s disease. The use of diffusion tensor imaging may be able to track in vivo the involvement of subcortical pathways in patients with Parkinson’s disease and controls (Leh et al., 2006a). The application of transcranial magnetic stimulation over the visual cortex, with transient blockade of the conscious visual pathways, without suppression of the non-conscious pathways may also be an appealing neurophysiological approach (Ro et al., 2004; Jolij and Lamme, 2005; Allen et al., 2014). By using this technique in patients with Parkinson’s disease, the extent to which both conscious and unconscious pathways contribute and interrelate to visual dysfunction could be better delineated. Patients with Parkinson’s disease may actually facilitate fundamental vision research by studying exclusively conscious primary visual cortex-extrastriate vision without concurrent ‘unconscious’ vision. From a clinical point of way, prospective studies examining the evolution of the blind to blindsight syndrome should explore secondarily reduced quality of life and other measures of Parkinson’s disease disability.
Abbreviations
- RCTA
retino-colliculo-thalamo-amygdala pathway
- RGES
retino-geniculo-extrastriate pathway