-
PDF
- Split View
-
Views
-
Cite
Cite
Calvin D De Louche, Manish Mandal, Lee Fernandes, Jason Lawson, Colin D Bicknell, Anna L Pouncey, Heart rate variability as a dynamic marker of surgeons’ stress during vascular surgery, BJS Open, Volume 8, Issue 5, October 2024, zrae097, https://doi.org/10.1093/bjsopen/zrae097
- Share Icon Share
Abstract
A surgeon experiences elevated stress levels when operating. Acute stress is linked to cognitive overload, worsening surgical performance. Chronic stress poses a significant risk to a surgeon’s health. Identifying intraoperative stress may allow for preventative strategies that reduce surgeons’ stress and subsequently improve patient outcomes. The aim of this study was to assess the feasibility of using heart rate variability as a marker of stress during vascular surgery.
A total of 11 senior surgeons were evaluated performing three different vascular surgery procedures. Heart rate variability metrics (low-frequency to high-frequency ratio and standard deviation of the normal-normal interval) were determined from single-lead ECG traces at predetermined procedural performance points. State-Trait Anxiety Inventory-6, a validated stress tool, was used to assess surgeon-reported stress. Subjective reports of procedural difficulty were also collected. One-way ANOVA compared heart rate variability at key performance points with baseline. Pearson’s coefficient assessed correlation between heart rate variability and subjective stress.
Data were collected for six carotid endarterectomies, six open abdominal aortic aneurysm repairs, and five lower limb bypasses. Heart rate variability metrics indicating markedly greater stress were observed at key performance points across all procedures. Peaks in stress were consistent across different surgeons performing the same procedure. A significant correlation was observed between heart rate variability metrics and subjective State-Trait Anxiety Inventory-6 stress reports (r = 0.768, P =<0.001). The most difficult procedural steps reported corresponded with heart rate variability metrics displaying the greatest stress.
Heart rate variability may be a viable approach to assess intraoperative stress and cognitive load during vascular surgery and could be used to evaluate whether a theatre intervention (for example timeout) could reduce stress in areas of surgical difficulty.
Introduction
Surgery is an intensely demanding profession, with surgeons regularly experiencing significant stress levels whilst operating1–3. Stress is defined as a dynamic physical and emotional response to developing environmental challenges that are perceived to be difficult or uncomfortable4. The physiological and psychological effects of stress result primarily via the activation of the autonomic nervous system (ANS), which can be divided into two components: the sympathetic nervous system (SNS) and the parasympathetic nervous system (PNS)5. During the stress response, an up-regulation in SNS activity and a decrease in PNS activity is associated with the classical physiological stress phenotype that includes an increase in heart rate, blood pressure, and respiratory rate4,5. When operating, surgeons are required to perform technically challenging tasks, for which a suitable level of intraoperative arousal is imperative to augment their performance6. However, significantly elevated stress levels (above the constructive threshold) can lead to cognitive overload6. Hence, determining the balance of intraoperative stress that maximizes surgical performance, whilst not being of detriment, is of particular interest.
More recently, intraoperative stress has become increasingly scrutinized, as it represents a notable occupational hazard to surgeons7. Chronic stress is associated with an increased risk of cardiovascular disease, morbidity, and mortality8–10. Considerably dysregulated stress levels may not solely affect a surgeon’s physiological well-being, but also adversely impact upon their technical performance and important non-technical skills, such as team awareness and communication6. Raised levels of intraoperative stress may endanger patient safety and lead to worse postoperative outcomes6,11. Dysregulated levels of intraoperative stress are thought to originate from the increased requirement for concentration and technical performance at critical procedural stages, in combination with repeated acute stressors, such as bleeding, distractions, or equipment issues12,13. Therefore, an ability to identify acute stress and cognitive overload may enhance the understanding of relevant risk factors for intraoperative stress. Specifically, identification of key performance points in core procedures that induce higher stress levels may allow for preventative strategies that reduce stress, improving outcomes for surgeons and their patients14,15.
Heart rate variability (HRV) is a measure of variation in the time between R waves of adjacent QRS complexes (termed the normal-normal (NN) interval) and is a powerful marker of physiological (cardiac) health16. Moreover, HRV measurements can be used to objectively measure a surgeon’s stress and cognitive load in the operating theatre15. By employing time and frequency domain analyses, HRV measurements provide a sensitive indication of fluctuation in ANS functioning and allow for the relative contributions of SNS and PNS outflow to be determined16,17. Overarchingly, increased HRV in time domain analyses and a reduced low-frequency to high-frequency (LF/HF) ratio in frequency domain analyses indicate good capability for the ANS to adapt to stressors. Conversely, reduced HRV in time domain analyses and an elevated LF/HF ratio in frequency domain analyses are associated with stress and cognitive overload16. Therefore, combined HRV measurements may serve as reliable, non-invasive measures of intraoperative stress and cognitive load15.
Multiple studies have already demonstrated the potential of HRV to indicate general intraoperative stress2,18–22, but also more specific stressful key procedural performance points14,17,23–25. For instance, in a study of neurosurgeons performing microsurgical aneurysm clipping, an increased LF/HF ratio and reduced HRV were observed at critical operative stages24. Similarly, in a case study of a cardiac surgeon performing repeated coronary artery bypass grafts, an increased LF/HF ratio was observed at key operative performance points (for example aortic cross-clamping)14. Further, for a group of trainee vascular surgeons performing a simulated carotid endarterectomy (CEA), increased HRV was associated with higher coping strategy scores, superior technical outcomes, and a reduction in observed stress6. Moreover, an overall decrease in HRV for surgeons self-reporting as stressed in the perioperative interval indicated poor ANS recovery in the postoperative interval26. These findings support the potential for HRV to indicate intraoperative stress at key performance points and suggest that self-reported stress levels may correlate with HRV metrics to predict autonomic recovery.
Despite promising findings in other surgical disciplines, the correlation between HRV, surgeons’ stress, and key intraoperative performance points during vascular surgery has not been fully explored outside of a simulated environment; a simulated environment is unlikely to reflect the real-world stress of undertaking vascular surgery. Additionally, many existing reports focus on HRV measurements alone, with such measurements providing a practical objective measure of stress, however, these reports do not assess any potential correlation between HRV measurements and a more subjective evaluation of intraoperative stress17. Therefore, the hypothesis was that changes in HRV measurements observed during vascular surgery correlated with key areas of surgical difficulty and subjective reports of a surgeon’s stress. The aim of this study was to assess the association between change in HRV measurements (specifically the LF/HF ratio and the standard deviation of the NN interval (SDNN)) and key performance points (associated with a greater cognitive load) during core vascular surgery procedures, subjective reports of procedural difficulty, and subjective reported measures of a surgeon’s stress to evaluate the feasibility of using HRV as a marker of intraoperative stress in vascular surgery.
Methods
Ethics approval
Ethics approval for this study was granted by the Health Research Authority (Integrated Research Application System (IRAS) project ID 311544, Research Ethics Committee (REC) reference 22/HRA/0474). Confirmation of local National Health Service (NHS) trust capacity and capability was obtained before commencement from Imperial College Healthcare NHS Trust.
Recruitment
This study was a prospective observational cohort study of senior vascular surgeons operating at the participating centre (St Mary’s Hospital, Paddington, London, UK) between 24 March 2022 and 22 April 2022. Exclusion criteria included any existing underlying cardiac disease (significant arrhythmias, pacemaker dependencies, and congestive cardiac failure). Eligible participants were provided with a participant information sheet explaining the study’s design and aims. Willing participants provided signed consent for their involvement in the study and were free to withdraw at any time.
Collection of routine clinical data
Participants were allocated a study identification number known only to them and the researcher, allowing data to be pseudonymized. Details related to factors that could alter HRV metrics and the timing/magnitude of the observed stress response were recorded. These included participant age (associated with vagal attenuation), sex, confounding clinical conditions (including diabetic polyneuropathy and renal disease, which both reduce HRV), confounding medications (including beta-blockers and tricyclic antidepressants), volume/intensity of physical exercise, and intake of caffeine, alcohol, and nicotine27.
Subjective assessment of stress
Subjective stress levels were assessed using the State-Trait Anxiety Inventory (STAI) questionnaire, a validated assessment tool used extensively to quantify self-reported stress levels amongst clinicians28,29. In this study, a validated shortened version of the STAI, termed the STAI-6, was used30. This contained only 6 items rather than the original 40 and was much faster to complete perioperatively30. Responses used a four-point Likert-style scale to indicate the level of agreement with statements. STAI-6 scores could range from 6 to 24, with a higher score indicating a more significant self-reported stress level. Participating surgeons completed the STAI-6 questionnaire before and after surgery. A procedure was deemed ‘stressful’ when there was a postoperative increase in raw STAI-6 score of greater than or equal to one compared with the preoperative score1. The percentage change in STAI-6 score was calculated. Additionally, surgeons rated how difficult they anticipated a procedure would be before surgery on a Likert scale of one to ten (1 = not at all and 10 = extremely difficult). After surgery, surgeons rated how difficult they felt the procedure was in retrospect and identified any procedural steps that they felt were difficult.
Assessment of heart rate variability
Ambulatory ECG monitoring was performed using the Bittium Faros™ 180 device (Bittium Corporation, Oulu, Finland)31. This was a medical Ce and Food and Drug Administration (FDA) (k) cleared device designed for single-lead ECG monitoring, with a capacity to sample up to 1000 Hz with three-dimensional acceleration sampling up to 100 Hz31. This allowed recording of HRV metrics to an appropriate level of precision as outlined by the Task Force of the European Society of Cardiology guidelines16.
The Bittium Faros™ 180 device was fitted securely to a surgeon’s sternum using two standard Ambu® WhiteSensor 4535M ECG electrodes (Ambu Inc., Ballerup, Denmark) (Figure S1). Continuous ambulatory monitoring occurred from the start of the preoperative baseline interval, which lasted for 10 min and required the surgeon to be de-scrubbed, sat down, and at rest, until the device was removed at the end of the procedure.
Key performance points associated with a greater cognitive load for three vascular surgical procedures (CEA, open abdominal aortic aneurysm (AAA) repair, and lower limb bypass (LLB)) were identified by two independent vascular surgeons not enrolled in this study (Table 1). Key performance points identified were also cross-referenced with the available literature32–35. During the operation, the timings of when these key performance points occurred were recorded to allow identification of the corresponding section of the ECG trace. Additionally, the timings of any procedure-related or non-procedure-related interruptions (for example distractions, anaesthetist input, and patient/equipment issues) were also recorded.
Key intraoperative performance points identified for each vascular surgery procedure
Procedure . | Key performance point . |
---|---|
Carotid endarterectomy |
|
Open abdominal aortic aneurysm repair |
|
Lower limb bypass |
|
Procedure . | Key performance point . |
---|---|
Carotid endarterectomy |
|
Open abdominal aortic aneurysm repair |
|
Lower limb bypass |
|
Key intraoperative performance points identified for each vascular surgery procedure
Procedure . | Key performance point . |
---|---|
Carotid endarterectomy |
|
Open abdominal aortic aneurysm repair |
|
Lower limb bypass |
|
Procedure . | Key performance point . |
---|---|
Carotid endarterectomy |
|
Open abdominal aortic aneurysm repair |
|
Lower limb bypass |
|
Data analysis
Data from the Bittium Faros™ 180 device were transferred to the secure Imperial environment in European Data Format. HRV analysis was performed using HRVanalysis software (SNA-EPIS Laboratory, Saint-Etienne, France), a dedicated package capable of determining HRV metrics from single-lead ECG traces36. ECG traces were processed to exclude artefact data, ectopic beats, and aberrant traces. NN intervals were detected. Gaps created by the removal of artefact data were interpolated. Timings on ECG traces were then aligned with timings recorded for key intraoperative events.
Short-term analysis of ECG traces at key performance points was performed according to the Task Force of the European Society of Cardiology guidelines16. After extensive pilot testing, 120 s was chosen as the appropriate window length for analysis, as it complied with the guidelines and was deemed short enough to capture HRV changes relating to the fast-paced nature of vascular surgery16,37. Time domain HRV analyses, including the SDNN, the square root of mean squared differences of adjacent NN intervals, and the number of interval differences of adjacent NN intervals greater than 50 ms, are all direct measures of variation in the NN interval. However, they do not provide detailed information regarding sympathetic output15,16,38. Frequency domain HRV analyses report the energy in specific frequencies (termed the power density spectrum) of the ECG trace15. The main frequency bands include the low-frequency band (0.04–0.15 Hz), associated with SNS activity that increases heart rate and contractility, and the high-frequency band (0.15–0.40 Hz), associated with PNS activity that reduces heart rate and contractility38. There is, however, likely substantial overlap between the contributions of the SNS and PNS to both bands16,38. Nonetheless, determining the LF/HF ratio is widely accepted as the most sensitive indicator of overall sympathovagal balance, as it forms a directly proportionate relationship with SNS outflow39. Hence, the LF/HF ratio represents a valid metric to quantify overall sympathetic tone as a surrogate marker of intraoperative stress16,40–42.
Time and frequency domain HRV metrics were calculated from ECG traces at pre-specified key performance points by determining the mean metric from all 120 s windows taken during the key step. The SDNN was reported in the time domain analyses, as it represented the most reported HRV time domain metric in the existing body of literature15. Frequency domain analyses used a fast Fourier transform algorithm to generate measurements of low-frequency and high-frequency bands, from which the LF/HF ratio could be calculated. HRV metrics were normalized against baseline readings, allowing for percentage change comparisons across different participants. Mean intraoperative HRV metrics were determined from data taken from intraoperative 120 s windows across all key steps. The primary outcome measure was the LF/HF ratio and the secondary outcome measure was the SDNN.
Statistical analysis
Statistical analyses were performed using GraphPad Prism (GraphPad Software Inc., CA, USA; version 9.0) and SPSS® (IBM, Armonk, NY, USA; version 26.0). Data normality was assessed via the Shapiro–Wilk test. Categorical data are presented as n (%). Descriptive data are presented as mean(s.d.) (parametric) or median (interquartile range) (non-parametric). Intraoperative HRV metric data are presented as mean(s.e.m.). Comparisons between subjective reports of stress were performed using an unpaired t test. Comparison of the percentage change in HRV metrics relative to baseline was performed using one-way ANOVA with Dunnett’s multiple comparisons43. An unpaired t test and Pearson’s correlation coefficient assessed the association between HRV metrics and subjective stress reports. P < 0.05 (two-tailed) was considered statistically significant.
Results
Summary of participants/cases
A total of 11 senior surgeons took part in this study (8 men and 3 women); seven were consultant grade and four were ST8-level registrars in their final year of vascular surgical training, of which three had achieved their certificate of completion of training. The mean age of participants was 41.64(7.50) years. The mean total surgical experience (calculated from the start of core surgical training) was 15.91(6.19) years. The median number of cups of coffee consumed by participants per day was 3 (2–3). The mean number of units of alcohol consumed per week was 11.32(10.39). The median number of hours of ‘heavy’ exercise performed per week was 2 (2–6). All participants were free from any cardiac, endocrine, or renal disorders. One participant smoked 40 cigarettes per week. One participant was taking candesartan for hypertension.
Single-lead ECG traces were obtained during 17 vascular surgical procedures: six CEAs, six open AAA repairs, and five LLB operations, with a mean operating time of 118.40(17.96), 281.20(82.69), and 195.60(71.42) min respectively. The monitored surgeon was the primary operator and familiar with their team in all cases. All procedures were completed successfully.
Assessment of heart rate variability
Carotid endarterectomy
Unadjusted changes in HRV metrics for each CEA are presented in Fig. 1a,b. Within operation 3, an episode of patient asystole occurred at the time point indicated with a resultant spike in LF/HF ratio (Fig. 1a). A decrease in SDNN was not observed at the same time point (Fig. 1b).
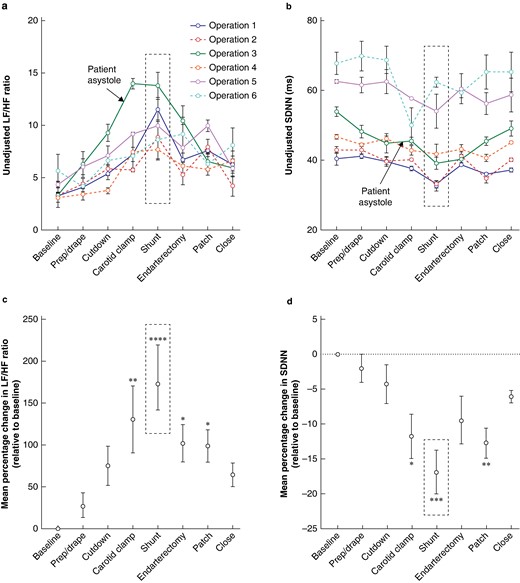
Changes in heart rate variability associated with key performance points during the carotid endarterectomy procedure
a Unadjusted changes in the heart rate variability metric in the frequency domain (low-frequency to high-frequency ratio) for each carotid endarterectomy operation and any procedure or non-procedure-related interruptions that occurred. b Unadjusted changes in the heart rate variability metric in the time domain (standard deviation of the normal-normal interval) for each carotid endarterectomy operation and any procedure or non-procedure-related interruptions that occurred. An increase in low-frequency to high-frequency ratio and a decrease in standard deviation of the normal-normal interval indicate greater stress. Individual points represent the mean values at each step; error bars represent s.e.m. The black dotted box surrounds the subjectively reported most difficult step. c Mean percentage change in the frequency (low-frequency to high-frequency ratio) heart rate variability metric relative to baseline across all carotid endarterectomy procedures. d Mean percentage change in the time (standard deviation of the normal-normal interval) heart rate variability metric relative to baseline across all carotid endarterectomy procedures. Points represent the mean percentage change in metric relative to baseline; error bars represent s.e.m. The black dotted box surrounds the subjectively reported most difficult step. One-way ANOVA with Dunnett’s multiple comparisons was performed to compare the mean percentage change in metric at key performance points with baseline (six CEAs). P < 0.05 was considered statistically significant. *P < 0.05. **P < 0.01. ***P < 0.001. ****P < 0.0001. When not indicated, the mean percentage change in metric relative to baseline was deemed non-significant (P ≥ 0.05). LF/HF, low-frequency to high-frequency; SDNN, standard deviation of the normal-normal interval.
In CEA procedures, the most significant mean percentage increase in LF/HF ratio relative to baseline was observed during shunt insertion (180.30%(38.95%); P < 0.001), with a significant increase also observed for carotid clamping (130.30%(40.07%); P = 0.004), endarterectomy (102.00%(22.46%); P = 0.034), and patching (98.59%(19.40%); P = 0.043) (Fig. 1c). The most significant mean percentage decrease in SDNN relative to baseline was also observed during shunt insertion (−16.90%(3.10%); P < 0.001), with a significant decrease also observed for patching (−12.73%(2.16%); P = 0.005) and carotid clamping (−11.77%(3.16%); P = 0.010) (Fig. 1d). Endarterectomy was non-significant in the time domain analysis, despite showing significance in the frequency domain.
The most difficult step reported subjectively in all cases was shunt insertion. This step produced the greatest changes in HRV metrics in both domains (Fig. 1a,b,c,d).
Open abdominal aortic aneurysm repair
Unadjusted changes in HRV metrics for each open AAA repair are presented in Fig. 2a,b. The most significant mean percentage increase in LF/HF ratio relative to baseline was observed during aortic clamping (238.10%(52.44%); P < 0.001), with a significant increase also observed for proximal graft anastomosis (182.50%(27.40%); P < 0.001), distal graft anastomosis (139.60%(18.89%); P = 0.004), and dissection of aneurysm sac (136.10%(8.53%); P = 0.005) (Fig. 2c).
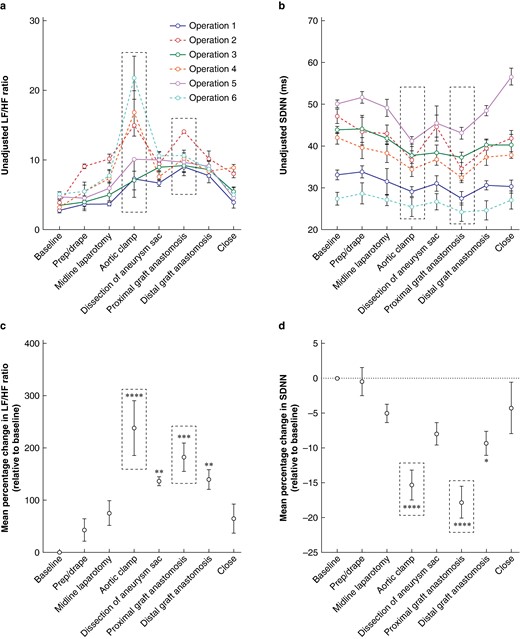
Changes in heart rate variability associated with key performance points during the open abdominal aortic aneurysm repair procedure
a Unadjusted changes in the heart rate variability metric in the frequency domain (low-frequency to high-frequency ratio) for each open abdominal aortic aneurysm repair operation. b Unadjusted changes in the heart rate variability metric in the time domain (standard deviation of the normal-normal interval) for each open abdominal aortic aneurysm repair operation. An increase in low-frequency to high-frequency ratio and a decrease in standard deviation of the normal-normal interval indicate greater stress. Individual points represent the mean values at each step; error bars represent s.e.m. The black dotted box surrounds the subjectively reported most difficult step. c Mean percentage change in the frequency (low-frequency to high-frequency ratio) heart rate variability metric relative to baseline across all open abdominal aortic aneurysm repair procedures. d Mean percentage change in the time (standard deviation of the normal-normal interval) heart rate variability metric relative to baseline across all open abdominal aortic aneurysm repair procedures. Points represent the mean percentage change in metric relative to baseline; error bars represent s.e.m. The black dotted box surrounds the subjectively reported most difficult step. One-way ANOVA with Dunnett’s multiple comparisons was performed to compare the mean percentage change in metric at key performance points with baseline (six open abdominal aortic aneurysm repairs). P < 0.05 was considered statistically significant. *P < 0.05. **P < 0.01. ***P < 0.001. ****P < 0.0001. When not indicated, the mean percentage change in metric relative to baseline was deemed non-significant (P ≥ 0.05). LF/HF, low-frequency to high-frequency; SDNN, standard deviation of the normal-normal interval.
The most significant mean percentage decrease in SDNN relative to baseline was observed during proximal graft anastomosis (−17.80%(2.30%); P < 0.001) and aortic clamping (−15.31%(2.14%); P < 0.001). A significant decrease was also observed during distal graft anastomosis (−9.32%(1.73%); P = 0.018) (Fig. 2d). Dissection of aneurysm sac was non-significant in the time domain analysis, despite showing significance in the frequency domain.
The most difficult steps reported subjectively in 83% of cases were aortic clamping and proximal graft anastomosis. Aortic clamping produced the greatest change in the HRV frequency domain metric, whilst proximal graft anastomosis produced the greatest change in the HRV time domain metric (Fig. 2a,b,c,d).
Lower limb bypass
Unadjusted changes in HRV metrics for each LLB are presented in Fig. 3a,b. At the time point indicated in operation 5, anaesthetic concerns resulted in repeated interruptions to the procedure. This correlated with a marked rise in LF/HF ratio (Fig. 3a) and a steep decrease in SDNN (Fig. 3b).

Changes in heart rate variability associated with key performance points during the lower limb bypass procedure
a Unadjusted changes in the heart rate variability metric in the frequency domain (low-frequency to high-frequency ratio) for each lower limb bypass operation and any procedure or non-procedure-related interruptions that occurred. b Unadjusted changes in the heart rate variability metric in the time domain (standard deviation of the normal-normal interval) for each lower limb bypass operation and any procedure or non-procedure-related interruptions that occurred. An increase in low-frequency to high-frequency ratio and a decrease in standard deviation of the normal-normal interval indicate greater stress. Individual points represent the mean values at each step; error bars represent s.e.m. The black dotted box surrounds the subjectively reported most difficult step. c Mean percentage change in the frequency (low-frequency to high-frequency ratio) heart rate variability metric relative to baseline across all lower limb bypass procedures. d Mean percentage change in the time (standard deviation of the normal-normal interval) heart rate variability metric relative to baseline across all lower limb bypass procedures. Points represent the mean percentage change in metric relative to baseline; error bars represent s.e.m. The black dotted box surrounds the subjectively reported most difficult step. One-way ANOVA with Dunnett’s multiple comparisons was performed to compare the mean percentage change in metric at key performance points with baseline (five lower limb bypasses). P < 0.05 was considered statistically significant. *P < 0.05. **P < 0.01. ***P < 0.001. ****P < 0.0001. When not indicated, the mean percentage change in metric relative to baseline was deemed non-significant (P ≥ 0.05). LF/HF, low-frequency to high-frequency; SDNN, standard deviation of the normal-normal interval; prox/dist, proximal and distal.
In LLB procedures, for both proximal anastomosis and distal anastomosis, a significant mean percentage increase in LF/HF ratio relative to baseline was observed (proximal anastomosis = 156.80%(34.60%); P < 0.001 and distal anastomosis = 169.30%(37.15%); P < 0.001) (Fig. 3c) and a significant mean percentage decrease in SDNN relative to baseline was observed (proximal anastomosis = −13.97%(2.89%); P < 0.001 and distal anastomosis = −12.94%(2.37%); P < 0.001) (Fig. 3d).
The most difficult steps reported subjectively in 80% of cases were tunnelling and distal anastomosis. Distal anastomosis produced the greatest changes in LF/HF ratio, but not SDNN. Despite subjective reporting, tunnelling was non-significant relative to baseline in both domains (Fig. 3a,b,c,d).
Subjective reports of stress
Of the 17 procedures, 11 were classified as ‘stressed’ and six were classified as ‘non-stressed’ (number of stressed procedures : non-stressed procedures = 3 : 3 for CEAs, 4 : 2 for open AAA repairs, and 4 : 1 LLBs).
A non-significant increase in mean postoperative STAI-6 score was observed in ‘stressed’ CEAs (before surgery = 8.00(2.65) and after surgery = 10.00(2.65); P = 0.407; three CEAs). A non-significant decrease was observed in ‘non-stressed’ CEAs (before surgery = 11.00(0.00) and after surgery = 10.67(0.58); P = 0.375; three CEAs). The mean difficulty score for ‘stressed’ CEAs increased non-significantly after surgery (before surgery = 6.33(3.22) and after surgery = 7.67(2.89); P = 0.621; three CEAs). In ‘non-stressed’ CEAs, the mean difficulty score was unchanged (before surgery = 7.00(1.00) and after surgery = 7.00(1.00); P > 0.999; three CEAs). The most difficult step reported subjectively was shunt insertion, which was cited in all six cases (100%).
A significant increase in mean postoperative STAI-6 score was observed in ‘stressed’ open AAA repairs (before surgery = 9.75(1.71) and after surgery = 12.50(1.00); P = 0.032; four open AAA repairs). There was no change in postoperative STAI-6 score in ‘non-stressed’ repairs (before surgery = 8.50(3.54) and after surgery = 8.50(3.54); P > 0.999; two open AAA repairs). The mean difficulty score for ‘stressed’ repairs increased non-significantly after surgery (before surgery = 7.50(2.08) and after surgery = 9.00(1.16); P = 0.254; four open AAA repairs). The mean difficulty score was unchanged in ‘non-stressed’ repairs (before surgery = 7.00(1.41) and after surgery = 7.00(1.41); P > 0.999; two open AAA repairs). The most difficult steps reported subjectively were aortic clamping and proximal graft anastomosis, which were both cited in five cases (83%).
A non-significant increase in mean postoperative STAI-6 score was observed in ‘stressed’ LLBs (before surgery = 9.50(2.89) and after surgery = 11.25(2.50); P = 0.395; four LLBs). In the one ‘non-stressed’ LLB, the postoperative STAI-6 score decreased from ten to nine. The mean difficulty score for ‘stressed’ LLBs increased significantly after surgery (before surgery = 6.00(1.63) and after surgery = 8.00(0.00); P = 0.050; 4 LLBs). The difficulty score in the one ‘non-stressed’ LLB decreased from seven to five. The most difficult steps reported subjectively were distal anastomosis and tunnelling, which were both cited in four cases (80%).
Subjective stress and heart rate variability
There was a significant percentage increase in mean intraoperative LF/HF ratio relative to baseline in ‘stressed’ versus ‘non-stressed’ procedures (stressed = 124.00%(12.67%) and non-stressed = 77.33%(15.73%); P = 0.040) (Fig. 4a). There was also a significant percentage decrease in mean intraoperative SDNN relative to baseline between the two groups (stressed = −9.94%(1.23%) and non-stressed = −6.18(0.63%); P = 0.049) (Fig. 4b).
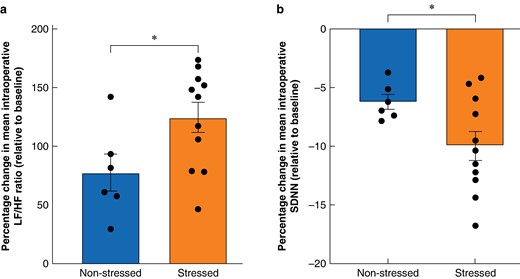
Percentage changes in mean intraoperative heart rate variability metrics relative to baseline depending on procedure classification
a Percentage change in mean intraoperative low-frequency to high-frequency ratio relative to baseline across all three procedures depending on procedure classification. A greater percentage increase represents greater stress. Points represent the percentage change in mean intraoperative low-frequency to high-frequency ratio relative to baseline for individual procedures. Bars represent the mean percentage change in mean intraoperative low-frequency to high-frequency ratio relative to baseline; error bars represent s.e.m. b Percentage change in mean intraoperative standard deviation of the normal-normal interval relative to baseline across all three procedures depending on procedure classification. A greater percentage decrease represents greater stress. Points represent the percentage change in mean intraoperative standard deviation of the normal-normal interval relative to baseline for individual procedures. Bars represent the mean percentage change in mean intraoperative standard deviation of the normal-normal interval relative to baseline; error bars represent s.e.m. An unpaired t test was used to compare the percentage change in mean intraoperative heart rate variability metric depending on classification (six non-stressed procedures and 11 stressed procedures). P < 0.05 was considered statistically significant. *P < 0.05. LF/HF, low-frequency to high-frequency; SDNN, standard deviation of the normal-normal interval.
The relationship between the percentage change in mean intraoperative LF/HF ratio relative to baseline and the percentage change in STAI-6 score demonstrated a significant strong positive correlation (r = 0.768, P < 0.001) (Fig. 5a). A significant strong negative correlation was observed when correlating the percentage change in mean intraoperative SDNN relative to baseline and the percentage change in STAI-6 score (r = −0.775, P < 0.001) (Fig. 5b).
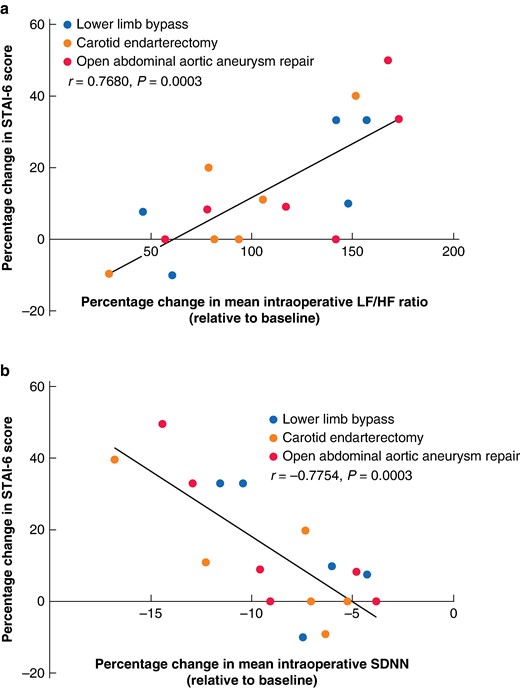
Correlation between the percentage change in State-Trait Anxiety Inventory-6 score (after surgery compared with before surgery) and the percentage changes in mean intraoperative heart rate variability metrics
a Correlation between the percentage change in State-Trait Anxiety Inventory-6 score (after surgery compared with before surgery) and the percentage change in mean intraoperative low-frequency to high-frequency ratio relative to baseline. A greater percentage increase in State-Trait Anxiety Inventory-6 score and a greater percentage increase in mean low-frequency to high-frequency ratio relative to baseline indicate greater stress. Points represent different procedures. A significant strong positive correlation between the parameters can be observed. b Correlation between the percentage change in State-Trait Anxiety Inventory-6 score (after surgery compared with before surgery) and the percentage change in mean intraoperative standard deviation of the normal-normal interval relative to baseline. A greater percentage increase in State-Trait Anxiety Inventory-6 score and a greater percentage decrease in mean standard deviation of the normal-normal interval relative to baseline indicate greater stress. Points represent different procedures. A significant strong negative correlation between the parameters can be observed. Pearson’s correlation coefficient was determined to assess the correlation between the percentage change in mean intraoperative heart rate variability metrics and the percentage change in State-Trait Anxiety Inventory-6 score (17 procedures). P < 0.05 was considered statistically significant. STAI, State-Trait Anxiety Inventory; LF/HF, low-frequency to high-frequency; SDNN, standard deviation of the normal-normal interval.
Discussion
This study has demonstrated significant changes in HRV metrics at key performance points in frequency (LF/HF ratio) and time (SDNN) domains during vascular surgery. Peaks in stress were generally consistent across surgeons performing the same procedure. Additionally, a significant correlation between HRV metrics indicating greater stress and subjective reports of procedural difficulty, and subjective reported measures of a surgeon’s stress, was observed.
Stress is routinely experienced by surgeons. An appropriate level of stress whilst operating may improve surgical performance; however, a rise in stress above the constructive threshold may impair technical ability and decision-making44,45. Striking the balance to create a theatre environment conducive to maximizing surgical performance is critical6. Despite this, identification of intraoperative stress within vascular surgery is a relatively underexplored field. Given its high pressure and performance-critical nature, it was deemed essential to evaluate the feasibility of using HRV to identify a surgeon’s stress. Successful validation may allow for interventional strategies that reduce the impact of dysregulated stress and instead achieve optimal levels that augment surgical performance as described by the Yerkes–Dodson law46.
The findings of the present study related to greater changes in HRV metrics at key performance points support those previously observed within the immediate context of surgery and in other high-stakes professions. Within surgery, these results directly corroborate previous reports detailing the psychological and physiological impact of the theatre environment on surgeons at points of increased cognitive load2,14,18–20,47. Moreover, the objective data identifying stress at procedural stages associated with increased cognitive load are reasonable considering the inherent operative challenge posed by the steps identified. For instance, HRV data identifying shunt insertion as the most stressful point of a CEA is entirely plausible. Shunt insertion is technically intricate, limits perfusion to the brain, and is associated with an increased risk of stroke48. This allows more confidence in the HRV data. The HRV data have accurately identified a procedural step with significant risk to patient safety that could have reasonably induced a spike in stress in the surgeon.
Outside of surgery, HRV has been used in military aviation to assist in training fighter pilots49. The published results revealed that HRV metrics were sensitive to fluctuation in task demand, a finding similar to what the present study and others have observed at key surgical performance points14,21,24. Findings further demonstrated that HRV could accurately distinguish a level of cognitive load at which pilots could not cope with increasing task demand and, as such, technical performance declined49. Whilst it was not possible to determine a point of cognitive overload, a finding in a similarly demanding profession indicates the potential for HRV to identify intervals of concern accurately. When applied to vascular surgery, this could allow for timely intervention in theatre when a point of cognitive overload is reached (for example via a recovery interval to relax/brief before an upcoming difficult step) and ensure that surgical performance is not compromised throughout the rest of the procedure. Similarly, the findings of the present study related to changes in HRV metrics during unplanned procedural disruptions (for example patient asystole and repeated anaesthetist interruptions) may be critical in supporting the use of HRV as a marker of stress to sustain operative performance. Although anecdotal, these findings display the capability for HRV metrics to fluctuate acutely. This is likely to be beneficial when considering the fast-paced nature of vascular surgery and may also be exploited to identify momentary HRV changes that may be concerning.
The observations of the present study identifying a correlation between self-reported stress levels via the STAI-6 and objective HRV measurements closely align with previous work assessing stress amongst students undertaking examinations50. These findings also corroborate those previously identified in colorectal surgery, where an increased intraoperative LF/HF ratio corresponded with greater subjective stress reports17. The correlation described in Jones et al.17 is similar to the findings of the present study, despite the present study having a smaller number of total procedures and heterogeneity in the operation type involved. Regardless, these findings indicate that STAI-6 may be used with HRV to identify intraoperative stress effectively within this context. Moreover, the results demonstrating an association between subjective reporting of the most difficult step and the greatest change in HRV provide preliminary evidence that validates the use of HRV in identifying cognitive load in vascular surgery. They also evidence that HRV metrics may be used to reliably indicate critical areas of mental strain across a full spectrum of vascular procedures, meaning the data are likely robust. Interestingly, operations where the subjectively reported most difficult step did not align with objective HRV data tended to be operations in which surgeons displayed lower intraoperative stress. This suggests that surgeons may only perceive more extreme changes in stress. However, the full significance of this result remains unclear until a more thorough delineation of the relationship between stress and surgical performance is revealed.
Limitations include a relatively small sample size, which may have led to overestimations regarding the magnitude of associations. Previous HRV effect size distribution analyses have indicated that, to achieve 80% power, a sample of 21 is required to detect large effect sizes27. Therefore, further observations for each of the procedures are required. Additionally, although many reports cite the reliability of HRV, there remains uncertainty surrounding the potential for varying levels of physical factors (for example exercise) and social factors (for example intake of nicotine, alcohol, and caffeine) to confound HRV metrics27. Likewise, recent evidence has suggested differences in HRV between sexes51. The present data were normalized by analysing the percentage change in HRV metrics from individual baselines to account for this. However, more data would allow for multivariate regression to adjust for such confounders/perform subgroup analyses. This model could also account for varying operation length and procedural difficulty. Furthermore, there is an argument to suggest that baseline recordings themselves could have been non-representative of true values as surgeons were likely not fully relaxed at the time of recording. This could be solved using overnight ambulatory recording to obtain a more representative baseline. It was also not possible to report intraoperative measures of subjective stress without interrupting the procedure and perhaps inducing additional stress. Thus, the reliability of retrospective reports of stressful performance points is reduced. Consideration must also be given to the impact of the observer effect and its potential to induce non-representative HRV changes.
Future studies should assess the efficacy of an intervention (for example a timeout before a stressful step) in controlling the theatre environment or reducing stress at key time points, by comparing interventional HRV data with the findings of the present study. This would serve as the first meaningful step that transitions HRV data into tangible improvement in the operating theatre. Additionally, the impact of psychological stress versus stress solely associated with cognitive load could be explored further to ensure a suitable metric is monitored at the relevant operative step so that surgeons are appropriately alerted to deranged HRV. The proposed benefits of this are shown by the open AAA repair data, which demonstrate that both of the subjectively reported most difficult steps produced the greatest changes in different HRV metrics (aortic clamping in the LF/HF ratio and proximal graft anastomosis in the SDNN). Thus, determining a relationship between an HRV metric and the type of stress experienced is an important avenue for future research to ensure adequate monitoring of intraoperative stress. Finally, an assessment of the relationship between deranged HRV and the subsequent impact on surgical performance could be performed. This could incorporate objective structured assessment of technical skill (OSATS)52 and non-technical (NOTECH)53 measures to objectify if stress identified by HRV at key vascular performance points corresponds to a cognitive overload-induced reduction in technical ability, as already evidenced elsewhere49. A greater understanding of HRV and its association with cognitive overload and deterioration in surgical performance would help to augment outcomes for surgeons and patients by achieving the optimal level of stress in the operating theatre.
HRV metrics and subjective stress reports may present a feasible method to assess intraoperative stress and cognitive load in key areas of procedural difficulty during vascular surgery. When HRV metrics surpass acceptable levels, an opportunity may be provided to implement preventative strategies that reduce stress levels in surgeons to benefit their health and patient safety.
Funding
The authors have no funding to declare.
Acknowledgements
The authors wish to thank all the vascular surgeons at St Mary’s Hospital, Imperial College Healthcare NHS Trust, for their participation in this study. This work was supported by the National Institute for Health Research (NIHR) as part of a doctoral fellowship and by the NIHR Biomedical Research Centre based at Imperial College Healthcare NHS Trust. A partial set of STAI-Ad items (STAI-6) was used with the permission of the publisher.
Author contributions
Calvin D. De Louche (Conceptualization, Data curation, Formal analysis, Investigation, Methodology, Resources, Visualization, Writing—original draft, Writing—review & editing), Manish Mandal (Conceptualization, Data curation, Investigation, Methodology, Writing—review & editing), Lee Fernandes (Conceptualization, Investigation, Methodology, Writing—review & editing), Jason Lawson (Conceptualization, Investigation, Methodology, Project administration, Supervision, Writing—review & editing), Colin D. Bicknell (Conceptualization, Investigation, Methodology, Project administration, Resources, Supervision, Writing—review & editing), and Anna L. Pouncey (Conceptualization, Data curation, Investigation, Methodology, Project administration, Resources, Supervision, Writing—review & editing)
Disclosure
The authors declare no conflict of interest.
Supplementary material
Supplementary material is available at BJS Open online.
Data availability
The data sets used and/or analysed during the present study are available from the corresponding author on reasonable request.
References
Author notes
Elements of this work were delivered as an oral presentation to the annual conference of the Association of Surgeons in Training (ASiT), Liverpool, UK, March 2023; published in abstract form as Br J Surg 2023; 110(Suppl 7): znad258.004