-
PDF
- Split View
-
Views
-
Cite
Cite
David N Fisher, Jonathan N Pruitt, Justin Yeager, Orb-weaving spiders show a correlated syndrome of morphology and web structure in the wild, Biological Journal of the Linnean Society, Volume 131, Issue 2, October 2020, Pages 449–463, https://doi.org/10.1093/biolinnean/blaa104
- Share Icon Share
Abstract
Extended phenotypes are traits that exist outside the physical body of organisms. Despite their role in the lives of the organisms that express them and other organisms influenced by extended phenotypes, the consistency and covariance with morphological and behavioural traits of extended phenotypes has rarely been evaluated. We repeatedly measured an extended phenotype involved in prey acquisition (web structure) of wild orb-weaving spiders (Micrathena vigorsii), which re-build their webs daily. We related web structure to behaviours and spider body length. Web diameter and web density were repeatable among individuals, reaction to a predation threat was very marginally so, and response to a prey stimulus and web evenness were not repeatable. Larger spiders spun wider webs, had webs with increased thread spacing, and the spider possibly tended to react more slowly to a predation threat. When a spider built a relatively larger web it was also a relatively less dense and less even web. The repeatability of web construction and relationship with spider body size we found may be common features of intra-population variation in web structure in spiders. By estimating the consistency and covariances of extended phenotypes we can begin to evaluate what maintains their variation and how they might evolve.
INTRODUCTION
Some phenotypes of organisms are “extended”, in that they exist beyond the physical body of the organism (Dawkins, 1978, 1982). Examples include bird nests, beaver dams, and spider webs. Extended phenotypes can relate to the survival, foraging or mating success of an individual, and those that use the environment the individual modifies (Jones et al., 1994, 1997, 2009; Rosell et al., 2005; Kooch & Jalilvand, 2008; White & O’Donnell, 2010; Ransom, 2011; Posthumus et al., 2015; Ringler et al., 2015; Fisher et al., 2019). When extended phenotypes are repeatably expressed over the life of an organism, they have the potential to show reversible plasticity, for example a bird may build a large nest one year and a small nest the following year. Despite this plasticity, traits with the potential to be labile are often consistent within an individual across time or in different environments, such that within a population there can be considerable variation among individuals in the mean trait they express (Bell et al., 2009). Causes and consequences for this consistent among-individual variation has been the source of great interest, especially within the last 20 years (Koolhaas et al., 1999; Dall et al., 2004; Réale et al., 2007; Bell et al., 2009; Stamps & Groothuis, 2010). However, among-individual variation in extended phenotypes is not extensively documented (Dirienzo & Montiglio, 2016a; Montiglio & DiRienzo, 2016). Furthermore, the expression of extended phenotypes may covary with other repeatably expressed traits such as behaviours, or more stable traits such as morphology, but again this is not often documented (Dirienzo & Montiglio, 2016a; Montiglio & DiRienzo, 2016). Yet it is important that we do so as extended phenotypes alter the environment an individual experiences, possibly changing selection pressures both for itself and for other organisms that use the same environment.
Evaluating the repeatability of extended phenotypes and their associations with other aspects of behaviour and morphology has a variety of implications. First, as only a portion of any among-individual variation will have a genetic basis, repeatability can set the upper limit for heritability (Falconer, 1981; Boake, 1989; but see Dohm, 2002), and repeatability in behaviour has various consequences for ecological and evolutionary processes (Dall et al., 2012; Sih et al., 2012; Wolf & Weissing, 2012). Meanwhile, extended phenotypes being correlated into “syndromes” (sets of correlated traits expressed within or between given contexts) with traits such as body size or boldness could help explain the maintenance of variation in extended phenotypes within populations (Roff, 1992; Sih et al., 2004a, b). Further, trait associations can influence how syndrome structure (i.e. the G-matrix) and its constituent traits evolve (Lande, 1979; Lande & Arnold, 1983), which can sometimes impinge on adaptive evolution (Dochtermann & Dingemanse, 2013; Royauté et al., 2020). The repeatability of extended phenotypes and their phenotypic integration with other traits is especially interesting because extended phenotypes help to engineer the external environment surrounding an organism, and therefore how selection acts on other traits. It is therefore necessary that we quantify the repeatability of extended phenotypes and assess how they are correlated with other traits.
Here we quantify the degree of consistency of various aspects of an extended phenotype and measure its covariance with body size and two behavioural traits. We studied the orb-weaving spider Micrathena vigorsi (Perty, 1833) (Araneae: Araneidae; Fig. 1A). Although spider webs were once thought to rigidly follow species specific patterns, more recent research has identified both among- and within-individual variation in web structure, such as differences in size, shape, symmetry, the number of different kinds of threads/lines, physical properties of web strands, and the number of mistakes made in the web’s construction (Sherman, 1994; Heiling & Herberstein, 2000; Venner et al., 2000; Benjamin & Zschokke, 2003; Blamires et al., 2007; Blamires, 2010; Blamires et al., 2011; Zschokke & Nakata, 2015; Dirienzo & Montiglio, 2016a; Montiglio & DiRienzo, 2016; Blamires et al., 2017a, b, c). See Heiling and Herberstein (2000) for a review. Studies on various different spider species have shown that variation in web structure can influence which and how many prey are caught (Uetz et al., 1978; Chacon & Eberhard, 1980; Sensenig et al., 2010), whether prey are retained (Blackledge & Zevenbergen, 2006; Blamires et al., 2017c), and, in cob-web building species, how much protection the web provides from predators (Zevenbergen et al., 2008). Web structure may also reflect a spider’s body condition (Blackledge & Zevenbergen, 2007; Dirienzo & Montiglio, 2016b), recent experience (Nakata & Ushimaru, 1999; Venner et al., 2000), and/or age (Anotaux et al., 2012, 2014). Spider web structure is therefore an important phenotype for various components of a spider’s fitness.
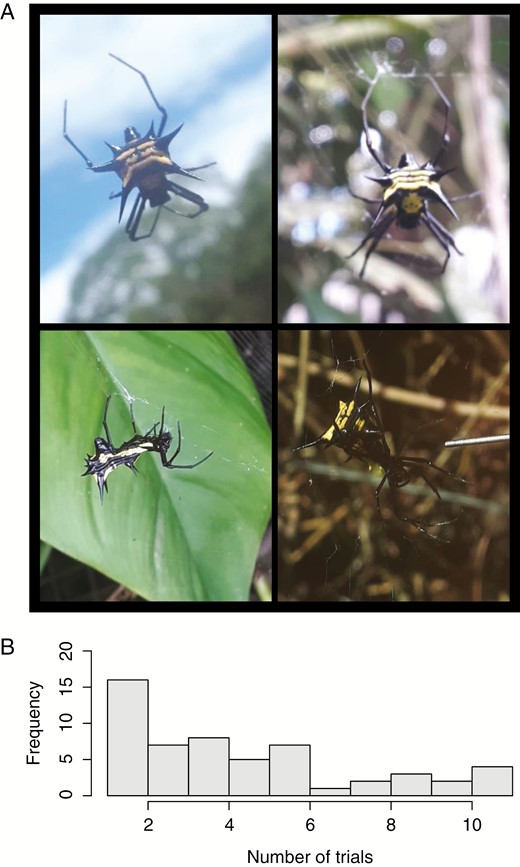
A, photographs of Micrathena vigorsii (taken by D.N.F.). B, histogram of the number of times individuals were tested for web structure.
Micrathena spp. replace their web daily (Shelly, 1984; Hodge, 1987), and so allow one to easily measure the repeated expression of an extended phenotype and determine if individuals consistently differ from each other over time. It also allows us to see if there is day-to-day variation in web structure, if for example certain environmental conditions consistently influence web structure for all individuals. We predicted that larger spiders will build bigger and less dense webs (webs with fewer threads per area of the web), as webs with larger spacing between threads can catch larger prey (Uetz et al., 1978; Chacon & Eberhard, 1980), and that larger spiders will more aggressively attack a prey stimulus and will react less readily towards a predator stimulus (Rundle & Brönmark, 2001; Mayer et al., 2016). We also predicted that there will be a positive among-date covariance between responses to predator and prey stimuli, as on hotter days spiders might be more responsive in general to a range of different cues (Briffa et al., 2013). Finally, we predicted a negative residual covariance between web diameter and both web density and web evenness (the variance in counts of spiral threads in four directions: up, down, left, and right; higher values indicate a less even web), as in instances where a spider builds a relatively bigger web, it may do this by spacing threads further apart, giving a less dense web (Blamires, 2010) and it may be more challenging to maintain consistency in larger webs, giving a less even web. We do not expect a relationship here between web density and anti-predator behaviour (e.g. Blackledge & Zevenbergen, 2007) as M. vigorsii do not use their web for defence.
Previous studies on among-individual consistency in web structure have been conducted in captivity (Dirienzo & Montiglio, 2016a,b; Montiglio & DiRienzo, 2016; DiRienzo & Dornhaus, 2017; DiRienzo & Aonuma, 2018); however, our study took place in the natural habitat of M. vigorsii. Conducting the study in the wild allowed us to assess the degree of consistency of behaviour and web structure, and how they covary with each other and with spider body size, in a completely natural setting. Studies in standardized laboratory settings are useful when we want to control for external factors, but studies in free-living populations help us assess the degree of consistency and correlations between traits that natural selection may be acting upon (Blamires et al., 2007). Conducting studies in the wild also avoids the potential confound that individual differences in phenotypes can be driven by individual differences in response to laboratory conditions (Roche et al., 2016).
We estimated the phenotypic correlations between spider predatory and anti-predator behaviour, web structure traits, and body length. We then partitioned our estimated covariances into the among-individual, among-date, and residual levels. We investigated covariances among six traits, all at the among-individual, among-date, and residual level (with some exceptions, see below), for a total of 45 covariances. For the sake of brevity we do not make predictions for each covariance.
MATERIAL & METHODS
Data collection
Our study took place between 16/6/19 and 15/7/19 near Tena in Ecuador (~ lat., long. = -1.044, -77.715), under the Ecuadorian Ministry of the Environment permit no. 014-2019-IC-FLO-DNB/MA. We located individual M. vigorsii (N = 55) in a hedgerow along a transect at the northern side of a road (route number: 436) between 9 and 121 cm above the ground (mean = 59 cm). We did not confirm the sex of the studied individuals, but assumed they were most likely females, as males of Micrathena spp. are much smaller than females and stop building webs upon reaching maturity (Chickering, 1961; Shelly, 1984; Hodge, 1987). The time of year we conducted our study was towards the end of this species’ season (see: https://www.inaturalist.org/taxa/512844-Micrathena-vigorsi), which suggests most individuals were mature adults. Once we found an individual, we marked its location with a piece of flagging tape with the unique ID of the spider written on it. This allowed us to return and phenotype the same individual on different days without disrupting the individual’s behaviour by capturing it and marking it directly. Although Micrathena spp. do rebuild their orb each day, the “frame” of the web remains in place, and so the position of the web will vary minimally day-to-day. When two individuals were close together, we used their size measurements and notes on individual characteristics such as coloration and exact web location to identify them. We are therefore confident that we were able to repeatably find and identify the same individuals. We only conducted testing between 14:00-17:00, after web construction should have been completed, limiting the impact the time of day could have on variation in behaviour and web structure.
The first time we found an individual, we measured its length (to the nearest 0.01 mm) using a pair of callipers (Traceable, Fisher Scientific, PA, USA) held up against the spider in its web. In 10/55 of cases (18.2%; mostly at the start of the study before the callipers were available) we did not measure the length of the individual. Following this, we tested the individual for responsiveness towards a prey stimulus. To do this, we touched a piece of wire attached to a modified vibrating device (see Dirienzo & Montiglio, 2016a; Lichtenstein et al., 2019) to the lowest point of the orb of the web. Assays like this are commonly used to estimate foraging aggression in both solitary and social spiders. In some cases, the spider fled on approach before the trial could begin (see below for how we dealt with this). If the spider remained on the web, we timed from the start of the vibrations until the spider touched the end of the vibrating wire. If the spider did not respond within 180 s (in 55/210 tests the spider did not flee before the trial began) we set the spider’s score at 180. Next, we waited for the spider to return to the centre of the web and adopt a resting position (facing down towards the floor), before testing it for a reaction to a predation threat. We tapped the abdomen of the spider lightly with the extended lead of a mechanical pencil and recorded how many taps transpired until the spider fled. This assay is somewhat similar to assays using air puffs to simulate an approaching avian predator in studies on other orb-weaving spiders (Jones et al., 2011; Watts et al., 2014). If the spider did not react within 24 taps (in 26/210 tests the spider did not flee before the trial began), we set the spider’s score at 24. These tests gave us measures of two behaviours: responsiveness to prey and reaction to a predator threat.
To quantify web structure, we counted the number of “radii” of the orb (strands extending from the centre outwards), and the number of “spirals” (strands perpendicular to the radii circling the centre) extending up, down, left and right from the centre of the web to its perimeter, giving four separate counts (Sensenig et al., 2010). We then measured the diameter of the web (to the nearest 1 cm) from side to side. We calculated web density as the number of radii multiplied by the mean number of spirals, divided by the web area (assuming a circular shape). Radii and spiral threads are made from different kinds of silk, and perform different roles in an orb web (Eberhard, 1990). However, we did not use them as different traits as the number of radii per cm2 was strongly and positively correlated with the mean number of spirals per cm2 (r = 0.805). Unfortunately, at the time of data collection we were not aware of Herberstein and Tso’s work, which indicates an ellipse is preferable to a circle for estimating web area [(Herberstein & Tso, 2000), although assuming a circle still gives reasonable approximations of true capture area; see also: Venner et al. (2001) for estimating the total capture thread length]. We calculated web evenness as the variance among the four counts of the number of spirals; for this measure lower values indicate a more even web (the correlation between this value and the coefficient of variation in spiral counts was 0.936). The time of day of our study (14:00-17:00) means that we did not necessarily measure the web as the spider constructed it, but only after it may have been damaged by contact with predators and/or prey. We did not want to assume that a spider initially made a web with exactly the same number of spirals in each direction, and so we did not actively avoid counting strands in areas of the web with gaps. We discuss the effect this decision may have had on our results in the Discussion. Orb-weaving spiders often build webs with up-down asymmetry; the bottom may be larger than the top (Zschokke & Nakata, 2015). We observed this in our study population; although we did not measure the radius of the web upwards or downwards, our counts of radii were higher downwards (mean = 28.4) than upwards (mean = 25.0). Therefore, our measure of web evenness would have included variation due to this tendency as well as any other variation in the counts of the spirals.
We returned to our transect regularly (but not daily due to other field work commitments or heavy rainfall), to locate and measure new individuals and re-measure previously marked individuals. The study took place over 29 days, of which we collected data on 17 of them, giving an average of a visit every 1.7 days. Not all individuals were located at the same time and so were not measured on the same days. When re-measuring individuals, we performed both behavioural tests and measures of the web described above; however, we did not re-measure spider length, as we assumed it was relatively invariant at the time scale we were working. Individuals’ web structures and behaviours were measured on average of 4.75 times (range 1–11; Fig. 1B), across on average 6.64 days (range = 1–23). If we could not see an individual, we removed the flagging type and recorded it as no longer present. If an individual was found but had spun no orb that day, we did not measure its behaviour or its web structure, but also did not remove the flagging tape, allowing us to return and identify the individual.
If an individual fled as we approached it, we measured its web structure and then left, returning later in the session to attempt to measure its behaviour. If we could not measure its behaviour that day, we recorded “NA” for both responsiveness to prey and reaction to a predation threat. If the spider fled from its web during the test for responsiveness to prey, we scored its responsiveness to prey as 180, and its reaction to a predation threat as 1. Our reasoning here is that a spider fleeing a potential prey item was both unresponsive to the opportunity and unwilling to face any potential predation risk. In five instances we re-tested such spiders once they had returned to the centre of the web, and in all cases they either fled again, or did not respond to the prey stimulus within 180 seconds, justifying our decision. We also tested whether these modelling decisions influenced our results, see Robustness to modelling decisions. In total we recorded 45 measures of length, 188 measures of responsiveness to a prey stimulus (146 of which were directly observed rather than assigned due to fleeing), 194 measures of reaction to a predation threat (146 of which were directly observed rather than assigned due to fleeing), 200 diameters, and 197 measures of web density and evenness across 55 unique individuals (see Table 1 for means and variance of each trait).
The means and variances of each of the six traits (to three significant figures). Note that web evenness was log-transformed prior to analysis, while this logged variable, body length, web diameter and web evenness were mean centred and variance standardized prior to being entered into the analysis
Trait . | Mean . | Variance . |
---|---|---|
Body length (mm) | 13.5 | 5.22 |
Responsiveness to prey (seconds) | 117 | 5300 |
Reaction to predator stimulus (taps) | 7.54 | 66.9 |
Web diameter (cm) | 19.4 | 16.1 |
Web density (threads/cm2) | 3.90 | 2.48 |
Web evenness (variance) | 32.1 | 1210 |
Trait . | Mean . | Variance . |
---|---|---|
Body length (mm) | 13.5 | 5.22 |
Responsiveness to prey (seconds) | 117 | 5300 |
Reaction to predator stimulus (taps) | 7.54 | 66.9 |
Web diameter (cm) | 19.4 | 16.1 |
Web density (threads/cm2) | 3.90 | 2.48 |
Web evenness (variance) | 32.1 | 1210 |
The means and variances of each of the six traits (to three significant figures). Note that web evenness was log-transformed prior to analysis, while this logged variable, body length, web diameter and web evenness were mean centred and variance standardized prior to being entered into the analysis
Trait . | Mean . | Variance . |
---|---|---|
Body length (mm) | 13.5 | 5.22 |
Responsiveness to prey (seconds) | 117 | 5300 |
Reaction to predator stimulus (taps) | 7.54 | 66.9 |
Web diameter (cm) | 19.4 | 16.1 |
Web density (threads/cm2) | 3.90 | 2.48 |
Web evenness (variance) | 32.1 | 1210 |
Trait . | Mean . | Variance . |
---|---|---|
Body length (mm) | 13.5 | 5.22 |
Responsiveness to prey (seconds) | 117 | 5300 |
Reaction to predator stimulus (taps) | 7.54 | 66.9 |
Web diameter (cm) | 19.4 | 16.1 |
Web density (threads/cm2) | 3.90 | 2.48 |
Web evenness (variance) | 32.1 | 1210 |
Data analysis
We first estimated the phenotypic correlations between each trait pair (Pearson’s correlations; Fig. 2). To partition these correlations to the among-individual, among-date, and residual levels, we built a multivariate mixed model with each of the six traits as response variables. Responsiveness to prey and reaction to predation used a Poisson error distribution (log-link), while each web structure trait and body length used a Gaussian error distribution. Web evenness was log-transformed, and then this transformed variable, web diameter, web density, and body length were mean centred and variance standardized (Schielzeth, 2010). We fitted the random effect of individual identity and estimated the among-individual covariances among all six traits. We fitted the random effect of date and estimated the among-date covariances among all traits except for length, which was only measured on a single day per spider, and so we fixed the among-date variance to 0.0001. We estimated the residual covariances between all traits, and also fixed the residual variance for length to 0.0001, as it is only measured once per individual, following Houslay and Wilson (2017). We estimated unique intercepts for each response variable. Spiders might adjust their web structure or foraging behaviour over time as they gain information about their foraging patch (Nakata & Ushimaru, 1999). Therefore, we fitted trial number (mean centred) as a fixed effect for each trait except length (which was only measured once). The model was fitted in R (v.3.5.3; R Development Core Team 2016) with the package “MCMCglmm” (Hadfield, 2010). We used 550 000 iterations, a burn-in of 50 000, and a thinning interval of 100. Priors were set to be flat and relatively uninformative, with 70% of the phenotypic variance for the logged values of each trait placed on the residual variance, 20% on the among-individual variance, and 10% on the among-date variance, following Brommer (2017). We calculated adjusted repeatabilities (after accounting for trial number) following Nakagawa and Schielzeth (2010). Note that individuals only measured once were still included, as they contribute to the estimation of the some model parameters, and excluding them could bias results if these individuals are not a random sample of the population (Martin et al., 2011).
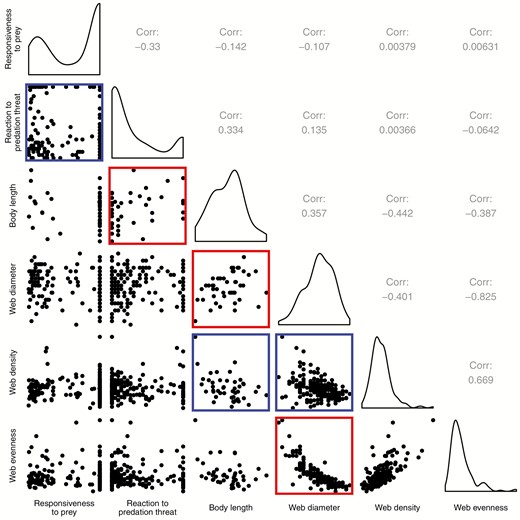
Phenotypic relationships between each of our six traits (web evenness has been log-transformed). Pearson’s correlations are shown above the diagonal, pairwise plots below the diagonal, and histograms of each variable along the diagonal. Pairwise plots are bordered with red if the correlation was significant and positive, or bordered with blue if significant and negative.
Robustness to modelling decisions
We repeated the analysis with all behavioural scores that were assigned when an individual fled rather than were directly observed set to “NA”. We also repeated this analysis with all behavioural scores that were ceiling values (180 for responsiveness to prey, 24 for reaction to a predation threat) set to “NA”. In each case our results did not change (full results of the original model are given in Supporting Information, Table S1, with these auxiliary results shown in Tables S2, S3). We therefore concluded that assigning behavioural scores to spiders that fled and giving unresponsive spiders the maximum score for each behaviour had not biased our results. We also repeated the original analysis without estimating any among-date covariances [as none were different from zero; see Supporting Information (Table S1; Fig. S1)]. This too did not change our results (Supporting Information, Table S4), indicating that estimating the among-date covariances had not reduced our power and prevented us from detecting any other among-individual or residual covariances. As such we discuss the results as from the model where the among-date covariances were estimated.
Data accessibility
The R code used in the analysis and all data are available as Supporting Information (Tables S4; Appendix S1).
RESULTS
Repeatabilities
Web diameter was repeatable [r = 0.427, credible intervals (CIs) = 0.239 to 0.587], as was web density (r = 0.488, CIs = 0.286 to 0.646). The response to the predator stimulus was very slightly repeatable (r = 0.045, CIs = < 0.001 to 0.159). Web evenness was not repeatable (r < 0.001, CIs < 0.001 to 0.145), nor was response to the prey stimulus (r < 0.001, CIs < 0.001 to 0.012). Estimates for each of the among-individual, among-date and residual variances for each trait are shown in Fig. 3. Individuals tended to react more quickly to the predation threat in later trials, although this effect marginally overlapped with zero (fixed effect of trial, mode = -0.117, CIs = -0.252 to 0.011). Trial number did not influence any other trait.
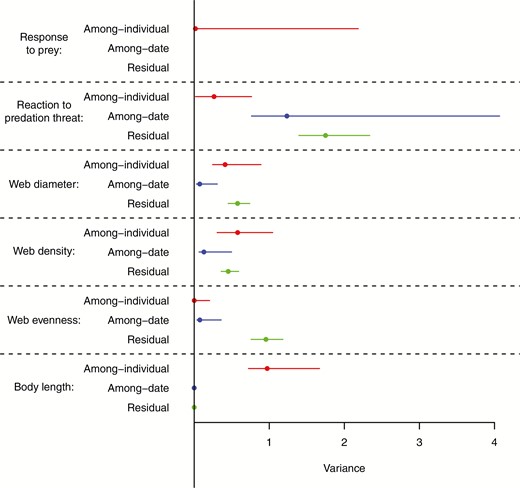
Variance in each trait partitioned to the among-individual (red), among-date (blue) or residual (green) levels. The among date variance for response to the prey stimulus was 87, while the residual variance was 44, and so are not shown on this plot. Both the among-date and residual variance for length were supressed to 0.0001.
Covariances
Body length, web diameter and web density were associated into a syndrome at the among-individual level, such that longer spiders had wider and less dense webs (body length-web diameter correlation = 0.404, CIs = 0.130 to 0.708; body length-web density correlation = -0.608, CIs = -0.769 to -0.305, web diameter-web density correlation = -0.689, CIs = -0.865 to -0.309). Reaction to the predation threat tended to be associated with these traits as well; spiders that reacted more slowly to the predation threat had longer bodies, and tended to have wider and less dense webs, but all correlations were quite uncertain, and the latter two of these correlations overlapped with zero (reaction to predation-body length correlation = 0.620, CIs = 0.099 to 0.906; reaction to predation-web diameter correlation = 0.554, CIs = -0.104 to 0.998; reaction to predation-web density correlation = -0.744, CIs = -0.990 to 0.079). Plots of the estimated among-individual relationships are shown in Figure 4, with estimates for the among-individual correlations shown in Figure 5. Response to the prey stimulus and web evenness were not associated with the other traits among individuals (Figs 4-5).
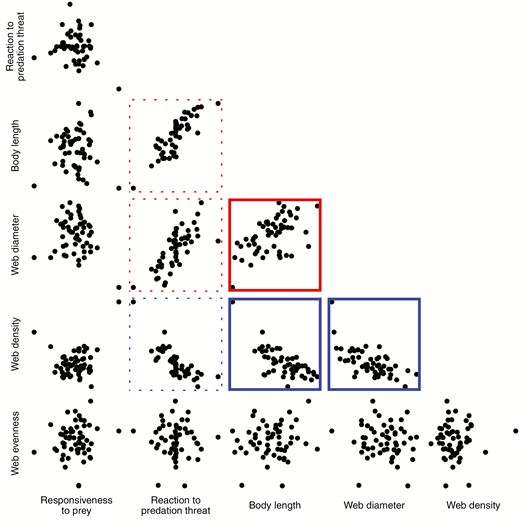
Pairwise plots of the estimated among-individual relationships between the six traits we studied, using best linear unbiased predictors extracted from the multivariate model. If the 95% credible intervals of the correlation did not overlap zero the pairwise plot has a solid border, which is red if the correlation was positive, or blue if the correlation was negative. The 95% credible intervals of the among-individual correlations between reaction to predation and web diameter, web density and body length all marginally overlapped zero, and so the borders are plotted with dashed lines.
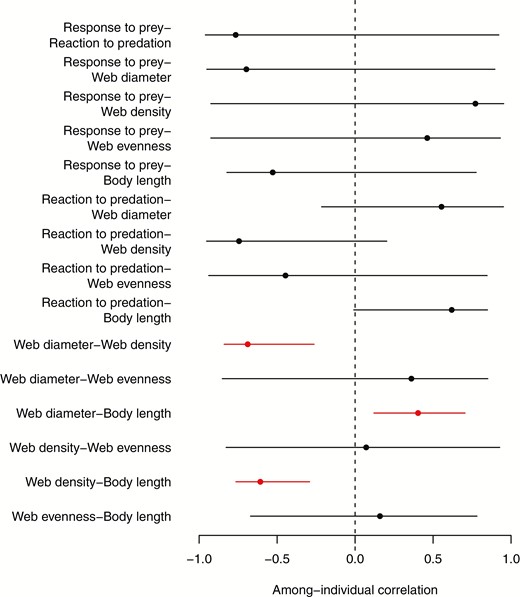
Estimated modes and 95% credible intervals of the among-individual correlations. Correlations that do not overlap zero are highlighted in red.
At the residual level there were associations between web diameter, density and evenness, such that when a spider built a relatively wider web it also built a relatively less even and less dense web (Fig. 6; web diameter-web density correlation = -0.303, CIs = -0.438 to -0.115; web diameter-web evenness correlation = 0.245, CIs = 0.073 to 0.379; web density-web evenness correlation = -0.277, CIs = -0.442 to -0.139). No other residual covariances were different from zero (Fig. 6). There was variation among days for responsiveness to prey, reaction to predation, and somewhat so for web density (Fig. 3), but no among-date covariances were different from zero (Supporting Information, Fig. S1).
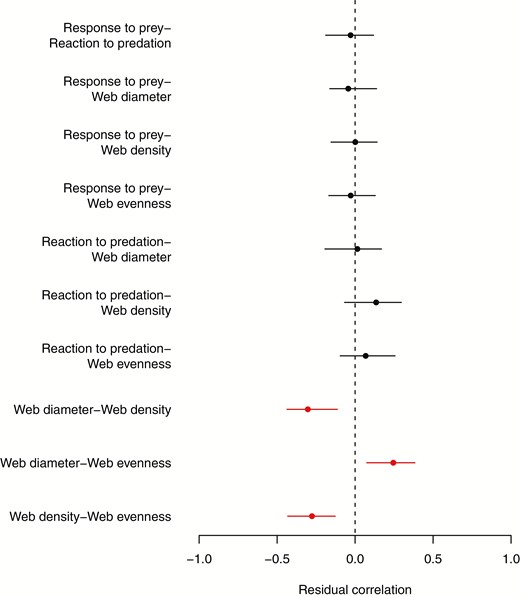
Estimated modes and 95% credible intervals of the residual correlations. Correlations that do not overlap zero are highlighted in red. Note that as the residual variance of body length was suppressed to 0.0001 it cannot covary at the residual level with the other traits, and so is not plotted here.
DISCUSSION
Individuals may show consistent differences in aspects of their behaviour, but whether their extended phenotypes are repeatable and covary with other behavioural traits is not well studied. We found that individual M. vigorsii have consistent differences in their web diameters and web densities. These two web traits are correlated among-individuals into a syndrome with body length, indicating they might represent a single axis of variation. Reaction to predation may be included with the syndrome as well, but the trait was only slightly repeatable and some of the among-individual correlations with other traits overlapped zero. Responsiveness to a prey stimulus and web evenness were not repeatable among-individuals and so were not associated with any traits among individuals.
Discovering a syndrome between web structure traits, morphology, and possibly behaviour suggests they may function together as a single integrated whole (e.g. they are an ‘evolutionary character’; Araya-Ajoy & Dingemanse, 2014). This then raises questions as to the syndrome’s functional role, and the processes maintaining variation in this syndrome among-individuals (Sih et al., 2004a, b; Araya-Ajoy & Dingemanse, 2014). The relationship between body length and web diameter suggests a simple biomechanical relationship between the size of spider (and perhaps the size of the steps it takes) and the size and cell spacing of the resulting web it spins. This may naturally cause a wider web to be less dense, as a similar amount of silk is then spread over a larger area. However, if the relationship between web diameter and density was purely due to silk limitation, we would expect to see this trade-off at each level (among-date and residual) as well as at the among-individual level. Yet, we did not. This hypothesis also does not explain why reaction to a predation threat might be part of the syndrome (albeit our evidence for this is weak). Instead, variation in this size and behaviour syndrome may represent an active strategy by larger individuals to catch larger and more nutritious, but possibly more dangerous, prey.
Orb-webs are thought to be adapted specifically for targeting “large but rare” prey (Venner & Casas, 2005; Blackledge, 2011; Sensenig et al., 2013). This hypothesis has however been questioned, given the possible overestimation of the biomass of “large” prey actually caught by spiders, and the expected availability of such prey (Eberhard, 2013; Harmer et al., 2015). As such there is considerable scope for spiders to vary in the size of prey they may be targeting. The size of the gaps between strands of a web may influence the type or size of prey it captures (Uetz et al., 1978; Chacon & Eberhard, 1980; Blackledge & Zevenbergen, 2006). Meanwhile, larger spiders and those more resistant to predation threats might be willing to tackle larger and more dangerous prey, which smaller and more docile spiders would not risk [(Mukherjee & Heithaus, 2013); although juveniles in some species may be forced to target sub-optimal, and possibly more dangerous, prey (Elbroch et al., 2017)]. If body size and web size do relate to the type of prey captured, variation in the syndrome we have detected here might instead represent variation in individual foraging strategies (Bolnick et al., 2002; Ingram et al., 2018). In which case, we might expect the types and sizes of the prey caught in individual M. vigorsii webs to show consistent among-individual variation; a prediction that requires testing.
To predict how the syndrome might evolve, we need to know the degree to which it is heritable, and whether it is under selection. Around 50% of among-individual variation in behaviours may be based on additive genetic variance (Dochtermann et al., 2015), and phenotypic correlations tend to have the same sign as underlying genetic correlations (Dochtermann, 2011) and so we could expect some of the (co)variation among-individuals in web structure and body length to have a genetic basis. For now, in terms of selection, we do not have any estimates available for M. vigorsii. The different strategies may have equal pay-offs, which would maintain variation even if the syndrome had a genetic basis (Mangel & Stamps, 2001; Stamps, 2007). Alternatively, spiders may change their position in the syndrome as they age, spinning wider and less dense webs (and possibly reacting less to predation) as they grow. For example, in the orb-web spider Zygiella x-notata, webs have a shorter total thread length, become less regular, and have more “anomalies” as individuals age (Anotaux et al., 2012, 2014), and these changes increase prey handling time (Anotaux et al., 2014). We did not detect a decrease in web evenness over time in our study, as it took place at a time scale (1 month, although each individual was tracked for considerably less time than this) where we might not expect to see much measurable aging. Determining whether M. vigorsii move through the morphological and behavioural syndrome as they grow would require a much longer study, and one probably performed in captivity to track individuals over their entire lifetimes.
Some of the consistent variation in web structure we observed could be due to variation in microhabitat, as in our study individuals were only ever assayed in one environment. Some variation in the amount of space a spider had to spin a web, the available structural supports, relative prey availability and diversity, or some microclimatic factor, could cause consistent differences in web structure among-individuals (Blamires et al., 2007; Zevenbergen et al., 2008; Blamires, 2010; Nakata, 2012; Wu et al., 2013). However, DiRienzo and Montiglio (DiRienzo & Montiglio, 2016a; Montiglio & DiRienzo, 2016) also found consistent differences among individuals in spider web structure, but in a laboratory setting. Such a setting should hypothetically control for variation in microhabitat, and so microhabitat variation could not be an explanation for consistent differences in individual web structure in their study; raising the possibility that microhabitat use may not fully explain our results either. Furthermore, a spider may select the microhabitat that allows the spinning of a web of a certain structure [see also ‘niche construction’ (Odling-Smee et al., 2003; Saltz & Nuzhdin, 2014)]. Therefore, while web structure and microhabitat could covary, this could still depend on the spider’s decision making, and so would still be classed as a trait of the spider, not as one driven by the environment.
Our results are generally in agreement with previous work studying among-individual variance in web structure, albeit work which was conducted on cob-web instead of orb-web building spiders. DiRienzo and Montiglio (2016a) found consistent differences among individuals in web structure, and that black widow spiders (Latrodectus hesperus) with longer femur–patellas build webs with more gum-footed lines. We also found consistent differences among individuals in web structure, and a positive relationship between body size and web size. We therefore tentatively suggest that these two elements could be general features of intra-population variation in spider webs. More studies in other taxa with different extended phenotypes are required to determine whether, within a population, larger individuals usually build bigger nests or larger dams, and so on. DiRienzo and Montilgio (2016a) also found that a higher number of gum-footed lines is associated with increased foraging aggression. However, Montiglio and DiRienzo (2016), also in L. hesperus, found a higher number of both gum-footed and structural lines is associated with decreased foraging aggression, while boldness was not associated with any web characteristics. Given that the relationship between behaviour and web structure we detected overlapped with zero, and that responsiveness to a prey stimulus was not associated with web structure, in aggregate it seems there is yet no clear pattern in how web structure and behaviours are associated among individuals.
The residual covariances between web diameter, web density and web evenness indicates that when a spider builds a relatively larger web (relative to its own typical web diameter) it also builds a relatively less even and less dense web. The decrease in evenness could result from an increase in up-down asymmetry, as a larger difference between the number of spirals upwards vs. downwards would give a higher score for our measure of web evenness. Alternatively, a spider may struggle to apply its usual web spinning strategy at a greater spatial scale without making mistakes, and so created a less even and less dense web when it tries to make a larger web than usual. A third explanation is that our measure of web evenness could reflect the amount of contact with prey the web experienced that day (webs are typically spun in the morning and all web measurements were taken in the afternoon). M. vigorsii do not leave prey remains in their web (D.N.F., pers obs.); however, contact with prey, whether leading to a successful capture or not, leads to the removal of spirals and sometimes radii. The removal of these threads reduces density, as well as change in the number of spirals along one axis but not others, giving the impression of a less even web. A greater rate of contact with prey would be expected with larger webs as they cover a greater area, but we would perhaps expect this covariance to be present at the among-individual and among-date levels as well as at the residual level. Ultimately, we do not have additional data that would allow us to identify what process might be driving the residual covariances between web diameter, density, and evenness at this time.
We note here that correlated measurement error can give residual covariances between traits. We think this is unlikely to have occurred here, as web diameter and the other measures of web structure were measured using different tools, and web density and web evenness were calculated once we had returned from the field. This reduces the chance that we could make mistakes that simultaneously influenced all measurements.
The correlation between responsiveness to prey and reaction to predation was not different from zero as an among-individual correlation or at any other level (Figs 4–6; Supporting Information, Fig. S1), yet was significant at the raw phenotypic level (Fig. 2). This acts as a cautionary tale against assuming any phenotypic correlations represent among-individual correlations (the ‘individual gambit’; Brommer, 2013). In general, we should be careful to identify at what level (within-individual, among-individual, among-day) any hypothesized relationships between traits should exist, and then construct statistical models that specifically estimate these terms, allowing us to directly evaluate our hypotheses (Dingemanse & Dochtermann, 2013; e.g. Moiron et al., 2016).
CONCLUSION
We found that M. vigorsii show consistent among-individual differences in aspects of web structure, and that there is a syndrome between web structure and morphology such that larger individuals spin wider and less dense webs. These may be general features of intra-population (co)variation in morphology and extended phenotypes and could represent among-individual variation in foraging strategies or aging-dependent changes in various aspects of the phenotype. Reaction to a predator stimulus was very slightly repeatable and this trait may be integrated into the among-individual syndrome with morphology and web structure, but uncertainty was high. These results highlight how extended phenotypes can be integrated into general suites of trait variation, and so selection likely acts upon these traits in concert. We also found that when a spider builds a relatively wider web the web is also relatively less dense and less even, although we are unable to separate three potential explanations for this pattern. Responsiveness to a prey stimulus and web evenness were not consistently different among individuals, and so are completely plastic or environmentally determined traits that are not based on more stable individual characteristics. Extended phenotypes like the web traits evaluated here represent a suite of biological traits that have perhaps been understudied in the past literature on individual variation, yet these traits represent important biological variation that can play key roles in organisms’ lives and in ecosystems more broadly. Here we have demonstrated that, in a population of wild organisms, aspects of an extended phenotype are integrated into a syndrome with morphological, and possibly behavioural, traits. Therefore, our expectations for selection on and evolution of extended phenotypes should consider how they integrate with other phenotypes under natural conditions.
SUPPORTING INFORMATION
Additional Supporting Information may be found in the online version of this article at the publisher’s web-site:
Appendix S1.Micrathena R code and Raw data.
Figure S1. Estimated modes and 95% credible intervals of the among-date correlations from the original model in the main text (see also Supporting Information, Table S1).
Table S1. Model results for the original multivariate model in the main text.
Table S2. Model results for the model of occasions where spiders fled during the prey test, and so were assigned “NAs” for responsiveness to prey and reaction to predation threat rather than 180 and 24, respectively.
Table S3. Model results for the model of occasions where spiders recorded maximum scores for responsiveness to prey and reaction to predation threat (180 and 24, respectively) were assigned “NAs” in place of these values.
Table S4. Model results from the model where all among-date covariances were assumed to be zero and so not estimated.
ACKNOWLEDGEMENTS
We thank J.B. Barnett, H.M. Anderson, B.L. McEwen, J.L.L. Lichtenstein, and R. Costa-Pereira for being excellent colleagues in the field. We also thank T.D. Swanson and the staff of the Andes and Amazon Field School at Iyarina for making our stay as comfortable and enjoyable as possible. Comments from three anonymous reviewers improved the manuscript. We have no conflicts of interest. D.N.F. and J.N.P. designed the study. J.Y. acquired the permits. D.N.F. collected and analysed the data and drafted the manuscript. All authors contributed to revisions of the manuscript and approved the final version. The work was supported by a Canada 150 Research Chair award to J.N.P.
REFERENCES