-
PDF
- Split View
-
Views
-
Cite
Cite
Lisa Ramaut, Linde Moonen, Thessa Laeremans, Joeri L Aerts, Maxim Geeroms, Moustapha Hamdi, Push-Through Filtration of Emulsified Adipose Tissue Over a 500-µm Mesh Significantly Reduces the Amount of Stromal Vascular Fraction and Mesenchymal Stem Cells, Aesthetic Surgery Journal, Volume 43, Issue 9, September 2023, Pages NP696–NP703, https://doi.org/10.1093/asj/sjad125
- Share Icon Share
Abstract
Mechanical isolation of the stromal vascular fraction (SVF) separates the stromal component from the parenchymal cells. Emulsification is currently the most commonly used disaggregation method and is effective in disrupting adipocytes and fragmenting the extracellular matrix (ECM). Subsequent push-through filtration of emulsified adipose tissue removes parts of the ECM that are not sufficiently micronized, thereby further liquifying the tissue.
The aim of this study was to investigate whether filtration over a 500-µm mesh filter might affect the SVF and adipose-derived mesenchymal stem cell (MSC) quantity in emulsified lipoaspirate samples by removing ECM fragments.
Eleven lipoaspirate samples from healthy nonobese women were harvested and emulsified in 30 passes. One-half of the sample was filtered through a 500-µm mesh filter and the other half was left unfiltered. Paired samples were processed and analyzed by flow cytometry to identify cellular viability, and SVF and MSC yield.
Push-through filtration reduced the number of SVF cells by a mean [standard deviation] of 39.65% [5.67%] (P < .01). It also significantly reduced MSC counts by 48.28% [6.72%] (P < .01). Filtration did not significantly affect viability (P = .118).
Retention of fibrous remnants by push-through filters removed ECM containing the SVF and MSCs from emulsified lipoaspirates. Processing methods should aim either to further micronize the lipoaspirate before filtering or not to filter the samples at all, to preserve both the cellular component carried within the ECM and the inductive properties of the ECM itself.
The current regulatory standards of both the US Food and Drug Administration (FDA)1 and the European Medicines Agency2 on the therapeutic use of biomaterials have imposed important limitations on the enzymatic isolation of the stromal vascular fraction (SVF) in clinical practice. This has resulted in a plethora of mechanical isolation methods that compete to offer superior cell yields and viability.3 Because the regenerative properties of the SVF are now being widely introduced in therapeutic settings, isolation methods should be safe, easy, effective, reproducible, and cost-efficient.4 Mechanical isolation of the SVF usually destroys the parenchymal adipose cells and removes debris to purify the stromal component. Therefore, isolation protocols are being optimized to successfully and selectively dissociate SVF from its surroundings without impairing cell yield and viability.3 Emulsification, as introduced by Tonnard and Verpaele in the original nanofat protocol,5 is an easy, applicable, and cheap blunt disaggregation method that is still the most applied method in bedside mechanical SVF isolation.6 It disrupts the adipocytes and micronizes the extracellular matrix (ECM) by the shear forces produced by shuffling the lipoaspirate back and forth between 2 interlocked syringes. After disaggregation by emulsification, the lipoaspirate is washed and filtered through a nylon cloth with 500-µm pores or a 500-µm mesh filter to remove fibrous remnants that might clog up fine needles. When this step is not preceded by sufficient disaggregation, SVF-containing ECM may be removed from the substrate because studies have shown that SVF is closely attached to the fibrous extracellular and perivascular matrix.6 In this study we aimed to evaluate, by flow cytometric analysis, whether push-through filtration of emulsified lipoaspirate over a 500-µm filter has an impact on the cell yield and viability of the SVF and adipose-derived mesenchymal stem cells (MSCs).
METHODS
This study was conducted between April 2021 and December 2021. Adipose tissue was obtained from the abdomen of 13 female patients undergoing liposuction under general anesthesia. Patients with BMI over 28 kg/m2, diabetes mellitus, connective tissue disease, or a history of smoking, radiotherapy, or chemotherapy were excluded. Written informed consent was obtained from each patient and the study was approved by the institutional ethics committee of the University Hospital of Brussels, Brussels, Belgium. Patients had a mean age of 39.1 years (range, 28-49 years) and a mean BMI of 24.2 kg/m2 (range, 21-26 kg/m2).
Tissue Harvesting
Tissue samples were harvested 10 minutes after infiltration of the subcutaneous tissue with a saline/adrenalin solution (1/1,000,000). Manual negative-pressure liposuction was performed using a blunt 3-mm multiport cannula with sharp 1-mm side holes connected to a 10-mL syringe. Centrifugation (1 minute, 1200g) was performed to separate and discard the watery portion before transporting the samples to the laboratory on ice. Lipoaspirates obtained were shuffled 30 times, as described in the original nanofat protocol,5 with a Tulip Nanotransfer kit; the lipoaspirate was shuffled 10 times between 2 connected 10-mL syringes with a 2.4-mm connector piece, 10 times with a 1.4-mm diameter connector piece, and 10 times with a 1.2-mm diameter connector piece (Tulip Nanotransfer, San Diego, CA). The 1.4-mm connector was used for the second 10 passes because our clinical experience taught us that this gently further emulsifies the lipoaspirate before transferring it through the smallest 1.2-mm connector piece. The obtained emulsified lipoaspirate was divided into 2 equal portions. One portion was filtered over a 500-µm mesh filter; the other portion was left unfiltered.
Isolation of the Stromal Vascular Fraction
From each sample, 10 mL of aspirated and emulsified fat was digested with the same volume of 0.2% collagenase (Sigma-Aldrich Corp., St Louis, MO) for 45 minutes at 37°C in a shaking incubator. Phosphate-buffered saline (PBS; VWR, Belgium) was added to stop digestion and the sample was centrifuged at 600g for 10 minutes. The oily portion was discarded and 2 further PBS washing and centrifugation steps were performed to obtain the SVF pellet. The pellet was incubated with erythrocyte lysis buffer for 2 minutes.
Flow Cytometry
The SVF pellet was stained with Fixable Viability Stain (FVS) eFluor 780 (Thermo Fisher Scientific, Waltham, MA; 1/4000 dilution in PBS) for 20 minutes at room temperature to exclude dead cells. Next, samples were stained with a 9-color panel to identify the SVF and MSCs using CD45-Alexa Fluor 700 (clone HI30, BioLegend, San Diego, CA), CD44-FITC (clone BJ18, BioLegend), CD90-PE-Cy7 (clone 5E10, BD Biosciences, Franklin Lakes, NJ), CD73-BV510 (clone AD2, BD Biosciences), CD105-BV605 (clone 266, BD Biosciences), TRAIL/CD253 (clone RIK-2, BioLegend), CD31-PE (MBC78.2, BD Biosciences), and CD34-BV421 (clone 561, BioLegend). Antibodies were diluted in fluorescence-activated cell sorting buffer (PBS containing 1% bovine serum albumin and 0.1% sodium azide) and were incubated for 30 minutes at 4°C. Samples were acquired on a BD LSR Fortessa flow cytometer (BD Biosciences). CompBeads (BD Biosciences) were used to perform automatic compensation. Flow cytometric data were analyzed with FlowLogic v. 7.3 software (Miltenyi Biotec, Bergisch Gladbach, Germany). Two samples were lost due to clustering of the antibodies during storage before read-out.
Gating
Selection of the population of interest was based on the size and complexity of the cells determined by forward and side scatter, respectively. Cell aggregates were excluded based on forward-scatter and side-scatter height/width ratios, followed by exclusion of dead cells (positive for FVS) to overcome autofluorescent signals. Based on the expression of surface markers, SVF was subsequently identified as CD45–, CD34+, CD31–, and CD235a–. Further gating on cells that express CD90, CD44, CD105, and CD73 was used to identify MSCs7,8 (Figure 1).
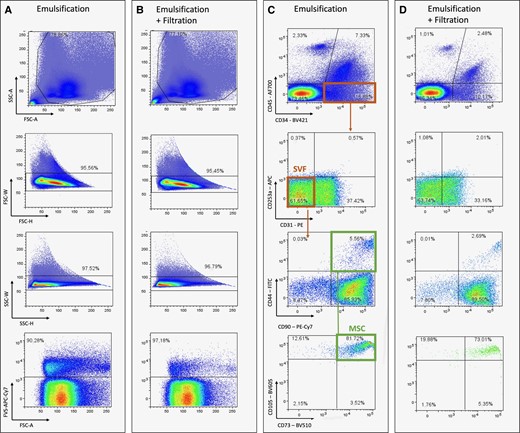
Representative flow-cytometry plots to define SVF and MSCs after emulsification only (A, C) and emulsification and filtration (B, D). Population of interest was defined from FSC-A/SSC-A plots followed by exclusion of cell aggregates based on FSC-W/H and SSC-W/H. Nonviable cells were excluded using Fixable Viability Dye 780 after which SVF and MSCs were defined. A, area; FSC, forward scatter; H, height; MSC, mesenchymal stem cell; SSC, side scatter; SVF, stromal vascular fraction; W, width.
Statistical Analysis
An a priori power analysis was performed, which suggested a minimum sample size of 8. Prism v. 9.1.2 (GraphPad, San Diego, CA) was used to analyze the obtained data. Results were considered significant when the P-value was <.05. The Shapiro-Wilk test was chosen to assess normality in consideration of the limited sample size. It did not show nonnormality for total cell yield, viability, SVF%, MSC%, and absolute SVF count. Absolute MSC count and MSC/SVF ratio did not pass the normality test and were analyzed with the Wilcoxon matched-pairs signed-rank test. For all other analysis, 1-way paired t test was used. Data are presented as mean [standard error of the mean] unless otherwise indicated. After evaluation of the data, 1 outlier was excluded; all analyses were conducted on the trimmed dataset.
RESULTS
Filtration of Emulsified Lipoaspirate Significantly Affects Total Cell Yield, but Does Not Affect Cell Viability
Emulsified adipose tissue had a mean total cell yield of 17.19 [1.4] × 104 cells/mL, which is significantly higher than the emulsified and filtered samples (mean, 12.41 [1.1] × 104 cells/ml; P = .015) The emulsified samples yielded more viable cells (15.70 [1.2] × 104 cells/mL) compared with the lipoaspirate that was both emulsified and filtered (11.55 [0.98] × 104 cells/mL) (P = .015). With a viability of 91.36% [0.607%] in the emulsification group and 93.21% [0.774%] in the filtration group, none of the samples showed inadequate viability. No significant difference in viability could be shown between both groups (P = .118) (Table 1; Figure 2).
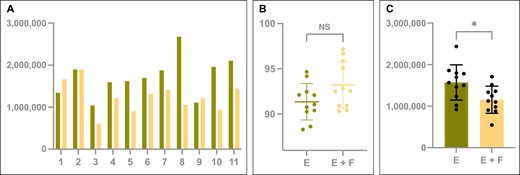
(A) The total cell yield of each individual sample and (B) the average cell viability (%). (C) The total amount of viable cells is shown for emulsified (green) and filtered (yellow) processed lipoaspirate samples after enzymatic digestion and staining for flow cytometry analysis. Box plots display mean and standard deviation. E, emulsified; F, filtered; NS, not significant. *P < .05.
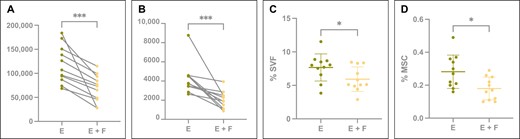
(A) The absolute SVF and (B) MSC yield in total detected viable cells for paired emulsified (green) and emulsified and filtered (yellow) samples. Concentration of SVF (D) and MSC (E) yield expressed as percentage of cells present in total detected viable cells for emulsified (green) and emulsified and filtered (yellow) samples. Box plots display mean and standard deviation. *P < .05; ***P < .001. E, emulsified; F, filtered; MSC, mesenchymal stem cell; SVF, stromal vascular fraction.
Total Cell Yield, Cell Viability, and Total Viable Cell Yield After Enzymatic Digestion and Staining for Fluorescence-Activated Cell Sorting Analysis of Emulsified (E) and Emulsified and Filtered (E + F) Samples
. | E . | E + F . | P-value . |
---|---|---|---|
Total cell yield (cells/mL) | 17.19 [1.4] × 104 | 12.41 [1.1] × 104 | .015 |
Total viable cell yield (cells/mL) | 15.70 [1.2] × 104 | 11.55 [0.98] × 104 | .015 |
Viability (%) | 91.36 [0.607] | 93.21 [0.774] | .118 |
. | E . | E + F . | P-value . |
---|---|---|---|
Total cell yield (cells/mL) | 17.19 [1.4] × 104 | 12.41 [1.1] × 104 | .015 |
Total viable cell yield (cells/mL) | 15.70 [1.2] × 104 | 11.55 [0.98] × 104 | .015 |
Viability (%) | 91.36 [0.607] | 93.21 [0.774] | .118 |
P-values are shown for each outcome for the comparison between E and E + F samples. Data are presented as mean [standard deviation].
Total Cell Yield, Cell Viability, and Total Viable Cell Yield After Enzymatic Digestion and Staining for Fluorescence-Activated Cell Sorting Analysis of Emulsified (E) and Emulsified and Filtered (E + F) Samples
. | E . | E + F . | P-value . |
---|---|---|---|
Total cell yield (cells/mL) | 17.19 [1.4] × 104 | 12.41 [1.1] × 104 | .015 |
Total viable cell yield (cells/mL) | 15.70 [1.2] × 104 | 11.55 [0.98] × 104 | .015 |
Viability (%) | 91.36 [0.607] | 93.21 [0.774] | .118 |
. | E . | E + F . | P-value . |
---|---|---|---|
Total cell yield (cells/mL) | 17.19 [1.4] × 104 | 12.41 [1.1] × 104 | .015 |
Total viable cell yield (cells/mL) | 15.70 [1.2] × 104 | 11.55 [0.98] × 104 | .015 |
Viability (%) | 91.36 [0.607] | 93.21 [0.774] | .118 |
P-values are shown for each outcome for the comparison between E and E + F samples. Data are presented as mean [standard deviation].
Filtration of Emulsified Lipoaspirate Significantly Reduces SVF and MSC Quantity and Concentration
Filtration of emulsified lipoaspirate resulted in a reduction in the absolute and relative amounts of SVF and MSCs that were isolated from the lipoaspirate samples. Filtration reduced the number of SVF cells by on average 39.65% [5.67%] (P < .01) and MSCs by on average 48.28% [6.72%] (P < .01) (Table 2, Figure 3).
Relative (%) and Absolute Cell Yield of SVF and MSCs After Enzymatic Digestion and Staining for Flow Cytometry Analysis of Emulsified (E) and Emulsified + Filtered (E + F) Samples
. | SVF percentage (%) . | SVF total cell yield (cells/mL) . | MSC percentage (%) . | MSC total cell yield (cells/mL) . |
---|---|---|---|---|
E | 7.67 [0.61] | 1.18 [0.12] × 104 | 0.28 [0.03] | 4.26 [0.50] × 102 |
E + F | 5.93 [0.55] | 0.69 [0.09] × 104 | 0.18 [0.02] | 2.04 [0.03] × 102 |
P-value | .012 | .0009 | .0087 | .0009 |
. | SVF percentage (%) . | SVF total cell yield (cells/mL) . | MSC percentage (%) . | MSC total cell yield (cells/mL) . |
---|---|---|---|---|
E | 7.67 [0.61] | 1.18 [0.12] × 104 | 0.28 [0.03] | 4.26 [0.50] × 102 |
E + F | 5.93 [0.55] | 0.69 [0.09] × 104 | 0.18 [0.02] | 2.04 [0.03] × 102 |
P-value | .012 | .0009 | .0087 | .0009 |
P-values are shown for each outcome for the comparison between E and E + F samples. Data are presented as mean [standard error of mean]. MSC, mesenchymal stem cell; SVF, stromal vascular fraction.
Relative (%) and Absolute Cell Yield of SVF and MSCs After Enzymatic Digestion and Staining for Flow Cytometry Analysis of Emulsified (E) and Emulsified + Filtered (E + F) Samples
. | SVF percentage (%) . | SVF total cell yield (cells/mL) . | MSC percentage (%) . | MSC total cell yield (cells/mL) . |
---|---|---|---|---|
E | 7.67 [0.61] | 1.18 [0.12] × 104 | 0.28 [0.03] | 4.26 [0.50] × 102 |
E + F | 5.93 [0.55] | 0.69 [0.09] × 104 | 0.18 [0.02] | 2.04 [0.03] × 102 |
P-value | .012 | .0009 | .0087 | .0009 |
. | SVF percentage (%) . | SVF total cell yield (cells/mL) . | MSC percentage (%) . | MSC total cell yield (cells/mL) . |
---|---|---|---|---|
E | 7.67 [0.61] | 1.18 [0.12] × 104 | 0.28 [0.03] | 4.26 [0.50] × 102 |
E + F | 5.93 [0.55] | 0.69 [0.09] × 104 | 0.18 [0.02] | 2.04 [0.03] × 102 |
P-value | .012 | .0009 | .0087 | .0009 |
P-values are shown for each outcome for the comparison between E and E + F samples. Data are presented as mean [standard error of mean]. MSC, mesenchymal stem cell; SVF, stromal vascular fraction.
Filtration Did Not Significantly Affect the MSC/SVF Ratio
Analysis of the ratio of MSCs/SVF cells in the emulsified (3.68 [1.07]) and filtered samples (3.55 [2.08]) did not show a statistically significant difference (P = .3667). However, the MSC/SVF ratio showed higher variability in the filtration group, while the ratio was more consistent in the emulsification sample.
DISCUSSION
This in vitro study has shown that retention of fibrous remnants by push-through filters removes a significant amount of SVF and MSCs from emulsified lipoaspirate. Our results show that filtration of emulsified lipoaspirates did not affect viability, but significantly reduced SVF quantity by one-third and MSC quantity by nearly half of their population on flow-cytometric analysis of fresh samples. Immunohistochemical research has shown that a large portion of the SVF cells reside close to and attached to the ECM and vascular structures.6 This explains why enzymatic isolation of SVF results in a 10- to 20-fold higher release of stromal cells compared with mechanical isolation: enzymatic cleavage of connective tissue releases cells that otherwise remain embedded in the matrix.3,9 Mechanical isolation methods dissociate SVF cells from parenchymal cells by mechanical force, but this process also produces significant amounts of cellular debris. Proper removal of oil, liquid, and other cellular debris was claimed to be essential to eliminate inflammatory triggers, as demonstrated by the better survival of filtered vs nonfiltered fat grafts.10,11 Purification steps intend to discard unwanted components, but therefore should be preceded by a disaggregation method that separates the components properly. Emulsification is a processing step in which the lipoaspirate is shuffled back and forth manually between 2 interconnected syringes, as introduced by Tonnard and Verpaele in the original nanofat protocol.5 Studies have shown that this destroys adipocytes and micronizes the ECM by shear stress without impairing viability or differentiation capacities.12,13 It appears that the mechanical force of processing induces a stress-induced progenitor population and phenotypic changes in the SVF with up to 3-fold up-regulation of mesenchymal markers,12,14 although prolonged emulsification protocols (>2 minutes) showed down-regulation of MSCs and endothelial population density.15 In the same regard, the direct use of connector pieces with a smaller diameter (<1.5 mm) (without first shuffling through a larger connecting piece) was shown to adversely affect the viability and number of MSCs.16 Thus, although effective in moderation, the degree to which the sample can be micronized by emulsification is also limited. Therefore, it is important that the disaggregation method is adapted to the order of magnitude of the purification step in order not to accidentally discard important amounts of the SVF during processing; there is a great risk of this occurring when purification actively retains tissue components based on magnitude, better known as filtration. This step is aimed at removing the fibrous remnants that are not sufficiently disaggregated and that clog up fine needles. This concept was introduced by Tonnard and Verpaele in the original nanofat protocol, where a nylon cloth with 500-µm pore size was used for this purpose. Currently, there are 500-µm and 400- to 600-µm meshes integrated in some commercially available processing kits (Nanofastkit, Corios, Milan, Italy and Tulip Nanotransfer, Tulip, San Diego, CA, respectively) and filtration is part of several more recent adaptations of the nanofat protocol, such as SVF gel15 and nanofat cell aggregates.17 Filtration of emulsified lipoaspirate through a nylon mesh has been discussed by Furno et al, who suggested that some of the adipose-derived MSCs are lost in the final mesh filtration step. They found that their nonfiltered emulsified lipoaspirate (which they termed “Nanofat 2.0”) showed higher cell counts and faster culture rates than filtered samples.18 Their findings corroborate the results in this paper, except that we performed a further population-specific gating to identify the cell population and could show the statistical significance of our results in a paired-sample analysis. This paper does not refer to the substrate as “nanofat” because our samples underwent 1 session of centrifugation and 2.4-, 1.4-, and 1.2-mm diameter Luer-Lock connector pieces were used to emulsify the lipoaspirate. Therefore, the terms emulsified fat and filtered emulsified fat are used to avoid any confusion.
The 500-µm metal single-mesh filter used in this experiment differs from the double-mesh 400- to 600-µm Tulip filter but has the same order of magnitude as the 500-µm nylon cloth. It was chosen to represent the most common order of magnitude of filtration in commercially available processing sets and in the scientific literature. The mesh has the disadvantage that it has a smaller surface area through which the lipoaspirate must be transferred. Subsequently, when it clogs up with fibrous remnants, the pore size through which the remaining lipoaspirate must pass is reduced, which entails the need to increase pressure on the remaining tissue in the syringe. In the nylon cloth, the lipoaspirate has a larger surface area through which the lipoaspirate can migrate when pores are clogging up, causing less pressure build-up on the lipoaspirate. The use of a mesh filter in a cannister between 2 syringes does ensure an equal applied force and transfers the lipoaspirate between syringes, compared to the manual squeeze that is applied on the nylon cloth in which the pressure is rather irregular and less reproducible. When using the nylon cloth, the lipoaspirate must also be transferred back into the syringes, which is an extra processing step.
Mashiko et al introduced sharp disaggregation with a spinning blade as an alternative to emulsification (ie, blunt disaggregation) and concluded that blunt pressure yielded less disaggregation of adipocytes and ECM than sharp disaggregation of fat tissue. They centrifuged the lipoaspirate once before and once after the sharp disaggregation and called this “squeezed fat.” It showed double the culture speed and higher amounts of MSCs and endothelial cells compared with samples that were centrifuged, emulsified, and filtered over a 500-µm nylon cloth.19 This double-centrifugation step was also applied by Pallua et al to further condense emulsified lipoaspirate samples, which they called “lipoconcentrate.” Their results showed that no significant amount of progenitor cells was lost in the aqueous and oily remnants, and therefore centrifugation was effective in removing unwanted debris.20 To be able to administer the processed lipoaspirate as SVF in a clinical setting, sufficient disaggregation of lipoaspirate still needs to take place. Thus, processing methods that use sharp disaggregation as proposed by Mashiko et al,19 cutting filters as proposed by Cohen et al,21 grinders,22 or multiple-hole emulsification connectors as proposed by van Dongen et al23 might serve as valuable alternatives to better micronize the adipose tissue while preserving the stem cell niche and soluble factors attached to the ECM. In vitro analysis shows superior cell yields compared with the original nanofat protocol.21,22,24 It has been frequently noted that the purpose of better micronization is to preserve the ECM and its stem cell niche to improve the regenerative action. Proper micronization serves to solve the issue of clogged-up needles in the clinical setting. When increased pressure is applied to clogged-up fine needles, the risk of sudden ejection with overinjection under high pressure might be very disadvantageous in delicate zones such as the periorbital or perioral area. If the tissue is still not liquid enough for administration, filtration can be defendable in order to properly administer the substrate, taking into account that the administered cell dosage will be lower. The use of a bigger pore size of the filter, for example >600 µm, might reduce the retention of SVF, but this should be investigated in a laboratory setting. Nanofat was identified as disaggregated fat graft with particles around 600 µm after proper emulsification, in which no intact adipocytes can be found that should be retained by a small-pore-sized filter.
Altogether, the scientific literature has previously questioned filtration of emulsified lipoaspirate and it is becoming clear that preservation of ECM in which the stem cell niche resides is favorable above the isolation of single-cell suspensions.15,17,25 Cell aggregates have an enhanced response to proliferation and differentiation capacities, which showed benefits in wound healing from skin flap necrosis in mice.26 The clinical relevance of preserving the ECM with the SVF was further investigated by Yao et al, who called it “SVF-gel.” Their findings showed that SVF-gel promotes wound healing in mice when compared with SVF-cell-based treatments alone. They disaggregated their samples by emulsification and favored a shorter emulsification time (1 minute) because they saw that ECM was less fragmented, which, according to them, better preserves the microenvironment referred to as the “stem-cell niche.”15,27 This microenvironment formed by adipose-derived ECM and its soluble factors has been shown to be able to induce formation of blood vessels and adipogenic differentiation in the absence of culture medium when seeded with adipose-derived MSCs in vitro.28 The importance of the preservation of the 3-dimensional structure and the inductive environment of the ECM is also reflected in the search for the ideal carrier for stem cell therapies, which aims to mimic this physiological environment in which the regenerative machinery can thrive.29 In wound-healing studies, the limited proliferative capacities of uncultured adipose-derived stem cells seem not to be useful. Their differentiation capacities are far more interesting and therefore the inductive environment of the ECM and its growth factors and cytokines should be preserved in wound-healing research.30
Finally, we must keep in mind that there is still no evidence on the clinical implications of the loss of a certain number of regenerative cells. Currently little is known about whether the administration of higher numbers of regenerative cells necessarily implies more regenerative action. Some studies have found an increase in fibrosis and a decrease in fat graft survival and adipogenesis when large numbers of SVF cells were added in cell-assisted lipotransfer.31 The constitutive cell dose concept proposed by Sesé et al suggests that the number of transplanted cells requires at least the density of the native cell population of a given tissue to initiate a sufficient reparative response.17 At this point, there is no known dose-dependent relationship between the SVF cellular concentration and its therapeutic effect. The methodologic and clinical heterogeneity of published papers makes it impossible to meta-analyze the efficacy of a certain cellular dosage or treatment protocol that has clinically superior results. In vitro and preclinical studies strongly suggest that the preservation of ECM in which SVF is embedded is advantageous for its paracrine properties, preservation of the stem cell niche, and migrational and angiogenetic support. Furno et al compared unfiltered and filtered nanofat in 4 clinical cases, which is insufficient to provide any evidence.18 Gentile et al compared different processing protocols with and without filtration in a larger group of 43 patients, but the design of their study lacked power to conclude one protocol was superior to another regarding clinical results.22 Altogether, the cost of losing tissue fragments in which SVF is embedded in order to further liquify the substrate for clinical use is currently not defendable.
Limitations
This study did not compare different disaggregation methods or different filters. Composition of filters might impact whether they are more retentive or further disaggregating. Differences in culture speed and differentiation between the samples were not reported and should be the subject of further research.
CONCLUSIONS
This study is the first to identify, by means of paired-sample flow cytometry analysis, uncultured SVF and MSC populations that are affected by the retention of fibrous remnants by push-through noncutting mesh filters. This processing step removes a significant amount of SVF- and MSC-containing ECM from emulsified lipoaspirate. It is widely agreed upon in the scientific literature that isolation methods should aim to preserve SVF and the ECM microenvironment as much as possible. Therefore, processing methods should aim either to further micronize the lipoaspirate before filtering or not to filter lipoaspirates at all in order to preserve both the cellular components carried within the ECM as the inductive properties of ECM itself. Proper disaggregation with a matching purification step is key in mechanically preparing lipoaspirates for SVF-based therapy.
Acknowledgments
Drs Ramaut and Moonen contributed equally to this work as co-first authors.
Disclosures
The authors declared no potential conflicts of interest with respect to the research, authorship, and publication of this article.
Funding
The authors received no financial support for the research, authorship, and publication of this article.
REFERENCES
Author notes
Dr Ramaut is a resident in training, Department of Plastic and Reconstructive Surgery, University Hospital of Brussels, Brussels, Belgium.
Dr Moonen is a research fellow, Department of Plastic and Reconstructive Surgery, University Hospital of Brussels, Brussels, Belgium.
Dr Hamdi is a professor, Department of Plastic and Reconstructive Surgery, University Hospital of Brussels, Brussels, Belgium.
Dr Hamdi is an international editor for Aesthetic Surgery Journal.
Ms Laeremans is a PhD candidate, Department of Neuro-Aging and Viro-immunotherapy, Free University of Brussels, Brussels, Belgium.
Dr Aerts is a professor, Department of Neuro-Aging and Viro-immunotherapy, Free University of Brussels, Brussels, Belgium.
Dr Geeroms is a reconstructive microsurgery fellow, Department of Plastic and Reconstructive Surgery, Division of Reconstructive Microsurgery, Chang Gung Memorial Hospital, Linkou, Taiwan.