-
PDF
- Split View
-
Views
-
Cite
Cite
He Qiu, Yichen Jiang, Chang Chen, Kelun Wu, Hang Wang, The Effect of Different Diameters of Fat Converters on Adipose Tissue and Its Cellular Components: Selection for Preparation of Nanofat, Aesthetic Surgery Journal, Volume 41, Issue 11, November 2021, Pages NP1734–NP1744, https://doi.org/10.1093/asj/sjab146
- Share Icon Share
Abstract
Nanofat is an autologous product prepared mechanically from harvested fat. In nanofat grafting, converters are employed for mechanical emulsification to facilitate fat injection. To date, the study of different converters has received scant attention regarding whether they affect the characteristics of nanofat in terms of the practical applications and indications.
The authors set out to investigate the influence of different internal diameters of converters on biological functionality of nanofat during shuffling.
The 3-dimensional finite element method was employed to simulate the process of mechanical emulsification of fat and to research the stress with 5 different converters (3.76 mm, 2.00 mm, 1.20 mm, 1.00 mm, 0.80 mm). An assessment of the morphology of emulsified fat was conducted. Isolated stromal vascular fraction (SVF) was analyzed for cellular components, number, and viability through flowcytometry and live/ dead staining. Adipocytic and angiogenic differentiation assay allowed assessment of differentiation capacity of the SVF.
The smaller the aperture of the converter, the greater the mechanical force on adipose tissue during mechanical emulsification, showing the different macroscopic and microscopic structure of the emulsified fat. No difference in viability or ratio of endothelial progenitor cells and other cells was found. Angiogenic and adipogenic differentiation capacity of the SVF significantly changed in 5 different converters.
The mechanical emulsification from different apertures of converters exerts different effects of adipose tissue structure, cell content, and multipotency differentiation but not its viability. Converters with different apertures can be selected according to clinical needs.
Autologous fat transplantation has emerged as one of the most commonly employed soft tissue-filling and repair approaches for a wide range of sources, avoiding implantation of prostheses, less immunogenicity, and good biocompatibility in clinical practice.1-4 According to the size of fat particles and processing method, fat transplantation can be divided into 3 categories: large-particle fat transplantation (macrofat), small-particle fat transplantation (microfat), and nanometer-fat transplantation (nanofat).1,5,6 Large fat particles are untreated adipose particles obtained by the structural fat transplantation technique invented by S.R. Coleman in 1988.1 Microfat grafting refers to lipofilling with cannulas as small as 0.7 mm in diameter.5 Nanofat is the liquid suspension obtained by further filtration of emulsified fat, mainly grafting in the superficial layer of the skin with fine sharp needles. Clinicians tend to treat volume losses with macrofat graft utilizing large blunt cannulas. If adipose tissue were forced to pass through the fine injection cannula under high pressure, it would render the procedure strenuous and lead to irregular deposits at the injection site. It is thought that when granular fat is transplanted into the deep dermis, postoperative complications such as fibrocystic invasion, apoptosis and necrosis of fat tissue, and calcified nodules could result.5 So mechanical emulsification may be an ideal alternative to process the fat before injection, especially for some non-structural injection sites with small injection volume and those needing to be fine-tuned, such as the eyelids, lips, and hands.7,8
Nanofat is a kind of non-structural fat resulting from the process of mechanical emulsification called shuffling.9 It is well known that the normal physiological structure of adipose tissue is mainly composed of 2 parts: mature adipocytes and vascular matrix fragment (stromal vascular fraction [SVF]).7,10 Mature adipocytes comprise less than 7% cell counts, but they account for more than 90% of the volume of the tissue and are highly oxygen consuming. Mainly located in the intercellular space of adipocytes, SVF contains extracellular matrix and functional cellular components of adipose tissue. No adipocytes and normal adipose tissue structure are visible in nanofat followed by an emulsification procedure. After the procedure, emulsified fat contains a higher proportion of endothelial progenitor cells (EPCs), multi-differentiated mesenchymal stem cells, including adipose-derived stem cells (ADSCs), and other cell populations related to regeneration.11-13 As previously proven, nanofat mainly facilitates the regeneration ability of stem cells to promote tissue regeneration at the injection site, besides the lipofilling property of adipocytes.14-16 Strictly speaking, it is more like a tissue rejuvenator than tissue filler. In reviewing the relevant literature, positive results were found on the effect of emulsified fat for skin rejuvenation, wound healing, tissue repair and regeneration, and scar treatment.14,17-23 To improve the biological functionality of nanofat, the relevant technical parameters involved in fat processing are worthy of being highlighted.
Regarding the emulsification process, we must mention the protagonist of the converter, which is connected by 2 syringes for shuffling the lipoaspirate. Georges et al employed the 2.4-, 1.4-, and 1.2-mm anaerobic transfer device to emulsify microfat for the treatment of dark circles. Some researchers emulsified fat only by a Luer-Lock fitting with an internal diameter of 2.4 mm.17,24 Emulsified fat also can be obtained by shifting through a 3-way stop cock connector.20
The shuffling converter selection varies from person to person while sharing a common goal of improvement of graft survival needs. In the process of emulsified fat preparation, the mechanobiology and biological functionality of adipocytes and SVF cells all may be affected by the aperture of the converter connected to the syringe.11 These factors are closely related to the clinical efficacy of nanofat transplantation. Unfortunately, no high-quality system studies about the effect of converters on fat preparation were studied. Therefore, this study makes a major contribution to the research regarding the effect of different internal diameters of converters on adipose tissue during shuffling. We established a 3-dimensional (3D) model of emulsified fat by finite element analysis. The stress characteristics of emulsified fat in the process of emulsified fat preparation were studied to explore the macroscopic and microscopic structure, cellular components, cell content, adipocyte viability, and multi-directional differentiation potentials of SVF prepared utilizing different diameters of converters.
METHODS
Finite Element Model Analysis
To simplify the shuffling process, a 3D model for preparation of emulsified fat was modeled and simulated by employing Finite Element Model. A schematic diagram of grid division of syringe was established by ANSYS (Canonsburg, PA) modeling and FLUENT software (shown in Figure 1).25-28 Owing to the lower force of fat in the other side of the syringe, the model only shows the force of adipose tissue in the side being pushed. FLUENT software was employed to implement grid reconstruction technology to simulate the movement of the syringe piston and record the stress of adipose tissue in the 3 parts of the syringe—piston, syringe nipple, and converter—under the condition of 2.5 mL/s at 4 time points (0.1, 0.5, 1.0, and 1.5 seconds). Based on the ANSYS Fluent Release 16.1 software, the fat fluid mechanics was measured by the SIMPLEC algorithm for obtaining the pressure and velocity field values and the volume of fluid method employed to track the phase interface of the fat particle.
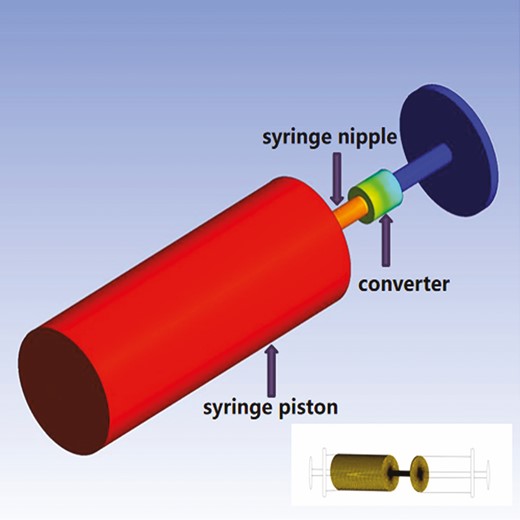
Schematic diagram of the position of syringe piston, syringe nipple, and converter.
Patients and Ethical Approval
Sixteen healthy volunteers were enrolled from patients who underwent cosmetic liposuction in West China Hospital of Stomatology Sichuan University from March 2019 to June 2020. The BMI of the patients included was between 19 and 25, and age ranged from 18 to 40 years. Both preoperative physical examination and clinical routine laboratory examination were performed to exclude those with severe organ dysfunction such as heart, liver, lung, kidney, etc. This experiment was approved by the ethics committee of West China Hospital of Stomatology Sichuan University and carried out after obtaining signed informed consent from all patients.
Emulsified Fat Processing and Collection
Liposuction procedures were performed to harvest subcutaneous adipose tissues from the abdomen of healthy adult donors under general anesthesia employing a blunt cannula (4-mm diameter). Standard liposuction techniques were employed as previously reported.29 Samples were centrifuged at 2000 g for 3 minutes, and we discarded the free lipids and aqueous layers to obtain the lipoaspirate. The same volume of samples was utilized to produce emulsified fat by converters with different hole diameters: 3.76 mm (a female Luer-Lock coupler; 1 round hole; total pore area, 11.10 mm2; Becton, Dickinson and Company, Franklin Lakes, NJ), 2.00 mm (3-way stopcock; 1 round hole; total pore area, 3.14 mm2; ABLE, Guangdong Baihe Medical Technology Co., Ltd., Foshan, China), 1.20 mm (nanofat converter; 3 sharp round holes; total pore area, 3.39 mm2; Xialong Medical Equipment Co., Ltd., Hunan, China), 1.00 mm (nanofat converter; 4 sharp round holes; total pore area, 3.14 mm2; Xialong Medical Equipment Co., Ltd.), and 0.80 mm (nanofat converter; 4 sharp round holes; total pore area, 2 mm2; Xialong Medical Equipment Co., Ltd.). The converters were each utilized to connect two 5-cc syringes. The lipoaspirate was emulsified by transferring the content of 1 syringe into the other at a rate of approximately 2.5 mL/s for 30 transfers. One sample was left unprocessed as a control group.
To measure adipocyte damage in lipoaspirates, every sample was centrifuged at 2330 g for 5 minutes to separate oil, fat, and fluid into distinct layers. The oil ratio (oil volume\[oil volume + fat volume]) was used as an index of adipocyte degeneration according to previous research.30 After double staining of Calcein-AM and propidium iodide for living and dead cells (KeyGen Biotech Co., Ltd., Nanjing, China), the microscopic appearance and adipocyte survival of 6 samples could be observed.31
Isolation and Culture of SVF
The same volume of emulsified fat and control sample was digested with a 0.2% collagenase (Sigma Chemical Co., St. Louis, MO)/phosphate-buffered saline (PBS) solution for 35 minutes at 37°C with gentle shaking, followed by centrifugation at 1800 rpm for 5 minutes. The remaining cellular pellet was resuspended in PBS solution and filtered through a 100-μm cell strainer (Corning, Inc., Durham, NC) and then centrifuged at 1800 rpm for 5 minutes. The SVF cells were resuspended in Dulbecco’s modified Eagle’s medium (Hyclone, Cramlington, UK) supplemented with 10% fetal bovine serum (Invitrogen, Carlsbad, CA) and 1% penicillin-streptomycin (Gibco, Grand Island, NY) and cultured at 37°C in a humidified atmosphere of 95% air and 5% CO2. SVF cells were subcultured at 85% confluence, and passage-3 cells were employed in the study procedures.
Cell Count and Viability Analysis of SVF
The SVF cell suspension obtained from each sample was centrifuged at 1800 rpm for 5 minutes, then the 1-mL PBS solution was added to re-suspension. A total of 20 μL of the liquid was put into the automatic cell counting analyzer for counting. The viability was investigated by live/dead staining (KeyGen Biotech Co. Ltd.) following the manufacturer’s protocol. Under the excitation wavelength of 490 nm and 545 nm, the number and distribution of living cells and dead cells were evaluated under a fluorescence microscope (Olympus Corp., Shinjuku, Tokyo, Japan).
Analysis of SVF Cellular Composition by Flow Cytometry
Approximately 5 × 105 cells of SVF were incubated with CD31-FITC (Abcam, Cambridge, MA), CD34-APC (Abcam), and CD45-PE (Abcam) fluorescence-conjugated antibodies in darkness at 4°C for 30 minutes and then with 7-aminoactinomycin D (KeyGen Biotech Co. Ltd.) for 5 minutes. The stained cells were washed twice with PBS, and a BD Accuri C6 flow cytometer (Becton, Dickinson and Company) was utilized for quantitative analysis of phenotypic profile of SVFs.
Adipocytic Differentiation of SVF by Oil Red O Staining
Adipogenic ability is one of the multipotency differentiation potentials of SVF cell population that ADSCs are mainly responsible for.32 The 3 passages of SVF were cultured in 6-well plates at a concentration of 5 × 104cells/well. After reaching 95% confluence, cells were cultured in AdipoDiff Medium supplemented with 10% fetal bovine serum, 1 μM dexamethasone, 5 μg/mL insulin, 0.5 mM 3-isobutyl-1-methylxanthine, 0.2 mM indomethacin, and 100 U/mL penicillin-streptomycin. Each sample was induced by adipogenesis for 2 weeks and then stained with 1% oil red O. The morphological distribution of the cells was observed by Olympus IX73 microscope (Olympus Corp., Tokyo, Japan), and the absorbance at 510 nm was measured by spectrophotometer (Thermo Fisher Scientific, Rockford, IL).
Matrigel-Based Capillary-Like Tube Formation Assays
A tube formation assay was performed evaluating the SVF angiogenic potential as previously described.33 Briefly, 120 μL of Matrigel (Corning Life Sciences, Corning, NY) was added to a 48-well plate and incubated at 37°C for 30 minutes. SVF cells (1.6 × 105 cells/well) were seeded to the coated wells. After incubation at 37°C for 6 hours, formation of cord-like structures was visualized under microscope (Olympus Corp.). Images were analyzed using Image-Pro Plus software 6.0 (Rockville, MD) according to the manufacturer’s instructions.
Statistical Analysis
All data were statistically analyzed by SPSS 20 and expressed as means ± standard deviation. Comparisons between 2 samples were performed with the paired t test or the multiple groups with 1-way analysis of variance. Statistical significance was defined as P < 0.05. GraphPad Prism 8.0.2 software was employed for charts.
RESULTS
Stress Value of Adipose Tissue at Different Parts and Time Points
In the stress cloud diagram, the stress presented by different color levels indicates the stress value of fat in different parts. From Figure 2A, we can see that the stress values of adipose tissue in syringe piston, syringe nipple, and converter gradually decreased at 4 time points. Figure 2B shows the results that the stress value on fat tissue decreased with an increasing aperture. From 0.5 seconds to 1.5 seconds, the stress was slightly reduced, which may be due to the exudation of oil from adipose cells. It is likely that the force is slightly affected by time and obviously influenced by the hole diameter. The adipose tissue is applied with different forces on the converter, syringe piston, and nipple. The force change from syringe to converter in the 1.20-mm group was similar to that in the 1.00-mm group. In the 0.80-mm group, adipose encountered the greatest force at different time points.
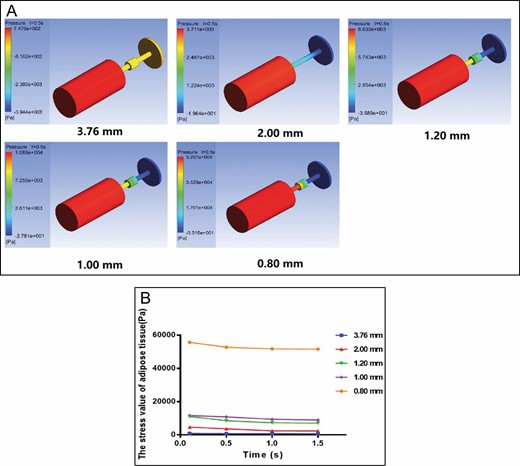
Finite element method results for the shuffling model. (A) Cloud map of stress distribution of adipose tissue in different converters and parts at 0.5 seconds. (B) Stress value in the 3.76-mm, 2.00-mm, 1.20-mm, 1.00-mm, and 0.80-mm converter group (Pa) at different times.
Structure and Oil Ratio of Emulsified Fat
The average age of the 16 donors, 0 men and 16 women, was 32.5 years (range, 25-40 years), and the average BMI of the patients was 21.9 kg/m2 (range, 19.2-24.6 kg/m2). After mechanical emulsification, we observed that the macroscopic view of the lipoaspirate changed (Figure 3A). Most of the yellow adipose tissue was preserved only with a small number of free oil droplets in the control group, 3.76-mm group, and 2.00 mm group. It is apparent that the content of oil droplets increased significantly, and the particles of adipose tissue were smaller in small-pore groups (1.20-mm, 1.00-mm, and 0.80-mm groups). These results in Figure 3B are in line with those in Figure 3A. After calculating the oil content, there was no statistical difference in oil ratio between the large-pore-diameter groups (3.76 mm, 13.6 ± 6.2%; 2.00 mm, 24.7 ± 19.5%) and the control group (9.0 ± 2.2%). However, a significant difference in the oil content was found between the small-pore groups (1.20 mm, 42.2 ± 22.0%; 1.00 mm, 81.6 ± 10.8%; 0.80 mm, 84.0 ± 14.4%) and large-pore groups (P < 0.05). Interestingly, the oil ratio of the 1-mm group was more than 3 times that of the 2-mm group with the same total hole area (Figure 3C). Taken together, these results assessing the degree of tissue damage suggest that there is an association between converters and structure of emulsified fat.
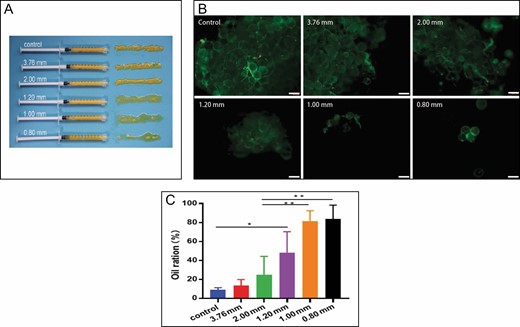
Structure and oil ratio of emulsified fat. (A) Macroscopic appearance of adipose tissue after shuffling by different converters. (B) Fluorescence microscopy of adipose tissue after calcein AM/propidium iodide staining (100×). (C) The oil ratio of different apertures of converters (*P < 0.05, **P < 0.01).
Cell Content and Viability of SVF Cells
In Figure 4, we can see the clear trend that the number of SVF cells gradually increased with the increase of apertures. There was no significant difference in number between the large-diameter group and the control group. Compared with the control group (1.23 ± 0.2 × 106 cells/mL) and the 3.76-mm group (8.6 ± 0.3 × 105 cells/mL), the small-diameter groups decreased significantly, especially in the 0.8-mm group (1.6 ± 0.4 × 105 cells/mL). The cell viability was illustrated by calculating the number of living cells/the total number of cells after staining with immunofluorescence. Figure 5A shows that green fluorescence labeled living cells and red fluorescence for dead cells. Only a very few dead cells (red) could be observed. Although the number of SVF cells in each group differed, no statistical difference was found in cell viability among the groups (Figure 5B). These results further support the result that the activity and quality of SVF do not seem affected by mechanical emulsification.9
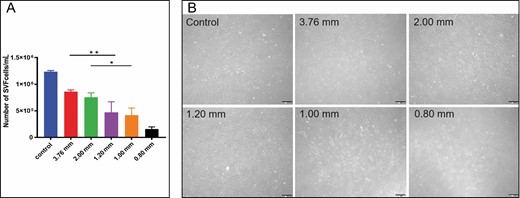
Evaluation of cell count for stromal vascular fraction (SVF) cells. (A) Total number of stromal vascular fraction cells (*P < 0.05, **P < 0.01). (B) Microscopic images under light microscope on the fifth day after culture (200×).
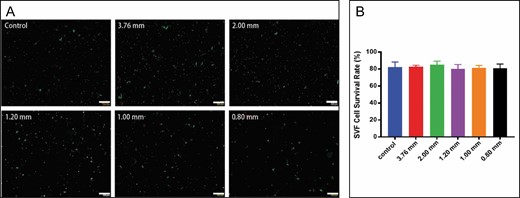
Cell viability analysis of stromal vascular fraction (SVF) cells. (A) Microscopic images of living (green fluorescence) and dead cells (red fluorescence) (200×). (B) Viability evaluation for stromal vascular fraction cells in all groups by live/dead staining.
Proportion of ADSCs, EPCs, and Other Cells in SVF Cell Population
The proportion of ADSCs (CD45−/CD31−/CD34+), EPCs (CD45−/CD31+/CD34+), and other cells (CD45−/CD31−/CD34−) in the SVF cell population was analyzed by flow cytometry.34-37 Dead cells can be excluded by 7-aminoactinomycin D staining. We observed a significant difference in the proportion of ADSCs in the 0.80-mm group (1.72 ± 1.44%) compared with the others. A possible explanation for this might be large mechanical stress. Notable was the approximately fourfold upregulation of ADSCs in the 3.76-mm group (6.30 ± 0.56%) vs the 0.80-mm group (P < 0.001) and a twofold upregulation in the 1.00-mm group (4.33 ± 2.10%) vs the 0.80-mm group (P < 0.05) (Figure 6A). For EPCs in each group, no significant difference was observed (Figure 6B), as was the same with the proportions of other cells (Figure 6C).
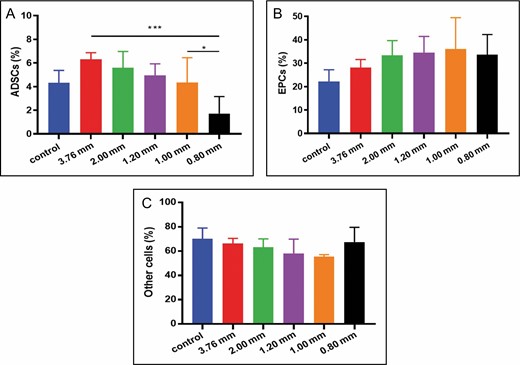
Statistical diagram of flow analysis results for stromal vascular fraction cells. (A) With the decrease of pore size, the proportion of adipose-derived stem cells (ADSCs) (CD45−/CD31−/CD34+) decreased gradually (*P < 0.05, ***P < 0.001). (B) None of these differences in proportion of endothelial progenitor cells (EPCs) (CD45−/CD31+/CD34+) were statistically significant. (C) The proportion of other cells (CD45−/CD31−/CD34−) appeared to be unaffected by apertures.
Adipogenic Differentiation Ability of SVF
The droplets of adipocytes were stained orange-red by oil red O staining and gathered in both the experimental group and the control group (Figure 7A). As shown in Figure 7B, the absorbance value of the 1.20-mm group and 1.00-mm group was significantly higher than that of the control group (P < 0.01). However, there was no significant difference among large-pore groups, the 0.80-mm group, and the control group. It has been demonstrated that ADSCs are sensitive to mechanical stimuli.38 As ADSCs are differentiated into adipocytes, they are affected by internal and external mechanical forces, which possibly contributed to our experimental results.
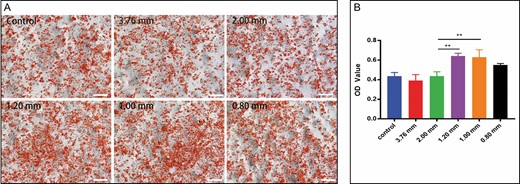
Adipogenic differentiation ability of stromal vascular fraction induced in AdipoDiff medium for 2 weeks. (A) Photomicrographs of 6 samples of stromal vascular fraction cells stained with oil red O (50×). (B) Absorbance optical density value of each group at a 520-nm wavelength (**P < 0.01).
Angiogenic Activities of SVF
Effects of different converters on angiogenesis of SVF cells were detected by test tube formation experiment. In the 3.76-mm, 2.00-mm, and control groups, SVF cells appeared to have sufficient activity to interact and achieve the formation of capillary-like structures in the matrigel (Figure 8A). After the fat was treated with a small-aperture converter (1.20 mm, 1.00 mm, 0.80 mm), it was difficult for SVF cells to form a tube-like structure; therefore, the number of tubules was significantly less than that of other groups. Differences in the results of the small converters were not significant (Figure 8B).
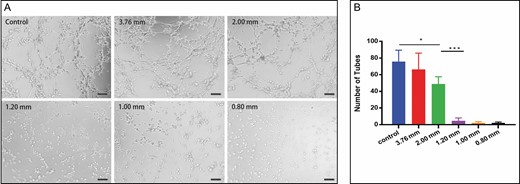
Angiogenic activities of stromal vascular fraction in vitro. (A) Photomicrograph of the differentiated stromal vascular fraction cells demonstrating tube network formation on matrigel (200×). (B) Quantification of total number of network tubules (*P < 0.05, ***P < 0.001).
DISCUSSION
The autologous fat obtained by emulsification is called nanofat, a kind of a liquid fat emulsion obtained by repeated mechanical cutting of a converter with 2 syringes. Shuffling represents one of the processing and refinement methods after fat harvesting to facilitate application and prevent uncontrolled, excessive fat injection into the recipient site.6 Nanofat is a much safer and more time-saving application to obtain SVF compared with the traditional approach by enzymatic digestion. The selection of converters is an important key in the preparation of emulsified fat. Consequently, finding a converter with a suitable aperture to improve the quality of emulsified fat is of great significance for the clinical effect of fat transplantation.
A detailed description about the influence of converters as a tool for nanofat shuffling is elaborated in our study. Overall, the smaller the aperture of the converter, the greater the mechanical force on adipose tissue. The small aperture converter will destroy the adipose tissue structure and reduce the number of SVF cells, whereas the cell activity and the proportion of EPCs are not affected by pore size. Different aperture converters had great effects on the adipogenic and tubule differentiation ability of SVF cells. Compared with the large-aperture group and 0.80-mm aperture group, 1.20-mm and 1.00-mm aperture converters enhanced the adipogenic ability of SVF cells, but the small-aperture converter weakened the ability of SVF cells to form tubes.
Osinga et al studied intersyringe processing through a 3-way stopcock (2-mm diameter) at a flow rate of 10 mL/s for 0, 5, or 30 passes. The results suggest that the mechanical procedure of shuffling lipoaspirated fat does not alter the tissue viability, its microscopic structure, and even the SVF in assessed parameters.9 As noted by Tonnard et al, mature adipocytes were significantly harmed by the shearing force when shuffling adipose tissue with a Luer fitting (3.76-mm diameter) at a flow rate of 20 mL/s, which was approximately twice the speed employed to create nanofat.6As a result, we chose to shuffle lipoaspirates at a flow rate of 2.5 mL/s and utilized a 5-cc syringe in our study. One reason for shuffling at a lower speed was the greater force needed to push syringes employing smaller converters. Through the finite element analysis, we were able to intuitively observe the stress on fat in each experimental group. With the decrease in aperture, the force on the adipose tissue increases as time goes by. Interestingly, the total hole area of the 2.00-mm group was equal to that of the 1.00-mm group, but the fat stress was less than that of the 1.00-mm group. We think the reason for this finding is that the joint structure and hole number of the 1.00-mm group was different from that of 2.00-mm group, which increases resistance to fat emulsification.
The adipose tissue after centrifugation is divided into 3 layers: the top layer is the oil layer, also named the triester glycerol layer; the middle layer is the yellow adipose tissue layer, containing a large number of adipocytes; and the lowest layer contains serum, plasma, local anesthetic, free hemoglobin, and so on. The composition and dosage of anesthetic and swelling fluid injected during liposuction are variable factors. To make experimental results more credible, we usually remove the uppermost oil layer and the lowest liquid layer to obtain the middle yellow fat layer as the experimental sample. Each group passed 30 times at the same speed to ensure the effect of the process. In our study, the adipose tissue of the control group and large-pore group showed obvious granular or yellow chylous structure, which was similar to results reported in the literature.12 As the pore size decreases, more unicameral adipocytes are destroyed to form liquid fat. Studies have shown that nanofat is more suitable for superficial injection, but a large number of oil droplets released by damaged monocular adipocytes may aggravate the inflammatory response in the graft area.17,39 Therefore, nanofat can be prepared by utilizing different pore size converters according to clinical application.
Because adipocytes are destroyed during nanofat processing, nanofat has a large number of SVF cells containing ADSCs, EPCs, and other adult stem cells. It has been demonstrated that ADSCs can differentiate into mature adipocytes and endothelial cells to maintain the volume of the graft and promote tissue regeneration.33,40 Multilineage differentiation was conducted to assess the quantity and viability of SVF. Osinga employed a 2.00-mm converter and found that the adipogenic ability of SVF cells showed no significant difference from that of the control group.9 In our results, the 2.00-mm and 3.76-mm groups did not significantly differ from the control group, consistent with the results of Osinga.9 However, the smaller aperture of the converter has an effect on SVF adipogenic differentiation ability, and the adipogenic ability of the 1.20-mm and 1.00-mm groups was significantly greater than the other groups. It is now well established that the characteristics of mechanosensitive and mechanoresponsive ADSCs can trigger their differentiation into adipocytes, mediated by changes in the cytoskeleton of ADSCs and/or various signaling pathways.38 Another important finding was that the adipogenic and angiogenic ability of the 1.20-mm and 1.00-mm groups showed the opposite trend. A possible explanation for these results may be that ADSCs are sensitive to adipogenic differentiation under the influence of mechanical force compared with vascular differentiation. Further research is needed to elucidate the mechanisms involved in adipogenic and angiogenic abilities. Early angiogenesis is essential for the survival of transplanted adipose tissue, including endothelial cell migration, proliferation, and catheterization to form vascular rings. Pericytes and EPCs are directly related to angiogenesis.41 Although there is no significant difference in the ratio of EPCs in each group, the observed difference among groups in the ratio of ADSCs was significant. The results of angiogenic experiments are basically consistent with the results of ADSC content. It has been found that mild mechanical force can increase the number of pericytes and mesenchymal stem cells in the process of fat transplantation.9 The angiogenic differentiation ability of the small-aperture group also needs to be elucidated by in vivo studies. Although this study focuses only on the analysis of differentiation capacity in vitro, the findings may well have a bearing on the shuffling of fat. One promising finding was that fat extract, a cell-free component derived from nanofat, also possesses proangiogenic, anti-apoptotic, and pro-proliferative activity.13 In fact, nanofat graft contains non-cellular components. So studies in vivo regarding the differentiation ability of small aperture group that take these variables into account will need to be undertaken. Of note, previous research has proven that the unfiltered-emulsified fat named nanofat 2.0 has a greater number of ADSCs compared with the conventional nanofat sample because filtration gives rise to the destruction of the stem cell population.12 In recent years, many nanofat derivative or analogs, such as nanofat 2.0, SVF-gel, and fat extract, have emerged based on different modified procedures of nanofat. A further focus on them could produce interesting insights for future research.
Taken together, the findings reported here appear to support the assumption that large converters with 2.00-mm and 3.76-mm-diameter holes may be a choice for nanofat graft utilized to augment limited volume and contour profile when grooves are present, whereas nanofat shuffled from 1.20-mm and 1.00-mm diameter converters is more inclined to be employed as a tissue rejuvenator for the improvement of fine wrinkles, pigmentation, or skin quality. But the nanofat obtained by the 0.8-mm aperture in our study is not recommended for clinical utilization. Combining the points maintained by Georges Ziade et al, different nanofat forms from different converters can be act as a single treatment or combined treatment through different planes of injection.17 Meanwhile, we can choose a suitable converter for nanofat transplantation according to biological functionality, which can be employed in combination with other treatments (platelet-rich plasma, growth factor, or cell therapy).
SVF is considered to be a mixed group of cell populations, which can be divided into 3 main communities according to the origin and location of cells, namely, vascular-related cells, blood-derived cells, and other cellular components. In our study, only flow cytometry was utilized to sort ADSCs and EPCs, both of which belong to vascular related cells. In fact, ADSCs are not simply a group of pluripotent mesenchymal cells but more like groups of cells at different stages of differentiation related to the maturity of adipose vessels. Therefore, the study would have been more interesting if it had explored the effect of cell surface markers on the sorted cell subsets. It is worth mentioning that the immune cells in SVF, especially macrophages, not only play an immune defense role but are also closely related to local vascularization and anti-inflammation of adipose tissue.42 So further work regarding other cellular components of SVFs would be worthwhile. Another weakness of this study is that the evaluation of multi-directional differentiation of SVF was limited in terms of the detection method, also not including osteogenesis and chondrogenic differentiation. An additional uncontrolled factor is the thrust and speed of the operator when we transferred the content of 1 syringe into the other. Surveys such as that conducted by Rik Osinga have shown that different numbers of times through a 3-way stopcock (2-mm diameter) have no impact on the SVF. Given this perspective, the numbers of transfers in shuffling with other diameters of fat converters would be a fruitful area for further work. Admittedly, this study should give sufficient consideration to the lipoaspirate process, larger samples, syringe selection, and donor parameters. Furthermore, being limited to in vitro analysis, this study needs to be further substantiated by corresponding findings in vivo. Notwithstanding these limitations, the study certainly offers some insight into performing this procedure.
CONCLUSIONS
The converters with different apertures cannot be ignored, which are responsible for the macroscopic and microscopic structure of nanofat and cell content, cellular composition, and differentiation capacity of SVF to some extent. These findings contribute in several ways to our understanding of the effect of different internal diameters of converters on shuffling and provide a basis for selection for preparation of nanofat.
Disclosures
The authors declared no potential conflicts of interest with respect to the research, authorship, and publication of this article.
Funding
The authors received no financial support for the research, authorship, and publication of this article.
Acknowledgments
The authors acknowledge the valuable insights and timely help provided by the relevant people in conducting the literature search and review. Dr Qiu and Dr Jiang contributed equally to this work as co-first authors.
REFERENCES