-
PDF
- Split View
-
Views
-
Cite
Cite
Mikaela Kislevitz, Christine Wamsley, Mason Bartels, Karen B Lu, Xingchen Li, Sydney Pinch, John Hoopman, Fritz Barton, Jeffrey Kenkel, Yucel Akgul, Clinical Translation of Scarless 0.33-mm Core Microbiopsy for Molecular Evaluation of Human Skin, Aesthetic Surgery Journal, Volume 41, Issue 11, November 2021, Pages NP1710–NP1720, https://doi.org/10.1093/asj/sjaa332
- Share Icon Share
Abstract
Skin scarring can occur after punch biopsies, prohibiting their routine utilization, especially in the central face.
This paper describes a scarless, 0.33-mm-diameter skin microbiopsy for molecular analysis of skin.
This is was single-center, randomized, prospective study with 15 patients receiving no biopsy or biopsy on the left or right nasolabial fold. Six blinded raters assessed participant photos at baseline, 1 month, and 3 months post biopsy to evaluate for a visualized scar. Patient and Observer Scar Assessment Scale was completed. Additionally, biopsies from various skin regions of body along with arm skin after treatment with a single Erbium-YAG laser were processed for molecular analysis.
No patients exhibited scar formation based on evaluation of photographs and patient feedback. There was no mark at the biopsy site 7 days post-procedure. Optical coherence tomography showed a complete closing of the biopsy-punch wound 48 hours post-biopsy. One month post-biopsy, photography reviewers were unable to identify a scar, on average, 90% of the time at 3-month follow-up. Microbiopsies from various anatomical regions were successfully extracted for histology, electron microscopy, and gene expression analysis. Selected skin rejuvenation markers in the biopsies from Erbium-YAG–treated forearm skin resulted in significant gene upregulation in extracellular matrix molecules at 1 month posttreatment compared with untreated skin.
A core microbiopsy of 0.33 mm can be extracted reproducibly for histological, ultrastructural, and gene expression analysis without scarring. This allows repeated sampling for assessment of skin treatments and diseases, including aesthetics and wound-healing progress.
The ultimate objective assessment of skin is through biopsies, which allow for ultrastructural and molecular analysis of skin, including both the epidermal and dermal layers. Molecular analysis of >2-mm skin biopsies for histology and gene expression are well-established and widely employed.1,2 Limited studies have utilized biopsies in facial aesthetics, including the finding that patients with chronically photo-damaged skin have elevated elastin and fibrillin gene expression.3 In another study, histological evaluation of skin was performed before and after laser treatments to evaluate follicular injury to the hair follicle for laser hair removal.4 In addition, our recent study effectively documented the utilization of microbiopsies to evaluate the efficacy of facial skin laser treatment for rejuvenation.5
Scarring is a potential complication that can result from routine punch biopsies, which usually measure 4 to 6 mm in diameter. Scars are generally regarded as unsightly and undesirable.6 Unlike regenerative healing, scarring or reparative healing does not replace damaged tissue with a new version of what existed before the injury. Rather, the damaged tissue is repaired with fibrous tissue comprising mostly heavy collagen fibers, with altered cellular and vascular composition.7 In the case of soft tissue repair, the scar may be raised or depressed, pruritic, erythematous, contracted, and hyper- or hypopigmented relative to intact surrounding skin.8
Healing with a scar following punch biopsy can present a significant problem in conducting histological studies and collecting quantitative outcomes data for aesthetic procedures. Although many studies select biopsy sites where small scars can be easily hidden, such as the buttock, scar development must be considered when facial sites are biopsied. Very few studies have described scarring in relation to punch biopsies.9 Furthermore, no studies to our knowledge have been published regarding the utilization of a punch microbiopsy for molecular analysis.
Facial skin is a large focus of cosmetic and anti-aging procedures. Many of the energy-based devices on the market are utilized for aesthetic facial procedures. Currently, the evaluation of the outcomes of these treatments is limited to patient-reported outcomes and patient, clinician, or observer rating scales. These subjective assessments cannot evaluate skin function and structure beyond the superficial epidermis. Because scarring limits the utilization of biopsy in analyzing the efficacy of these facial procedures, there are no validated scarless microbiopsy procedures that allow the assessment of histological or genetic changes on the face. Biopsies can be taken from behind the ear or on the periphery of the face, where the treatment may not be adequately performed and where the dermal components of this skin differ from the central face. The skin in different anatomical regions may have significant structural, biophysical, and functional differences prohibiting extrapolation of non-facial biopsies to facial biopsies. Previous studies demonstrated that the variations in anatomical, biophysical, and biomechanical properties in facial skin cause an attenuation of the laser column depths in facial skin compared with abdominal skin.9 Extracting a biopsy from the center of the face, in a densely treated area, would therefore allow more objective assessment of the efficacy of aesthetic treatments such as lasers and microneedling. In the current study, we evaluated whether a scar forms in facial skin after a 0.33-mm-diameter core microbiopsy extraction and how the microbiopsy can be employed for structural, histological, and genetic analysis of skin.
METHODS
Scar Assessment Study
The study protocols were approved by the Institutional Review Board at the University of Texas Southwestern Medical Center. This study was conducted from September 2018 through March 2019. Fifteen healthy volunteers, 9 females and 6 males, were randomized to receive no biopsy, a 0.33-mm microbiopsy 2 mm from the left alar base of the nose, or a 0.33-mm microbiopsy 2 mm from the right alar base of the nose (Figure 1B). A third (5 patients) of the participants did not receive a facial biopsy. Patients were randomized utilizing www.randomizer.org. Prior to study initiation, sealed pre-numbered randomization envelopes were provided to the research staff to obtain a randomization assignment for each patient. The randomization envelope was opened at the first study visit. At baseline, skin exams were performed to assess for hypertrophic scars, tattoos, or deformities in the area planned for the biopsy site. Ineligible patients included those with scars, tattoos or deformities, or skin disorders; were taking anticoagulants; or were pregnant or lactating.
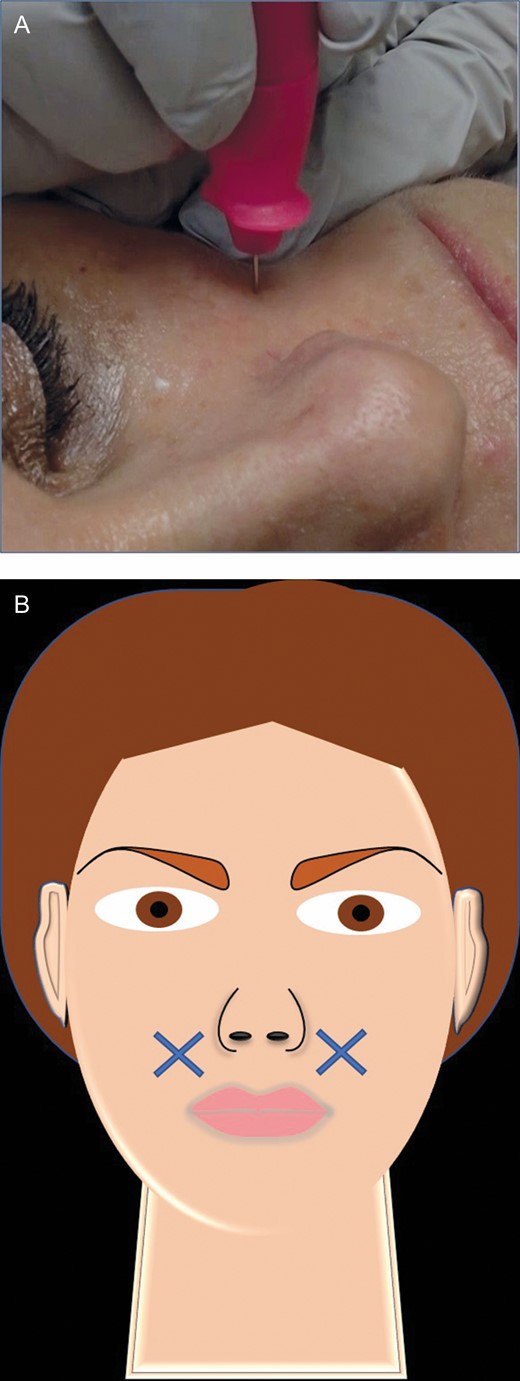
(A) Microbiopsy extraction procedure. The tip of the biopsy punch is inserted directly perpendicular to the patient’s skin, this 55-year-old female. (B) The Xs in the artwork depict the facial biopsy locations, 2 cm from the left and right alar bases.
Photographs were taken at baseline, immediately after the biopsy was performed, and at 1- and 3-month follow-up visits. In the biopsy group, the wound was examined 1 month and 3 months post-biopsy for the presence or absence of scar formation, which was assessed utilizing the Patient and Observer Scar Assessment Scale. Additionally, 6 blinded photo reviewers were asked to determine which side of the face was biopsied, with the option to select left, right, or neither. If the reviewers felt they could not make a decision based on the photo provided, they were instructed to select “cannot determine.”
2940nm Erbium-doped Yttrium Aluminum Garnet Treatment of Forearm
The forearm skin of 2 female study participants (26 and 36 years of age) was treated with 5 passes of a 2940nm Erbium-doped Yttrium Aluminum Garnet (Erbium-YAG) laser at 5 mm in diameter and 100 μm depth for each pass, resulting in a total treatment depth of 500 μm, which was confirmed with optical coherence tomography (OCT) imaging. Two areas were treated on each participant’s forearm (4 treatment areas per participant). One month following treatment, microbiopsies were collected from the treated and adjacent untreated areas. Study procedures were conducted in June and July 2020, with approval from the Institutional Review Board at the University of Texas Southwestern Medical Center.
Microbiopsy Procedure
The core facial skin microbiopsies were extracted utilizing 0.33-mm-inner diameter and 5-mm-long microbiopsy punches (World Precision Instruments, Sarasota, FL). After sanitizing the biopsy site with an alcohol wipe and chilling the skin with ice, the microbiopsy was performed by pressing the plunger down and inserting the tip of the punch biopsy perpendicular to the skin (Figure 1; Video, available online at www.aestheticsurgeryjournal.com). A popping sound indicated a punch penetration through the full thickness of skin. The biopsy pen was then pulled straight up, 90°C from the skin surface, followed by releasing the core microbiopsy into the collection tube with solutions for histology (4% paraformaldehyde), gene expression (RNA-Later), and electron microscopy (2.5% glutaraldehyde fixative).
Monitoring the Progression of Biopsy Site Healing
OCT is a real-time, 3-dimensional skin imaging system that can be employed for evaluation of skin ultrastructure.10 We utilized the VivoSight OCT (Michelson Diagnostics Inc Software version.3, Maidstone, UK) to acquire images at 1305 nm laser center wavelength with a 6-mm × 6-mm skin area and 250 frames real-time image of facial skin. The OCT probe was placed over the biopsy site to image at several time points: immediately after the biopsy and at 10 minutes, 20 minutes, and 1, 2, 3, 5, 7, 24, and 48 hours post-biopsy. This series allowed us to visualize the column created by the biopsy punch and monitor the progression of its disappearance over time.
Immunostaining
Following collection, microbiopsies were immediately fixed in 4% paraformaldehyde (Sigma-Aldrich St. Louis, MO) for 4 hours then kept in 50% ethanol until paraffin embedding. Immunostainings were conducted as described previously.11 Briefly, sections (5 μm thick) were deparaffinized and blocked with 10% normal goat serum (ThermoFisher, Waltham, MA) for 20 minutes at room temperature. Subsequently, tissue sections were incubated with one of the primary antibodies for approximately 16 hours at 4°C. Primary antibodies were biotinylated hyaluronic acid-binding protein (Cosmobio, Carlsbad, CA, HKD-BC41), Collagen I (ab6308, Abcam, Cambridge, MA), Collagen III (Abcam, ab7778), elastin (Abcam, ab2160), TUNEL (Abcam, ab66110), CD44 (ThermoFisher, PA5-21419), and Ki67 (VP-K452, Vector labs, Burlingame, CA). The slides were then washed 3 times with phosphate buffered saline and incubated with an appropriate secondary antibody solution in 10% normal goat serum for 1 hour at 25°C. The secondary antibodies are fluorescein-tagged streptavidin (1:500 dilution), Alexa Fluor 488, and 555-conjugated secondary antibodies (Ig, heavy and light chains; 1:1000 dilution; ThermoFisher). Slides were washed 3 times in phosphate buffered saline, and coverslips were mounted to the slides with Prolong Gold containing DAPI (ThermoFisher) to stain cell nuclei. Collagen and elastin sections were subject to antigen retrieval by boiling in sodium citrate buffer (10 mM, pH 6) for 20 minutes prior to blocking. TUNEL staining for apoptotic cells was conducted utilizing paraffin sections (5 μm thick) according to the protocol supplied with the Promega DeadEnd Fluorometric TUNEL System (Promega, Madison, WI). Apoptotic cells were labeled with fluorescein, and sections were counterstained with nuclear marker DAPI. Images were obtained employing a Zeiss laser scanning confocal microscope (Zeiss, Jena, Germany). ImageJ software was employed to generate individual images. Paraffin processing, embedding, sectioning, and histological staining with hematoxylin-eosin were performed as described previously.11
RNA Isolation, Amplification, and Quantitative Real-Time PCR
Total RNA was extracted utilizing Quiqen microRNeasy kit (ThermoFisher) and treated with Ambion DNase-I DNA-Free (ThermoFisher). cDNA synthesis was then performed employing iScript Reverse Transcription Supermix followed by SSO Advanced PreAmp supermix according to the manufacturer’s protocol (Bio-Rad, Hercules, CA). Quantitative real-time PCR was performed utilizing Taqman primers and an Applied Biosystems PRISM 7900HT Sequence Detection System (ThermoFisher). Aliquots of amplified cDNA were employed for each reaction and were run in duplicates. Each gene was normalized to the expression of the housekeeping genes, human ActinB. Gene expression was calculated according to the 2–ΔΔCt method as described by the manufacturer (user bulletin no. 2; Applied Biosystems).
Transmission electron microscopy (TEM) was performed as described previously with slight modifications.12 Briefly, microbiopsies were fixed in 2.5% glutaraldehyde in 0.1 M cacodylate buffer containing 0.1% ruthenium red overnight at 4°C. Tissue was then postfixed with 1% osmium in 0.1 M cacodylate buffer containing 0.1% ruthenium red for 90 minutes. Specimens were dehydrated through a graded series of ethanols (50%, 70%, 95%, and 100%) followed by propylene oxide and embedded in Epon (EMbed-812; Electron Microscopy Sciences, Hatfield, PA). Thin sections were stained with uranyl acetate and lead citrate. Sections were viewed by TEM with a FEI Co Tecnai microscope (ThermoFisher), and images were captured with a Morada 11 1-megapixel charge-coupled device camera.
Statistics
Blinded reviewer results from the control patient and biopsy patient photos at 1 month and 3 months post-microbiopsy were compared utilizing an unpaired t test. Specifically, the average percentage of correctly identified photos and the average percentage of photos deemed undeterminable were employed to compare biopsy vs control groups, respectively. Graphpad Prism Version 8 was utilized for statistical analysis. The t test with 2-tail distribution and heteroskedastic (Microsoft Excel Software) was utilized to compare gene and immunostaining results.
RESULTS
Scar Assessment Study
Study patients ranged in age from 24 to 58 years, with the mean age of 36.3 ± 15.8 (standard deviation). All 10 biopsied patients did not experience scarring at both 1 and 3 months post-microbiopsy based on study participant self-reporting. Clinical photography comparing pre-biopsy skin with post-biopsy skin at 1 month and 3 months post-biopsy failed to show scarring or any indication of injury to that skin (Figure 2). Because the patients and study observers determined that the biopsied facial skin area was free of scarring at both the 1- and 3-month follow-up visits, the Patient and Observer Scar Assessment Scales was not completed.
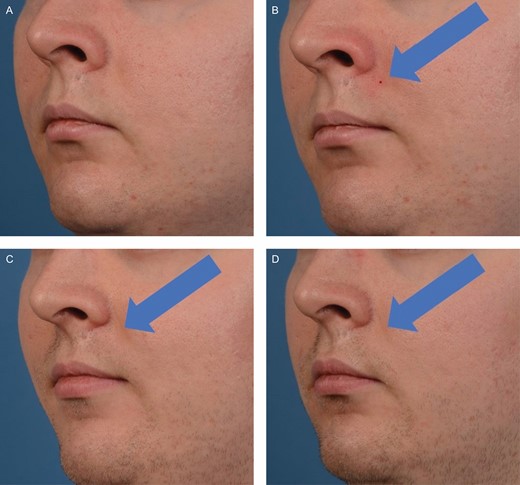
Photo progression of the biopsy site healing on this 26-year-old male. Photographs were taken immediately before biopsy (A), 1 minute post-biopsy (B), 1 month post-biopsy (C), and 3 months post-biopsy (D).
For the blinded evaluations, photos of all 15 patients were available at 1 month post-biopsy, and photos of 14 patients were available at 3 months post-biopsy. Figure 3 illustrates the averaged blinded evaluation results for both follow-up visits along with the control group of no biopsy taken. For the 1-month series, the 6 evaluators were unable to see a scar or could not determine the existence of a scar, on average, 90% of the time while correctly identifying biopsy scars on average 10% of the time at 1 month following puncture (Figure 3A). At 3 months post-procedure, the evaluators did not see or could not determine the existence of a scar on average about 98% of the time, whereas about 2% of the time scarring was correctly identified (Figure 3B). Blinded evaluators who were asked to identify a scar from patient photos identified the scar correctly 16.7% of the time at 1 month and 13.3% of the time at 3 months (Figure 3). There was no statistical significance comparing the average percentage of correct responses by blinded evaluators for the biopsy vs the control group. In addition, no statistical significance was determined comparing the average percentage of responses labeled as “cannot determine” for biopsy and control groups.
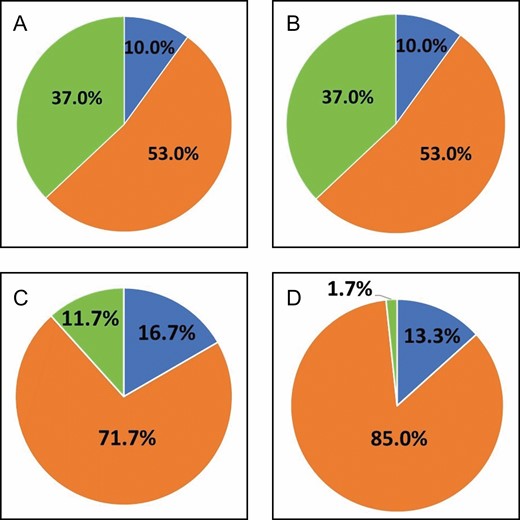
Averaged blinded evaluation results for biopsied patients at 1 month (A) and 3 months (B) follow-up and control group, who did not receive biopsy punching, at 1 month (C) and 3 months (D) follow-up. Blue, scar seen; yellow, no scar seen; orange, cannot be determined.
Monitoring the Progression of Biopsy Site Healing
The core biopsy from the skin is extracted from the wound area in the shape of a column. To document the healing progress of the biopsied area over time, a non-invasive OCT was employed for the analysis of skin ultrastructure and vasculature (Figure 4). Right after removal of the microbiopsy punch, the average 800-μm-deep column filled with blood and fibrin cloth (Figure 4, 1 minute, top row) and shrunk to a 150-μm column by 20 minutes. By 48 hours post-biopsy, the column was completely covered, and the surface of the skin returned to its normal architecture with slight redness. The biopsied area was free of blood flow at the 24-hour time point and regained the flow by 48 hours (Figure 4, bottom row).
Cross-section at 0.5-mm-depth optical coherence tomography images of 0.33-mm punch biopsy site on facial skin before biopsy (A) and 1 minute (B), 20 minutes (C), 24 hours (D), and 48 hours (E) following biopsy puncture. Blood flow at 0.5 mm depth of 0.33-mm punch biopsy site before biopsy (F) and 1 minute (G), 20 minutes (H), 24 hours (I), and 48 hours (J) following biopsy puncture. Blue arrows and circles represent wound edge.
Microbiopsy Extraction Analysis
Core skin microbiopsies were extracted with no structural damage to the sample in a consistently reproducible manner. Following the biopsy, samples were placed directly into the proper solutions for molecular processes. We successfully and reproducibly processed tissues for histology, gene expression, and electron microscopy. Over 20 paraffin sections 5 μm thick were cut for histology from a single microbiopsy, allowing evaluation of approximately 40 to 60 markers utilizing immunofluorescence staining when multiplexed as examples of markers for tissue regeneration shown in Figure 5. Gene expression evaluation with quantitative RT-PCR was completed with microbiopsies. Because of the preamplification step, it was possible to evaluate an unlimited number of genes. Figure 6 shows the proof-of-concept experiment with forearm skin samples treated with the Erbium-YAG laser. With this limited study, we recorded statistical upregulation of extracellular matrix genes (elastin, collagen 1, and collagen 3) in contrast to no statistical change in growth factors (FGF7 and VEGF) and inflammatory genes (TNF-alpha and IL10) 1 month after laser treatment compared with untreated controls (Figure 6). In addition, we have successfully processed and imaged biopsies employing a TEM as shown in Figure 7. The TEM allows visualization of skin ultrastructure at the nanometer level, including epidermal layers, dermal-epidermal junction, hair follicle structure, and extracellular matrix structures (collagen and elastin fibers).
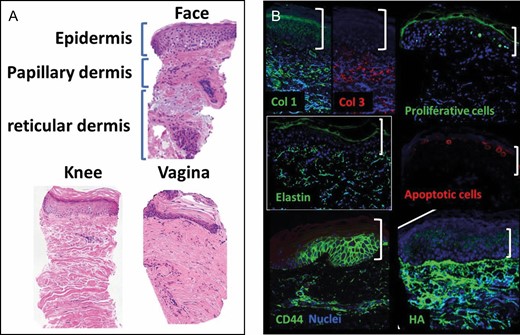
Examples of skin regeneration markers. (A) Hematoxylin and eosin staining of various skin and mucosa microbiopsies. (B) Immunofluorescence staining (green and red) of abdominal skin biopsies counter stained against nuclear marker DAPI (blue). apoptotic cells, TUNEL staining; Col 1, collagen I; Col 2, collagen 2; Elastin, CD44; HA, hyaluronic acid or hyaluronan; proliferative cells, Ki67 staining. White brackets indicate epidermis.

Relative gene expression of skin regeneration molecules 1 month following Erbium-YAG treatment. Genes of extracellular matrix molecules (COL, collagen; ELN, elastin; LOX, lysyl oxidase), growth hormones (FGF, fibroblast growth factor; VEGF, vascular endothelial growth factor), and inflammatory gene TNF-alpha. N = 5-8.
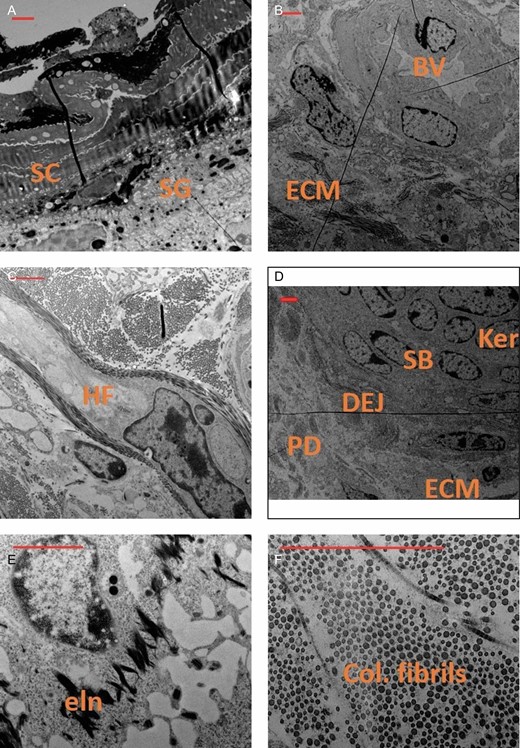
Transmission electron microscopy scanning of various regions and structures in a facial skin biopsy preparation, including epidermis (A), blood vessel (B), hair follicle (C), epidermal dermal junction (D), elastin (E), and collagen (F). Scale bars in red represents 2 µm. BV, blood vessel; col, collagen; DEJ, dermal epidermal junction; ECM, extracellular matrix; eln, elastin fibrils; Fb, fibroblast; HF, hair follicle; Ker, keratinocyte; PD, papillary dermis or subepidermis; SB, stratum basale; SC, stratum corneum; SG, stratum granulosum.
DISCUSSION
Punch biopsies are routinely employed in clinical practice to evaluate skin disease processes at the molecular level, including skin histopathology and gene makeup. Specifically, they have been utilized in dermatological studies to monitor treatment responses and understand changes in skin due to interventions. These biopsies are typically 4 to 6 mm in diameter and can potentially heal with scars. Currently, the utilization of biopsies to evaluate non-surgical outpatient aesthetic procedures is limited, especially in facial skin, as the resultant scarring would be counterproductive to these treatments. Our current study suggests that a 0.33-mm skin microbiopsy extraction is minimally invasive and results in no identifiable scar formation allowing histological, ultrastructural, and gene expression analysis.
Due to its small diameter, thinner sidewalls, and the sharp cutting edge of the microbiopsy punch, it is fairly easy to push down the punch at a 90° angle to penetrate the skin and extract a core biopsy without twisting the punch (Figure 1). The procedure causes minimal pain, because the size of a microbiopsy punch is equivalent to a 23-gauge needle. Chilling the biopsy area with ice further helps minimize discomfort.
Our study documented that the blinded evaluators were unable to determine which side of the face was microbiopsied from the high-resolution photographs taken 3 months after the procedure. Furthermore, imaging via OCT demonstrated that the column shape of the wound created by punching collapses and completely closes within 48 hours. There was no visual indication of a facial biopsy extraction 7 days after the biopsy based on standard photography.
The current study demonstrates a reproducible method of core biopsy sampling of central facial skin without scar formation, potentially eliminating a need to utilize non-facial skin to demonstrate the efficacy of facial aesthetic procedures. Due to potential complications with conventional biopsies in the facial skin, particularly the risk of scar formation, skin from other anatomical regions such as the abdomen has been widely employed to demonstrate the efficacy of various facial skin treatments.13,14 This model is believed to be advantageous because it provides a large surface area, eliminates discrepancies introduced via animal models, and the tissue is usually excised and discarded during the abdominoplasty procedure, eliminating biopsy complications such as hypo-/hyperpigmentation, pain, and scarring. However, central facial skin ultrastructure differs from the skin of these other anatomical locations. Thus, the skin at other sites may not respond to a given treatment in the same manner.9,15,16
This scarless, 0.33-mm punch microbiopsy allows for histological, ultrastructural, and gene expression analysis after cosmetic skin procedures. Previous proof-of-concept studies have successfully employed microbiopsies in skin lesions for histopathology and gene evaluation but failed to establish reproducible results. Two studies in particular highlighted the ability of a microbiopsy punch to collect a small amount of tissue to be utilized for molecular analysis without adverse effects. However, these studies were limited to a small participant pool of only 2 patients. Therefore, a larger study size is needed to confirm the reproducibility of this extraction.17,18 Recently, we have shown that core tissue extraction with a 0.33-mm skin microbiopsy for histology and gene expression is consistently reproducible and can be performed multiple times.5 In this study, we evaluated the efficacy of a single treatment with a dual wave laser to the face, demonstrating changes in epidermal and dermal ultrastructure, extracellular matrix, and immune activity in the skin as a result of treatment. In addition, we have employed this microbiopsy method to extract prepatellar core micro skin samples to assess the efficacy of radiofrequency microneedling devices (Kislevitz et al 2020, unpublished data). The microbiopsy technique is fairly simple to perform, with virtually no biopsy-related complications such as bleeding and infection. Utilizing this microbiopsy method, we have successfully extracted hundreds of microbiopsies from the skin of various anatomical locations, including the face, forearm, abdomen, knee, and vaginal mucosa (unpublished data).
The major limitation of the microbiopsy is the volume of the core tissue extraction, resulting in variation in results even if the same skin were to be sampled multiple times. For example, the biopsy may include a sebaceous gland, vasculature, or previously injured area that may not represent the gene and histological makeup of a given skin area. Because this volume of the tissue yields a very small amount of total RNA extraction (approximately 2 ng), it requires preamplification that may make small differences between samples much larger, resulting in higher variations compared with gene expression assays without preamplification. In addition, the success rate of microbiopsy processing is approximately 60% to 70% in our hands, because the microbiopsy is very delicate and requires careful extraction and handling.
In summary, this technique can allow for clinical trials to evaluate beneficial changes to skin properties, such as increased collagen expression, after facial treatments. Most importantly, it allows sequential biopsies of the central face, providing important objective data in this cosmetically sensitive region. Ultimately, this biopsy method can provide more scientific knowledge and bring more objectivity to aesthetic medicine with information about changes to skin histology and gene expression. With more clinical evidence about the effects of these potentially expensive treatments, physicians may be able to better counsel patients on the costs, risks, and benefits of these procedures.
Conclusions
A microbiopsy of 0.33 mm in diameter does not yield a detectable scar, allowing for a more objective assessment of the efficacy of aesthetic skin treatments. This novel scarless procedure could have widespread applications, particularly in evaluating the effects of cosmetic procedures or skin disorders, such as impaired wound healing, at the molecular level while minimizing unwanted adverse effects.
Acknowledgments
The authors thank Holly Mayo and Deepti Mangalagiri in the Department of Plastic Surgery at UT Southwestern for their assistance with manuscript preparation.
Disclosures
Dr Kenkel, Dr Kislevitz, Ms Wamsley, and Mr Hoopman report sponsor-supported funding from Venus Concept (Toronto, Canada) and Bellus Medical (Dallas, TX) for research studies outside of the submitted work.
Funding
Funding was provided by the Department of Plastic Surgery, UT Southwestern Medical Center.
References