-
PDF
- Split View
-
Views
-
Cite
Cite
Xiaonan Yang, Jonathan P Brower, Lauren Kokai, Beth R Gusenoff, Jeffrey A Gusenoff, A New Device for Autologous Small Volume Fat Grafting, Aesthetic Surgery Journal, Volume 41, Issue 11, November 2021, Pages NP1686–NP1694, https://doi.org/10.1093/asj/sjaa385
- Share Icon Share
Abstract
Many techniques and devices have been developed to improve small volume fat grafting efficiency and consistency for use in the operating room and outpatient procedure room. These methods require cumbersome or messy processing to remove excess oil and fluid from adipose graft.
The aim of this study was to compare the fat processing efficiency of a novel handheld device, the Push-to-Spin (P2S) system, with that of other common processing techniques and validate tissue quality after lipoaspirate processing.
Human lipoaspirate samples were processed by 1 of 3 methods: cotton gauze (Telfa) rolling, centrifugation (Coleman technique), or the P2S system. Efficiency of fat processing was evaluated in terms of total processing time, fat harvest ratio, and fat processed ratio. Histologic examination and immunohistochemical staining were used to compare tissue morphology and adipocyte viability, respectively. Experimental samples were compared with unprocessed lipoaspirate controls.
Lipoaspirate processing was significantly faster with the P2S device than with other techniques. All 3 methods achieved similar fat harvest and fat processing ratios. Additionally, the P2S, Telfa, and Coleman techniques yielded grafts with similar cellularity and with similar perilipin and glycerol-3-phosphate dehydrogenase 1 expression. Measured differences between experimental and control samples were statistically significant.
The P2S device is an easy-to-use, efficient, and potentially cost-effective handheld device that can be used for lipoaspirate harvest, processing, and grafting in any procedural setting. The resulting adipocytes have similar morphology, viability, and function to those yielded by other techniques. This handheld technology decreases procedure time, thereby improving surgeon efficiency and patient experience.
Autologous adipose tissue transfer, or fat grafting, is an increasingly common procedure in all areas of plastic surgery. Approximately 80,000 patients underwent fat grafting procedures in 2018, a dramatic increase from 2014.1 Applications for small volume fat grafting for face, foot, and hand have enjoyed particularly robust growth in both functional and aesthetic scenarios. These procedures have been undertaken in both operating room and office-based procedure room settings. This growth demands evolution of techniques in fat harvest, processing, and injection to maximize efficacy, consistency of results, and safety. Inquiries undertaken to meet these expanding needs have yielded a diversity of methods and devices, the majority of which have proven to be safe and effective. In addition to these clinical endpoints, however, it is important to consider procedure cost, efficiency (of time and personnel), convenience, and the patient experience.2
According to leading developers and practioners of small volume fat grafting, both cotton gauze (eg, Telfa) rolling and centrifugation as described by Coleman3 are the gold-standard methods for separating graftable fat from the unwanted oil and aqueous portions of the lipoaspirate prior to grafting. Each of these methods, however, has inherent disadvantages. Telfa rolling of the lipoaspirate is time consuming, limiting its use to small graft volumes. Additionally, some investigators propose that exposing the graft to air reduces graft take and increases the risk of infection as a result of oxidative damage (this phenomenon has not been observed in laboratory studies).4 Centrifugation, on the other hand, requires costly special equipment and personnel. Additionally, the force exerted on the tissue may damage the adipocytes and adipose-derived stem cells, decreasing their concentration and threatening the graft’s efficacy.5,6 Various systems for lipoaspirate processing, many of which integrate centrifugation, washing, or filtration, have been developed and brought to market.7 However, the majority of these devices are expensive and require a complex setup, including tubing, vacuum devices, and assistants.8
The aim of this study was to examine the efficiency of fat processing with the Push-to-Spin (P2S) device—a novel, all-in-one, handheld device that is used for lipoaspirate harvest, processing, and/or injection. The device was evaluated against Telfa rolling and the Coleman technique in terms of several parameters, including procedural efficiency, as well as preservation of tissue architecture and adipocyte viability and function.
METHODS
P2S Device
The concept for the P2S device was developed by the senior author. The complete P2S is shown in Figure 1. The device acts as a conventional syringe during harvest of lipoaspirate, with suction generated manually by the surgeon. A Luer lock tip permits universal integration with a variety of cannulas for both lipoaspirate harvest and graft injection. Upon harvest, the aspirate is drawn into the 10-mL inner barrel, which is lined with a filter (250-µm pore size). A shield within the device allows the vacuum to be maintained and is retracted prior to spinning. The user compresses the push-rod, inducing an in-situ 360° oscillating rotation that promotes egress of effluent (aqueous and oil components) into the outer barrel by centrifugal force. The effluent is then drained via a side port, also equipped with a Luer lock tip. The protective shield is then replaced and the remaining processed lipoaspirate can be grafted immediately through any cannula by operating the plunger rod. Alternatively, it can be transferred to a smaller syringe for grafting at the surgeon’s discretion. The device can be used repeatedly in the same patient. A video demonstrating use of the current P2S device is provided (Video).
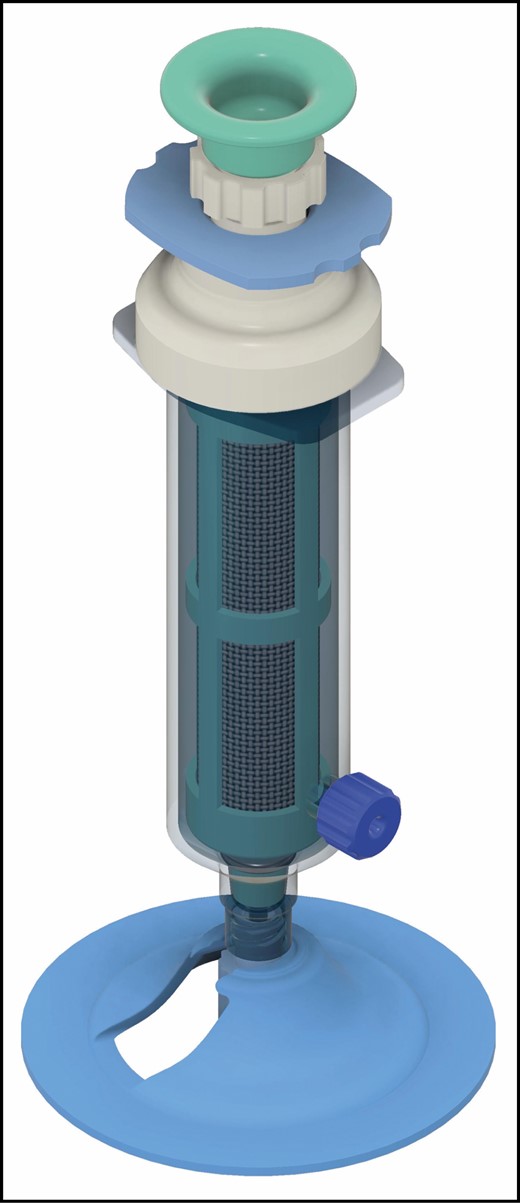
Sample Procurement and Processing
The study was approved by the University of Pittsburgh Institutional Review Board (PRO13090506) and was performed between September 2019 and January 2020. A total of 6 adipose specimens (abdominal pannus) were obtained from 6 nonconsecutive, healthy, nondiabetic, never-smoker, adult female patients undergoing a medically indicated panniculectomy. Exclusion criteria included giant pannus and active infection/panniculitis. Their mean age was 43 years (range, 27-62 years) and body mass index was 28.2 kg/m2 (range, 23.2-32.9 kg/m2). Lipoaspirate was harvested by the handheld liposuction technique with a 10-mL syringe (Becton Dickinson, Franklin Lakes, NJ) or the P2S (in the P2S group) with a Luer lock tip connected to a Khouri 12-hole harvesting cannula (#800-205; Marina Medical Inc., Davie, FL). No tumescent was infiltrated prior to lipoaspirate harvest to avoid inconsistent wetness of samples. From each pannus (6 unique donors), 8 lipoaspirate samples were harvested. Six samples were obtained with a standard 10-mL syringe, whereas 2 were harvested with the P2S device. That is, for each individual donor, 2 syringes were left inverted with no further processing (control), 2 syringes were processed with centrifugation (Coleman), 2 syringes were processed with Telfa, and 2 syringes were processed with the P2S device.
Eight lipoaspirate samples (5 mL each) were obtained from each specimen, yielding 48 total samples.
Samples in the Telfa group (12) were manually rolled over 2 Telfa gauze pads (Covidien, Mansfield, MA). Samples in the Coleman group (12) were centrifuged at 1200g (3000 rpm) for 3 minutes, with the oil and aqueous fractions evacuated as described by Coleman.3 Samples in the P2S group (12) were processed by pushing for 30 seconds at a rate of 1 to 2 pushes/second. Processed lipoaspirates from each group were transferred to 5-mL syringes (BD) for volume measurement. The remaining 6 samples were not processed any further and served as the control. The time spent processing fat was recorded for each group.
A 1-mL portion of processed lipoaspirate was sampled from one 5-mL syringe of each group for histologic exam. The other 5-mL syringe from each group underwent high-speed centrifugation (HSC) at 2000g for 5 minutes before they were treated with liquid nitrogen and stored at –80°C.
Processing Efficiency
The fat harvest ratio was calculated by dividing the volume of processed fat by the original volume (5 mL) and multiplying by 100%. The fat process ratio was calculated by dividing the volume of fat after HSC by the volume of fat prior to HSC and multiplying by 100%.
Adipocyte Viability
To determine adipocyte viability, frozen samples were thawed to room temperature. Then, 300 mg lipoaspirate of each sample was treated with 1 mL 1× cell lysis buffer (Cell Signaling Technology, Inc., Danvers, MA). The resulting lipoaspirate solution was stirred, then centrifuged at 3000 rpm for 10 minutes. Supernatant liquid was harvested and total glycerol-3-phosphate dehydrogenase 1 (GPDH) was quantified by enzyme-linked immunosorbent assay (MyBioSource, Inc., San Diego, CA).
Histology With Hematoxylin-Eosin and Perilipin
To assess the morphologic and cellular characteristics of harvested adipocytes, histologic examination with hematoxylin-eosin (HE) staining and perilipin immunohistochemistry were performed on all samples. Lipoaspirate samples (1 mL) were placed in 10% formalin for 48 hours, embedded in paraffin, and sectioned at 5 µm. Two sections of each sample were examined for architectural disruption. Histologic evaluation of adipocyte lobules was performed, as well as cell count per randomly selected high-powered (×200) field with ImageJ software (National Institutes of Health, Bethesda, MD). Immunohistochemistry for adipocyte viability was performed with the antibody against perilipin (NB110-40760, Novus Biologicals, Littleton, CO; BA-1000, Vector Laboratories, Burlingame, CA) and Hoechst 33342 (1:500; MedChemExpress LLC, Monmouth Junction, NJ) for nuclear staining after antigen retrieval with 10 mM sodium citrate buffer pH 6.0 at 97 ± 1°C for 45 minutes. Semiquantitative analysis of perilipin immunofluorescent density per randomly selected high-powered (×100) field was also performed with ImageJ software. All histologic measurements and descriptions were made by 2 independent blinded observers.
Statistical Analysis
All data are presented as mean [standard deviation] for all groups. Groups were compared by one-way analysis of variance performed with GraphPad Prism software (GraphPad Software, Inc., San Diego, CA). Statistically significant differences were determined as P values <0.05.
RESULTS
Evaluation of Processing Time and Efficiency
The mean lipoaspirate processing time with the P2S device was 35 [0.58, range 34-36] seconds vs 61.5 [3.69, range 54-65] and 200.83 [1.46, range 200-204] seconds, in the Telfa rolling and Coleman techniques, respectively. This difference was statistically significant (P < 0.01). The fat harvesting ratio and fat process ratio in the P2S group (48% [17%] and 84% [8%]) had no significant difference (P > 0.05) vs the Telfa (50% [13%] and 93% [4%]) and Coleman groups (61% [20%] and 92% [2%]). Compared with the experimental groups, the control group had greater efficiency of fat harvesting (100%, P < 0.01) but a lower fat process ratio (59% [16%], P < 0.01), as shown in Figure 2.
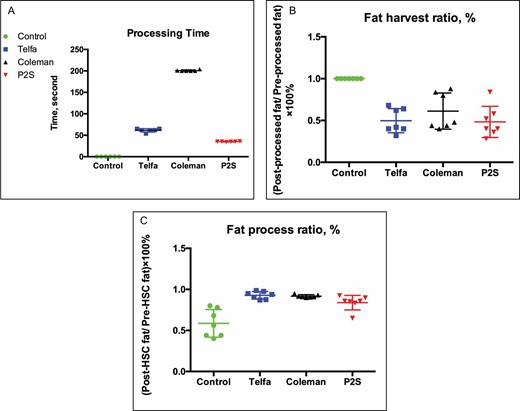
Plot of lipoaspirate sample (A) processing time, (B) fat harvest ratio, and (C) fat process ratio. *P < 0.01 vs control, ▲P < 0.01 vs Telfa, ▼P < 0.01 vs Coleman.
Histologic and Immunohistochemical Assessment of Lipoaspirate
Evaluation of HE-stained sections demonstrated adipocytes more densely packed with more fibrous connective tissue fragments observed in the experimental groups than in the control group, as shown in Figure 3. The experimental groups had a higher mean cell count per high-powered field than the control group (P < 0.0002), with 77.33 (range 40-119) in the Telfa group, 69.67 (range 31-104) in the Coleman group, 66 (range 29-121) in the P2S group, and 49.17 (range 18-86) in the control group. All processing methods yielded more intact adipocytes per cross-sectional area than the unprocessed control (P < 0.0002). Mean adipocyte cross-sectional areas were 4110.81 [1974.58, range 729.355-4584.121], 3337.54 [1581.9, range 779.273-6215.694], 3498.51 [1525.06, range 1198.028-8410.23], and 4110.81 [1974.58, range 1365.345-10462.41] µm2 in the Telfa, Coleman, P2S, and control groups, respectively. Additionally, the cells processed by Telfa rolling had a significantly smaller cross-sectional area than the cells in the unprocessed control, Coleman and P2S groups (P < 0.0001), as shown in Figure 4.
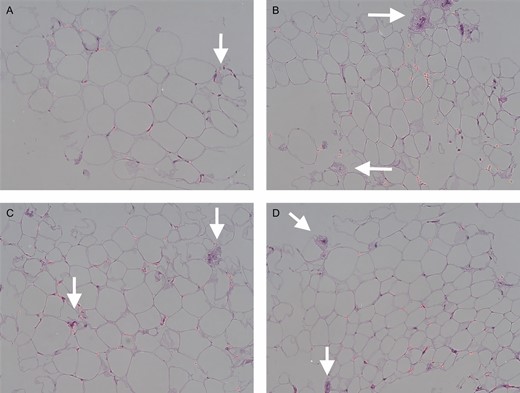
Hematoxylin-eosin staining of paraffin sections of lipoaspirates. Control group (A), Telfa group (B), Coleman group (C), and P2S group (D). Arrow, fibrous connective tissue. Scale bar, 50 μm.
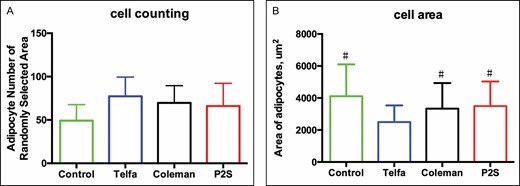
Histologic evaluations of processed lipoaspirate, including cell count (A) and area per randomly selected high-power field (B). *P < 0.0002 vs control group. #P < 0.0001 vs Telfa group.
Immunofluorescence staining resulted in detection of more polygonal perilipin-positive viable adipocytes and Hoechst-positive cells in the 3 experimental groups than in the control group. Some adipocytes with diminished or absent fluorescence were noted in all groups (Figure 5). Semiquantitative expression of perilipin was higher in the experimental groups (10.85 [2.26], 11.17 [2.88], and 11.7 [3.45]) than in the control group (9.35 [2.15]), but this difference was not statistically significant (Figure 6).
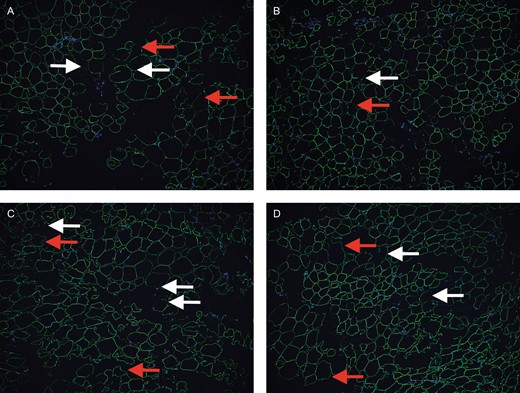
Immunofluorescence of specimens. Control group (A), Telfa group (B), Coleman group (C), and P2S group (D). White arrow, fragmentary adipocyte; red arrow, weakly perilipin-positive adipocyte. Scale bar, 50 μm.
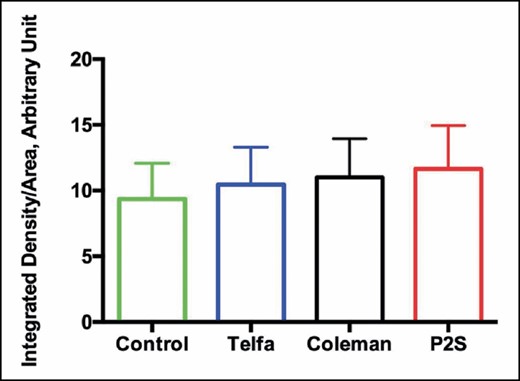
Semiquantitative analysis of immunofluorescence density performed with ImageJ software.
GPDH Expression Analysis
Adipocyte metabolic activity was evaluated quantitatively by measuring the concentration of GPDH, a cytosolic enzyme that is activated in functional adipocytes. GPDH levels tended to be higher in samples processed by the Coleman technique and with the P2S system (1.43 [1.1] and 1.57 [1.25] ng/mL, respectively) than in the Telfa and control groups (1.21 [0.9] and 1.28 [0.95] ng/mL, respectively), but this difference was not statistically significant (Figure 7).
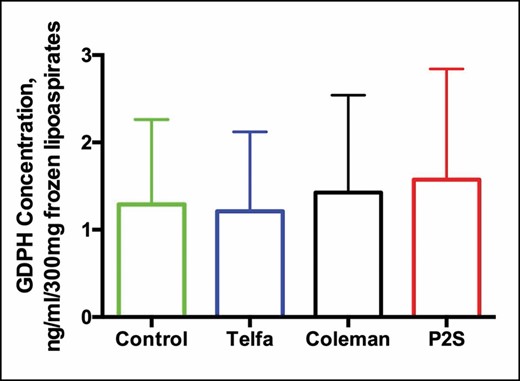
Glycerol-3-phosphate dehydrogenase 1 concentration of frozen processed lipoaspirates in control, Telfa, Coleman, and P2S groups.
DISCUSSION
Various techniques and devices have been developed for lipoaspirate processing, the critical step in autologous fat grafting that eliminates aspirated aqueous fluid, free oil, cellular debris, and hematogenous cells. Centrifugation is perhaps the most common method for fat graft processing, with the Coleman technique hailed as the standard against which most comparisons are made.9 However, some surgeons caution against potential damage to adipocytes and adipose-derived stem cells resulting from high centrifugal forces, which may reduce the concentration of these cells and compromise outcomes.10,11 The centrifuge equipment is costly and bulky, limiting its portability and widespread access among small surgery centers and offices.12 Additionally, it typically requires an assistant to maximize procedural efficiency.
Other handheld devices for lipoaspirate processing include LipiVage and LipoGrafter. The LipiVage system is designed for small volume fat grafting, but its use is somewhat time consuming, and it requires both accessories and an assistant. The LipoGrafter is quick to use, but is not specially designed for small volume fat grafting, and also requires accessories and an assistant for use.
We sought to evaluate the implementation of a novel lipoaspirate processing device and examine the physiology of the resulting adipose tissue. Our data suggest that the P2S device permits fat processing more quickly than 2 of the most common such methods, namely Telfa rolling and Coleman centrifugation. This reduction in time does appear to translate into increased procedural efficiency; the device achieves fat harvesting and process ratios similar to these conventional techniques. This efficiency will expedite procedures in both the operating room and office-based procedure room. The same device can be used repeatedly in the same patient without any required additional equipment or disposables, other than sterilizable cannulas. These features will likely improve the patient experience and reduce costs.
Histologic examination of fat processed with the P2S device shared several important features with fat processed by standard techniques. First, there were remarkable similarities in the tissue architecture among samples from all experimental groups, with comparable numbers of adipocytes per high-powered field. These findings corroborate prior studies showing that Telfa rolling and centrifugation are equivalent in their ability to concentrate the fat fraction.4 The dense cellularity and prevalence of fibrous connective tissue among all 3 experimental groups starkly contrasted the appearance of unprocessed lipoaspirate controls. It has been proposed that greater prevalence of fibrous connective tissue may provide a better scaffold for graft retention.13 Further studies are necessary to define the relation between lipoaspirate histology and clinical fat grafting outcomes.
Immunohistochemical assays reflective of adipocyte viability and metabolic activity provide important insight into the cells’ biology during lipoaspirate processing. Adipocyte viability, as measured by perilipin expression, appeared to be better in the experimental groups than in the controls. However, this difference was not statistically significant. GPDH expression, indicative of adipocyte function, confirmed the perilipin results. These findings support the widespread use of Telfa rolling and Coleman centrifugation for lipoaspirate processing because they appear to have no appreciable impact on adipocyte viability or function. The P2S device appears to similarly preserve cells during lipoaspirate processing. Importantly, our methods included freezing the samples, which could influence overall adipocyte viability. We also did not investigate other procedural variables that may influence adipocyte viability or function. The harvest technique and cannula, mechanical forces in graft handling, and overall time to graft may affect adipocyte viability regardless of lipoaspirate processing method employed.14,15
The P2S device builds on the merits of the Coleman technique, incorporating gentle centrifugation into a portable, handheld, intuitive, all-in-one device that requires few additional resources in personnel or equipment. Its small size and simple design make it user friendly and permit its use by a solo surgeon without an assistant. It reduces the use of office consumables (eg, syringes, syringe caps, neuro patties) necessary in the Telfa and Coleman techniques. These features, in addition to its procedural efficiency, highlight the device’s potential cost-effectiveness.
The P2S device also allows the surgeon to customize the consistency of fat grafts. In an effort to standardize the centrifugal force exerted on lipoaspirate in our methods, we used a standard processing protocol involving 30 seconds of spin time. However, angular (rotational) velocity and duration of processing may be adjusted to achieve the desired texture. Although gentle oscillation may damage the adipocytes, it stands to reason that such damage might be less severe than would be expected after HSC as employed in the Coleman technique. More gentle oscillation may result in better-quality fat, which merits investigation in future studies.
Forces generated by suction and injection were not evaluated because these parameters are controlled by the user, just as in any handheld small volume fat grafting technique. However, because the internal chamber of the P2S device is composed of a modified 10-mL syringe, the force of suction should be similar to that applied during standard handheld liposuction procedures. It is important to note that the data presented in this proof-of-concept study were obtained without the use of tumescent to limit variables to be considered in our evaluation. Future studies, including clinical trials, will seek to define parameters for optimal use, such as ideal filter pore size, push speed, and processing time. One important caveat is that those future investigations may not elucidate superiority in efficacy of engraftment or adipose-derived stem cell preservation, as these endpoints rely on a number of technique- and subject-related variables.16
An additional benefit of the P2S system is that the final volume of the fat graft is clearly visible to the surgeon because fat processing occurs within the device’s inner chamber, which incorporates a 10-mL syringe. This precision in volume measurement minimizes the possibility of harvesting and processing too much or too little lipoaspirate. The 10-mL syringe, however, does limit the device’s utility in procedures involving large volumes of fat transfer. The same device is intended to be used for sequential rounds of grafting in the same patient during a single session, but the number of uses a single device can safely provide will be determined once a manufactured product is available for testing. Recent iterations of the device have scaled the internal syringe to a volume of 20 mL, which may be appropriate for intermediate volume procedures. Further developments include mechanical modifications that permit continuous spinning 360° in one direction with the push of a button, rather than oscillating in a stand with the current device.
CONCLUSIONS
The P2S device is an easy, efficient, and likely cost-effective handheld device for autologous fat grafting; it permits lipoaspirate harvest, processing, and grafting in a single closed system. Cellular and histologic studies demonstrate that it results in processed lipoaspirate that is equivalent to that yielded by common techniques in standard practice, including Telfa rolling and Coleman centrifugation. Further studies are needed to evaluate parameters to achieve optimal clinical results.
Disclosures
Dr J Gusenoff and Dr B Gusenoff are co-inventors of the Push-to-Spin (P2S) (Pittsburgh, PA) device and hold intellectual property with the University of Pittsburgh. Patent is pending. This intellectual property does not hold any financial implications at this time.
Funding
The authors received no financial support for the research, authorship, and publication of this article.
REFERENCES