-
PDF
- Split View
-
Views
-
Cite
Cite
Davide De Battisti, Plant biomass allocation advances our understanding of plant adaptation to environmental gradients: a commentary on ‘Contrasting biomass allocations explain adaptations to cold and drought in the world’s highest-growing angiosperms’, Annals of Botany, Volume 134, Issue 3, 1 September 2024, Pages i–iii, https://doi.org/10.1093/aob/mcae069
- Share Icon Share
Plants must compete for resources such as nutrients, water and light that may be scarce or heterogeneously distributed. Specific organs are of course devoted to this purpose: leaves harvest solar energy to fix carbon dioxide, stems provide mechanical support, and roots take up nutrients and water below-ground. There is extensive coverage in the literature concerning the relative investment into different plant organs, referred to as biomass allocation, which allows for an understanding of how species optimize their responses to the environment according to limiting factors, assemble in communities and ultimately can coexist (e.g. Enquist and Niklas, 2002; MacCarthy and Enquist, 2007, Poorter et al., 2012, 2015). Allocation of resources also influences critical plant processes underlying responses to climate change, such as carbon storage.
Two main theories have been proposed to explain plant biomass allocation (see Poorter et al., 2012): the optimal partitioning theory (OPT) and the allometric partitioning theory (APT). According to the OPT, plants preferentially allocate biomass to the organ devoted to acquiring a specific, limiting resource. For instance, under low light conditions, the OPT predicts that plants will invest more in leaf and stem mass fractions (LMF and SMF, respectively) than in root mass fraction (RMF) to increase light interception and thus photosynthesis (Poorter et al., 2012). In contrast, under low nutrient conditions plants will preferentially invest in RMF instead of LMF and SMF, to explore a larger soil volume and therefore increase nutrient uptake (Poorter et al., 2012; Freschet et al., 2018). In the APT, biomass allocation to organs depends on biophysical constraints (e.g. vessel number and diameter for water and nutrient transport) and is fixed across species, across biomes and along environmental gradients (Enquist and Niklas, 2002). Specifically, the APT predicts that leaf biomass scales as a 3/4 power of the biomass of both stem and root, while stem scales isometrically with root biomass (Enquist and Niklas, 2002).
Both theories have found empirical support when analysing global datasets (e.g. Enquist and Niklas, 2002, Poorter et al., 2012, 2015; Puglielli et al., 2021). Poorter et al. (2012) provide evidence for the OPT across a wide range of species, showing that in arid environments species invest more biomass in RMF, while shade-tolerant species invest more in stems to increase light interception and thus competition. In another global-scale analysis, MacCarthy and Enquist, (2007) found strong support for the APT across species, but they also suggested that the OPT could explain part of the variation in biomass allocation at the intraspecific level. They highlighted that biomass allocation within species can respond differently to the same environmental gradient, which would mask any OPT signal arising from species differences. Overall, despite the focus in this field of research, we still do not fully comprehend the patterns of biomass allocation across different species along environmental gradients, particularly at smaller, regional scales. This scale of investigation is critical for habitat and ecosystem management, conservation, and estimation of ecosystem services.
Given this context, the study of Doležal et al. (2024) investigating plant biomass allocation in the Himalayas pushes forward our knowledge of biomass allocation at relevant regional scales. The authors focused on three different habitats, namely alpine, dry steppe and wetland, along with strong temperature and precipitation gradients related to the extreme heights of these mountains. Doležal et al. (2024) found marked deviations from the APT in all three investigated habitats. Alpine plants preferentially allocated biomass to leaves over stems or roots, while in both wetland and dry steppe plants preferentially allocated biomass to roots or below-ground stems and rhizomes (thus, overall deviating from the theoretical 3/4 power law). Additionally in wetland plants, biomass investment in stems and roots deviated from isometry, with a preferential investment in below-ground compartments.
Although plants follow general allometric rules in biomass allocation across a large range of species (Enquist and Niklas, 2002), the study of Doležal et al. (2024) highlights that the OPT better reflects plants’ adaptation to the specific requirements of their environment. In cold, alpine habitats, plants preferentially invested biomass in LMF, SBF, reproductive fraction and rhizome mass fraction. These large below-ground structures are sustained by investing in LMF during the short summer period, allowing plants to maintain adequate photosynthesis levels. In contrast, plants growing in dry steppe invested more in SMF and RMF, common plant responses in dry soil to explore large volumes and thus acquire more resources (i.e. nutrients and water; Poorter et al., 2012; Reich, 2014; Ma et al., 2017; Puglielli et al., 2021). Wetland plants preferentially invested in SMF and RMF, to increase competition for light and resources, respectively. Similarly to these overall patterns, Doležal et al. (2024) also found that climate, i.e. temperature and drought, can influence biomass allocation, but the pattern of allocation is habitat dependent. For example, wetland and dry steppe plants preferentially invested in stem and root mass fraction, while alpine plants reduced allocation to above-ground fractions. Altogether, Doležal et al.’s (2024) results highlight that different ecosystems under the same environmental change, or the same ecosystem in facing different intensities of the same stress, may exhibit contrasting responses. Thus, managers should consider these smaller, regional-scale responses to optimally manage or restore ecosystems and their valuable services.
Plant responses to drought are good examples to highlight the relevance of considering biomass allocation at regional (or smaller) scales for optimal management and restoration (Fig. 1). The decrease in above-ground fraction in favour of below-ground fraction under more arid conditions appears to be a general pattern observed in herbaceous plants living at high altitudes (Ma et al., 2017; Doležal et al., 2024), trees (Puglielli et al., 2021) and across a wide range of species (Poorter et al., 2012; Eziz et al., 2017; Doležal et al., 2024). These biomass shifts within species and/or at the community level probably result in a more conservative ecological strategy (Reich, 2014), where resources (e.g. nitrogen and carbon) are conserved for longer periods within plant tissues. Thus, biomass shifts under an increasing drought may ultimately alter biogeochemical cycles and carbon storage. Yet, the work of Doležal et al. (2024) indicates that these changes will probably be habitat- and region-specific. For instance, in their study, drought (viewed as lower precipitation) leads to a higher biomass investment in roots in both wetland and steppe plants but to a lower biomass investment in roots in alpine plants. Therefore, the expected alteration in biogeochemical cycles and carbon storage under drought is not uniform but it may need to be reviewed in this light. Further studies such as that by Doležal et al. (2024) will provide us with the crucial knowledge to understand future habitat dynamics under climate change and, possibly, on how to intervene to ensure ecosystem persistence.
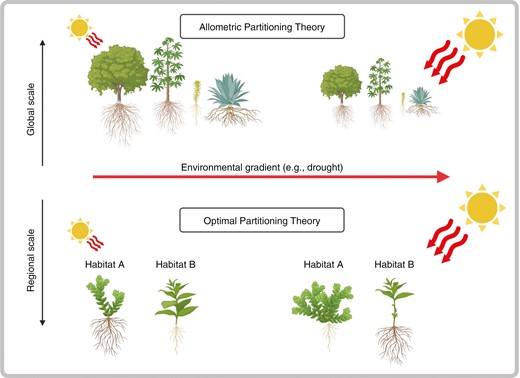
Schematic representation of the allometric partitioning theory (APT) at the global scale and optimal partitioning theory (OPT) at regional scales. At the global scale, the APT predicts that across plant species biomass allocation along a gradient reflects allometric relationships between organs (i.e. leaf scales with a 3/4 power law with respect to both stem and roots, while stem scales isometrically with roots). In contrast, at smaller, regional scales, the OPT predicts that plants allocate biomass towards the limiting factor (e.g. roots under drought conditions). Yet, Doležal et al. (2024) highlight that this biomass investment can vary depending on the habitat considered (e.g. wetland and dry steppe plants allocate more biomass below ground, while alpine plants invest less biomass above ground). Note, plants represented in the figure are only explicative and do not represent the species investigated by Doležal et al. (2024). Figure created with BioRender.com.
ACKNOWLEDGEMENTS
I am grateful to Laurie Anne Wocjik and the editors – Renata Cantoro, Erik Murchie, and Rowan Sage – for their valuable comments that improved this commentary.