-
PDF
- Split View
-
Views
-
Cite
Cite
Marie Voillemot, John R. Pannell, Maintenance of mixed mating after the loss of self-incompatibility in a long-lived perennial herb, Annals of Botany, Volume 119, Issue 1, January 2017, Pages 177–190, https://doi.org/10.1093/aob/mcw203
- Share Icon Share
Abstract
Background and Aims Many hermaphroditic plants avoid self-fertilization by means of a molecular self-incompatibility (SI) system, a complex trait that is difficult to evolve but relatively easy to lose. Loss of SI is a prerequisite for an evolutionary transition from obligate outcrossing to self-fertilization, which may bring about rapid changes in the genetic diversity and structure of populations. Loss of SI is also often followed by the evolution of a ‘selfing syndrome’, with plants having small flowers, little nectar and few pollen grains per ovule. Here, we document the loss of SI in the long-lived Spanish toadflax Linaria cavanillesii, which has led to mixed mating rather than a transition to a high rate of selfing and in which an outcrossing syndrome has been maintained.
Methods We performed crosses within and among six populations of L. cavanillesii in the glasshouse, measured floral traits in a common-garden experiment, performed a pollen-limitation experiment in the field and conducted population genetic analyses using microsatellites markers.
Key Results Controlled crosses revealed variation in SI from fully SI through intermediate SI to fully self-compatible (SC). Flowers of SC individuals showed no evidence of a selfing syndrome. Although the SC population of L. cavanillesii had lower within-population genetic diversity than SI populations, as expected, population differentiation among all populations was extreme and represents an FST outlier in the distribution for both selfing and outcrossing species of flowering plants.
Conclusions Together, our results suggest that the transition to SC in L. cavanillesii has probably been very recent, and may have been aided by selection during or following a colonization bottleneck rather than in the absence of pollinators. We find little indication that the transition to SC has been driven by selection for reproductive assurance under conditions currently prevailing in natural populations.
INTRODUCTION
Most flowering plants are hermaphroditic, with both male and female functions in the same flowers (Darwin, 1877; Barrett, 2002; Charlesworth, 2002). Although hermaphroditism may allow self-fertilization, numerous strategies have evolved to promote outcrossing (Darwin 1877; Barrett, 2003; Karron et al., 2012). These strategies include both morphological and phenological adaptations involving the spatial (Webb and Lloyd, 1986) and temporal (Bertin and Newman, 1993) separation of the two sexual functions, as well as molecular self-incompatibility (SI) mechanisms that prevent fertilization following self-pollination (Franklin-Tong, 2008; Shimizu and Tsuchimatsu, 2015). Yet the loss of outcrossing mechanisms such as SI represents perhaps the most frequent shift to have taken place in angiosperm evolution (Stebbins, 1974; Igic et al., 2008). Seeking reasons for these shifts and understanding their consequences have been pre-eminent goals in our quest to understanding plant reproductive evolution (Barrett, 2002).
Two main reasons have been hypothesized for the breakdown of outcrossing mechanisms in plants. First, because selfing immediately allows a plant to transmit two copies of its genome to its seed progeny rather than just one, there should be automatic selection of mutations that increase the frequency of selfed progeny (Fisher, 1941), as long as the quality of offspring is not overly compromised by inbreeding depression (Charlesworth and Charlesworth, 1987) and selfing does not compromise outcross siring success (i.e. there is no pollen discounting; Harder and Wilson, 1998). The maintenance of outcrossing in the face of automatic selection for selfing likely attests to the high levels of inbreeding depression that characterize outcrossing species (Lande and Schemske, 1985; Husband and Schemske, 1996; Goodwillie et al., 2005; Winn et al., 2011).
Second, selfing may be selected under conditions where outcrossing is difficult. Being able to self-fertilize allows a plant to reproduce in the absence of mates or pollinators (Jain, 1976; Lloyd and Schoen, 1992). Reproductive assurance provides the most plausible reason for the breakdown of outcrossing mechanisms, and there is growing empirical support for it (Herlihy and Eckert, 2002; Elle and Carney, 2003; Kalisz and Vogler, 2003; Moeller and Geber, 2005; Eckert et al., 2006; Busch and Delph, 2012). For example, Kalisz and Vogler (2004) found an increase in the rate of selfing and fruit production in the herb Collinsia verna in conditions of low pollinator availability. The enrichment of self-compatibility (SC) on islands colonized by long-distance dispersal provides indirect support for the loss of outcrossing mechanisms in the absence of mates (Baker, 1955, 1967; McMullen, 1987; Webb and Kelly, 1993), as does the observation of increased selfing at the margins of a species’ range (Busch, 2005; Michalski and Durka, 2007; Mimura and Aitken, 2007), and the greater range sizes of selfing species than their outcrossing relatives (Grossenbacher et al., 2015; Pannell et al., 2015; reviewed in Pannell, 2015).
Whatever its initial cause, once a transition to increased self-fertilization has occurred, we expect rapid changes in the distribution of genetic diversity (Hamrick and Godt, 1996; Wright et al., 2008, 2013) and floral traits (Ornduff, 1969). Because a transition to selfing increases homozygosity and reduces effective recombination (Nordborg and Donnelly, 1997), we expect and observe a loss of diversity within populations (Hamrick and Godt, 1996; Charlesworth and Pannell, 2001), an increase in genetic differentiation among populations (Glémin et al., 2006; Duminil et al., 2009) and changes in genome organization and sequence, such as a reduction in genome size or an increase in linkage disequilibrium (Wright et al., 2008; reviewed in Shimizu and Tsuchimatsu, 2015). Transitions to selfing can also reduce the capacity of a population to respond to selection in a changing environment (Morran et al., 2009), potentially providing an explanation for the observed increase in extinction probability of plant lineages that have lost their SI system (Stebbins, 1974; Wright et al., 2007; Goldberg et al., 2010; Wright and Barrett, 2010).
Species that have evolved a selfing strategy typically also show changes in the size, morphology and phenology of their flowers (Ornduff, 1969; Fenster and Armbruster, 2004; Goodwillie et al., 2010; Sicard and Lenhard, 2011). Plants that no longer rely on pollinators for their reproduction should reduce allocation to pollinator attraction and reward through decreases in flower size, floral scent and nectar production, as well as in the ratio of pollen grains to ovules (Cruden, 1977; Charlesworth and Charlesworth, 1981). This ‘selfing syndrome’ has been observed frequently in populations with increased selfing rates (Goodwillie et al., 2010), and can evidently evolve rapidly (Bodbyl Roels and Kelly, 2011). Bodbyl Roels and Kelly (2011) prevented pollinators from accessing flowers in experimental populations of the SC plant Mimulus guttatus and observed reduced anther–stigma separation in only five generations, and several studies have shown similarly rapid changes in floral size in response to artificial selection (Worley and Barrett, 2000; Lendvai and Levin, 2003; Delph et al., 2004). Given the rapidity of such responses, we should perhaps not be surprised that a selfing syndrome was able to evolve following the loss of SI in the selfing species Capsella rubella over several thousand generations (Foxe et al., 2009; Slotte et al., 2012).
Although apparently common, the evolution of a selfing syndrome following the loss of an outcrossing mechanism is not inevitable (e.g. Busch, 2005; Zhang et al., 2005; Fenster and Martén-Rodríguez, 2007; Dart et al., 2012). Indeed, Fenster and Martén-Rodríguez (2007) reported that many species with relatively high selfing rates nevertheless maintain many of the hallmarks of outcrossing, including showy flowers, high pollen/ovule ratios and substantial nectar production. Most of these species appear to have specialized pollinators, and almost all show delayed selfing, with self-fertilization occurring when opportunities for outcrossing have been exhausted (Schoen and Lloyd, 1992). As Fenster and Martén-Rodríguez (2007) point out, such a syndrome of SC, intermediate selfing rates and traits otherwise associated with outcrossing probably represents a response to selection for reproductive assurance in populations that usually enjoy high pollinator visitation. However, it is less obvious why SC species that fail to delay their self-fertilization should maintain attractive flowers, particularly in those lineages that were previously SI (though see below).
Traits promoting outcrossing appear to have been maintained in a number of species despite loss of SI. For example, outcrossing and the attraction and reward of pollinators appear to have been maintained in some populations of the plant Leavenworthia alabamica following the loss of SI, despite the absence of a delayed mode of self-fertilization, whereas other populations of the same species have evolved a selfing syndrome (Lloyd, 1965; Busch, 2005). Similarly, North American populations of Arabidopsis lyrata that have lost SI continue to display flowers with no reduction in size and no evidence of traits that would promote only delayed selfing (Mable et al., 2005; Hoebe, 2009), as have a number of populations of the North American plant Camissoniopsis cheiranthifolia (Raven, 1969; Dart et al., 2012). The loss of SI in these species has led neither to the evolution of selfing and a selfing syndrome nor to the evolution of mechanisms that would delay selfing under continued investment in outcrossing.
The examples just cited represent revealing case studies of within-species variation in annual or short-lived perennial plants in which SI has broken down and populations have come to differ in their mating strategies. Here, we document the breakdown of SI in the long-lived perennial toadflax Linaria cavanillesii, in which there also appears to have been no accompanying transition towards a selfing syndrome. Linaria cavanillesii, a plant endemic to southeastern Spain, was recently described as SC on the basis of crossing experiments performed on one of its populations (Carrió et al., 2013). We were surprised by this finding, as individuals produce large, showy flowers with copious nectar. Our investigations of other populations of the species reveal that the species is in fact largely strongly SI, likely possessing the same gametophytic SI system that is widespread in the genus Linaria and its family, Plantaginaceae (Pandey, 1960; Franklin-Tong, 2008). We hypothesized that SC in L. cavanillesii represents a potentially recent loss of this SI mechanism in part of the species’ range.
Our main aim in this paper is to characterize variation in the mating system and floral biology of L. cavanillesii across its range in southeastern Spain, and to investigate the morphological and genetic consequences of a transition from SI to SC. We were particularly interested in understanding why an outcrossing syndrome appears to be maintained in populations that have lost SI. We used hand-pollination experiments in the glasshouse to ascertain the extent to which individuals of L. cavanillesii are capable of self-fertilization, and whether self-fertilization could occur autonomously in the absence of pollinators. We assessed the extent to which L. cavanillesii shows patterns of genetic diversity within and between populations that are consistent with expectations of a transition from outcrossing to self-fertilization, and whether they have begun to evolve any characteristics of a selfing syndrome. Finally, we asked whether reproductive assurance could have resulted in the transition to SC in this mostly outcrossing species. Comparison of our results with those obtained with other species reveals that L. cavanillesii occupies a relatively extreme combination of high genetic differentiation among populations despite high outcrossing rates.
MATERIALS AND METHODS
Site and study species
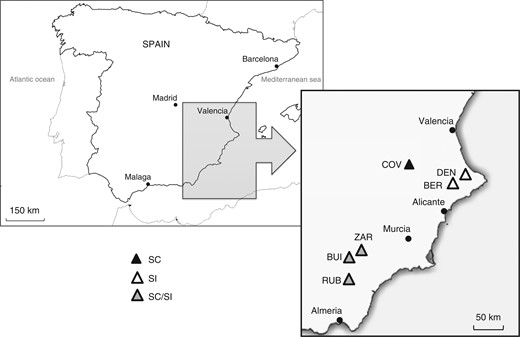
Geographical location of the six different populations of Linaria cavanillesii sampled in Spain, with their respective mating systems as revealed by controlled crosses (SC, self-compatible population; SI, self-incompatible population; SC/SI, mixed population containing SI plants and leaky SI plants).
Hand-pollination crosses
To characterize the distribution of SI and SC within and among populations, we carried out hand-pollination crosses on plants grown in glasshouses at the University of Lausanne, Switzerland, from seeds collected in the field. Experimental crosses were performed mostly between November 2012 and March 2013. Five treatments were applied to flowers: (1) autonomous pollination (inflorescences were bagged with small fine-meshed nylon bags at the bud stage a few days before flowers opened, and flowers and eventually fruits were allowed to develop on their own); (2) self-pollination (inflorescences were bagged at the bud stage, anthers were removed prior to anthesis with fine forceps, and self pollen was applied to stigmas); (3) outcrossing within populations (inflorescences were bagged at the bud stage, anthers were removed prior to anthesis, and outcross pollen from another plant in the same population was applied to stigmas); (4) outcrossing between populations (inflorescences were bagged at bud stage, anthers were removed prior to anthesis, and outcross pollen from a donor plant in another population was applied to stigmas); and (5) a control cross (inflorescences were bagged at the bud stage, anthers were removed prior to anthesis with fine forceps, and no further manipulation was performed). Two days after flowers opened, pollen was applied by gently brushing an anther against a stigma until it was covered by pollen. Additionally, we also tested delayed selfing by applying pollen to flowers 5 d after opening. We assessed fruit development after 14 d. We applied rubber glue to the tips of developing fruit capsules ∼18 d after pollination (when fruits were fully formed) to prevent the loss of seeds upon fruit dehiscence. We collected fruits ∼10 d after gluing, at which point they were fully mature, and we counted and weighed seeds. Each treatment was performed once on different flowers for each individual, with a total of 20 different plants from each population.
Fruit set and pollen limitation experiment
To assess whether individuals in the SC population enjoy the benefit of reproductive assurance, we estimated the proportion of flowers setting fruits (fruit set) in the field. In L. cavanillesii, fruits that contain viable seeds are much larger than those that have not been pollinated, and can thus be easily distinguished. Because capsules remain open on the plant after seed dispersal, without dropping off, fruit set can be estimated even after seed dispersal. We took measurements in each population on as many plants as were available in both 2013 and 2015 (sample sizes are given in the Results section).
We also performed a pollen limitation experiment in the field in May 2015. In each population, we randomly selected plants and applied the four following treatments on marked flowers: (1) control (inflorescences left unmanipulated with no bagging); (2) control bag (inflorescences bagged at bud stage, anthers removed prior to anthesis, and flowers left unmanipulated); (3) supplementary outcross pollen (no bagging, anthers removed prior to anthesis, and, when mature, outcross pollen applied to stigmas from at least two donor plants from the same population); and (4) emasculation (no bagging, anthers removed prior to anthesis, and the flowers left unmanipulated). Control and supplementary outcross treatments were used to compare pollination success under natural conditions or under conditions of pollen supplementation. Control bag and emasculation treatments were used to identify plants that had an ability to self-fertilize autonomously without the aid of pollinators. Finally, we assessed fruit development after 14 d.
Phenotypic comparison of plants among populations
To assess the degree to which the loss of SI might have precipitated the evolution of a selfing syndrome, we compared flowers from the single known fully SC population with those from three other populations comprising SI individuals. For each population, we conducted measurements on 30 individuals grown from seeds, each produced by a different individual sampled in the field. Seeds were first cleaned with bleach and germinated in Petri dishes placed in phytotron (conditions: 13 h light/11 h dark, 20:15 °C day:night, 80 % humidity), before transplantation into pots. Plants were grown in a randomized block design on glasshouse benches, with five plants from each population placed in each of six blocks. Plant positions were randomized once a week.
We measured the following traits for each individual: number of days to germination, total number of germinated seeds, plant size after 25 d and 16 weeks of growth, number of days to flowering, total number of flowers produced over three months of flowering, flower longevity, flower (length from the top of the corolla to the tip of the spur) and spur size (length from the beginning to the tip of the spur), nectar quality (measured with two refractometers to cover a range of nectar concentration of 0–50 and 45–80 Brix; Bellingham & Stanley Ltd, Tunbridge Wells, UK) and pollen/ovule ratio. In order to account for flower variability within plants, every phenotypic measure of flowers was taken and averaged using at least three flowers per plant, and flower longevity was estimated for at least two flowers per plant. Due to some plant mortality, every measure was taken on an average of 19 plants per population (minimum 16, maximum 23).
We estimated the number of pollen grains using a particle counter (Elzone II 5390 Micromeritics®). For each sample, one upper and one lower anther of a non-opened flower were fixed in formaldehyde–acetic acid alcohol solution (FAA; 5 parts glacial acetic acid, 5 parts 38 % formaldehyde and 90 parts 70 % ethanol). Before analysis, samples were sonicated (Branson 52, Emerson Industrial Automation) for 2 min to release pollen from anthers, and then transferred into the analysis beaker of the particle counter, which contained 100 mL of ddH2O with 2 % NaCl. Each sample was analysed four times for 30 s, and an average of the four replicates was used to estimate the number of pollen grains per anther. We estimated pollen production for each plant as an average of measures taken for three flowers per plant. For each population, the diameter range of pollen grains was estimated using photos taken with a microscope equipped with a digital camera (sample sizes: 67 to 93 pollen grains per population; Olympus DP-10 digital camera, Hamburg, Germany). We estimated the number of ovules for the same flowers used for pollen counting, based on floral dissections using a razor blade and counted under a binocular microscope (Leica MZ 125; Leica Microsystems®).
Genetic diversity and selfing rate estimation
To estimate genetic diversity and differentiation among populations, as well as their respective selfing rates, we assayed variation at 16 microsatellite loci (Supplementary Data Note S1). We collected leaf material from the six natural populations (sample size 29–41 per population), and extracted DNA with the DNeasy 96 Plant kit (Qiagen). Polymorphic primers (Note S1) were amplified by PCR (Biometra thermocycler; Göttingen, Germany) using the following reagents: 1× PCR mix: 2 ng μL-1 template DNA, 10× PCR buffer, 25 mm MgCl2, 5× Q-solution, 2·5 mm dNTP, 0·2 μm of each primer and 0·5 U/μL of Taq DNA polymerase (HotStarTaq®; Qiagen). The thermocycling conditions were 15 min at 95 °C followed by 32 cycles of 30 s at the annealing temperature (Supplementary Data Note S1, Table S2), 30 s at 72 °C and 30 s at 95 °C, followed by one cycle of 1 min at the annealing temperature (Note S1, Table S2) and a final extension of 30 min at 72 °C. The PCR products were then sequenced in an ABI3100 Genetic Analyzer (Applied Biosystems). We used the program Genemapper® to analyse microsatellite data, and the program Fstat (Goudet, 1995) to calculate and compare genetic diversity, allelic richness, population structure (FST) and inbreeding coefficient (FIS) among populations. We estimated selfing rates using the software RMES (David et al., 2007), which infers selfing rates from the distribution of multilocus heterozygosity taking into account the possible presence of null alleles.
Statistical analyses
We used linear mixed models to investigate phenotypic differences among populations, using the lme4 package in R (Bates et al., 2014), with population as a fixed effect and block as a random factor in order to account for spatial variation. For the assessment of phenotypic differences, we included the date of measurement as an additional random factor. Significance of variables was analysed using a stepwise deletion procedure, and multiple comparisons of means were performed using the lmerTest package in R (Kuznetsova et al., 2014). For binomial data (i.e. proportion of germination or fruit set), a generalized linear model (glm) was used with quasibinomial errors. If significant, differences within treatments were then tested by means of posthoc tests, using the glht function of the multcomp package in R. Measures of allelic richness and genetic diversity were compared among populations using analysis of variance (ANOVA), with differences among populations tested using posthoc Tukey HSD tests. All analyses reported were conducted in R (version 3.1.2 or later, R Core Team 2014).
RESULTS
Fruit set and seed production following hand-pollination in the greenhouse
Experimental hand-pollination revealed variation in selfing ability among the populations. In total, 466 crosses were performed. The outcross treatment (within and among populations) produced fruit consistently at 91 % (successful fruit formation/number of crosses: 205/226; Supplementary Data Fig. S1). Self-pollination resulted in total fruit production in one population (COV) and in moderate fruit production (<35 %) in three populations (BUI, RUB, ZAR; Fig. S1). Additionally, the rate of autonomous selfing was high in the COV population (>80 %) and moderate in the BUI, RUB and ZAR populations (<15 %; Fig. S1). No plants showed any evidence of delayed selfing.
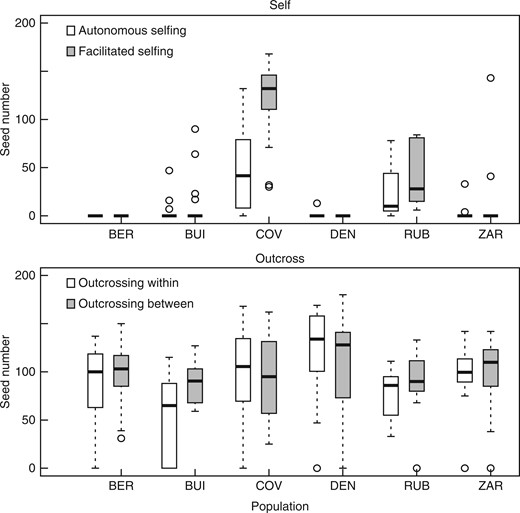
Comparison of mean seed number produced after controlled crosses of Linaria cavanillesii in the greenhouse. Treatments were performed on 20 plants in each population: autonomous selfing (flower not manipulated); facilitated selfing (pollen coming from the same plant); outcrossing within (pollen coming from another plant within population); and outcrossing between (pollen coming from plants in a different population). In the box plots, middle lines represent median, boxes represent the first and third quartiles, lower and upper bars represent the minimum and the maximum, and points represent outliers (i.e. points above 1·5 s.d.).
Variation in fruit set under open pollination in the field
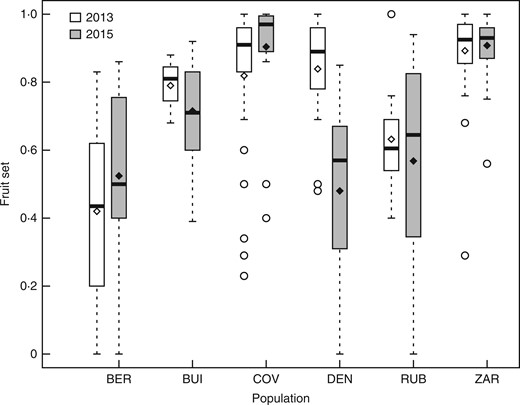
Estimation of fruit set in six populations of Linaria cavanillesii in 2013 and 2015. In the box plots, middle lines represent median, boxes represent the first and third quartiles, lower and upper bars represent the minimum and the maximum, and points represent outliers (i.e. points above 1·5 s.d.). Diamonds represent means for each population. COV, self-compatible population; DEN, BEN, self-incompatible populations; ZAR, RUB, BUI, mixed populations with self-incompatible and leaky self-incompatible individuals.
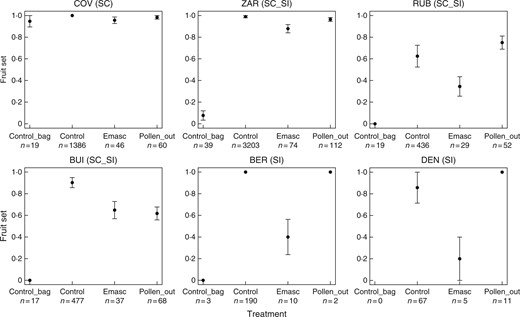
Comparison of fruit set in six populations of Linaria cavanillesii after different treatments: control bag (inflorescences were bagged at bud stage, anthers were removed prior to anthesis, and flowers were left unmanipulated); control (inflorescences were left unmanipulated); emasculation (emasc; anthers were removed prior to anthesis and the flowers were left unmanipulated) and supplementary outcross pollen (pollen_out; anthers were removed prior to anthesis, and, when mature, outcross pollen was applied to stigmas from at least two donor plants from the same population). SC, self-compatible population; SI, self-incompatible population; SC_SI, mixed population containing both types of plant. Bars represent mean ± s.e.m. and the number of plants used for each treatment is indicated.
Phenotypic variation among populations
Populations varied for a number of plant traits, with a significant effect in eight of the 11 traits measured (non-significant traits were flower longevity, pollen/ovule ratio and size after 25 d; Table 1, Supplementary Data Figs S2–S5). The mean number of days to germination as well as nectar quality were significantly different between one SI population (ZAR) and all the other populations (Table 1, Fig. S2). However, no significant differences were consistently found among the other populations (Figs S2–S5). We found little evidence of trait variation consistent with evolution towards a selfing syndrome in the SC population, except that plants in the SC population showed a somewhat shorter time to flowering (Table 1, Fig. S4).
Summary of statistical results for the different traits measured in four populations of Linaria cavanillesii. Degrees of freedom (d.f.), F ratios (F) and P values are reported for linear mixed models or glm models with binomial data (indicated with a *). Population was treated as a fixed effect. Significant results are shown in bold type
. | d.f. . | F . | P value . |
---|---|---|---|
Time to germination | 3,101·2 | 6·69 | <0·001 |
Proportion germination* | 3,111 | 9·77 | <0·001 |
Size after 25 d | 3,109·1 | 0·26 | 0·26 |
Size after 16 weeks | 3,114 | 5·91 | <0·001 |
Time to flowering | 3,115 | 8·4 | <0·001 |
Flower production | 3,110·1 | 2·82 | 0·04 |
Flower size | 3,50·6 | 7·81 | <0·001 |
Nectar quantity | 3,56·6 | 4·23 | 0·009 |
Nectar quality | 3,37·4 | 3·56 | 0·02 |
Flower longevity | 3,60·6 | 1·25 | 0·3 |
Pollen/ovule | 3,83 | 0·55 | 0·6 |
. | d.f. . | F . | P value . |
---|---|---|---|
Time to germination | 3,101·2 | 6·69 | <0·001 |
Proportion germination* | 3,111 | 9·77 | <0·001 |
Size after 25 d | 3,109·1 | 0·26 | 0·26 |
Size after 16 weeks | 3,114 | 5·91 | <0·001 |
Time to flowering | 3,115 | 8·4 | <0·001 |
Flower production | 3,110·1 | 2·82 | 0·04 |
Flower size | 3,50·6 | 7·81 | <0·001 |
Nectar quantity | 3,56·6 | 4·23 | 0·009 |
Nectar quality | 3,37·4 | 3·56 | 0·02 |
Flower longevity | 3,60·6 | 1·25 | 0·3 |
Pollen/ovule | 3,83 | 0·55 | 0·6 |
Summary of statistical results for the different traits measured in four populations of Linaria cavanillesii. Degrees of freedom (d.f.), F ratios (F) and P values are reported for linear mixed models or glm models with binomial data (indicated with a *). Population was treated as a fixed effect. Significant results are shown in bold type
. | d.f. . | F . | P value . |
---|---|---|---|
Time to germination | 3,101·2 | 6·69 | <0·001 |
Proportion germination* | 3,111 | 9·77 | <0·001 |
Size after 25 d | 3,109·1 | 0·26 | 0·26 |
Size after 16 weeks | 3,114 | 5·91 | <0·001 |
Time to flowering | 3,115 | 8·4 | <0·001 |
Flower production | 3,110·1 | 2·82 | 0·04 |
Flower size | 3,50·6 | 7·81 | <0·001 |
Nectar quantity | 3,56·6 | 4·23 | 0·009 |
Nectar quality | 3,37·4 | 3·56 | 0·02 |
Flower longevity | 3,60·6 | 1·25 | 0·3 |
Pollen/ovule | 3,83 | 0·55 | 0·6 |
. | d.f. . | F . | P value . |
---|---|---|---|
Time to germination | 3,101·2 | 6·69 | <0·001 |
Proportion germination* | 3,111 | 9·77 | <0·001 |
Size after 25 d | 3,109·1 | 0·26 | 0·26 |
Size after 16 weeks | 3,114 | 5·91 | <0·001 |
Time to flowering | 3,115 | 8·4 | <0·001 |
Flower production | 3,110·1 | 2·82 | 0·04 |
Flower size | 3,50·6 | 7·81 | <0·001 |
Nectar quantity | 3,56·6 | 4·23 | 0·009 |
Nectar quality | 3,37·4 | 3·56 | 0·02 |
Flower longevity | 3,60·6 | 1·25 | 0·3 |
Pollen/ovule | 3,83 | 0·55 | 0·6 |
Genetic diversity, levels of inbreeding and population genetic structure
We found significant differences in measures of genetic diversity between the SC and SI populations (Table 2). In particular, while one SI (ZAR) population showed a negative FIS (−0·047), all other populations showed positive FIS (Table 2). After correction for multiple testing (corrected P value = 0·0005), only one leaky SI (RUB) and the SC (COV) population showed a significantly positive FIS (Table 2).
Estimates (mean±s.d.) of selfing rates, allelic richness, genetic diversity and FIS for the six populations of Linaria cavanillesii studied. SC, SI and SC/SI denote self-compatible, self-incompatible and mixed populations (with both SI and leaky SI individuals), respectively. Selfing rate estimates are based on multilocus heterozygosity data using microsatellites and the software RMES (David et al., 2007). Allelic richness, genetic diversity and FIS were calculated using microsatellite data and the software FSTAT (Goudet, 1995)
Population . | Selfing rate . | Allelic richness . | Genetic diversity . | FIS . |
---|---|---|---|---|
COV (SC) | 0·59±0·2 | 1·49±0·5a | 0·09±0·13a | 0·36* |
RUB (SC/SI) | 0·14±0·16 | 2·12±1·3 | 0·28±0·25 | 0·23* |
ZAR (SC/SI) | 0·15±0·08 | 2·36±1·1 | 0·36±0·27b | −0·05 |
BUI (SC/SI) | 0±0 | 2·30±1·3 | 0·31±0·25 | 0·04 |
DEN (SI) | 0·05±0·12 | 2·65±0·7b | 0·27±0·21 | 0·05 |
BER (SI) | 0±0 | 2·19±0·9 | 0·34±0·26b | 0·12 |
Population . | Selfing rate . | Allelic richness . | Genetic diversity . | FIS . |
---|---|---|---|---|
COV (SC) | 0·59±0·2 | 1·49±0·5a | 0·09±0·13a | 0·36* |
RUB (SC/SI) | 0·14±0·16 | 2·12±1·3 | 0·28±0·25 | 0·23* |
ZAR (SC/SI) | 0·15±0·08 | 2·36±1·1 | 0·36±0·27b | −0·05 |
BUI (SC/SI) | 0±0 | 2·30±1·3 | 0·31±0·25 | 0·04 |
DEN (SI) | 0·05±0·12 | 2·65±0·7b | 0·27±0·21 | 0·05 |
BER (SI) | 0±0 | 2·19±0·9 | 0·34±0·26b | 0·12 |
Significant differences among populations for the allelic richness and genetic diversity are indicated where there is no letter in common.
Significant FIS values after Bonferroni correction.
Estimates (mean±s.d.) of selfing rates, allelic richness, genetic diversity and FIS for the six populations of Linaria cavanillesii studied. SC, SI and SC/SI denote self-compatible, self-incompatible and mixed populations (with both SI and leaky SI individuals), respectively. Selfing rate estimates are based on multilocus heterozygosity data using microsatellites and the software RMES (David et al., 2007). Allelic richness, genetic diversity and FIS were calculated using microsatellite data and the software FSTAT (Goudet, 1995)
Population . | Selfing rate . | Allelic richness . | Genetic diversity . | FIS . |
---|---|---|---|---|
COV (SC) | 0·59±0·2 | 1·49±0·5a | 0·09±0·13a | 0·36* |
RUB (SC/SI) | 0·14±0·16 | 2·12±1·3 | 0·28±0·25 | 0·23* |
ZAR (SC/SI) | 0·15±0·08 | 2·36±1·1 | 0·36±0·27b | −0·05 |
BUI (SC/SI) | 0±0 | 2·30±1·3 | 0·31±0·25 | 0·04 |
DEN (SI) | 0·05±0·12 | 2·65±0·7b | 0·27±0·21 | 0·05 |
BER (SI) | 0±0 | 2·19±0·9 | 0·34±0·26b | 0·12 |
Population . | Selfing rate . | Allelic richness . | Genetic diversity . | FIS . |
---|---|---|---|---|
COV (SC) | 0·59±0·2 | 1·49±0·5a | 0·09±0·13a | 0·36* |
RUB (SC/SI) | 0·14±0·16 | 2·12±1·3 | 0·28±0·25 | 0·23* |
ZAR (SC/SI) | 0·15±0·08 | 2·36±1·1 | 0·36±0·27b | −0·05 |
BUI (SC/SI) | 0±0 | 2·30±1·3 | 0·31±0·25 | 0·04 |
DEN (SI) | 0·05±0·12 | 2·65±0·7b | 0·27±0·21 | 0·05 |
BER (SI) | 0±0 | 2·19±0·9 | 0·34±0·26b | 0·12 |
Significant differences among populations for the allelic richness and genetic diversity are indicated where there is no letter in common.
Significant FIS values after Bonferroni correction.
Allelic richness was lower for the SC population compared with all the other populations, and this difference was significant for one SI population (DEN; ANOVA, F5,90 = 2·34, P = 0·05; Tukey HSD, P < 0·048; Table 2). Genetic diversity showed the same pattern, with a lower diversity for the SC population, which differed significantly from two other populations (BER and ZAR; ANOVA, F5,91 = 2·73, P = 0·02; Tukey HSD, P < 0·05; Table 2).
Population genetic differentiation was generally high, being strongest among the three northernmost and the three southernmost populations (FST > 0·70), and lowest among the three southernmost populations (FST < 0·35; Table 3). Differentiation was also strong between the SC population and the two SI populations in the north (FST > 0·56), and somewhat weaker between the two SI populations (FST = 0·44; Table 3).
Pairwise FST among six populations of Linaria cavanillesii. SC, SI and SC/SI denote self-compatible, self-incompatible, and mixed populations (with both SI and leaky SI individuals), respectively. See text for details
. | COV . | RUB . | ZAR . | BUI . | DEN . | BER . |
---|---|---|---|---|---|---|
. | (SC) . | (SC/SI) . | (SC/SI) . | (SC/SI) . | (SI) . | (SI) . |
COV | 0 | 0·75 | 0·73 | 0·75 | 0·63 | 0·56 |
RUB | – | 0 | 0·32 | 0·46 | 0·64 | 0·60 |
ZAR | – | – | 0 | 0·28 | 0·60 | 0·53 |
BUI | – | – | – | 0 | 0·63 | 0·55 |
DEN | – | – | – | – | 0 | 0·44 |
BER | – | – | – | – | – | 0 |
. | COV . | RUB . | ZAR . | BUI . | DEN . | BER . |
---|---|---|---|---|---|---|
. | (SC) . | (SC/SI) . | (SC/SI) . | (SC/SI) . | (SI) . | (SI) . |
COV | 0 | 0·75 | 0·73 | 0·75 | 0·63 | 0·56 |
RUB | – | 0 | 0·32 | 0·46 | 0·64 | 0·60 |
ZAR | – | – | 0 | 0·28 | 0·60 | 0·53 |
BUI | – | – | – | 0 | 0·63 | 0·55 |
DEN | – | – | – | – | 0 | 0·44 |
BER | – | – | – | – | – | 0 |
Pairwise FST among six populations of Linaria cavanillesii. SC, SI and SC/SI denote self-compatible, self-incompatible, and mixed populations (with both SI and leaky SI individuals), respectively. See text for details
. | COV . | RUB . | ZAR . | BUI . | DEN . | BER . |
---|---|---|---|---|---|---|
. | (SC) . | (SC/SI) . | (SC/SI) . | (SC/SI) . | (SI) . | (SI) . |
COV | 0 | 0·75 | 0·73 | 0·75 | 0·63 | 0·56 |
RUB | – | 0 | 0·32 | 0·46 | 0·64 | 0·60 |
ZAR | – | – | 0 | 0·28 | 0·60 | 0·53 |
BUI | – | – | – | 0 | 0·63 | 0·55 |
DEN | – | – | – | – | 0 | 0·44 |
BER | – | – | – | – | – | 0 |
. | COV . | RUB . | ZAR . | BUI . | DEN . | BER . |
---|---|---|---|---|---|---|
. | (SC) . | (SC/SI) . | (SC/SI) . | (SC/SI) . | (SI) . | (SI) . |
COV | 0 | 0·75 | 0·73 | 0·75 | 0·63 | 0·56 |
RUB | – | 0 | 0·32 | 0·46 | 0·64 | 0·60 |
ZAR | – | – | 0 | 0·28 | 0·60 | 0·53 |
BUI | – | – | – | 0 | 0·63 | 0·55 |
DEN | – | – | – | – | 0 | 0·44 |
BER | – | – | – | – | – | 0 |
Variation in selfing rates
The inferred selfing rate (s) varied among the six populations, with the five largely SI populations showing predominant outcrossing (s < 0·2) and the SC population showing mixed mating (s = 0·59, falling within the range between 0·2 and 0·8 commonly regarded as mixed mating; Table 2) (Schemske and Lande, 1985).
DISCUSSION
Our study documents variation in self-incompatibility and the mating system among populations of the Spanish long-lived perennial herb Linaria cavanillesii. This species has hitherto been described as self-compatible (SC) (Carrió et al., 2013), but our results indicate that it is in fact largely an SI outcrossing species that has likely undergone a shift to SC in the northern extreme of its range. Self-incompatibility in the SI populations was generally strong, but some populations showed evidence for leaky expression of SI. Although plants in the SC population could benefit from reproductive assurance in the absence of pollinators, we found that pollinator activity was generally sufficient to ensure reproduction. Moreover, although the fully SC population showed some of the population-genetic hallmarks of a history of selfing, it has strikingly retained floral traits characteristic of an outcrossing syndrome, and its outcrossing rate remains moderately high. Interestingly, both the SC and SI populations of L. cavanillesii represent outliers in the plane relating the realized mating system to patterns of population genetic differentiation, with particularly high FST values found among populations. Below we discuss the significance of these findings, emphasizing what they contribute to an understanding of the drivers and consequences of the loss of SI in flowering plants. In particular, we consider what uncommon aspects of the biology of L. cavanillesii might be responsible for the unusual patterns it shows in terms of its floral biology and genetic variation.
Variation in self-compatibility among populations
Our study documents a new example of within-species variation in SI in a flowering plant. Crosses in the greenhouse revealed that, of the six isolated populations sampled, two are strongly SI (with all individuals tested being incapable of selfing), one is fully SC (with all individuals capable of autonomous selfing) and three are variable in their self-compatibility, with SI and a few SC plants occurring. Several cases of such within-species variation have been investigated in some detail, most of which are annuals or short-lived perennial species (e.g. Lloyd, 1965; Raven, 1969; Busch, 2005; Mable et al., 2005; Dart et al., 2012). Linaria cavanillesii is a long-lived perennial plant in which an ability to self-fertilize is likely to be less important for reproductive assurance (Baker, 1955; Pannell and Barrett, 1998; Aarssen, 2000; Larson and Barrett, 2000), and in which selection to avoid inbreeding depression caused by selfing should be particularly high (Duminil et al., 2009; and see below).
Not only does L. cavanillesii vary among populations in the strength of SI, but individuals also vary within some populations. In these populations, a few plants that we isolated from pollinators in a glasshouse occasionally produced fruits by autonomous selfing, albeit with fewer seeds than those that had been outcrossed. The sporadic production of a low proportion of seeds upon self-pollination has been shown in many SI species and has been referred to as ‘leaky SI’ or ‘pseudo-self-incompatibility’ (Levin, 1996) (e.g. Brennan et al., 2005; Busch, 2005; Crawford et al., 2008, 2010; Dart et al., 2012; Zhang et al., 2014). As a transitional state, leaky SI may have played a role in the transition from SI to full SC in some species (Good-Avila and Stephenson, 2002), as it may have done in the loss of SI in at least one population of L. cavanillesii. It is noteworthy that the transition to full SC has occurred in only a small part of the species range, despite the occurrence of leaky SI in other populations. In these populations, the fact that inbreeding depression is high (M. Voillemot and J. R. Pannell, unpubl. res.) may have selected against further independent transitions to SC. Strong inbreeding depression is likely to have been a key factor maintaining the SI system in the colonizing species Senecio squalidus, where ∼3 % of wild plants investigated show leaky SI (Brennan et al., 2005).
Variation in the mating system among populations
Despite the possibility of occasional selfing in populations of L. cavanillesii with leaky SI, our estimates of the inbreeding coefficient of their adult plants point either to very limited selfing in the wild, or to strong viability selection against selfed progeny. Either way, it is clear that the occasional ability to self-fertilize in some populations of L. cavanillesii has not affected the realized mating system very much. In contrast, high inbreeding coefficients estimated for the fully SC population indicate that it has intermediate realized selfing rates (an inferred selfing rate of s=0·59), i.e. this population appears to have a mixed mating system. The finding of substantial numbers of inbred adults in the SC population suggests that inbreeding depression must be sufficiently low to allow at least some selfed individuals to reach reproductive maturity. Direct estimates of inbreeding depression for this population (M. Voillemot and J. R. Pannell, unpubl. res.) are consistent with this inference.
Although mixed mating (commonly defined as 0·2<s<0·8; Schemske and Lande, 1985) is quite common in flowering plants (Vogler and Kalisz, 2001; 42 % out of 345 plant species tested: Goodwillie et al., 2005), our finding of substantial outcrossing in the SC population of L. cavanillesii is somewhat surprising. While outcrossing may be maintained in SC species through other outcrossing mechanisms, such as the temporal (Bertin and Newman, 1993, e.g. Kalisz et al., 2012) or spatial separation of the sexes within flowers (Webb and Lloyd, 1986; e.g. Karron et al., 1997; Takebayashi et al., 2006), the stigmas of L. cavanillesii are fully surrounded by, and in contact with, the anthers, and they appear to be receptive as soon as the anthers dehisce. We might thus have expected SC individuals to self-fertilize all their ovules autonomously, precluding opportunities for outcrossing. Even if plants are indeed able to self-fertilize autonomously, we found that the flowers of SC individuals of L. cavanillesii are as large and rewarding as those of SI individuals, and they continue to be visited by pollinators. The maintenance of substantial levels of outcrossing despite the loss of SI thus points either to a ‘competing’ or a ‘delayed’ mode of selfing in L. cavanillesii (Lloyd and Schoen, 1992). In discussing their survey of the literature, Fenster and Martén-Rodríguez (2007) suggested that species in which SC individuals continue to attract and reward pollinators seemed mainly to be capable of delayed selfing, a device that promotes outcrossing while nevertheless assuring reproductive assurance via selfing when pollinators are absent. Specifically, they found that 84 % of 38 SC species investigated displayed delayed selfing, with only 16 % showing prior or competing selfing. Of these, half showed competing selfing with protandrous flowers and half showed no temporal separation between male and female functions within flowers. A particularly interesting example is provided by the species Camissoniopsis cheiranthifolia, a species with mixed mating in which the selfing rate is associated with differences in floral morphology among populations (Dart et al., 2012). In C. cheiranthifolia, as in L. cavanillesii, the fact that flowers remain attractive and are visited by pollinators accounts for the maintenance of substantial outcrossing, despite an ability of individuals to self. Similarly, in populations of the annual plant Collinsia verna, high pollinator visitation ensures high levels of outcrossing despite the ability to self autonomously (Kalisz and Vogler, 2003, 2004).
Pollen limitation and reproductive assurance
Results of our pollen supplementation experiment, as well as global fruit set observed at the end of each of two reproductive seasons in the field, revealed variation among populations in the extent to which their seed set was pollinator-limited. Whereas plants in the fully SC population were able to set fruit autonomously in the absence of pollinators, pollinator visitation levels were such that this ability was not necessary for high seed set, as indicated by substantial seed production by emasculated flowers. The same was found in one of the SI populations of L. cavanillesii, in which pollinator visitation ensured seed set equivalent to that produced by hand-pollinated flowers. Our results thus provide little support for the importance of selection for reproductive assurance in driving the loss of SI in the northern extreme of the range of L. cavanillesii, where the SC population is found. This conclusion contrasts with that reached for other species, in which pollen limitation appears to be reduced in geographically marginal populations that have evolved selfing (Busch, 2005; Michalski and Durka, 2007; Mimura and Aitken, 2007; but see Herlihy and Eckert, 2005; Moeller et al., 2012).
Although pollen limitation and selection for reproductive assurance may be of limited importance in the northern range of L. cavanillesii, pollinator visitation was insufficient to ensure full seed set in four of the SI populations investigated, where pollen supplementation substantially increased seed set. Although results of pollen supplementation tests must be interpreted with caution in absolute terms, and perhaps conservatively (Ashman et al., 2004; Knight et al., 2005), it nevertheless seems clear that these SI populations of L. cavanillesii were at least more pollen-limited than the SC and one other SI population that showed an absence of pollen limitation. Only in these four SI populations might one thus expect selection potentially to favour mutations conferring SC and the evolution of selfing as reproductive assurance (Lloyd and Schoen, 1992). As noted above, however, inbreeding depression might be sufficient to maintain outcrossing despite this potential advantage, as argued for the mixed mating species Aquilegia canadensis (Herlihy and Eckert, 2002).
It is widely thought that selection for reproductive assurance in the face of pollen limitation is likely to be strongest in short-lived or annual species, in which reproductive failure is particularly consequential (Larson and Barrett, 2000; Duminil et al., 2009). Given that L. cavanillesii is a long-lived perennial, we should therefore not expect selection for reproductive assurance to be particularly important, even in those populations that were somewhat pollen-limited. Of course, our field observations were carried out over only two reproductive seasons, and pollinator activity might have been higher in other years in the pollen-limited SI populations. Nevertheless, it does seem unlikely that transition to SC was driven by selection for reproductive assurance under the conditions we observed in the SC population. Rather, reproductive assurance may have been an important selective factor when that population was first colonized, e.g. through mate limitation (see below).
Variation in genetic diversity and differentiation among populations
We found that the SC population was genetically less diverse than the SI populations. This pattern is a typical finding in comparisons between selfing and outcrossing species or populations (Hamrick and Godt, 1996; Mable et al., 2005; Glémin et al., 2006), and is consistent with theoretical expectations (Charlesworth, 2003). We also found strong differentiation between northern and southern populations, and particularly between the SC population and all the other populations. Again, this is consistent with the observation that selfing populations tend to be more strongly differentiated from one another than outcrossing populations (Hamrick and Godt, 1996; Duminil et al., 2009). Low genetic diversity in selfing populations has been generally attributed to their lower inbreeding effective sizes (which, for fully selfing populations, should be 50 % of the effective size of a fully outcrossing population; Pollak, 1987; Nordborg and Donnelly, 1997), and thus increased effects of drift locally. It is difficult to estimate the effective size of the populations we sampled, but the current census size of the selfing population is probably substantially greater than the size of most of the outcrossing populations we sampled (M. Voillemot and J. R. Pannell, pers. observ.). Given that the selfing rate in the SC population is intermediate, we doubt that the short-term effects of increased inbreeding are sufficiently severe to account for the lower diversity found.
The higher levels of genetic differentiation generally observed among selfing populations (Hamrick and Godt, 1996; Duminil et al., 2009) are likely to be a result of both the reduced local inbreeding effective population sizes and, particularly, the tendency of selfing species to disperse less pollen (Harder and Barrett, 1996; Charlesworth and Pannell, 2001), not least because pollen dispersal is usually the dominant mode of gene flow among outcrossing populations (Ennos, 1994). However, individuals in the SC population of L. cavanillesii do not produce substantially less pollen than those in SI populations (see below) and, as discussed above, are at least as frequently visited by pollinators. It is thus difficult to account for the high level of differentiation between the SC population and the other populations in terms of reduced pollen dispersal. It is more likely that the population lost its diversity as a result of a bottleneck at the point of colonization, perhaps by an SC individual, as has been hypothesized for other SC species or populations (e.g. Foxe et al., 2010; Busch et al., 2011).
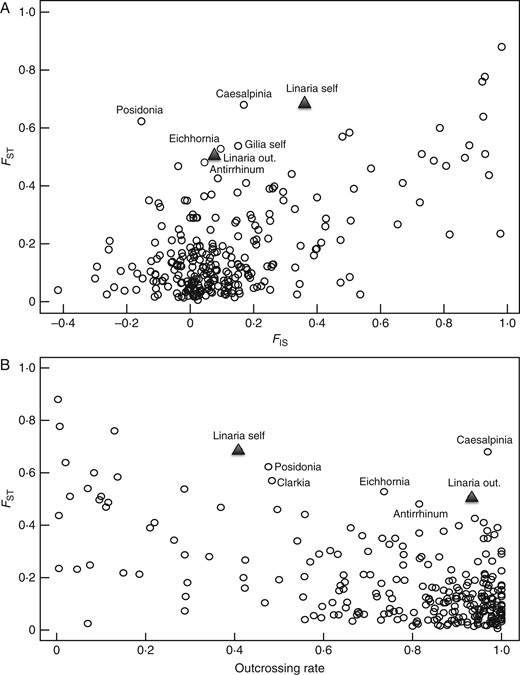
Correlation between FST and (A) FIS and (B) estimated outcrossing rates for species reviewed in Duminil et al. (2009). Estimates for Linaria cavanillesii, calculated on the basis of microsatellite data, are shown with closed triangles for the selfing and outcrossing population (labelled “Linaria self”, and “Linaria out”, respectively; black triangles). The other species are Antirrhinum valentinum (Antirrhinum), Caesalpinia echinata (Caesalpinia), Clarkia tembloriensis (Clarkia), Eichhornia paniculata (Eichhornia), selfing Gilia achilleifolia (Gilia self) and Posidonia australis (Posidonia). See text for details.
Absence of a selfing syndrome
The loss of SC in angiosperms is often followed by an increase in selfing rates and the evolution of a so-called selfing syndrome, with smaller flowers and reduced nectar and pollen production (Ornduff, 1969; Cruden, 1977; Goodwillie et al., 2010; Sicard and Lenhard, 2011). In contrast, we find no evidence for the evolution of such traits in L. cavanillesii. Instead, individuals in the SC population have large flowers and produce copious amounts of nectar, both at levels that were in the upper range for the populations we studied. Similarly, the pollen:ovule ratio found in both SC and SI populations of L. cavanillesii (around 600) falls in the range commonly observed for facultative SI species (244–2599; Fig. S5) (Cruden, 1977).
We can think of at least three possible reasons for the lack of any shift in the SC population of L. cavanillesii towards a floral syndrome typical of selfing species. First, it is possible that the loss of SC simply did not lead to predominant self-fertilization because outcrossing has been maintained by selection to avoid inbreeding depression. As we have seen, this does indeed seem to be the case to some extent, as individuals continue to be visited by pollinators and outcross substantial numbers of their offspring. If self-fertilized offspring were doomed to failure as a result of inbreeding depression, the loss of SI would not be expected to prompt a shift to selfing, but rather to the evolution of other mechanisms that ensure outcrossing (Baker, 1967). For example, the loss of SI in species of Lycium evidently led to the evolution of separate sexes as an outcrossing device (Miller and Venable, 2002), as apparently has occurred in many species that lost SI during colonization of oceanic islands following long-distance dispersal (Sakai et al., 1995; Barrett et al., 1996; Pannell et al., 2015). Although it would appear that traits presumably selected for outcrossing in SI L. cavanillesii are being maintained in the SC population (large, zygomorphic flowers with nectar spurs containing much nectar), we do not believe this results from selection to avoid inbreeding depression. Our finding of a strong heterozygote deficiency among adults in the SC population (FIS = 0·36) suggests that inbreeding depression is low (Ritland, 1990), a hypothesis that is also consistent with direct measures of inbreeding depression (M. Voillemot and J. R. Pannell, unpubl. res.).
Second, it is possible that outcrossing is being maintained in the SC population of L. cavanillesii to avoid other costs of selfing, such as pollen discounting, i.e. the loss of pollen through selfing that would otherwise have been destined for outcrossing. Empirical work has shown that pollen discounting can constitute a substantial cost of selfing, particularly in species with large floral displays (Chang and Rausher, 1998; Fishman, 2000), and theory indicates that it can contribute to the maintenance of outcrossing or mixed mating (Holsinvger, 1988; Harder and Wilson, 1998; Goodwillie et al., 2005; Porcher and Lande, 2005). However, again we do not believe that this hypothesis helps to explain the maintenance of an outcrossing syndrome in L. cavanillesii. Importantly, the loss of SI will have led to no immediate change in the floral biology of the individuals concerned; they presumably will have continued to produce similar numbers of flowers, with similar amounts of pollen dispersed from anthers placed in the same position beside the stigma. Thus, a recently evolved SC individual should experience no more pollen discounting than an SI individual (unless there were pleiotropic effects of the mutation conferring SC, which also seems unlikely). It is of course possible that SC individuals could have evolved novel floral traits subsequent to the loss of SI, such as subtle changes in phenology, but if pollen discounting constituted an important cost, we should then expect traits to evolve to reduce rather than increase it.
Finally, it is possible that an outcrossing syndrome is observed in SC individuals of L. cavanillesii simply because there has been insufficient time for a selfing syndrome to evolve (e.g. Hoebe, 2009; Busch et al., 2011). We do not know how long ago SI broke down in L. cavanillesii, but it is plausible that the transition to SC was very recent, perhaps too recent for substantial morphological change to have occurred. Immediately following the loss of SI, particularly if it coincided with the colonization of a new population, there would likely have been little genetic variation on which selection could act. Because the population may have remained relatively small throughout its (possible young) history, new mutations that might have been beneficial (e.g. by reducing investment in outcrossing) could have been lost easily by drift before being fixed by selection. Ultimately, the extent to which adaptive evolution towards a selfing syndrome occurs (assuming that it is indeed adaptive) must depend not only on how recent the transition to SC was, but also on the size of the bottleneck through which the new population was established and on the effective size of the population during its history since the transition. The fact that many species have evolved a selfing syndrome after a transition to self-fertilization involving a genetic bottleneck suggests either that the bottleneck was not sufficiently severe to deplete variation upon which selection could act, or that sufficient time has elapsed for the accumulation of new adaptive mutations.
Although there is good evidence for the evolution of a selfing syndrome in many species that have lost SI (Lloyd, 1965; Ritland, 1989; Foxe et al., 2009; Goodwillie et al., 2010), L. cavanillesii is by no means the first example of one that has failed to do so. For instance, SI has broken down independently in two different populations of the mustard relative Leavenworthia alabamica, in one of which there is little evidence for evolution towards a selfing syndrome, perhaps because the loss of SI was so recent (Busch, 2005; Busch et al., 2011). Similarly, SI has broken down in North American populations of Arabidopsis lyrata, but this has not been accompanied by important changes in floral morphology, despite increased selfing rates (Mable et al., 2005; Hoebe, 2009; Foxe et al., 2010). In Camissoniopsis cheiranthifolia, different mating systems can be found in different populations, with some containing large SC flowers associated with mixed mating (Dart et al., 2012), but in contrast to L. cavanillesii, inbreeding depression appears quite strong in these populations (Dart and Eckert, 2013). It therefore seems that several factors can explain the absence of a selfing syndrome after the loss of SI, with perhaps the most satisfactory for L. cavanillesii being a recent transition to SC.
Concluding remarks
We were originally prompted to carry out a detailed study of the mating system of L. cavanillesii because its large floral displays and apparently massive investment in pollinator reward pointed towards an outcrossing rather than a selfing system, in contrast to what had been claimed in the literature (Carrió et al., 2013). The fact that most of the populations we have now studied do indeed comprise obligate outcrossers provides an illuminating example of how observations of the floral biology and allocation strategies of a plant can inform hypotheses about its mating system before genetic assays or experiments are conducted: we were ultimately not surprised that L. cavanillesii is largely an SI outcrosser, after all. Nor is it surprising that in some of these SI populations a low frequency of individuals exist that show leaky incompatibility and produce some selfing, leaky SI being a common phenomenon in SI species (Levin, 1996; e.g. Brennan et al., 2005; Busch, 2005; Crawford et al., 2008, 2010; Dart et al., 2012; Zhang et al., 2014). The fact that the inbreeding coefficient for adults in these populations of L. cavanillesii is low suggests that inbreeding depression kills selfed offspring before they reach reproductive maturity. This observation, together with the fact that pollinator activity in the populations can be quite high, suggests that selection in these SI populations most likely acts to maintain outcrossing. What does remain a puzzle, however, is that one population has not only lost SI, but now has a mixed mating system with probably low inbreeding depression – it thus joins rank with a great many species with mixed mating systems that have proved enigmatic to explain (Goodwillie et al., 2005). It also remains puzzling that a species that has taken steps on a path towards self-fertilization shows no signs of the selfing syndrome. The hypothesis that the transition to SC was recent is plausible, but it remains somewhat unsatisfactory as an explanation for all the patterns we observe.
SUPPLEMENTARY DATA
Supplementary data are available online at www.aob.oxfordjournals.org and consist of the following. Note S1: details of the development and characterization of 16 new polymorphic microsatellites markers. Table S1: names and locations of the different populations sampled in Spain. Table S2: summary of characteristics for the 16 polymorphic markers. Figure S1: proportion of fruit formation resulting from controlled crosses. Figure S2: comparison of germination time and germination rate. Figure S3: comparison of flower longevity, flower size, nectar quantity and nectar quality. Figure S4: mean number of days from seed germination to flowering, and total number of flowers produced over three months. Figure S5: pollen/ovule ratio.
ACKNOWLEDGEMENTS
We thank D. Savova Bianchi and J. El-Assad for their help with microsatellite development, L. Liberati and M. Staiff for their help with phenotypic measurements, and N. Ruch for his technical help in the greenhouse as well as with the particle counter. We are grateful to the University of Lausanne for funding the studentship awarded to M.V. This work was supported by a studentship from the University of Lausanne.