-
PDF
- Split View
-
Views
-
Cite
Cite
Cornelius L Nel, Julius H J van der Werf, Wendy M Rauw, Schalk W P Cloete, Challenges and strategies for genetic selection of sheep better adapted to harsh environments, Animal Frontiers, Volume 13, Issue 5, October 2023, Pages 43–52, https://doi.org/10.1093/af/vfad055
- Share Icon Share
Sheep production enterprises are a common choice for farming in extensive conditions, leaving sheep exposed to environmental elements such as harsh climates or low-quality forage.
It is important for animal breeding to consider adaptability to challenging environments in the genetic selection of sheep, especially given the expected effects anticipated from climate change.
Breeding animals for unpredictable environments requires attention to the issue of genotype by environment interaction. Research into these issues for sheep has not matched that seen for intensive species such as pigs or dairy, but opportunities exist both within existing breeding structures as well as from the development of new, novel phenotypes.
Health and fitness traits are likely to remain difficult to measure, but current methods and new protocols should be combined with other developments in animal breeding such as genomic selection for worth-while genetic gain.
Sheep breeding structures and economics are very different from intensive species such as dairy and thus require particular approaches to promote cooperation and investment from individual breeders and industry.
Introduction
Extensive sheep farming is often associated with small flocks grazing large areas, delivering limited economic returns compared with the revenue of examples such as dairy or intensive beef cattle farming. However, sheep are a popular choice of livestock in challenging environments where few other production systems are viable (Rust and Rust, 2013). Low input, extensive systems make use of the ability of ruminants to metabolize low-quality, high fiber materials that characterize pastoral grazing. By producing wool, meat, and sometimes dairy, extensive sheep production can deliver high-quality output products from a low-quality input that would otherwise have had little value for sustaining mankind. Furthermore, extensive pastoral systems can arguably be excused from some of the criticisms aimed at factory farming (Frank, 1979).
Assuming sustainable stocking rates, extensive sheep farming may be favored in terms of the social and environmental concerns around animal production. However, low input systems are also associated with specific challenges. Outside the support of intensive systems, livestock are expected to survive, produce, and reproduce with little assistance from human intervention or shelter. In addition to requiring a high level of independence, they are also more exposed to the environmental elements such as harsh climates, disease, and parasite challenge. These environmental stressors directly affect production, but the implications for health and welfare should also be considered (Dwyer, 2009).
Environmental intervention can be effective (Masters et al., 2023) and should aim to ensure the best outcome for animals wherever possible. In the majority of extensive systems, however, opportunities are limited for being either impractical or too expensive. Animals kept in extensive systems should thus possess an ability to adapt and cope with environmental challenges—a concern of animal breeding and genetics. The issue of adaptability has been previously reviewed according to different definitions such as the genetic selection for animal “robustness” (Rauw and Gomez-Raya, 2015) or “resilience” (Colditz and Hine, 2016; Berghof et al., 2019). The underlying concept is to achieve genetic selection of candidates most capable of withstanding or coping with environmental changes or challenges, with a desirable outcome for both performance and welfare (Rauw et al., 2021). A conceptual framework for genetic robustness was outlined by Knap (2005), and the reader interested in a wider treatment of “adaption and fitness” applied to both animal and plant species should also see the topics compiled by van der Werf et al. (2009).
In extensive sheep flocks, climatic stress is a key focus of environmental adaption, and will likely become a greater concern with the effects expected from climate change (Rust and Rust, 2013). Heat stress, for example, has important implications for the well-being of ruminants (Silanikove, 2000). Accordingly, opportunities for genetic improvement of heat stress resistance has received considerable attention in the last two decades, but with the majority of studies aimed at intensive species such as dairy cattle (Pryce et al., 2022). Despite the very high level of exposure to heat stress for sheep, information on the genetic components of heat stress resistance is scarce . In turn, certain stages of the sheep reproduction cycle can also be particularly susceptible to cold stress, which has severe implications for mortality rate when lambs are born into cold, wet, and windy conditions (Donnelly, 1984). Premature death is not only a major contributor to overall reproductive wastage but also an important social concern (Dwyer, 2008).
In addition to other stressors such as parasite and disease challenge, this review will address the challenges and opportunities in considering important environmental factors in genetic selection of sheep. This paper aims to (1) provide a short overview of the issues for genetic selection across variable environments; (2) consider the challenges in adapting these concepts to sheep breeding; and (3) suggest strategies promoting research and implementation, both through opportunities within existing datasets as well as in development of novel breeding objectives.
Genotype by environment interaction
Genetic gain is achieved through selection of breeding candidates according to estimated breeding value (EBV) derived from the well-known method of best linear unbiased prediction (BLUP) analysis of performance records. An important characteristic of EBVs is that they present the expected performance of the animal or genotype in the “average” environment (i.e., genetic merit is determined after environmental influences have been accounted for). In the two-tier system common in sheep breeding; however, there may be problems with this assumption. Stud breeders form the top-tier, where performance and pedigree recording is used to identify and market superior males as breeding stock. Producers, who form the lower (commercial) tier, purchase the sires for improving the genetics of their flocks. Producers often make use of the low input, extensive systems described as the target environment of this review, but here performance is not usually recorded on an individual level. In turn, stud animals in the top tier are more likely to be maintained under more intensive management with access to supplementary feeding, shelter, and supervision. Evidence of special treatment of stud animals is not formally recorded, but a well-known occurrence in commercial sheep breeding.
The issue is important, because the more challenging circumstances of the progeny born in the lower tier are not accounted for in the “average” environment according to BLUP analysis. This leads not only to a biased EBV but also brings the issue of genotype by environment interaction (G×E), the contention that the genetic merit of an animal is dependent on the environment in which it is recorded. In a G×E scenario, the highest-ranking genotype (e.g., sire) in a given environment (e.g., intensive) is not necessarily the preferred candidate in a different environment. This issue of re-ranking is outlined in Figure 1, an exaggerated example where performance is directly linked to a continuous gradient between either warm or very cold climates.
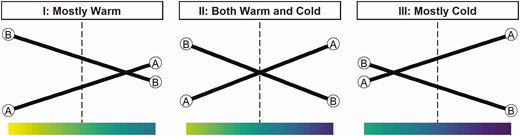
The effects of genotype by environment interaction on performance (y-axis) according to different windows of environmental change (x-axis). Roughly adapted from Fig(s) 3 and 4 of Falconer (1990).
If genotype B is well adapted to cope with heat stress, and genotype A with the cold, selecting the best genotype depends on the “environmental window” within which they are measured (e.g., I or III). The extent of re-ranking between A and B will depend on 1) how sensitive genetic performance is to environmental change and 2) the extent of environmental variation, or “width of the window", within which they were recorded. Genetic selection for genotype B in environment I (or vice versa for A in III) should result in genetic progress because in this environment it is likely the preferred candidate more often than not (see Falconer, 1990 for discussion). However, if both heat and cold stress are present, such as in environment II (Figure 1), genetic selection could be ineffective since both A and B could be the preferred choice depending on the climate at a particular time. This describes the general issue of G×E, which can slow genetic progress if the environment is not considered (Mulder and Bijma, 2005). The effect of temperature is an important example, but the environmental stressor could also be the plane of nutrition, disease, or parasite challenge. These are common stressors in extensive systems, and considering adaptability to these factors should be a priority in sheep breeding (Figures 2–4).
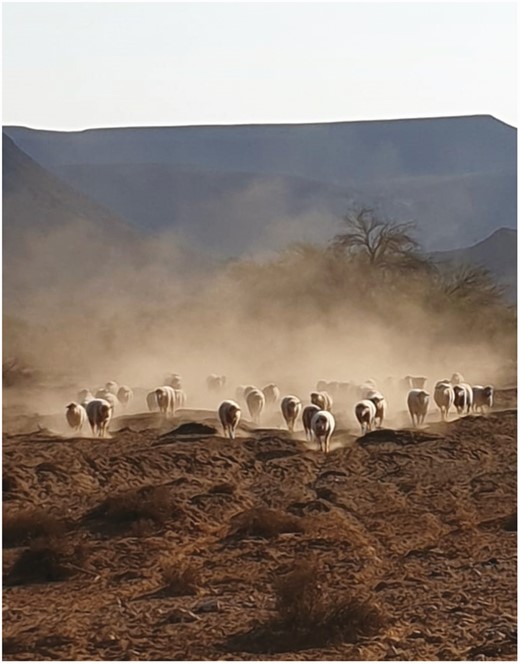
The dual purpose Dohne Merino sheep breed navigating the hot and arid landscape of the Karoo region, South Africa (Source: Koenas van der Westhuizen).
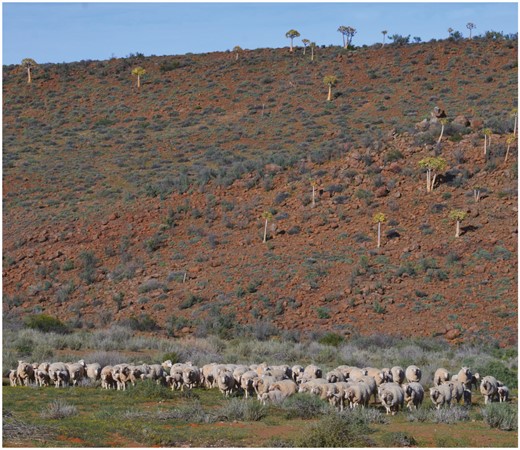
In addition to hot and dry climates, extensive landscapes can also be mountainous, requiring healthy, capable sheep to best make use of the available grazing (Source: Kobus Delport).
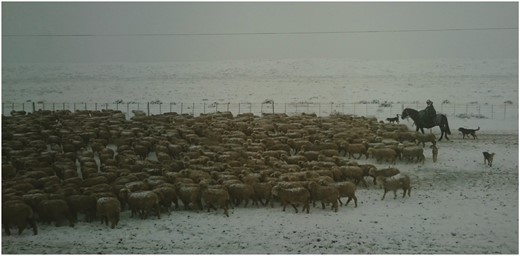
Here, the same Dohne Merino sheep breed is found in the cold and snowy regions of Patagonia, Chile (Source: Kobus Delport).
The most desirable characteristic of production animals is the ability to express good performance or fitness independent of the environment, i.e., a genotype “robust” to external stressors (Knap, 2005). A robust genotype is not necessarily the best performing in either environment I or III, for example, but rather the animal with the best average performance across all environments. The review by Knap (2008) discusses two approaches to testing genetic robustness and resilience. The first involves “reaction norm” analysis (see De Jong and Bijma, 2002), where genetic performance of production or “output” traits are tested for sensitivity to environmental change. The second is to phenotypethe animal’s response to a particular stressor explicitly, for example, the ability to cope with a harsh climate or resistance to a parasitic challenge. The reaction norm approach could be possible with existing datasets recorded to measure production, while the method of directly measuring health or fitness will most likely require the development of new, novel phenotypes to be recorded additionally.
Reaction norm analysis: opportunities in sheep
In reaction norm analysis, robustness of a genotype can be tested by deriving the random regression of genetic performance on an environmental covariate (e.g., heat or cold stress in Figure 1). In the simplest case of a linear reaction norm, the EBV consists of two components: the intercept or the predicted merit for the average environment and the slope or the predicted sensitivity to environmental change.
A necessary structure is repeated measures along different levels of the environmental variable that can be linked to the genotype. This can be in the form of multiple records on the individual itself, or links to different records through the pedigree, in which case the structure of a sire model is commonly used. The analysis also requires an ability to quantify a variable that can suffice as a description of the environment at recording. The influence of heat stress, for example, can be represented by the temperature humidity index (THI; Ravagnolo et al., 2000). The THI can be easily derived from commonly available weather station data, assuming that the source provides a good description of on-farm conditions. This approach has been widely applied to test the genetic components of heat stress resistance in dairy cattle, as reported by Ravagnolo and Misztal (2000). In sheep, the pronounced physiological effects of heat stress (Marai et al., 2007) will affect the capacity for production and reproduction in sensitive individuals. With the exception of repeated measures in dairy sheep (Finocchiaro et al., 2005), the data structure for most sheep production traits (see review by Safari et al., 2005) differs from that used to estimate reaction norms in dairy. Production traits tend to be recorded once and at the same time point (and thus THI level) for all animals in a herd-year contemporary group (CGroup). A single animal is recorded only once in a CGroup and linked to only a single environmental level, but the pedigree can be used to link a genotype to performance at different environmental levels.
Besides climate data, another environmental measure could be using on farm data to grade low to high input systems into a continuous scale, or “farm environment” (McLaren et al., 2015). The most common approach to date has been to use the phenotypic CGroup mean itself as the environmental descriptor. In this way, robustness can be tested by fitting reaction norms across low- to high-quality environments, but the particulars of the environmental differences are not known. This approach relies on numerous assumptions (Strandberg, 2006), but is useful for evaluating the presence of G×E in production traits, such as growth rate (Hollema et al., 2018).
In reproduction and survival traits, the stability of EBVs are particularly important, since a low level of robustness in these trait groups has direct and major implications for animal fitness and welfare. For these traits, time of recording varies slightly between animals, since ewes in the same management group are not mated or give birth in the same narrow timeframe as for the recording of production traits. Since climatic variables such as THI can also vary considerably within the period of a lambing or mating season, individual records will be linked to varying levels of the environmental covariate.
Ideally, robust ewes should reproduce regardless of environmental or seasonal effects, including conceiving, giving birth, and successfully rearing their lambs. In dairy cattle, studies have evaluated genetic components of conception rate in the presence of high heat stress (Haile-Mariam et al., 2008). It would be of great interest to repeat these studies in sheep, especially in regions where the mating season commences during the high heat of summer. In commercial sheep production, ewes within a CGroup are mated across a period of approximately 42 days, and theoretically, records could be associated with varying levels of THI. Unfortunately, it would be difficult to link conception rate records to the corresponding climate data because individual mating dates are not known in natural mating systems, but the novel phenotypes section below discussed new opportunities in this regard. With artificial insemination, mating dates are known but ewes are commonly synchronized by progestogen sponges and inseminated within a very short space of time, resulting in a structure more similar to that seen for production traits with little to no variation in THI within the CGroup. Another opportunity remains in the form of hand mating systems, where ewes are mated as they naturally come into heat over the 21- to 36-day cycle (i.e., high chance of variation in THI) and mating dates are also known. Hand mating systems are not common, but with climate data easily available it should be possible to apply this study retrospectively for any case where such datasets exist.
The case of lamb survival
As an alternative to ewe rearing ability, survival at various intervals from birth to weaning can also be defined as a genetic trait of the lamb (Brien et al., 2014). Survival outcome is determined by a complicated interaction of both the ewe and her lamb (Hinch and Brien, 2014), but survival phenotypes are easy to measure for any breeding flock that undergoes daily lambing rounds. Despite a low heritability, worthwhile genetic gain in lamb survival has been reported (Nel et al., 2021b) with desirable economic and welfare outcomes. Unfortunately, examples of recording of lamb survival traits outside of the research sector are very scarce, with the important exception of the protocol of Sheep Improvement Limited (SIL; http://www.sil.co.nz) in New Zealand, which has been providing breeding values for lamb survival since 1999. A problem with survival phenotypes is that the binary (yes/no) records are not always a reflection of the fitness of the lamb or the ewe’s ability to rear her young. To counter this issue, a recommended phenotyping protocol has been proposed by Vanderick et al. (2015), who proposed a system that adjusts phenotypes if cases could be identified as unrelated to animal fitness. A lamb identified as succumbing to misadventure or predation, for example, will maintain the record of having survived. In turn, a lamb identified as having a high likelihood of mortality (or a ewe having difficulty lambing) can receive assistance, but the records should be adjusted to an unfavourable phenotype—achieving both the goals for genetic selection as well as maintaining animal welfare by human intervention.
Furthermore, if the environment at recording could be better described, the survival phenotype can be a better indicator of lamb fitness. Records of lambs surviving cold, wet, and windy conditions (i.e., high cold stress) should be a much better indicator of fitness than lambs surviving when stress levels were low. Similar to THI, the level of cold stress can be defined from climate data (Donnelly, 1984). Using the sire model, preliminary findings have shown a higher heritability for neonatal mortality at increasing levels of cold stress (Nel et al., 2023). Heat stress could also have direct or indirect effects on new-born lambs (van Wettere et al., 2021), but analysis on a genetic level is still needed. A key question is whether adaptation to cold stress and adaptation to heat stress are negatively genetically correlated. Climate data can be linked to any existing datasets where age of death was recorded, but investigating the relationship between cold and heat stress is not straightforward using currently available measures. The chill index used to measure cold stress does not consider the effect of relative humidity, while THI does not account for the cooling effect of wind speed or rainfall. A bilateral stress index is therefore needed to allow for G×E studies over wide climatic extremes of both heat and cold stress.
Direct measures of response: difficult to measure traits and novel phenotypes
If only production traits are recorded, there is no opportunity to balance selection for production and fitness traits, which are not necessarily favorably correlated. Genetic selection based only on production output can be detrimental for animal well-being (Rauw et al., 1998), and there is thus a need to explicitly measure traits that indicate animal fitness and health. There is no strict definition for this trait group, but the terms “fitness”, “health” and “resilience” are often used, and are also arguably interchangeable with “robustness”, but for the purposes of this review this latter term is reserved to describe the slope of reaction norm analysis.
Many traits recorded as an indication of fitness or resilience are considered “difficult to measure” due to recording being both expensive and/or time consuming. In sheep, examples of fitness or health traits have predominantly focused on resistance to mastitis, fly strike, internal parasites, and footrot. In a recent meta-analysis by Mucha et al. (2022), resilience traits were reported with a low-to-moderate h2 suggesting useful genetic components for selection. However, estimates of genetic parameters tended to be highly variable across different studies (Mucha et al., 2022), and far fewer studies were available when compared to a similar meta-analysis for production traits reported much earlier (Safari et al., 2005). An additional complication in fitness traits is that the ability to evaluate an animal is dependent on the stress challenge being present at recording, which can vary markedly across environments. Despite this, worthwhile genetic gains have been reported for examples such as selection for reduced fecal worm egg count (Morris et al., 1997) or the incidence of breech fly strike (Brien et al., 2021). Genetic selection targeting disease incidence has also been gaining increasing attention in recent years (Knap and Doeschl-Wilson, 2020), but requires particular procedures dealing with disease incidence data (see Walkom et al., 2022 for development on EBVs for footrot). In addition to these traits, a direct measure of the response to harsh climates would be an important target for extensive small stock. Development of novel phenotypes to capture these parameters are required, but faces the formidable challenge of being both accurate and sufficiently practical to be measured on the scale needed for genetic analysis.
For heat stress, the animal’s thermoregulatory response is often measured according to rectal temperature, respiration rate, or blood chemistry (Srikandakumar et al., 2003). These methods are reliable, but difficult to implement on a large scale and in extensive production systems. Rectal temperature requires the handling of large sheep, which is not practical for field conditions and handling in itself could affect rectal temperature. The use of infrared thermograph measurements could be a viable proxy for core temperature (George et al., 2014) but also requires some level of restraint. Manually counting respiration rate (Cloete et al., 2021) or panting score (Marcone et al., 2021) has been applied in field paddocks, but is time consuming and would become impractical in the case of large paddocks.
In the case of cold stress, the greatest concern is the response in neonatal lambs (Plush et al., 2016). Newborn lambs are handled routinely in any flock that maintains a pedigree, making additional measurements easier to implement. Rectal temperature of day-old lambs has been measured under field conditions, and linked to using weather-station-derived chill index (Nel et al., 2021a). Rectal temperature phenotypes have been subject to genetic analysis by Brien et al. (2010), who reported a low heritability, but phenotypes were not linked to the cold stress gradient at recording. In turn, Slee et al. (1991) reported a moderate-to-high heritability for “cold stress resistance” specifically, but not in field conditions and based on a limited number of records. A study combining these elements is needed: a reaction norm analysis of rectal temperature across a cold stress gradient, measured in sufficient numbers to allow for a genetic analysis of lambs recorded in field conditions. Rectal temperature could be a promising target as an indicator trait for lamb survival (Brien at al., 2010; Nel et al., 2021a), and the continuous distribution is less problematic to analyze than survival or mortality phenotypes (Nel et al., 2023).
In most cases, capturing highly specific animal responses to fitness challenge is difficult. However, new opportunities are likely to be presented by the recent developments of “digital phenotyping”, with various applications for resilience traits already outlined by Brito et al. (2020). Proximity sensors have been used for predicting lambing dates (Paganoni et al., 2020), but could also be used to address the problem of obtaining mating dates for ewes in natural mating systems. In monitoring animal stress response, digital phenotypes has the potential to deliver two key benefits by (1) allowing animals to continue unhindered by restraint or human presence (their “natural” state) and (2) providing time-continuous, high-frequency measurements. In sheep, an interesting example is the continuous measurement of rumen temperature as a proxy for core temperature, where sheep requires no further handling after initial adminstration (Vesterdorf et al., 2022). Tri-axial accelerometers have also been applied to capture various behavioral issues associated with health and welfare for multiple livestock species (Chapa et al., 2020). This could be very useful for genetic selection targeting better welfare, since examples include the use of digital monitoring to detect lameness (Barwick et al., 2018), signs of internal parasite infestation (Fogarty et al., 2023), and events relating to parturition (Fogarty et al., 2020).
New phenotypes offer promising oppertunities to measure reslience, but are, however, likely to remain difficult to measure, requiring particular infrastructure and investment. For these traits, worth-while genetic gain will likely depend on further developments in quantitative genetics, such as the use of genome-wide marker data.
The role of molecular information
The ability to consider molecular information brought about new approaches for the genetic selection of traits with a low heritability that are sex limited, or difficult to measure. There are, however, various approaches apply molecular information for the genetic improvement of resilience or welfare traits (Rauw et al. (2021)). There is interest in identifying the underlying genes or quantitative trait loci (QTL) for traits related to fitness and resilience. For example, candidate “genes for reliance to heat stress” have been discussed in detail for small ruminants specifically (Sejian et al., 2019). The ability to identify well-adapted candidates on the information from a few important QTL would be a highly desirable strategy, but the pitfalls of “marker assisted selection” have already been outlined early in the 21st century (Dekkers, 2004). Despite a considerable risk of false positives, assuming a small amount of very important QTL does not deliver reliable prediction of quantitative genetic variance.
Traits related to fitness or resilience are often expressed on a binary scale, but the underlying genetic components are polygenic (i.e., trait outcome is determined by many genes each of small to moderate effect). Accordingly, the best approach for genetic gain for these traits is most likely by genomic selection, the method of considering all the information made available by dense, genome-wide single-nucleotide polymorphism markers as an estimate of “total genetic merit” (see Meuwissen et al., 2016 for an overview). Studies have applied genomic selection in sheep to target difficult to measure or fitness traits specifically, such as incidence of facial eczema (Phua et al., 2014), internal parasite resistance (Pickering et al., 2015), and lambing ease (Li et al., 2021). Marker data can also be incorporated into G×E studies to derive so-called genomic reaction norm models, better defining genetic linkage between different levels of the environmental trajectory (see Shi et al., (2021) for an example applied to heifer fertility during heat stress). For investigating variable genome-wide effects related to robustness, it is also possible to back-solve SNP-marker effects according to the estimated slope of reaction norm analyses (Waters et al., 2022).
The modern method of genomic selection, termed single-step genomic BLUP, (ssGBLUP) is still in continuous development (Misztal et al., 2021) but has become commonplace in breeding programs including large-scale implementation for sheep (Dodds et al., 2014; Brown et al., 2018). The use of genomic selection methods is likely to play a key role in the adoption of resilience traits into sheep breeding programs (van der Werf et al., 2014). By setting up “reference populations” that are dedicated to recording difficult to measure traits, genotyping could facilitate prediction of these traits in flocks where the traits are not routinely recorded. However, despite the advantages, a universal truth has been established in the “genomics revolution” in that a minimum level of continuous and accurate phenotyping cannot be circumvented. In other words, difficult to measure traits still have to be measured, which could be challenging within certain sectors of commercial sheep breeding.
Challenges in expanding commercial breeding schemes: the South African example
A key challenge in breeding sheep for better resilience or fitness will be the adoption of additional recording and genotyping needed to deliver breeding values to commercial breeders. One way to approach this problem is to an establish the reference population as a centralized breeding flock, similar to the Meat and Livestock Australia Resource Flock (Alexandri et al., 2022). In this case, the high cost of genotyping and difficult to measure traits is subsidized through national levy funds, and the information generated within the resource flock contributes to the national evaluation of breeding values. A resource population acting as a nucleus of information for commercial flocks needs to be strategically designed and managed for this purpose (van der Werf et al., 2014) as well as being sufficiently large to deliver reliable prediction. Coordinating a centralized infrastructure is difficult in sheep breeding where the industry structure is vastly different to the business models of the intensive livestock industries. Furthermore, it is likely that at least a proportion of breeders will need to participate in the recording of difficult to measure traits. More widespread recording will assist in increasing the size of the reference population, but also ensure that traits are measured across a variety of environments and that any possible genetic groups are represented.
In South Africa, breeding flocks are small (~400 to 600 breeding ewes), privately owned enterprises. In the absence of subsidies, any additional recording and/or genotyping is at the expense of the breeder. The genetic selection of health and welfare traits can deliver indirect economic benefits by reducing input costs and reproductive wastage (Rauw, 2016), but indirect benefits can be more difficult to quantify compared to the perceived monetary value of production traits. Naturally, breeders could be hesitant to agree to the either the time or investment needed to expand recording schemes to include health and fitness traits.
The most simple strategy would be to make recording compulsory for all stud flocks registered to the relevant breeding society, as is already the case for selected production traits in certain breeds. However, involuntary participation runs a risk of compromised data quality, and it is arguable that poorly recorded information could be worse than no records at all. A particular concern is that quality issues will be more pronounced in “difficult to measure” traits. Recently, Brown et al. (2022) presented a system aimed at addressing data quality problems, rightfully highlighting accurate phenotype data as the “cornerstone of genetic evaluation systems”. A quality control system would be very valuable in sheep breeding, but its effectiveness is not yet known.
Another approach could be incentivized participation, such as certification that delivers access to more attractive markets. An example is the “Responsible Wool Standard”, a voluntary global standard recently introduced to South Africa (Vlok, 2018). This certification offers financial benefits to participating wool producers by an increase in wool price per unit at marketing but demands the practice of production principles aligning with its sustainability goals, including “Protecting animal welfare” and “Preserving land health”. Certification initiatives are often implemented according to consumer preferences surrounding emotional and social issues of animal production. These issues fit well with the goals of improving animal fitness, since the genetic selection of better welfare (Rauw, 2016; Rauw et al., 2021) strongly align with the parallel objective of animal robustness or resilience (Rauw and Gomez-Raya, 2015; Colditz and Hine, 2016).
Conclusions
This paper briefly reviewed the challenges and opportunities for animal breeding to respond to the high exposure of sheep to harsh conditions, a problem that cannot be ignored given the expected effects associated with climate change. Regardless of the specific objective, being increased adaption, fitness, robustness, resilience or welfare, it is clear that animal breeding’s traditional approach of simply selecting animals with the highest production output is not the appropriate strategy for achieving these goals. Deliberate research into these issues should continue, especially for sheep, which have not been subject to the same level of investigation and development as the more intensively farmed species. There are opportunities within existing structures and datasets, but the new developments in novel phenotyping are the most promising platform for achieving progress towards these objectives in future. The majority of these new phenotypes are very likely to remain “difficult to measure”, however, and worthwhile genetic gain will depend on being combined with other technologies and analytical methods such as genomic selection. This will require widespread participation and investment, but routine genotyping has already been initiated in many breeding programs. It is also likely that no single strategy will be fully effective in aligning sheep production with the demands of the modern market. This review has not included the objectives of lessening the contribution of small stock to green-house gas emissions, an equally important but also challenging objective for sheep breeding and genetics (Hegarty et al., 2022). It also did not detail developments that could deliver more cost-effective environmental intervention, such as “photovoltaic farms”, where a dual use of land utilizes solar panels as shade or shelter for sheep as well as production of green energy (Handler and Pearce, 2022). Genetic selection for more robust or resilient sheep should proceed jointly with these climate mitigation and intervention strategies. However, genetic gain takes time, and it can thus be recommended that both the research and commercial sectors should be proactive in exploiting opportunities and overcoming the challenges such as those outlined in this review.
About the Authors
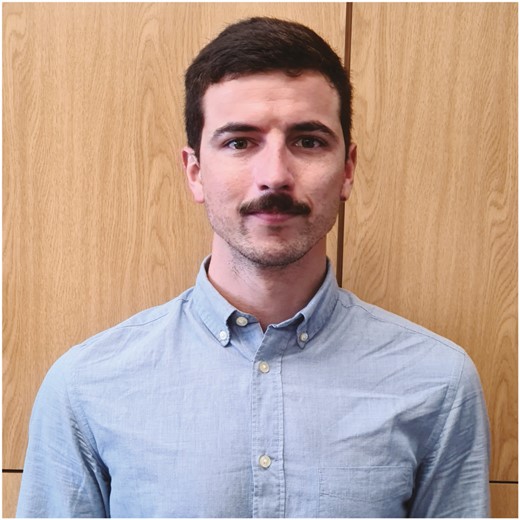
Dr Cornelius Nel is a scientist within the Department of Animal Science of the Western Cape Department of Agriculture, South Africa. He obtained his BSc, MSc, and PhD in Animal Science at the University of Stellenbosch, and currently participates in research in quantitative genetics of farmed animals, mostly sheep. He has been the principal investigator of the first studies incorporating marker data into genetic prediction of South African Merino sheep but has also worked on lamb survival and cold stress resilience. His interests are in the genetic prediction of health and welfare traits, and the use of genomic information to enhance such breeding objectives.
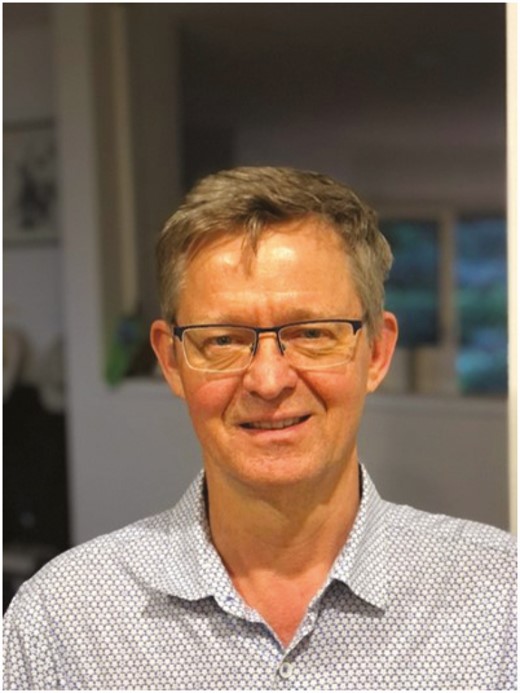
Julius van der Werf holds a PhD from Wageningen University and also studied at Cornell University, was an assistant professor in Wageningen from 1985 to 1993 and a senior researcher at the National Institute for Animal Science and Health between 1993 and 1997. In 1997, he moved to Armidale, where he is now professor in Animal Breeding and Genetics at the University of New England. He specializes in research into genetic evaluation, breeding program design, breeding objectives, and genomic selection. He supervised more than 60 PhD students and he managed the Sheep CRC genetics program from 2003 to 2019 during which genomic selection was introduced to the Australian sheep industry. He has been a member of several industry advisor committees and was in the panel of experts of the Australian Research Council. He was editor of leading journals in animal breeding such as GSE and JABG. He is now Head of School of Environmental and Rural Science at UNE.
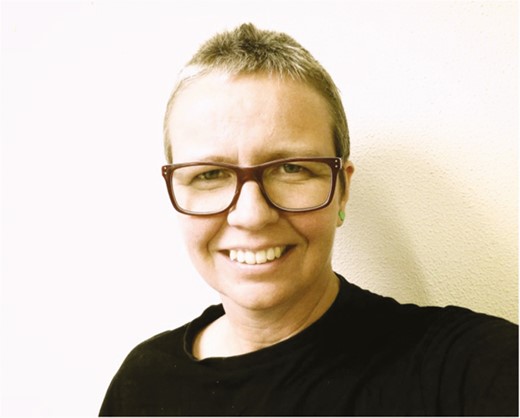
Dr. Wendy Mercedes Rauw has an MSc from Wageningen University and Research in The Netherlands and a PhD from the Norwegian University of Life Sciences in Ås. She has been a research scientist at the IRTA, Department of Animal Production in Lleida, Spain between 2000 and 2004, and an assistant professor at the University of Reno, Department of Animal Biotechnology in the United States between 2004 and 2010. Since 2010, she is a research scientist at INIA-CSIC, Department of Animal Breeding and Genetics in Madrid and at the INIA-CSIC Genetic Resources Conservation and Investigation Program of Spanish Breeds El Encín in Alcala de Henares. Her research encompasses animal resilience in a climate change context, feed intake and efficiency, animal genetics, and animal behavior and welfare.

Schalk Cloete: After retiring from a specialist scientist position at the Western Cape Department of Agriculture in March 2019, professor Schalk Cloete continued his career as an extraordinary professor at Stellenbosch University. He obtained his BSc (Animal Science and Animal Physiology), BSc Hons (Sheep and Wool Science), and MSc (Sheep and Wool Science) all from Stellenbosch University before obtaining a PhD in Animal Breeding and Genetics from the University of the Free State. He was employed by the Department of Cooperation and Development from February 1978 to February 1979, before joining the Winter Rainfall Region in January 1980. He was transferred to the Western Cape Department of Agriculture in April 1996, where he served until his retirement in March 2019. He specializes in Animal Breeding and Genetics with specific interests in Reproduction, Animal Behaviour, and Welfare. Most of his work is on sheep and ostriches.