-
PDF
- Split View
-
Views
-
Cite
Cite
Daniel C Huston, Scott C Cutmore, Terrence L Miller, Pierre Sasal, Nico J Smit, Thomas H Cribb, Gorgocephalidae (Digenea: Lepocreadioidea) in the Indo-West Pacific: new species, life-cycle data and perspectives on species delineation over geographic range, Zoological Journal of the Linnean Society, Volume 193, Issue 4, December 2021, Pages 1416–1455, https://doi.org/10.1093/zoolinnean/zlab002
- Share Icon Share
Abstract
The digenetic trematode family Gorgocephalidae comprises just a few species, and the literature devoted to the lineage consists of only a handful of reports. With one exception, all reports have been based on material collected in the Indo-West Pacific, an expansive marine ecoregion stretching from the east coast of Africa to Easter Island, Hawaii and French Polynesia. We collected adult and intramolluscan gorgocephalids from kyphosid fishes and littorinid gastropods from several Australian localities, and from South Africa and French Polynesia. Specimens of Gorgocephalus kyphosi and G. yaaji were collected from, or near, their type-localities, providing new morphological and molecular (COI, ITS2 and 28S) data needed for a revised understanding of species boundaries in the family. Two new species are recognized: Gorgocephalus euryaleae sp. nov. and Gorgocephalus graboides sp. nov. New definitive host records are provided for described species and three new intermediate hosts are identified. These new records are all associated with Kyphosus fishes and littorinid gastropods, reaffirming the restriction of gorgocephalids to these hosts. Most significantly, we provide evidence that G. yaaji is distributed from South Africa to French Polynesia, spanning the breadth of the Indo-West Pacific. Our findings have significant relevance regarding digenean species delineation over geographic range.
INTRODUCTION
The Gorgocephalidae Manter, 1966 is one of the smaller families of the Lepocreadioidea Odhner, 1905, presently comprising just a single genus and three species (Bray, 2005b; Bray & Cribb, 2005). Gorgocephalids exhibit a number of peculiar morphological features that readily distinguish them from other lepocreadioids, including an oral sucker bearing a crown of bifid tentacles, a single caecum and a duct arising from the oesophagus that passes to a pore on the ventral surface, the so-called ‘ventral anus’ (Manter, 1966; Zhukov, 1983; Bray, 2005b; Bray & Cribb, 2005). Adult gorgocephalids have been reported only from herbivorous marine fishes of the genus Kyphosus Lacepède, 1801 (Perciformes: Kyphosidae), the drummers or sea-chubs (Bray & Cribb, 2005). Although another lepocreadioid lineage, the Enenteridae Yamaguti, 1958, also exhibits a concentration of species in kyphosids (Bray & Cribb, 2001, 2002; Bray, 2005a; Huston et al., 2019a), the Gorgocephalidae has been demonstrated as phylogenetically distinct and has been convincingly resolved as sister to the lepocreadioid families Aephnidiogenidae Yamaguti, 1934 + Lepocreadiidae Odhner, 1905 (Olson et al., 2003; Bray & Cribb, 2012; Bray et al., 2018a; Pérez-Ponce de León & Hernández-Mena, 2019).
The type species of the Gorgocephalidae, Gorgocephalus kyphosiManter, 1966, was described from the silver drummer Kyphosus sydneyanus (Günther, 1886) collected from off South Australia (Manter, 1966), and has subsequently been reported from Kyphosus vaigiensis (Quoy & Gaimard, 1825) collected off Lizard Island, on the Great Barrier Reef (GBR), Australia, and from off Moorea, French Polynesia (Olson et al., 2003; Bray, 2005b; Bray & Cribb, 2005). The second species to be recognized, Gorgocephalus manteriZhukov, 1983, was described from Kyphosus sectatrix (Linnaeus, 1758) collected from the Gulf of Mexico, off Havana, Cuba (Zhukov, 1983) and, to date, has not been reported again. The most recently recognized species, Gorgocephalus yaajiBray & Cribb, 2005, was described from K. vaigiensis off Lizard Island, GBR (Bray & Cribb, 2005). This species has been reported on only one additional occasion (Huston et al., 2016), from the same locality, but from a different host, Kyphosus cinerascens (Forsskål, 1775).
In a survey of the digenean community parasitizing the littorinid gastropod Austrolittorina unifasciata (Gray, 1826) from along the coasts of south and south-eastern Australia, O’Dwyer et al. (2015) detected the first-known intramolluscan gorgocephalid infections. At the time of this discovery, the only molecular sequence data available for the Gorgocephalidae were for specimens identified as Gorgocephalus kyphosi, collected from off Lizard Island, GBR (Olson et al., 2003). O’Dwyer et al. (2015) characterized their intramolluscan gorgocephalids with molecular data and, because their sequences did not match those from the study of Olson et al. (2003), concluded that their infections represented a species other than G. kyphosi. The following year, Huston et al. (2016) reported intramolluscan gorgocephalid infections in a second littorinid species, Echinolittorina austrotrochoidesReid, 2007, from Lizard Island, GBR. Huston et al. (2016) matched these infections to adult G. yaaji collected from Kyphosus cinerascens from the same locality, and demonstrated that cercariae encyst as metacercariae on algae, elucidating the life cycle. Based on morphology and molecular sequence data, Huston et al. (2016) surmised that the cercariae of O’Dwyer et al. (2015) did not represent G. yaaji either, suggesting that additional gorgocephalid diversity awaited discovery.
Here, we evaluate adult and intramolluscan specimens of Gorgocephalus from kyphosids and littorinid gastropods collected from Australian, French Polynesian and South African marine ecosystems using morphological and molecular data. We propose two new species and provide new host records demonstrating broad host-specificity at both the definitive and intermediate host levels, but at the same time affirming the restriction of gorgocephalids to kyphosid fishes and littorinid gastropods, respectively. We show that this broad host-specificity allows multiple gorgocephalid species to occupy a wide geographic range and we provide the first evidence, supported by molecular sequence-data, of naturally occurring populations of a single digenean species ranging across the entire Indo-West Pacific (IWP) marine region. Our findings have significant relevance for our ability to delineate species of marine digeneans across a broad geographic range.
MATERIAL AND METHODS
Specimen collection and host identification
This is the fourth in a series of reports on the trematode fauna of kyphosid fishes from the IWP (see: Huston et al., 2019a, b; Martin et al., 2019). The present study focuses on digenean material recovered from 77 kyphosid fishes collected between 2015 and 2018. Most were caught in Australian waters, but kyphosids were also collected from French Polynesia, Japan and South Africa. Kyphosids were identified following Knudsen & Clements (2013a, b, 2016) and, where necessary, identities were confirmed using molecular data (see: Martin et al., 2019). Fish were collected mainly by spear, but some specimens were captured on line, and others were purchased from local fishers or fish markets. The gut of each fish was excised and examined for trematodes following Cribb & Bray (2010). Adult trematodes were collected alive, fixed without pressure in near-boiling saline and preserved in 70–80% ethanol for parallel morphological and molecular analyses.
Following previous findings of gorgocephalid intramolluscan stages in littorinid gastropods (Littorinimorpha: Littorinidae) by O’Dwyer et al. (2015) and Huston et al. (2016), between 2016 and 2018 further collections of littorinids were made from several IWP localities (Supporting Information, Table S1). Littorinids were collected by hand, identified following Reid & Williams (2004) and Reid (2007), and screened for digenean infection following Huston et al. (2016). In the few cases where there was any doubt regarding the identification of littorinids, a tissue sample was taken and the mitochondrial cytochrome c oxidase subunit I gene (COI) was amplified with the primers and cycling conditions of Folmer et al. (1994). Amplicons were purified with a Bioline ISOLATE II PCR and Gel Kit, following the manufacturer’s instructions. Sequence data were generated using the amplification primers via cycle sequencing with an AB3730xl capillary sequencer and ABI Big Dye v.3.1 chemistry, performed by the Australian Genome Research Facility (AGRF), Brisbane. Gastropod identities were confirmed through Basic Local Alignment Search Tool (BLAST) analyses (Altschul et al., 1990) against the National Center for Biotechnology Information (NCBI) database. Sequences generated from littorinid gastropods were uploaded to GenBank under the accession numbers MW346677–MW346678.
Morphological study
Trematode specimens used for morphological study were removed from their preservative, rinsed in fresh water, overstained in Mayer’s haematoxylin, de-stained in a solution of 1.0% hydrochloric acid and neutralized in a 0.5% ammonium hydroxide solution. Specimens were then dehydrated in a graded ethanol series. Some dehydrated specimens were selected for scanning electron microscopy (SEM); these specimens were transferred to hexamethyldisilazane, air-dried overnight and mounted on 12.5 mm pin-stubs using an adhesive carbon tab. Before SEM, specimens were coated with 15 nm of iridium with a Quorumtech Q150TS sputter coater. Scanning electron microscopy images were obtained on a Hitachi SU3500 scanning electron microscope in secondary electron mode. Specimens used for light-microscopy were cleared in methyl salicylate and mounted on slides in Canada balsam. Measurements for slide-mounted specimens were made with cellSens standard imaging software paired with an Olympus SC50 digital camera. Measurements are reported in micrometres (µm). Morphometric data were log-transformed and explored with principal component analyses (PCAs) of the covariance matrix in R (https://www.R-project.org) and visualized with the package ggfortify (Tang et al., 2016). Drawings were made using a camera lucida, mounted on an Olympus BX-53 compound microscope. Drawings were digitized, and PCA plots were annotated, in Adobe Illustrator CS6. Specimen vouchers are lodged in the South Australian Museum (SAM), Western Australian Museum (WAM), Queensland Museum (QM), Museum National d’Histoires Naturelles, Paris, France (MNHN) and National Museum Parasite Collection, South Africa (NMB); accession numbers are presented in the taxonomic section of this manuscript.
Molecular and phylogenetic study
Three molecular markers were targeted for the study of gorgocephalid trematodes: the second internal transcribed spacer region (ITS2), the large subunit ribosomal RNA (28S rRNA) and the mitochondrial cytochrome c oxidase I (COI) gene region. Genomic DNA was extracted from 44 adult gorgocephalid trematodes using phenol/chloroform extraction techniques (Sambrook & Russell, 2001). For 32 of these specimens, only part of the worm was consumed in molecular analyses; the remainder was processed for morphological study, as above, and retained as a morphological and molecular voucher [hologenophore sensuPleijel et al. (2008)]. Sequences for gorgocephalid intramolluscan stages from eight infected littorinid gastropods were obtained through direct PCR of individual cercariae or rediae (see: Huston et al., 2018b). In addition, previously extracted genomic DNA from the study of Huston et al. (2016), representing one adult gorgocephalid and six intramolluscan infections, was reused to generate new COI sequences.
Primers, amplification and sequencing protocols for the ITS2 and 28S regions are detailed in Huston et al. (2016). For the COI region, two primer pairs were used in amplification: the forward JB3 (5’-TTT TTT GGG CAT CCT GAG GTT TAT-3′) of Bowles et al. (1993) and the reverse Plag16S-COIdR (5’-TCG GGG TCT TTC CGT CT-3′) of Blasco-Costa et al. (2012), and the forward Dig_cox1Fa (5’-ATG ATW TTY TTY TTY YTD ATG CC-3′) and reverse Dig_cox1R (5’-TCN GGR TGH CCR AAR AAY CAA AA-3′) of Wee et al. (2017). These two primer sets do not generate overlapping COI fragments. In their characterization of the first-known gorgocephalid intramolluscan infections (‘Gorgocephalus sp. Aus’) from Austrolittorina unifasciata from New South Wales, Australia, O’Dwyer et al. (2015) amplified and sequenced the COI gene using the first above-mentioned primer pair. We amplified and generated sequences using this primer pair to confirm the conspecificity of the intramolluscan material collected by Huston et al. (2016) from the same host and a nearby locality in New South Wales, Australia, with ‘Gorgocephalus sp. Aus’ (deposited to Genbank under the accession numbers MW350141–MW350143; see Supporting Information, Table S2). These newly generated sequences were compared with those of O’Dwyer et al. (2015) using BLAST analyses (Altschul et al., 1990) against the NCBI database. However, we obtained relatively limited amplification success using these primers for other specimens in the present collection. Therefore, we used the primers of Wee et al. (2017) to obtain COI sequences for the whole collection. Amplification for the COI regions followed the protocols of O’Dwyer et al. (2015) and Wee et al. (2017), respectively. Sequencing was performed by the AGRF, as described above.
Sequences of the ITS2 and 28S rDNA gene regions for gorgocephalid and selected outgroup taxa were obtained from GenBank (Table 1). Alignments for each gene region were constructed separately using MUSCLE (Edgar, 2004), as implemented in MEGA7 (Kumar et al., 2016). The ITS2 and 28S rDNA alignments were trimmed to the median sequence length. To ensure correct reading frame, the COI alignment was transferred to MESQUITE v.3.31 (Maddison & Maddison, 2020), translated (echinoderm/flatworm mitochondrial code) and inspected for internal stop codons. After the correct reading frame was determined, the alignment was initially trimmed to the median sequence length. The first column was then removed so that the reading frame began on position one, simplifying position-coding in downstream analyses. Using the COI single-gene alignment, all codon positions in the COI sequences were evaluated for non-stationarity and substitution saturation using χ 2 tests in PAUP (Swofford, 2002) and Xia’s test as implemented in DAMBE6 (Xia et al., 2003; Xia & Lemey, 2009; Xia, 2017), respectively. These tests did not detect significant non-stationarity or substitution saturation for the COI data, thus all codons were used in downstream analyses. Pairwise differences were estimated for the COI, ITS2 and 28S datasets (without outgroup taxa) in MEGA7, employing the number of differences method without variance estimation, including transitions + transversions and complete deletion of gaps and missing data.
Gorgocephalidae and selected outgroup taxa from GenBank used in phylogenetic analyses including host, provenance data, accession numbers and original reference
Digenean . | Host . | Collection Locality . | GenBank Acc. . | Reference . |
---|---|---|---|---|
Gorgocephalidae | ||||
Gorgocephalus kyphosi | Kyphosus vaigiensis | Lizard Island, QLD, Australia | AY222234 | Olson et al. (2003) |
Gorgocephalus yaaji | Kyphosus cinerascens | Lizard Island, QLD, Australia | KU951489–90 | Huston et al. (2016) |
Echinolittorina austrotrochoides | Lizard Island, QLD, Australia | KU951487–88 | Huston et al. (2016) | |
Gorgocephalus yaaji as ‘Gorgocephalus sp. Aus’ (synonym) | Austrolittorina unifasciata | Gerringong, NSW, Australia | KP903414 | O’Dwyer et al. (2015) |
Austrolittorina unifasciata | Kioloa, NSW, Australia | KU951485–86 | Huston et al. (2016) | |
Outgroup | ||||
Endochortophagus protoporus | Kyphosus cornelii | Point Peron, WA, Australia | MK396257 | Huston et al. (2019b) |
Lepotrema moretonense | Prionurus microlepidotus | Moreton Bay, QLD, Australia | MH730023 | Bray et al. (2018b) |
Digenean . | Host . | Collection Locality . | GenBank Acc. . | Reference . |
---|---|---|---|---|
Gorgocephalidae | ||||
Gorgocephalus kyphosi | Kyphosus vaigiensis | Lizard Island, QLD, Australia | AY222234 | Olson et al. (2003) |
Gorgocephalus yaaji | Kyphosus cinerascens | Lizard Island, QLD, Australia | KU951489–90 | Huston et al. (2016) |
Echinolittorina austrotrochoides | Lizard Island, QLD, Australia | KU951487–88 | Huston et al. (2016) | |
Gorgocephalus yaaji as ‘Gorgocephalus sp. Aus’ (synonym) | Austrolittorina unifasciata | Gerringong, NSW, Australia | KP903414 | O’Dwyer et al. (2015) |
Austrolittorina unifasciata | Kioloa, NSW, Australia | KU951485–86 | Huston et al. (2016) | |
Outgroup | ||||
Endochortophagus protoporus | Kyphosus cornelii | Point Peron, WA, Australia | MK396257 | Huston et al. (2019b) |
Lepotrema moretonense | Prionurus microlepidotus | Moreton Bay, QLD, Australia | MH730023 | Bray et al. (2018b) |
Gorgocephalidae and selected outgroup taxa from GenBank used in phylogenetic analyses including host, provenance data, accession numbers and original reference
Digenean . | Host . | Collection Locality . | GenBank Acc. . | Reference . |
---|---|---|---|---|
Gorgocephalidae | ||||
Gorgocephalus kyphosi | Kyphosus vaigiensis | Lizard Island, QLD, Australia | AY222234 | Olson et al. (2003) |
Gorgocephalus yaaji | Kyphosus cinerascens | Lizard Island, QLD, Australia | KU951489–90 | Huston et al. (2016) |
Echinolittorina austrotrochoides | Lizard Island, QLD, Australia | KU951487–88 | Huston et al. (2016) | |
Gorgocephalus yaaji as ‘Gorgocephalus sp. Aus’ (synonym) | Austrolittorina unifasciata | Gerringong, NSW, Australia | KP903414 | O’Dwyer et al. (2015) |
Austrolittorina unifasciata | Kioloa, NSW, Australia | KU951485–86 | Huston et al. (2016) | |
Outgroup | ||||
Endochortophagus protoporus | Kyphosus cornelii | Point Peron, WA, Australia | MK396257 | Huston et al. (2019b) |
Lepotrema moretonense | Prionurus microlepidotus | Moreton Bay, QLD, Australia | MH730023 | Bray et al. (2018b) |
Digenean . | Host . | Collection Locality . | GenBank Acc. . | Reference . |
---|---|---|---|---|
Gorgocephalidae | ||||
Gorgocephalus kyphosi | Kyphosus vaigiensis | Lizard Island, QLD, Australia | AY222234 | Olson et al. (2003) |
Gorgocephalus yaaji | Kyphosus cinerascens | Lizard Island, QLD, Australia | KU951489–90 | Huston et al. (2016) |
Echinolittorina austrotrochoides | Lizard Island, QLD, Australia | KU951487–88 | Huston et al. (2016) | |
Gorgocephalus yaaji as ‘Gorgocephalus sp. Aus’ (synonym) | Austrolittorina unifasciata | Gerringong, NSW, Australia | KP903414 | O’Dwyer et al. (2015) |
Austrolittorina unifasciata | Kioloa, NSW, Australia | KU951485–86 | Huston et al. (2016) | |
Outgroup | ||||
Endochortophagus protoporus | Kyphosus cornelii | Point Peron, WA, Australia | MK396257 | Huston et al. (2019b) |
Lepotrema moretonense | Prionurus microlepidotus | Moreton Bay, QLD, Australia | MH730023 | Bray et al. (2018b) |
Phylogenetic analyses were performed on XSEDE (Towns et al., 2014), accessed through the CIPRES portal (Miller et al., 2010), for three single-gene datasets (COI, ITS2, 28S) and a concatenated dataset (COI + ITS2 + 28S). Nucleotide substitution models for phylogenetic analyses (Supporting Information, Table S3) were selected using PartitionFinder v.2.1.1, employing the Akaike’s information criterion (AICc), the greedy algorithm and PhyML (Guindon et al., 2010; Lanfear et al., 2012; Lanfear et al., 2017). Majority-rule consensus trees were constructed using Bayesian inference (BI) and maximum likelihood (ML) analyses. Bayesian inference was performed using MrBayes v.3.2.6 (Ronquist et al., 2012) with default priors and four chains sampled every 1000 generations of 10 000 000 generations; the first 2500 samples were discarded as burn-in. Maximum likelihood analyses were performed using RAxML (Stamatakis, 2014) with 1000 bootstrap pseudoreplicates. Combined BI/ML trees were edited and annotated in Adobe Illustrator CS6.
Data accessibility
All data underlying morphological and molecular analyses, including raw morphometric data, R code, sequence alignments, phylogenetic files and trees, have been uploaded to the Zenodo data repository with the doi:10.5281/zenodo.4435227.
RESULTS
Approximately 50% of the kyphosids examined between 2015 and 2018 were infected with gorgocephalids (Supporting Information, Table S4), although true prevalence was likely higher as low-intensity infections are easily overlooked. Adult gorgocephalids were recovered from Kyphosus cinerascens, K. elegans (Peters, 1869), K. gladius Knudsen & Clements, 2013, K. sydneyanus and K. vaigiensis collected from six IWP localities (Fig. 1), but not from Kyphosus bigibbus Lacepède, 1801 (N = 23), K. cornelii (Whitley, 1944) (N = 7) or from a fish collected off Japan. Additionally, 1893 littorinid gastropods, comprising eight species, were collected from five localities between 2016 and 2018 (Supporting Information, Table S1). No infections were found among 120 and 101 A. unifasciata collected from Noosa Heads, Queensland, Australia and the Yorke Peninsula, South Australia, respectively. Furthermore, no infections were detected from 202 Nodilittorina pyramidalis (Quoy & Gaimard, 1833) collected from Noosa Heads or from 108 Littoraria articulata (Philippi, 1846) and three Littoraria luteola (Quoy & Gaimard, 1833) collected in Moreton Bay, Queensland, Australia. Fifteen individual littorinids were found infected with gorgocephalids: six Bembicium auratum (Quoy & Gaimard, 1834) from Moreton Bay; two Echinolittorina vidua (Gould, 1859) from Lizard Island, GBR, Australia; and seven Echinolittorina cinerea (Pease, 1869) from Rangiroa Atoll, in the Tuamotu Islands, French Polynesia. Infection prevalence from these hosts and sites were 0.6%, 2% and 35%, respectively. These infections were successfully matched to adult gorgocephalids using molecular methods (see below).
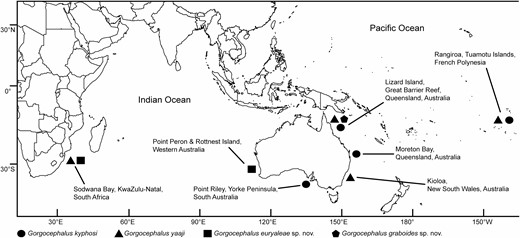
Map of Indo-West Pacific collection localities where specimens of the digenean family Gorgocephalidae were obtained for the present study. Adult specimens were obtained at all localities except for Kioloa, NSW, Australia. At this locality, intramolluscan specimens had been obtained from littorinid gastropods for the study of Huston et al. (2016); genomic DNA from this previous study was used for the generation of additional COI mtDNA gene sequences in the present work.
Species recognition
Traditional morphometric and principal component analyses of adult specimens from across all of our IWP collections suggested the presence of only two morphotypes (Fig. 2; Tables 2–4): a narrow, cylindrical morphotype and a robust, dorsoventrally flattened morphotype. These two morphotypes broadly correspond to the two species described from the region, Gorgocephalus kyphosi and G. yaaji, respectively. However, phylogenetic analyses (Figs 3–6) suggested the presence of between four and eight species in our collection, depending upon the gene-region utilized and the interpretation of the resultant trees. Reassessment of the morphology of adult specimens in light of molecular data led to the identification of features allowing delineation of additional species. Following an integrative approach, we recognize the presence of four species of the family Gorgocephalidae in our IWP collections: Gorgocephalus euryaleae sp. nov., G. graboides sp. nov., G. kyphosi and G. yaaji.
Morphometric data for Gorgocephalus kyphosi expressed as a range and mean in micrometres or as percentages. Number of specimens measured presented as total (whole mounts + hologenophores). A dash (-) indicates distance between two features. Abbreviations: Bd, body; L, length; B, breadth; FBd, forebody; OS, oral sucker; ant, measurement taken at anterior; post, measurement taken at posterior; VS, ventral sucker; PPh, prepharynx; Ph, pharynx; Oe, oesophagus; Cae, caecum; PCae, post-caecal space; Csac, cirrus-sac; AT, anterior testis; PT, posterior testis; Ptest, post-testicular space; OV, ovary; Previt, previtteline region; Postvit, postvitteline region; VitOcc, vitellarium occupies. Egg measurements represent the mean obtained from multiple subsamples per specimen. An asterisk (*) next to a feature indicates it was included in the PCA
Species . | Gorgocephalus kyphosi . | Gorgocephalus kyphosi . | Gorgocephalus kyphosi . | Gorgocephalus kyphosi . |
---|---|---|---|---|
Locality | Point Riley, SA, Australia | Moreton Bay, QLD, Australia | Lizard Island, QLD, Australia | Rangiroa, French Polynesia |
Host(s) | Kyphosus sydneyanus | Kyphosus cinerascens | Kyphosus cinerascens; K. vaigiensis | Kyphosus cinerascens; K. elegans |
No. Measured | 13 (10 + 3) | 13 (10 + 3) | 15 (12 + 3) | 16 (10 + 6) |
BdL* | 1050–1354 (1172) | 1189–2046 (1756) | 1412–1894 (1735) | 1082–1644 (1343) |
BdB* | 123–177 (145) | 145–315 (251) | 135–319 (200) | 99–200 (153) |
BdL / BdB | 6.48–9.40 (8.21) | 5.21–7.43 (6.66) | 8.51–13.27 (9.98) | 7.35–11.38 (8.91) |
BdB % BdL | 11–15 (12) | 13–19 (15) | 8–12 (10) | 9–14 (11) |
FBdL* | 330–425 (361) | 396–542 (466) | 368–615 (456) | 287–453 (371) |
FBdL % BdL | 26–34 (31) | 25–37 (28) | 22–30 (26) | 22–29 (26) |
OSL* | 143–202 (170) | 146–240 (212) | 136–198 (163) | 124–172 (147) |
OSB ant* | 93–138 (113) | 129–165 (150) | 109–163 (122) | 95–136 (114) |
OSB post* | 55–80 (71) | 63–97 (83) | 60–96 (70) | 51–76 (64) |
OSL % BdL | 13–17 (15) | 11–19 (13) | 7–10 (9) | 9–13 (11) |
OSL / OSB ant | 1.30–1.65 (1.51) | 1.13–1.56 (1.41) | 1.21–1.55 (1.36) | 1.13–1.53 (1.3) |
OSL / OSB post | 2.13–2.95 (2.41) | 2.17–2.93 (2.56) | 1.94–2.70 (2.34) | 2.06–2.6 (2.33) |
VSL* | 57–72 (64) | 63–105 (85) | 54–95 (68) | 50–68 (59) |
VSB* | 57–71 (62) | 50–116 (88) | 41–91 (64) | 40–71 (59) |
VSL % BdL | 4.7–6.2 (5.5) | 4.5–7.5 (5.2) | 3.2–4.4 (3.7) | 3.9–4.8 (4.3) |
VSL / VSB | 0.90–1.16 (1.03) | 0.90–1.26 (0.98) | 0.93–1.32 (1.07) | 0.89–1.3 (1.01) |
VSB / OSB ant | 0.49–0.69 (0.55) | 0.39–0.70 (0.58) | 0.38–0.61 (0.52) | 0.36–0.62 (0.52) |
VSB / OSB post | 0.74–1.07 (0.88) | 0.79–1.21 (1.06) | 0.68–1.10 (0.91) | 0.71–1.10 (0.93) |
VSL / OSL | 0.33–0.44 (0.38) | 0.34–0.45 (0.41) | 0.34–0.51 (0.42) | 0.34–0.47 (0.40) |
PPhL | up to 84 (59) | up to 81 (51) | up to 127 (67) | up to 82 (48) |
PhL* | 53–77 (66) | 64–109 (92) | 51–88 (63) | 46–65 (55) |
PhB* | 53–77 (66) | 71–97 (84) | 60–95 (69) | 47–78 (56) |
PhL % BdL | 4.7–7.3 (5.8) | 4.4–8.4 (5.5) | 2.7–4.0 (3.4) | 3.5–4.5 (4.1) |
PhL / PhB | 0.83–1.45 (1.03) | 0.90–1.33 (1.09) | 0.76–1.04 (0.92) | 0.82–1.18 (0.99) |
PhL / OSL | 0.30–0.49 (0.39) | 0.37–0.49 (0.43) | 0.31–0.46 (0.39) | 0.30–0.45 (0.37) |
PhB / OSB ant | 0.45–0.69 (0.58) | 0.47–0.71 (0.56) | 0.52–0.64 (0.57) | 0.41–0.63 (0.5) |
PhB / OSB post | 0.70–1.27 (0.94) | 0.81–1.21 (1.02) | 0.88–1.22 (0.99) | 0.72–1.11 (0.89) |
OesL | 38–81 (64) | 50–99 (74) | 24–116 (68) | 41–94 (71) |
CaeL | 643–951 (770) | 1295–1409 (1360) | 1145–1507 (1360) | 802–1262 (994) |
CaeB | 39–88 (54) | 41–72 (54) | 36–88 (49) | 30–54 (37) |
CaeL % BdL | 61–71 (66) | 73–78 (75) | 77–83 (79) | 72–78 (75) |
CaeL / CaeB | 8.8–24.4 (15.6) | 24.6–27.6 (26.6) | 21.3–34.3 (30.4) | 21.5–35.1 (27.7) |
PCae | 71–133 (105) | 123–143 (135) | 67–119 (92) | 75–130 (98) |
PCae % BdL | 6.8–10.7 (8.9) | 6.5–8.0 (7.4) | 3.6–6.9 (5.5) | 5.8–10.5 (7.5) |
CsacL* | 224–344 (298) | 472–650 (534) | 315–616 (435) | 333–531 (403) |
CsacB* | 34–47 (41) | 39–81 (67) | 49–118 (70) | 41–76 (58) |
CsacL % BdL | 21–28 (26) | 27–40 (31) | 20–26 (24) | 27–34 (29) |
CsacL / CsacB | 6.2–10.1 (7.3) | 6.7–9.2 (7.6) | 4.53–7.93 (6.57) | 5.1–8.8 (7.0) |
ATL* | 76–119 (91) | 122–181 (152) | 106–193 (144) | 86–126 (105) |
ATB* | 51–82 (70) | 11–127 (108) | 74–178 (112) | 67–105 (87) |
ATL % BdL | 5.7–11.1 (7.9) | 6.4–14.1 (8.8) | 6.8–8.8 (7.8) | 7.0–9.7 (7.8) |
AT-VS* | 310–456 (376) | 550–796 (687) | 554–957 (712) | 411–672 (536) |
AT-VS % BdL | 29–35 (32) | 33–62 (40) | 35–42 (40) | 33–45 (39) |
PTL* | 73–117 (97) | 146–194 (164) | 110–228 (152) | 96–142 (112) |
PTB* | 56–80 (68) | 104–146 (119) | 86–163 (108) | 67–108 (91) |
PTL % BdL | 5.8–11 (8.4) | 7.9–14 (9.3) | 7.5–9.8 (8.5) | 7.1–10 (8.4) |
Ptes* | 131–269 (198) | 207–327 (272) | 219–336 (278) | 179–354 (223) |
Ptes % BdL | 11–22 (17) | 11–24 (16) | 13–19 (16) | 13–29 (17) |
OVL* | 39–76 (57) | 83–124 (99) | 60–116 (83) | 50–94 (71) |
OVB* | 37–56 (47) | 63–86 (73) | 47–96 (66) | 38–64 (50) |
OVL % BdL | 3.1–5.4 (4.8) | 4.7–8.0 (5.7) | 3.8–5.5 (4.5) | 3.7–6.7 (5.1) |
OV-VS* | 150–319 (245) | 381–600 (505) | 369–678 (513) | 293–514 (398) |
OV-VS % BdL | 19–24 (22) | 23–50 (30) | 25–30 (28) | 23–35 (29) |
OV-AT* | 47–98 (68) | 35–133 (90) | 70–213 (128) | 17–117 (63) |
OV-AT % BdL | 4.5–8.1 (6) | 2.9–6.8 (5.1) | 3.9–8.8 (6.7) | 3.7–7.5 (5.1) |
OS-VS* | 149–241 (184) | 190–324 (249) | 216–414 (292) | 169–528 (241) |
OS-VS % BdL | 11–18 (16) | 11–18 (14) | 14–21 (16) | 12–35 (17) |
OS-OV* | 365–631 (485) | 685–979 (854) | 621–1131 (860) | 488–929 (678) |
OS-OV % BdL | 39–47 (42) | 42–77 (50) | 44–51 (47) | 45–62 (49) |
OS-Vit* | 293–434 (347) | 360–601 (486) | 403–752 (574) | 343–552 (436) |
OS-Vit % BdL | 25–32 (30) | 23–41 (29) | 27–37 (31) | 27–34 (30) |
Previt | 427–658 (529) | 541–821 (702) | 535–947 (736) | 464–716 (586) |
Previt % Bdl | 40–49 (45) | 35–60 (42) | 36–43 (40) | 38–46 (41) |
Postvit | 34–80 (54) | 35–74 (47) | 23–44 (35) | 29–61 (37) |
Postvit % BdL | 3.2–7.5 (4.7) | 2.0–4.5 (2.8) | 1.2–2.7 (2) | 2.1–4.1 (2.8) |
VitOcc % BdL | 47–55 (50) | 36–63 (56) | 54–62 (58) | 52–60 (56) |
EggL | 63–80 (69) | 63–75 (70) | 60–72 (66) | 56–67 (62) |
EggB | 30–39 (35) | 30–39 (35) | 30–43 (37) | 29–39 (32) |
Species . | Gorgocephalus kyphosi . | Gorgocephalus kyphosi . | Gorgocephalus kyphosi . | Gorgocephalus kyphosi . |
---|---|---|---|---|
Locality | Point Riley, SA, Australia | Moreton Bay, QLD, Australia | Lizard Island, QLD, Australia | Rangiroa, French Polynesia |
Host(s) | Kyphosus sydneyanus | Kyphosus cinerascens | Kyphosus cinerascens; K. vaigiensis | Kyphosus cinerascens; K. elegans |
No. Measured | 13 (10 + 3) | 13 (10 + 3) | 15 (12 + 3) | 16 (10 + 6) |
BdL* | 1050–1354 (1172) | 1189–2046 (1756) | 1412–1894 (1735) | 1082–1644 (1343) |
BdB* | 123–177 (145) | 145–315 (251) | 135–319 (200) | 99–200 (153) |
BdL / BdB | 6.48–9.40 (8.21) | 5.21–7.43 (6.66) | 8.51–13.27 (9.98) | 7.35–11.38 (8.91) |
BdB % BdL | 11–15 (12) | 13–19 (15) | 8–12 (10) | 9–14 (11) |
FBdL* | 330–425 (361) | 396–542 (466) | 368–615 (456) | 287–453 (371) |
FBdL % BdL | 26–34 (31) | 25–37 (28) | 22–30 (26) | 22–29 (26) |
OSL* | 143–202 (170) | 146–240 (212) | 136–198 (163) | 124–172 (147) |
OSB ant* | 93–138 (113) | 129–165 (150) | 109–163 (122) | 95–136 (114) |
OSB post* | 55–80 (71) | 63–97 (83) | 60–96 (70) | 51–76 (64) |
OSL % BdL | 13–17 (15) | 11–19 (13) | 7–10 (9) | 9–13 (11) |
OSL / OSB ant | 1.30–1.65 (1.51) | 1.13–1.56 (1.41) | 1.21–1.55 (1.36) | 1.13–1.53 (1.3) |
OSL / OSB post | 2.13–2.95 (2.41) | 2.17–2.93 (2.56) | 1.94–2.70 (2.34) | 2.06–2.6 (2.33) |
VSL* | 57–72 (64) | 63–105 (85) | 54–95 (68) | 50–68 (59) |
VSB* | 57–71 (62) | 50–116 (88) | 41–91 (64) | 40–71 (59) |
VSL % BdL | 4.7–6.2 (5.5) | 4.5–7.5 (5.2) | 3.2–4.4 (3.7) | 3.9–4.8 (4.3) |
VSL / VSB | 0.90–1.16 (1.03) | 0.90–1.26 (0.98) | 0.93–1.32 (1.07) | 0.89–1.3 (1.01) |
VSB / OSB ant | 0.49–0.69 (0.55) | 0.39–0.70 (0.58) | 0.38–0.61 (0.52) | 0.36–0.62 (0.52) |
VSB / OSB post | 0.74–1.07 (0.88) | 0.79–1.21 (1.06) | 0.68–1.10 (0.91) | 0.71–1.10 (0.93) |
VSL / OSL | 0.33–0.44 (0.38) | 0.34–0.45 (0.41) | 0.34–0.51 (0.42) | 0.34–0.47 (0.40) |
PPhL | up to 84 (59) | up to 81 (51) | up to 127 (67) | up to 82 (48) |
PhL* | 53–77 (66) | 64–109 (92) | 51–88 (63) | 46–65 (55) |
PhB* | 53–77 (66) | 71–97 (84) | 60–95 (69) | 47–78 (56) |
PhL % BdL | 4.7–7.3 (5.8) | 4.4–8.4 (5.5) | 2.7–4.0 (3.4) | 3.5–4.5 (4.1) |
PhL / PhB | 0.83–1.45 (1.03) | 0.90–1.33 (1.09) | 0.76–1.04 (0.92) | 0.82–1.18 (0.99) |
PhL / OSL | 0.30–0.49 (0.39) | 0.37–0.49 (0.43) | 0.31–0.46 (0.39) | 0.30–0.45 (0.37) |
PhB / OSB ant | 0.45–0.69 (0.58) | 0.47–0.71 (0.56) | 0.52–0.64 (0.57) | 0.41–0.63 (0.5) |
PhB / OSB post | 0.70–1.27 (0.94) | 0.81–1.21 (1.02) | 0.88–1.22 (0.99) | 0.72–1.11 (0.89) |
OesL | 38–81 (64) | 50–99 (74) | 24–116 (68) | 41–94 (71) |
CaeL | 643–951 (770) | 1295–1409 (1360) | 1145–1507 (1360) | 802–1262 (994) |
CaeB | 39–88 (54) | 41–72 (54) | 36–88 (49) | 30–54 (37) |
CaeL % BdL | 61–71 (66) | 73–78 (75) | 77–83 (79) | 72–78 (75) |
CaeL / CaeB | 8.8–24.4 (15.6) | 24.6–27.6 (26.6) | 21.3–34.3 (30.4) | 21.5–35.1 (27.7) |
PCae | 71–133 (105) | 123–143 (135) | 67–119 (92) | 75–130 (98) |
PCae % BdL | 6.8–10.7 (8.9) | 6.5–8.0 (7.4) | 3.6–6.9 (5.5) | 5.8–10.5 (7.5) |
CsacL* | 224–344 (298) | 472–650 (534) | 315–616 (435) | 333–531 (403) |
CsacB* | 34–47 (41) | 39–81 (67) | 49–118 (70) | 41–76 (58) |
CsacL % BdL | 21–28 (26) | 27–40 (31) | 20–26 (24) | 27–34 (29) |
CsacL / CsacB | 6.2–10.1 (7.3) | 6.7–9.2 (7.6) | 4.53–7.93 (6.57) | 5.1–8.8 (7.0) |
ATL* | 76–119 (91) | 122–181 (152) | 106–193 (144) | 86–126 (105) |
ATB* | 51–82 (70) | 11–127 (108) | 74–178 (112) | 67–105 (87) |
ATL % BdL | 5.7–11.1 (7.9) | 6.4–14.1 (8.8) | 6.8–8.8 (7.8) | 7.0–9.7 (7.8) |
AT-VS* | 310–456 (376) | 550–796 (687) | 554–957 (712) | 411–672 (536) |
AT-VS % BdL | 29–35 (32) | 33–62 (40) | 35–42 (40) | 33–45 (39) |
PTL* | 73–117 (97) | 146–194 (164) | 110–228 (152) | 96–142 (112) |
PTB* | 56–80 (68) | 104–146 (119) | 86–163 (108) | 67–108 (91) |
PTL % BdL | 5.8–11 (8.4) | 7.9–14 (9.3) | 7.5–9.8 (8.5) | 7.1–10 (8.4) |
Ptes* | 131–269 (198) | 207–327 (272) | 219–336 (278) | 179–354 (223) |
Ptes % BdL | 11–22 (17) | 11–24 (16) | 13–19 (16) | 13–29 (17) |
OVL* | 39–76 (57) | 83–124 (99) | 60–116 (83) | 50–94 (71) |
OVB* | 37–56 (47) | 63–86 (73) | 47–96 (66) | 38–64 (50) |
OVL % BdL | 3.1–5.4 (4.8) | 4.7–8.0 (5.7) | 3.8–5.5 (4.5) | 3.7–6.7 (5.1) |
OV-VS* | 150–319 (245) | 381–600 (505) | 369–678 (513) | 293–514 (398) |
OV-VS % BdL | 19–24 (22) | 23–50 (30) | 25–30 (28) | 23–35 (29) |
OV-AT* | 47–98 (68) | 35–133 (90) | 70–213 (128) | 17–117 (63) |
OV-AT % BdL | 4.5–8.1 (6) | 2.9–6.8 (5.1) | 3.9–8.8 (6.7) | 3.7–7.5 (5.1) |
OS-VS* | 149–241 (184) | 190–324 (249) | 216–414 (292) | 169–528 (241) |
OS-VS % BdL | 11–18 (16) | 11–18 (14) | 14–21 (16) | 12–35 (17) |
OS-OV* | 365–631 (485) | 685–979 (854) | 621–1131 (860) | 488–929 (678) |
OS-OV % BdL | 39–47 (42) | 42–77 (50) | 44–51 (47) | 45–62 (49) |
OS-Vit* | 293–434 (347) | 360–601 (486) | 403–752 (574) | 343–552 (436) |
OS-Vit % BdL | 25–32 (30) | 23–41 (29) | 27–37 (31) | 27–34 (30) |
Previt | 427–658 (529) | 541–821 (702) | 535–947 (736) | 464–716 (586) |
Previt % Bdl | 40–49 (45) | 35–60 (42) | 36–43 (40) | 38–46 (41) |
Postvit | 34–80 (54) | 35–74 (47) | 23–44 (35) | 29–61 (37) |
Postvit % BdL | 3.2–7.5 (4.7) | 2.0–4.5 (2.8) | 1.2–2.7 (2) | 2.1–4.1 (2.8) |
VitOcc % BdL | 47–55 (50) | 36–63 (56) | 54–62 (58) | 52–60 (56) |
EggL | 63–80 (69) | 63–75 (70) | 60–72 (66) | 56–67 (62) |
EggB | 30–39 (35) | 30–39 (35) | 30–43 (37) | 29–39 (32) |
Morphometric data for Gorgocephalus kyphosi expressed as a range and mean in micrometres or as percentages. Number of specimens measured presented as total (whole mounts + hologenophores). A dash (-) indicates distance between two features. Abbreviations: Bd, body; L, length; B, breadth; FBd, forebody; OS, oral sucker; ant, measurement taken at anterior; post, measurement taken at posterior; VS, ventral sucker; PPh, prepharynx; Ph, pharynx; Oe, oesophagus; Cae, caecum; PCae, post-caecal space; Csac, cirrus-sac; AT, anterior testis; PT, posterior testis; Ptest, post-testicular space; OV, ovary; Previt, previtteline region; Postvit, postvitteline region; VitOcc, vitellarium occupies. Egg measurements represent the mean obtained from multiple subsamples per specimen. An asterisk (*) next to a feature indicates it was included in the PCA
Species . | Gorgocephalus kyphosi . | Gorgocephalus kyphosi . | Gorgocephalus kyphosi . | Gorgocephalus kyphosi . |
---|---|---|---|---|
Locality | Point Riley, SA, Australia | Moreton Bay, QLD, Australia | Lizard Island, QLD, Australia | Rangiroa, French Polynesia |
Host(s) | Kyphosus sydneyanus | Kyphosus cinerascens | Kyphosus cinerascens; K. vaigiensis | Kyphosus cinerascens; K. elegans |
No. Measured | 13 (10 + 3) | 13 (10 + 3) | 15 (12 + 3) | 16 (10 + 6) |
BdL* | 1050–1354 (1172) | 1189–2046 (1756) | 1412–1894 (1735) | 1082–1644 (1343) |
BdB* | 123–177 (145) | 145–315 (251) | 135–319 (200) | 99–200 (153) |
BdL / BdB | 6.48–9.40 (8.21) | 5.21–7.43 (6.66) | 8.51–13.27 (9.98) | 7.35–11.38 (8.91) |
BdB % BdL | 11–15 (12) | 13–19 (15) | 8–12 (10) | 9–14 (11) |
FBdL* | 330–425 (361) | 396–542 (466) | 368–615 (456) | 287–453 (371) |
FBdL % BdL | 26–34 (31) | 25–37 (28) | 22–30 (26) | 22–29 (26) |
OSL* | 143–202 (170) | 146–240 (212) | 136–198 (163) | 124–172 (147) |
OSB ant* | 93–138 (113) | 129–165 (150) | 109–163 (122) | 95–136 (114) |
OSB post* | 55–80 (71) | 63–97 (83) | 60–96 (70) | 51–76 (64) |
OSL % BdL | 13–17 (15) | 11–19 (13) | 7–10 (9) | 9–13 (11) |
OSL / OSB ant | 1.30–1.65 (1.51) | 1.13–1.56 (1.41) | 1.21–1.55 (1.36) | 1.13–1.53 (1.3) |
OSL / OSB post | 2.13–2.95 (2.41) | 2.17–2.93 (2.56) | 1.94–2.70 (2.34) | 2.06–2.6 (2.33) |
VSL* | 57–72 (64) | 63–105 (85) | 54–95 (68) | 50–68 (59) |
VSB* | 57–71 (62) | 50–116 (88) | 41–91 (64) | 40–71 (59) |
VSL % BdL | 4.7–6.2 (5.5) | 4.5–7.5 (5.2) | 3.2–4.4 (3.7) | 3.9–4.8 (4.3) |
VSL / VSB | 0.90–1.16 (1.03) | 0.90–1.26 (0.98) | 0.93–1.32 (1.07) | 0.89–1.3 (1.01) |
VSB / OSB ant | 0.49–0.69 (0.55) | 0.39–0.70 (0.58) | 0.38–0.61 (0.52) | 0.36–0.62 (0.52) |
VSB / OSB post | 0.74–1.07 (0.88) | 0.79–1.21 (1.06) | 0.68–1.10 (0.91) | 0.71–1.10 (0.93) |
VSL / OSL | 0.33–0.44 (0.38) | 0.34–0.45 (0.41) | 0.34–0.51 (0.42) | 0.34–0.47 (0.40) |
PPhL | up to 84 (59) | up to 81 (51) | up to 127 (67) | up to 82 (48) |
PhL* | 53–77 (66) | 64–109 (92) | 51–88 (63) | 46–65 (55) |
PhB* | 53–77 (66) | 71–97 (84) | 60–95 (69) | 47–78 (56) |
PhL % BdL | 4.7–7.3 (5.8) | 4.4–8.4 (5.5) | 2.7–4.0 (3.4) | 3.5–4.5 (4.1) |
PhL / PhB | 0.83–1.45 (1.03) | 0.90–1.33 (1.09) | 0.76–1.04 (0.92) | 0.82–1.18 (0.99) |
PhL / OSL | 0.30–0.49 (0.39) | 0.37–0.49 (0.43) | 0.31–0.46 (0.39) | 0.30–0.45 (0.37) |
PhB / OSB ant | 0.45–0.69 (0.58) | 0.47–0.71 (0.56) | 0.52–0.64 (0.57) | 0.41–0.63 (0.5) |
PhB / OSB post | 0.70–1.27 (0.94) | 0.81–1.21 (1.02) | 0.88–1.22 (0.99) | 0.72–1.11 (0.89) |
OesL | 38–81 (64) | 50–99 (74) | 24–116 (68) | 41–94 (71) |
CaeL | 643–951 (770) | 1295–1409 (1360) | 1145–1507 (1360) | 802–1262 (994) |
CaeB | 39–88 (54) | 41–72 (54) | 36–88 (49) | 30–54 (37) |
CaeL % BdL | 61–71 (66) | 73–78 (75) | 77–83 (79) | 72–78 (75) |
CaeL / CaeB | 8.8–24.4 (15.6) | 24.6–27.6 (26.6) | 21.3–34.3 (30.4) | 21.5–35.1 (27.7) |
PCae | 71–133 (105) | 123–143 (135) | 67–119 (92) | 75–130 (98) |
PCae % BdL | 6.8–10.7 (8.9) | 6.5–8.0 (7.4) | 3.6–6.9 (5.5) | 5.8–10.5 (7.5) |
CsacL* | 224–344 (298) | 472–650 (534) | 315–616 (435) | 333–531 (403) |
CsacB* | 34–47 (41) | 39–81 (67) | 49–118 (70) | 41–76 (58) |
CsacL % BdL | 21–28 (26) | 27–40 (31) | 20–26 (24) | 27–34 (29) |
CsacL / CsacB | 6.2–10.1 (7.3) | 6.7–9.2 (7.6) | 4.53–7.93 (6.57) | 5.1–8.8 (7.0) |
ATL* | 76–119 (91) | 122–181 (152) | 106–193 (144) | 86–126 (105) |
ATB* | 51–82 (70) | 11–127 (108) | 74–178 (112) | 67–105 (87) |
ATL % BdL | 5.7–11.1 (7.9) | 6.4–14.1 (8.8) | 6.8–8.8 (7.8) | 7.0–9.7 (7.8) |
AT-VS* | 310–456 (376) | 550–796 (687) | 554–957 (712) | 411–672 (536) |
AT-VS % BdL | 29–35 (32) | 33–62 (40) | 35–42 (40) | 33–45 (39) |
PTL* | 73–117 (97) | 146–194 (164) | 110–228 (152) | 96–142 (112) |
PTB* | 56–80 (68) | 104–146 (119) | 86–163 (108) | 67–108 (91) |
PTL % BdL | 5.8–11 (8.4) | 7.9–14 (9.3) | 7.5–9.8 (8.5) | 7.1–10 (8.4) |
Ptes* | 131–269 (198) | 207–327 (272) | 219–336 (278) | 179–354 (223) |
Ptes % BdL | 11–22 (17) | 11–24 (16) | 13–19 (16) | 13–29 (17) |
OVL* | 39–76 (57) | 83–124 (99) | 60–116 (83) | 50–94 (71) |
OVB* | 37–56 (47) | 63–86 (73) | 47–96 (66) | 38–64 (50) |
OVL % BdL | 3.1–5.4 (4.8) | 4.7–8.0 (5.7) | 3.8–5.5 (4.5) | 3.7–6.7 (5.1) |
OV-VS* | 150–319 (245) | 381–600 (505) | 369–678 (513) | 293–514 (398) |
OV-VS % BdL | 19–24 (22) | 23–50 (30) | 25–30 (28) | 23–35 (29) |
OV-AT* | 47–98 (68) | 35–133 (90) | 70–213 (128) | 17–117 (63) |
OV-AT % BdL | 4.5–8.1 (6) | 2.9–6.8 (5.1) | 3.9–8.8 (6.7) | 3.7–7.5 (5.1) |
OS-VS* | 149–241 (184) | 190–324 (249) | 216–414 (292) | 169–528 (241) |
OS-VS % BdL | 11–18 (16) | 11–18 (14) | 14–21 (16) | 12–35 (17) |
OS-OV* | 365–631 (485) | 685–979 (854) | 621–1131 (860) | 488–929 (678) |
OS-OV % BdL | 39–47 (42) | 42–77 (50) | 44–51 (47) | 45–62 (49) |
OS-Vit* | 293–434 (347) | 360–601 (486) | 403–752 (574) | 343–552 (436) |
OS-Vit % BdL | 25–32 (30) | 23–41 (29) | 27–37 (31) | 27–34 (30) |
Previt | 427–658 (529) | 541–821 (702) | 535–947 (736) | 464–716 (586) |
Previt % Bdl | 40–49 (45) | 35–60 (42) | 36–43 (40) | 38–46 (41) |
Postvit | 34–80 (54) | 35–74 (47) | 23–44 (35) | 29–61 (37) |
Postvit % BdL | 3.2–7.5 (4.7) | 2.0–4.5 (2.8) | 1.2–2.7 (2) | 2.1–4.1 (2.8) |
VitOcc % BdL | 47–55 (50) | 36–63 (56) | 54–62 (58) | 52–60 (56) |
EggL | 63–80 (69) | 63–75 (70) | 60–72 (66) | 56–67 (62) |
EggB | 30–39 (35) | 30–39 (35) | 30–43 (37) | 29–39 (32) |
Species . | Gorgocephalus kyphosi . | Gorgocephalus kyphosi . | Gorgocephalus kyphosi . | Gorgocephalus kyphosi . |
---|---|---|---|---|
Locality | Point Riley, SA, Australia | Moreton Bay, QLD, Australia | Lizard Island, QLD, Australia | Rangiroa, French Polynesia |
Host(s) | Kyphosus sydneyanus | Kyphosus cinerascens | Kyphosus cinerascens; K. vaigiensis | Kyphosus cinerascens; K. elegans |
No. Measured | 13 (10 + 3) | 13 (10 + 3) | 15 (12 + 3) | 16 (10 + 6) |
BdL* | 1050–1354 (1172) | 1189–2046 (1756) | 1412–1894 (1735) | 1082–1644 (1343) |
BdB* | 123–177 (145) | 145–315 (251) | 135–319 (200) | 99–200 (153) |
BdL / BdB | 6.48–9.40 (8.21) | 5.21–7.43 (6.66) | 8.51–13.27 (9.98) | 7.35–11.38 (8.91) |
BdB % BdL | 11–15 (12) | 13–19 (15) | 8–12 (10) | 9–14 (11) |
FBdL* | 330–425 (361) | 396–542 (466) | 368–615 (456) | 287–453 (371) |
FBdL % BdL | 26–34 (31) | 25–37 (28) | 22–30 (26) | 22–29 (26) |
OSL* | 143–202 (170) | 146–240 (212) | 136–198 (163) | 124–172 (147) |
OSB ant* | 93–138 (113) | 129–165 (150) | 109–163 (122) | 95–136 (114) |
OSB post* | 55–80 (71) | 63–97 (83) | 60–96 (70) | 51–76 (64) |
OSL % BdL | 13–17 (15) | 11–19 (13) | 7–10 (9) | 9–13 (11) |
OSL / OSB ant | 1.30–1.65 (1.51) | 1.13–1.56 (1.41) | 1.21–1.55 (1.36) | 1.13–1.53 (1.3) |
OSL / OSB post | 2.13–2.95 (2.41) | 2.17–2.93 (2.56) | 1.94–2.70 (2.34) | 2.06–2.6 (2.33) |
VSL* | 57–72 (64) | 63–105 (85) | 54–95 (68) | 50–68 (59) |
VSB* | 57–71 (62) | 50–116 (88) | 41–91 (64) | 40–71 (59) |
VSL % BdL | 4.7–6.2 (5.5) | 4.5–7.5 (5.2) | 3.2–4.4 (3.7) | 3.9–4.8 (4.3) |
VSL / VSB | 0.90–1.16 (1.03) | 0.90–1.26 (0.98) | 0.93–1.32 (1.07) | 0.89–1.3 (1.01) |
VSB / OSB ant | 0.49–0.69 (0.55) | 0.39–0.70 (0.58) | 0.38–0.61 (0.52) | 0.36–0.62 (0.52) |
VSB / OSB post | 0.74–1.07 (0.88) | 0.79–1.21 (1.06) | 0.68–1.10 (0.91) | 0.71–1.10 (0.93) |
VSL / OSL | 0.33–0.44 (0.38) | 0.34–0.45 (0.41) | 0.34–0.51 (0.42) | 0.34–0.47 (0.40) |
PPhL | up to 84 (59) | up to 81 (51) | up to 127 (67) | up to 82 (48) |
PhL* | 53–77 (66) | 64–109 (92) | 51–88 (63) | 46–65 (55) |
PhB* | 53–77 (66) | 71–97 (84) | 60–95 (69) | 47–78 (56) |
PhL % BdL | 4.7–7.3 (5.8) | 4.4–8.4 (5.5) | 2.7–4.0 (3.4) | 3.5–4.5 (4.1) |
PhL / PhB | 0.83–1.45 (1.03) | 0.90–1.33 (1.09) | 0.76–1.04 (0.92) | 0.82–1.18 (0.99) |
PhL / OSL | 0.30–0.49 (0.39) | 0.37–0.49 (0.43) | 0.31–0.46 (0.39) | 0.30–0.45 (0.37) |
PhB / OSB ant | 0.45–0.69 (0.58) | 0.47–0.71 (0.56) | 0.52–0.64 (0.57) | 0.41–0.63 (0.5) |
PhB / OSB post | 0.70–1.27 (0.94) | 0.81–1.21 (1.02) | 0.88–1.22 (0.99) | 0.72–1.11 (0.89) |
OesL | 38–81 (64) | 50–99 (74) | 24–116 (68) | 41–94 (71) |
CaeL | 643–951 (770) | 1295–1409 (1360) | 1145–1507 (1360) | 802–1262 (994) |
CaeB | 39–88 (54) | 41–72 (54) | 36–88 (49) | 30–54 (37) |
CaeL % BdL | 61–71 (66) | 73–78 (75) | 77–83 (79) | 72–78 (75) |
CaeL / CaeB | 8.8–24.4 (15.6) | 24.6–27.6 (26.6) | 21.3–34.3 (30.4) | 21.5–35.1 (27.7) |
PCae | 71–133 (105) | 123–143 (135) | 67–119 (92) | 75–130 (98) |
PCae % BdL | 6.8–10.7 (8.9) | 6.5–8.0 (7.4) | 3.6–6.9 (5.5) | 5.8–10.5 (7.5) |
CsacL* | 224–344 (298) | 472–650 (534) | 315–616 (435) | 333–531 (403) |
CsacB* | 34–47 (41) | 39–81 (67) | 49–118 (70) | 41–76 (58) |
CsacL % BdL | 21–28 (26) | 27–40 (31) | 20–26 (24) | 27–34 (29) |
CsacL / CsacB | 6.2–10.1 (7.3) | 6.7–9.2 (7.6) | 4.53–7.93 (6.57) | 5.1–8.8 (7.0) |
ATL* | 76–119 (91) | 122–181 (152) | 106–193 (144) | 86–126 (105) |
ATB* | 51–82 (70) | 11–127 (108) | 74–178 (112) | 67–105 (87) |
ATL % BdL | 5.7–11.1 (7.9) | 6.4–14.1 (8.8) | 6.8–8.8 (7.8) | 7.0–9.7 (7.8) |
AT-VS* | 310–456 (376) | 550–796 (687) | 554–957 (712) | 411–672 (536) |
AT-VS % BdL | 29–35 (32) | 33–62 (40) | 35–42 (40) | 33–45 (39) |
PTL* | 73–117 (97) | 146–194 (164) | 110–228 (152) | 96–142 (112) |
PTB* | 56–80 (68) | 104–146 (119) | 86–163 (108) | 67–108 (91) |
PTL % BdL | 5.8–11 (8.4) | 7.9–14 (9.3) | 7.5–9.8 (8.5) | 7.1–10 (8.4) |
Ptes* | 131–269 (198) | 207–327 (272) | 219–336 (278) | 179–354 (223) |
Ptes % BdL | 11–22 (17) | 11–24 (16) | 13–19 (16) | 13–29 (17) |
OVL* | 39–76 (57) | 83–124 (99) | 60–116 (83) | 50–94 (71) |
OVB* | 37–56 (47) | 63–86 (73) | 47–96 (66) | 38–64 (50) |
OVL % BdL | 3.1–5.4 (4.8) | 4.7–8.0 (5.7) | 3.8–5.5 (4.5) | 3.7–6.7 (5.1) |
OV-VS* | 150–319 (245) | 381–600 (505) | 369–678 (513) | 293–514 (398) |
OV-VS % BdL | 19–24 (22) | 23–50 (30) | 25–30 (28) | 23–35 (29) |
OV-AT* | 47–98 (68) | 35–133 (90) | 70–213 (128) | 17–117 (63) |
OV-AT % BdL | 4.5–8.1 (6) | 2.9–6.8 (5.1) | 3.9–8.8 (6.7) | 3.7–7.5 (5.1) |
OS-VS* | 149–241 (184) | 190–324 (249) | 216–414 (292) | 169–528 (241) |
OS-VS % BdL | 11–18 (16) | 11–18 (14) | 14–21 (16) | 12–35 (17) |
OS-OV* | 365–631 (485) | 685–979 (854) | 621–1131 (860) | 488–929 (678) |
OS-OV % BdL | 39–47 (42) | 42–77 (50) | 44–51 (47) | 45–62 (49) |
OS-Vit* | 293–434 (347) | 360–601 (486) | 403–752 (574) | 343–552 (436) |
OS-Vit % BdL | 25–32 (30) | 23–41 (29) | 27–37 (31) | 27–34 (30) |
Previt | 427–658 (529) | 541–821 (702) | 535–947 (736) | 464–716 (586) |
Previt % Bdl | 40–49 (45) | 35–60 (42) | 36–43 (40) | 38–46 (41) |
Postvit | 34–80 (54) | 35–74 (47) | 23–44 (35) | 29–61 (37) |
Postvit % BdL | 3.2–7.5 (4.7) | 2.0–4.5 (2.8) | 1.2–2.7 (2) | 2.1–4.1 (2.8) |
VitOcc % BdL | 47–55 (50) | 36–63 (56) | 54–62 (58) | 52–60 (56) |
EggL | 63–80 (69) | 63–75 (70) | 60–72 (66) | 56–67 (62) |
EggB | 30–39 (35) | 30–39 (35) | 30–43 (37) | 29–39 (32) |
Morphometric data for Gorgocephalus yaaji expressed as a range and mean in micrometres or as percentages. Number of specimens measured presented as total (whole mounts + hologenophores). A dash (-) indicates distance between two features. Abbreviations: Bd, body; L, length; B, breadth; FBd, forebody; OS, oral sucker; ant, measurement taken at anterior; post, measurement taken at posterior; VS, ventral sucker; PPh, prepharynx; Ph, pharynx; Oe, oesophagus; Cae, caecum; PCae, post-caecal space; Csac, cirrus-sac; AT, anterior testis; PT, posterior testis; Ptest, post-testicular space; OV, ovary; Previt, previtteline region; Postvit, postvitteline region; VitOcc, vitellarium occupies. Egg measurements represent the mean obtained from multiple subsamples per specimen. An asterisk (*) next to a feature indicates it was included in the PCA
Species . | Gorgocephalus yaaji . | Gorgocephalus yaaji . | Gorgocephalus yaaji . |
---|---|---|---|
Locality | Lizard Island, QLD, Australia | Sodwana Bay, KZN, South Africa | Rangiroa, French Polynesia |
Host(s) | Kyphosus vaigiensis | Kyphosus cinerascens; K. vaigiensis | Kyphosus cinerascens; K. elegans |
No. Measured | 13 (10 + 3) | 10 (9 + 1) | 10 (7 + 3) |
BdL* | 1419–1683 (1490) | 1172–1561 (1344) | 931–1434 (1056) |
BdB* | 318–387 (354) | 292–392 (346) | 163–294 (221) |
BdL / BdB | 3.87–4.47 (4.24) | 3.32–4.80 (3.96) | 3.59–5.89 (4.92) |
BdB % BdL | 22–26 (24) | 21–30 (26) | 17–28 (21) |
FBdL* | 442–615 (538) | 451–561 (497) | 317–511 (368) |
FBdL % BdL | 31–42 (36) | 34–41 (37) | 32–39 (35) |
OSL* | 185–242 (209) | 195–257 (226) | 161–233 (182) |
OSB ant* | 156–195 (169) | 130–186 (149) | 106–166 (133) |
OSB post* | 101–124 (111) | 88–128 (107) | 63–103 (83) |
OSL % BdL | 13–15 (14) | 14–20 (17) | 16–20 (17) |
OSL / OSB ant | 1.14–1.31 (1.24) | 1.33–1.69 (1.52) | 1.30–1.52 (1.38) |
OSL / OSB post | 1.67–2.1 (1.88) | 1.94–2.46 (2.11) | 2.03–2.59 (2.2) |
VSL* | 80–113 (92) | 74–97 (86) | 65–90 (76) |
VSB* | 85–123 (97) | 75–105 (88) | 65–95 (75) |
VSL % BdL | 5.6–6.7 (6.1) | 5.8–6.9 (6.3) | 5.9–8.7 (7.2) |
VSL / VSB | 0.90–1.07 (0.96) | 0.91–1.08 (0.98) | 0.88–1.22 (1.01) |
VSB / OSB ant | 0.49–0.63 (0.57) | 0.54–0.64 (0.59) | 0.51–0.65 (0.57) |
VSB / OSB post | 0.77–1.02 (0.87) | 0.78–0.86 (0.82) | 0.82–1.03 (0.91) |
VSL / OSL | 0.38–0.47 (0.44) | 0.32–0.44 (0.38) | 0.36–0.47 (0.42) |
PPhL | up to 121 (72) | up to 120 (87) | up to 103 (57) |
PhL* | 90–114 (102) | 79–109 (98) | 67–99 (80) |
PhB* | 93–123 (106) | 84–118 (95) | 62–99 (77) |
PhL % BdL | 6.1–7.8 (6.7) | 6.0–8.6 (7.2) | 6.6–10.2 (7.7) |
PhL / PhB | 0.86–1.16 (0.96) | 0.91–1.18 (1.03) | 0.82–1.27 (1.04) |
PhL / OSL | 0.44–0.55 (0.49) | 0.37–0.51 (0.43) | 0.38–0.53 (0.44) |
PhB / OSB ant | 0.56–0.70 (0.63) | 0.60–0.67 (0.64) | 0.50–0.67 (0.58) |
PhB / OSB post | 0.84–1.09 (0.96) | 0.80–0.98 (0.89) | 0.82–1.03 (0.93) |
OesL | 71–103 (86) | 21–121 (70) | 24–85 (48) |
CaeL | 885–1125 (955) | 628–983 (806) | 523–912 (639) |
CaeB | 59–117 (88) | 59–84 (70) | 34–74 (48) |
CaeL % BdL | 62–67 (64) | 50–66 (60) | 54–64 (60) |
CaeL / CaeB | 8.7–13.8 (11) | 7.5–15.3 (11.6) | 11.9–16.2 (13.7) |
PCae | 135–186 (155) | 135–246 (178) | 94–201 (127) |
PCae % BdL | 9.2–12.8 (10.4) | 9.2–19.7 (13.4) | 9.4–20.7 (12.2) |
CsacL* | 426–579 (506) | 319–596 (475) | 360–597 (427) |
CsacB* | 89–113 (104) | 50–96 (69) | 55–98 (71) |
CsacL % BdL | 30–39 (34) | 26–42 (35) | 38–49 (41) |
CsacL / CsacB | 4.21–5.79 (4.89) | 5.41–9.18 (7.29) | 5.09–7.58 (6.08) |
ATL* | 137–171 (149) | 62–145 (106) | 82–121 (100) |
ATB* | 132–199 (170) | 75–143 (108) | 93–159 (118) |
ATL % BdL | 8.6–11.6 (10) | 5.1–9.9 (7.8) | 8.4–10.9 (9.5) |
AT-VS* | 350–437 (387) | 262–426 (341) | 233–376 (290) |
AT-VS % BdL | 24–28 (26) | 22–28 (25) | 25–32 (28) |
PTL* | 147–193 (170) | 54–157 (108) | 85–162 (121) |
PTB* | 143–197 (175) | 87–144 (114) | 87–155 (113) |
PTL % BdL | 10.1–12.5 (11.4) | 4.4–10.5 (7.8) | 9.1–13.7 (11.4) |
Ptes* | 129–277 (191) | 204–254 (236) | 98–162 (127) |
Ptes % BdL | 9–16 (13) | 14–21 (18) | 10–15 (12) |
OVL* | 83–113 (96) | 49–96 (70) | 46–81 (60) |
OVB* | 72–100 (84) | 45–79 (60) | 49–64 (57) |
OVL % BdL | 5.7–7.2 (6.4) | 3.9–5.9 (5) | 4.9–6.6 (5.7) |
OV-VS* | 203–267 (236) | 158–267 (211) | 138–269 (183) |
OV-VS % BdL | 14–17 (16) | 13–17 (15) | 16–21 (18) |
OV-AT* | 34–78 (56) | 46–92 (73) | 30–55 (43) |
OV-AT % BdL | 2.3–5.3 (3.7) | 3.1–6.6 (5.5) | 2.1–5.7 (4.2) |
OS-VS* | 222–405 (313) | 234–324 (256) | 76–282 (176) |
OS-VS % BdL | 15–28 (21) | 16–23 (19) | 8–21 (16) |
OS-OV* | 535–731 (636) | 486–662 (556) | 354–638 (432) |
OS-OV % BdL | 37–50 (43) | 38–42 (41) | 36–45 (41) |
OS-Vit* | 132–252 (204) | 158–271 (197) | 61–209 (106) |
OS-Vit % BdL | 9–17 (13) | 11–19 (15) | 6–15 (10) |
Previt | 365–547 (435) | 388–527 (430) | 239–455 (309) |
Previt % Bdl | 25–38 (29) | 28–37 (32) | 23–33 (28) |
Postvit | 26–73 (50) | 65–120 (86) | 29–54 (45) |
Postvit % BdL | 1.8–5.0 (3.4) | 4.4–8.6 (6.5) | 3.0–5.3 (4.3) |
VitOcc % BdL | 59–71 (68) | 55–67 (61) | 64–71 (68) |
EggL | 56–65 (62) | 52–69 (61) | 55–63 (59) |
EggB | 28–36 (32) | 22–34 (28) | 25–34 (30) |
Species . | Gorgocephalus yaaji . | Gorgocephalus yaaji . | Gorgocephalus yaaji . |
---|---|---|---|
Locality | Lizard Island, QLD, Australia | Sodwana Bay, KZN, South Africa | Rangiroa, French Polynesia |
Host(s) | Kyphosus vaigiensis | Kyphosus cinerascens; K. vaigiensis | Kyphosus cinerascens; K. elegans |
No. Measured | 13 (10 + 3) | 10 (9 + 1) | 10 (7 + 3) |
BdL* | 1419–1683 (1490) | 1172–1561 (1344) | 931–1434 (1056) |
BdB* | 318–387 (354) | 292–392 (346) | 163–294 (221) |
BdL / BdB | 3.87–4.47 (4.24) | 3.32–4.80 (3.96) | 3.59–5.89 (4.92) |
BdB % BdL | 22–26 (24) | 21–30 (26) | 17–28 (21) |
FBdL* | 442–615 (538) | 451–561 (497) | 317–511 (368) |
FBdL % BdL | 31–42 (36) | 34–41 (37) | 32–39 (35) |
OSL* | 185–242 (209) | 195–257 (226) | 161–233 (182) |
OSB ant* | 156–195 (169) | 130–186 (149) | 106–166 (133) |
OSB post* | 101–124 (111) | 88–128 (107) | 63–103 (83) |
OSL % BdL | 13–15 (14) | 14–20 (17) | 16–20 (17) |
OSL / OSB ant | 1.14–1.31 (1.24) | 1.33–1.69 (1.52) | 1.30–1.52 (1.38) |
OSL / OSB post | 1.67–2.1 (1.88) | 1.94–2.46 (2.11) | 2.03–2.59 (2.2) |
VSL* | 80–113 (92) | 74–97 (86) | 65–90 (76) |
VSB* | 85–123 (97) | 75–105 (88) | 65–95 (75) |
VSL % BdL | 5.6–6.7 (6.1) | 5.8–6.9 (6.3) | 5.9–8.7 (7.2) |
VSL / VSB | 0.90–1.07 (0.96) | 0.91–1.08 (0.98) | 0.88–1.22 (1.01) |
VSB / OSB ant | 0.49–0.63 (0.57) | 0.54–0.64 (0.59) | 0.51–0.65 (0.57) |
VSB / OSB post | 0.77–1.02 (0.87) | 0.78–0.86 (0.82) | 0.82–1.03 (0.91) |
VSL / OSL | 0.38–0.47 (0.44) | 0.32–0.44 (0.38) | 0.36–0.47 (0.42) |
PPhL | up to 121 (72) | up to 120 (87) | up to 103 (57) |
PhL* | 90–114 (102) | 79–109 (98) | 67–99 (80) |
PhB* | 93–123 (106) | 84–118 (95) | 62–99 (77) |
PhL % BdL | 6.1–7.8 (6.7) | 6.0–8.6 (7.2) | 6.6–10.2 (7.7) |
PhL / PhB | 0.86–1.16 (0.96) | 0.91–1.18 (1.03) | 0.82–1.27 (1.04) |
PhL / OSL | 0.44–0.55 (0.49) | 0.37–0.51 (0.43) | 0.38–0.53 (0.44) |
PhB / OSB ant | 0.56–0.70 (0.63) | 0.60–0.67 (0.64) | 0.50–0.67 (0.58) |
PhB / OSB post | 0.84–1.09 (0.96) | 0.80–0.98 (0.89) | 0.82–1.03 (0.93) |
OesL | 71–103 (86) | 21–121 (70) | 24–85 (48) |
CaeL | 885–1125 (955) | 628–983 (806) | 523–912 (639) |
CaeB | 59–117 (88) | 59–84 (70) | 34–74 (48) |
CaeL % BdL | 62–67 (64) | 50–66 (60) | 54–64 (60) |
CaeL / CaeB | 8.7–13.8 (11) | 7.5–15.3 (11.6) | 11.9–16.2 (13.7) |
PCae | 135–186 (155) | 135–246 (178) | 94–201 (127) |
PCae % BdL | 9.2–12.8 (10.4) | 9.2–19.7 (13.4) | 9.4–20.7 (12.2) |
CsacL* | 426–579 (506) | 319–596 (475) | 360–597 (427) |
CsacB* | 89–113 (104) | 50–96 (69) | 55–98 (71) |
CsacL % BdL | 30–39 (34) | 26–42 (35) | 38–49 (41) |
CsacL / CsacB | 4.21–5.79 (4.89) | 5.41–9.18 (7.29) | 5.09–7.58 (6.08) |
ATL* | 137–171 (149) | 62–145 (106) | 82–121 (100) |
ATB* | 132–199 (170) | 75–143 (108) | 93–159 (118) |
ATL % BdL | 8.6–11.6 (10) | 5.1–9.9 (7.8) | 8.4–10.9 (9.5) |
AT-VS* | 350–437 (387) | 262–426 (341) | 233–376 (290) |
AT-VS % BdL | 24–28 (26) | 22–28 (25) | 25–32 (28) |
PTL* | 147–193 (170) | 54–157 (108) | 85–162 (121) |
PTB* | 143–197 (175) | 87–144 (114) | 87–155 (113) |
PTL % BdL | 10.1–12.5 (11.4) | 4.4–10.5 (7.8) | 9.1–13.7 (11.4) |
Ptes* | 129–277 (191) | 204–254 (236) | 98–162 (127) |
Ptes % BdL | 9–16 (13) | 14–21 (18) | 10–15 (12) |
OVL* | 83–113 (96) | 49–96 (70) | 46–81 (60) |
OVB* | 72–100 (84) | 45–79 (60) | 49–64 (57) |
OVL % BdL | 5.7–7.2 (6.4) | 3.9–5.9 (5) | 4.9–6.6 (5.7) |
OV-VS* | 203–267 (236) | 158–267 (211) | 138–269 (183) |
OV-VS % BdL | 14–17 (16) | 13–17 (15) | 16–21 (18) |
OV-AT* | 34–78 (56) | 46–92 (73) | 30–55 (43) |
OV-AT % BdL | 2.3–5.3 (3.7) | 3.1–6.6 (5.5) | 2.1–5.7 (4.2) |
OS-VS* | 222–405 (313) | 234–324 (256) | 76–282 (176) |
OS-VS % BdL | 15–28 (21) | 16–23 (19) | 8–21 (16) |
OS-OV* | 535–731 (636) | 486–662 (556) | 354–638 (432) |
OS-OV % BdL | 37–50 (43) | 38–42 (41) | 36–45 (41) |
OS-Vit* | 132–252 (204) | 158–271 (197) | 61–209 (106) |
OS-Vit % BdL | 9–17 (13) | 11–19 (15) | 6–15 (10) |
Previt | 365–547 (435) | 388–527 (430) | 239–455 (309) |
Previt % Bdl | 25–38 (29) | 28–37 (32) | 23–33 (28) |
Postvit | 26–73 (50) | 65–120 (86) | 29–54 (45) |
Postvit % BdL | 1.8–5.0 (3.4) | 4.4–8.6 (6.5) | 3.0–5.3 (4.3) |
VitOcc % BdL | 59–71 (68) | 55–67 (61) | 64–71 (68) |
EggL | 56–65 (62) | 52–69 (61) | 55–63 (59) |
EggB | 28–36 (32) | 22–34 (28) | 25–34 (30) |
Morphometric data for Gorgocephalus yaaji expressed as a range and mean in micrometres or as percentages. Number of specimens measured presented as total (whole mounts + hologenophores). A dash (-) indicates distance between two features. Abbreviations: Bd, body; L, length; B, breadth; FBd, forebody; OS, oral sucker; ant, measurement taken at anterior; post, measurement taken at posterior; VS, ventral sucker; PPh, prepharynx; Ph, pharynx; Oe, oesophagus; Cae, caecum; PCae, post-caecal space; Csac, cirrus-sac; AT, anterior testis; PT, posterior testis; Ptest, post-testicular space; OV, ovary; Previt, previtteline region; Postvit, postvitteline region; VitOcc, vitellarium occupies. Egg measurements represent the mean obtained from multiple subsamples per specimen. An asterisk (*) next to a feature indicates it was included in the PCA
Species . | Gorgocephalus yaaji . | Gorgocephalus yaaji . | Gorgocephalus yaaji . |
---|---|---|---|
Locality | Lizard Island, QLD, Australia | Sodwana Bay, KZN, South Africa | Rangiroa, French Polynesia |
Host(s) | Kyphosus vaigiensis | Kyphosus cinerascens; K. vaigiensis | Kyphosus cinerascens; K. elegans |
No. Measured | 13 (10 + 3) | 10 (9 + 1) | 10 (7 + 3) |
BdL* | 1419–1683 (1490) | 1172–1561 (1344) | 931–1434 (1056) |
BdB* | 318–387 (354) | 292–392 (346) | 163–294 (221) |
BdL / BdB | 3.87–4.47 (4.24) | 3.32–4.80 (3.96) | 3.59–5.89 (4.92) |
BdB % BdL | 22–26 (24) | 21–30 (26) | 17–28 (21) |
FBdL* | 442–615 (538) | 451–561 (497) | 317–511 (368) |
FBdL % BdL | 31–42 (36) | 34–41 (37) | 32–39 (35) |
OSL* | 185–242 (209) | 195–257 (226) | 161–233 (182) |
OSB ant* | 156–195 (169) | 130–186 (149) | 106–166 (133) |
OSB post* | 101–124 (111) | 88–128 (107) | 63–103 (83) |
OSL % BdL | 13–15 (14) | 14–20 (17) | 16–20 (17) |
OSL / OSB ant | 1.14–1.31 (1.24) | 1.33–1.69 (1.52) | 1.30–1.52 (1.38) |
OSL / OSB post | 1.67–2.1 (1.88) | 1.94–2.46 (2.11) | 2.03–2.59 (2.2) |
VSL* | 80–113 (92) | 74–97 (86) | 65–90 (76) |
VSB* | 85–123 (97) | 75–105 (88) | 65–95 (75) |
VSL % BdL | 5.6–6.7 (6.1) | 5.8–6.9 (6.3) | 5.9–8.7 (7.2) |
VSL / VSB | 0.90–1.07 (0.96) | 0.91–1.08 (0.98) | 0.88–1.22 (1.01) |
VSB / OSB ant | 0.49–0.63 (0.57) | 0.54–0.64 (0.59) | 0.51–0.65 (0.57) |
VSB / OSB post | 0.77–1.02 (0.87) | 0.78–0.86 (0.82) | 0.82–1.03 (0.91) |
VSL / OSL | 0.38–0.47 (0.44) | 0.32–0.44 (0.38) | 0.36–0.47 (0.42) |
PPhL | up to 121 (72) | up to 120 (87) | up to 103 (57) |
PhL* | 90–114 (102) | 79–109 (98) | 67–99 (80) |
PhB* | 93–123 (106) | 84–118 (95) | 62–99 (77) |
PhL % BdL | 6.1–7.8 (6.7) | 6.0–8.6 (7.2) | 6.6–10.2 (7.7) |
PhL / PhB | 0.86–1.16 (0.96) | 0.91–1.18 (1.03) | 0.82–1.27 (1.04) |
PhL / OSL | 0.44–0.55 (0.49) | 0.37–0.51 (0.43) | 0.38–0.53 (0.44) |
PhB / OSB ant | 0.56–0.70 (0.63) | 0.60–0.67 (0.64) | 0.50–0.67 (0.58) |
PhB / OSB post | 0.84–1.09 (0.96) | 0.80–0.98 (0.89) | 0.82–1.03 (0.93) |
OesL | 71–103 (86) | 21–121 (70) | 24–85 (48) |
CaeL | 885–1125 (955) | 628–983 (806) | 523–912 (639) |
CaeB | 59–117 (88) | 59–84 (70) | 34–74 (48) |
CaeL % BdL | 62–67 (64) | 50–66 (60) | 54–64 (60) |
CaeL / CaeB | 8.7–13.8 (11) | 7.5–15.3 (11.6) | 11.9–16.2 (13.7) |
PCae | 135–186 (155) | 135–246 (178) | 94–201 (127) |
PCae % BdL | 9.2–12.8 (10.4) | 9.2–19.7 (13.4) | 9.4–20.7 (12.2) |
CsacL* | 426–579 (506) | 319–596 (475) | 360–597 (427) |
CsacB* | 89–113 (104) | 50–96 (69) | 55–98 (71) |
CsacL % BdL | 30–39 (34) | 26–42 (35) | 38–49 (41) |
CsacL / CsacB | 4.21–5.79 (4.89) | 5.41–9.18 (7.29) | 5.09–7.58 (6.08) |
ATL* | 137–171 (149) | 62–145 (106) | 82–121 (100) |
ATB* | 132–199 (170) | 75–143 (108) | 93–159 (118) |
ATL % BdL | 8.6–11.6 (10) | 5.1–9.9 (7.8) | 8.4–10.9 (9.5) |
AT-VS* | 350–437 (387) | 262–426 (341) | 233–376 (290) |
AT-VS % BdL | 24–28 (26) | 22–28 (25) | 25–32 (28) |
PTL* | 147–193 (170) | 54–157 (108) | 85–162 (121) |
PTB* | 143–197 (175) | 87–144 (114) | 87–155 (113) |
PTL % BdL | 10.1–12.5 (11.4) | 4.4–10.5 (7.8) | 9.1–13.7 (11.4) |
Ptes* | 129–277 (191) | 204–254 (236) | 98–162 (127) |
Ptes % BdL | 9–16 (13) | 14–21 (18) | 10–15 (12) |
OVL* | 83–113 (96) | 49–96 (70) | 46–81 (60) |
OVB* | 72–100 (84) | 45–79 (60) | 49–64 (57) |
OVL % BdL | 5.7–7.2 (6.4) | 3.9–5.9 (5) | 4.9–6.6 (5.7) |
OV-VS* | 203–267 (236) | 158–267 (211) | 138–269 (183) |
OV-VS % BdL | 14–17 (16) | 13–17 (15) | 16–21 (18) |
OV-AT* | 34–78 (56) | 46–92 (73) | 30–55 (43) |
OV-AT % BdL | 2.3–5.3 (3.7) | 3.1–6.6 (5.5) | 2.1–5.7 (4.2) |
OS-VS* | 222–405 (313) | 234–324 (256) | 76–282 (176) |
OS-VS % BdL | 15–28 (21) | 16–23 (19) | 8–21 (16) |
OS-OV* | 535–731 (636) | 486–662 (556) | 354–638 (432) |
OS-OV % BdL | 37–50 (43) | 38–42 (41) | 36–45 (41) |
OS-Vit* | 132–252 (204) | 158–271 (197) | 61–209 (106) |
OS-Vit % BdL | 9–17 (13) | 11–19 (15) | 6–15 (10) |
Previt | 365–547 (435) | 388–527 (430) | 239–455 (309) |
Previt % Bdl | 25–38 (29) | 28–37 (32) | 23–33 (28) |
Postvit | 26–73 (50) | 65–120 (86) | 29–54 (45) |
Postvit % BdL | 1.8–5.0 (3.4) | 4.4–8.6 (6.5) | 3.0–5.3 (4.3) |
VitOcc % BdL | 59–71 (68) | 55–67 (61) | 64–71 (68) |
EggL | 56–65 (62) | 52–69 (61) | 55–63 (59) |
EggB | 28–36 (32) | 22–34 (28) | 25–34 (30) |
Species . | Gorgocephalus yaaji . | Gorgocephalus yaaji . | Gorgocephalus yaaji . |
---|---|---|---|
Locality | Lizard Island, QLD, Australia | Sodwana Bay, KZN, South Africa | Rangiroa, French Polynesia |
Host(s) | Kyphosus vaigiensis | Kyphosus cinerascens; K. vaigiensis | Kyphosus cinerascens; K. elegans |
No. Measured | 13 (10 + 3) | 10 (9 + 1) | 10 (7 + 3) |
BdL* | 1419–1683 (1490) | 1172–1561 (1344) | 931–1434 (1056) |
BdB* | 318–387 (354) | 292–392 (346) | 163–294 (221) |
BdL / BdB | 3.87–4.47 (4.24) | 3.32–4.80 (3.96) | 3.59–5.89 (4.92) |
BdB % BdL | 22–26 (24) | 21–30 (26) | 17–28 (21) |
FBdL* | 442–615 (538) | 451–561 (497) | 317–511 (368) |
FBdL % BdL | 31–42 (36) | 34–41 (37) | 32–39 (35) |
OSL* | 185–242 (209) | 195–257 (226) | 161–233 (182) |
OSB ant* | 156–195 (169) | 130–186 (149) | 106–166 (133) |
OSB post* | 101–124 (111) | 88–128 (107) | 63–103 (83) |
OSL % BdL | 13–15 (14) | 14–20 (17) | 16–20 (17) |
OSL / OSB ant | 1.14–1.31 (1.24) | 1.33–1.69 (1.52) | 1.30–1.52 (1.38) |
OSL / OSB post | 1.67–2.1 (1.88) | 1.94–2.46 (2.11) | 2.03–2.59 (2.2) |
VSL* | 80–113 (92) | 74–97 (86) | 65–90 (76) |
VSB* | 85–123 (97) | 75–105 (88) | 65–95 (75) |
VSL % BdL | 5.6–6.7 (6.1) | 5.8–6.9 (6.3) | 5.9–8.7 (7.2) |
VSL / VSB | 0.90–1.07 (0.96) | 0.91–1.08 (0.98) | 0.88–1.22 (1.01) |
VSB / OSB ant | 0.49–0.63 (0.57) | 0.54–0.64 (0.59) | 0.51–0.65 (0.57) |
VSB / OSB post | 0.77–1.02 (0.87) | 0.78–0.86 (0.82) | 0.82–1.03 (0.91) |
VSL / OSL | 0.38–0.47 (0.44) | 0.32–0.44 (0.38) | 0.36–0.47 (0.42) |
PPhL | up to 121 (72) | up to 120 (87) | up to 103 (57) |
PhL* | 90–114 (102) | 79–109 (98) | 67–99 (80) |
PhB* | 93–123 (106) | 84–118 (95) | 62–99 (77) |
PhL % BdL | 6.1–7.8 (6.7) | 6.0–8.6 (7.2) | 6.6–10.2 (7.7) |
PhL / PhB | 0.86–1.16 (0.96) | 0.91–1.18 (1.03) | 0.82–1.27 (1.04) |
PhL / OSL | 0.44–0.55 (0.49) | 0.37–0.51 (0.43) | 0.38–0.53 (0.44) |
PhB / OSB ant | 0.56–0.70 (0.63) | 0.60–0.67 (0.64) | 0.50–0.67 (0.58) |
PhB / OSB post | 0.84–1.09 (0.96) | 0.80–0.98 (0.89) | 0.82–1.03 (0.93) |
OesL | 71–103 (86) | 21–121 (70) | 24–85 (48) |
CaeL | 885–1125 (955) | 628–983 (806) | 523–912 (639) |
CaeB | 59–117 (88) | 59–84 (70) | 34–74 (48) |
CaeL % BdL | 62–67 (64) | 50–66 (60) | 54–64 (60) |
CaeL / CaeB | 8.7–13.8 (11) | 7.5–15.3 (11.6) | 11.9–16.2 (13.7) |
PCae | 135–186 (155) | 135–246 (178) | 94–201 (127) |
PCae % BdL | 9.2–12.8 (10.4) | 9.2–19.7 (13.4) | 9.4–20.7 (12.2) |
CsacL* | 426–579 (506) | 319–596 (475) | 360–597 (427) |
CsacB* | 89–113 (104) | 50–96 (69) | 55–98 (71) |
CsacL % BdL | 30–39 (34) | 26–42 (35) | 38–49 (41) |
CsacL / CsacB | 4.21–5.79 (4.89) | 5.41–9.18 (7.29) | 5.09–7.58 (6.08) |
ATL* | 137–171 (149) | 62–145 (106) | 82–121 (100) |
ATB* | 132–199 (170) | 75–143 (108) | 93–159 (118) |
ATL % BdL | 8.6–11.6 (10) | 5.1–9.9 (7.8) | 8.4–10.9 (9.5) |
AT-VS* | 350–437 (387) | 262–426 (341) | 233–376 (290) |
AT-VS % BdL | 24–28 (26) | 22–28 (25) | 25–32 (28) |
PTL* | 147–193 (170) | 54–157 (108) | 85–162 (121) |
PTB* | 143–197 (175) | 87–144 (114) | 87–155 (113) |
PTL % BdL | 10.1–12.5 (11.4) | 4.4–10.5 (7.8) | 9.1–13.7 (11.4) |
Ptes* | 129–277 (191) | 204–254 (236) | 98–162 (127) |
Ptes % BdL | 9–16 (13) | 14–21 (18) | 10–15 (12) |
OVL* | 83–113 (96) | 49–96 (70) | 46–81 (60) |
OVB* | 72–100 (84) | 45–79 (60) | 49–64 (57) |
OVL % BdL | 5.7–7.2 (6.4) | 3.9–5.9 (5) | 4.9–6.6 (5.7) |
OV-VS* | 203–267 (236) | 158–267 (211) | 138–269 (183) |
OV-VS % BdL | 14–17 (16) | 13–17 (15) | 16–21 (18) |
OV-AT* | 34–78 (56) | 46–92 (73) | 30–55 (43) |
OV-AT % BdL | 2.3–5.3 (3.7) | 3.1–6.6 (5.5) | 2.1–5.7 (4.2) |
OS-VS* | 222–405 (313) | 234–324 (256) | 76–282 (176) |
OS-VS % BdL | 15–28 (21) | 16–23 (19) | 8–21 (16) |
OS-OV* | 535–731 (636) | 486–662 (556) | 354–638 (432) |
OS-OV % BdL | 37–50 (43) | 38–42 (41) | 36–45 (41) |
OS-Vit* | 132–252 (204) | 158–271 (197) | 61–209 (106) |
OS-Vit % BdL | 9–17 (13) | 11–19 (15) | 6–15 (10) |
Previt | 365–547 (435) | 388–527 (430) | 239–455 (309) |
Previt % Bdl | 25–38 (29) | 28–37 (32) | 23–33 (28) |
Postvit | 26–73 (50) | 65–120 (86) | 29–54 (45) |
Postvit % BdL | 1.8–5.0 (3.4) | 4.4–8.6 (6.5) | 3.0–5.3 (4.3) |
VitOcc % BdL | 59–71 (68) | 55–67 (61) | 64–71 (68) |
EggL | 56–65 (62) | 52–69 (61) | 55–63 (59) |
EggB | 28–36 (32) | 22–34 (28) | 25–34 (30) |
Morphometric data for two new species of Gorgocephalus expressed as a range and mean in micrometres or as percentages. Number of specimens measured presented as total (whole mounts + hologenophores). A dash (-) indicates distance between two features. Abbreviations: Bd, body; L, length; B, breadth; FBd, forebody; OS, oral sucker; ant, measurement taken at anterior; post, measurement taken at posterior; VS, ventral sucker; PPh, prepharynx; Ph, pharynx; Oe, oesophagus; Cae, caecum; PCae, post-caecal space; Csac, cirrus-sac; AT, anterior testis; PT, posterior testis; Ptest, post-testicular space; OV, ovary; Previt, previtteline region; Postvit, postvitteline region; VitOcc, vitellarium occupies. Egg measurements represent the mean obtained from multiple subsamples per specimen. An asterisk (*) next to a feature indicates it was included in the PCA
Species . | Gorgocephalus euryaleae . | Gorgocephalus euryaleae . | Gorgocephalus graboides . |
---|---|---|---|
Locality | Point Peron and Rottnest Island, Western Australia | Sodwana Bay, KZN, South Africa | Lizard Island, QLD, Australia |
Host(s) | Kyphosus gladius; Kyphosus sydneyanus | Kyphosus cinerascens | Kyphosus cinerascens |
No. Measured | 15 (10 + 5) | 10 (8 + 2) | 15 (12 + 3) |
BdL* | 1071–1555 (1242) | 920–1463 (1168) | 1109–1768 (1326) |
BdB* | 129–210 (155) | 134–213 (180) | 100–201 (136) |
BdL / BdB | 7.17–9.62 (8.19) | 5.97–7.91 (6.83) | 9.02–12.59 (10.65) |
BdB % BdL | 10–14 (12) | 13–17 (15) | 8–11 (9) |
FBdL* | 352–463 (399) | 300–400 (359) | 271–387 (311) |
FBdL % BdL | 27–34 (32) | 27–33 (31) | 21–26 (24) |
OSL* | 141–184 (167) | 134–161 (147) | 140–194 (159) |
OSB ant* | 108–143 (128) | 91–106 (100) | 97–130 (111) |
OSB post* | 66–83 (75) | 49–65 (58) | 48–69 (58) |
OSL % BdL | 11–16 (13) | 11–15 (13) | 10–13 (12) |
OSL / OSB ant | 1.10–1.44 (1.31) | 1.40–1.61 (1.48) | 1.24–1.66 (1.44) |
OSL / OSB post | 1.98–2.46 (2.23) | 2.25–2.98 (2.56) | 2.25–3.12 (2.76) |
VSL* | 54–72 (61) | 53–68 (60) | 50–78 (61) |
VSB* | 52–70 (62) | 51–66 (59) | 49–83 (62) |
VSL % BdL | 4.3–5.5 (4.9) | 4.3–5.8 (5.1) | 4.1–4.9 (4.5) |
VSL / VSB | 0.88–1.1 (0.99) | 0.95–1.11 (1.02) | 0.88–1.14 (0.99) |
VSB / OSB ant | 0.42–0.53 (0.48) | 0.54–0.64 (0.59) | 0.46–0.68 (0.56) |
VSB / OSB post | 0.72–0.89 (0.82) | 0.96–1.24 (1.03) | 0.83–1.32 (1.07) |
VSL / OSL | 0.30–0.42 (0.37) | 0.37–0.44 (0.41) | 0.32–0.47 (0.38) |
PPhL | up to 74 (54) | up to 64 (39) | up to 72 (44) |
PhL* | 44–71 (56) | 48–64 (55) | 38–67 (50) |
PhB* | 58–83 (67) | 44–59 (51) | 49–78 (56) |
PhL % BdL | 3.8–5.8 (4.6) | 3.7–5.5 (4.6) | 3.0–4.3 (3.6) |
PhL / PhB | 0.63–1.22 (0.84) | 0.81–1.24 (1.09) | 0.73–1.17 (0.89) |
PhL / OSL | 0.26–0.46 (0.34) | 0.34–0.40 (0.37) | 0.27–0.35 (0.31) |
PhB / OSB ant | 0.41–0.61 (0.53) | 0.46–0.58 (0.51) | 0.42–0.67 (0.50) |
PhB / OSB post | 0.74–1.04 (0.90) | 0.78–1.06 (0.88) | 0.83–1.20 (0.96) |
OesL | 15–87 (61) | 37–66 (52) | 19–93 (51) |
CaeL | 652–1131 (854) | 595–1069 (809) | 749–1323 (1006) |
CaeB | 38–72 (52) | 37–62 (47) | 34–63 (42) |
CaeL % BdL | 60–73 (68) | 65–73 (69) | 66–76 (73) |
CaeL / CaeB | 11.2–20.9 (15.8) | 15.3–23.8 (18.8) | 19.7–30.9 (24.2) |
PCae | 83–165 (118) | 74–161 (109) | 79–147 (103) |
PCae % BdL | 7.1–12.7 (9.2) | 6.4–12.5 (9.2) | 6.2–9.2 (7.6) |
CsacL* | 261–412 (333) | 221–325 (287) | 279–467 (382) |
CsacB* | 51–95 (65) | 35–58 (48) | 41–82 (57) |
CsacL % BdL | 22–30 (26) | 19–28 (24) | 24–31 (28) |
CsacL / CsacB | 4.05–6.22 (5.32) | 4.85–6.72 (5.97) | 5.24–8.44 (6.84) |
ATL* | 84–125 (102) | 77–129 (101) | 66–127 (98) |
ATB* | 74–111 (89) | 70–95 (83) | 64–105 (81) |
ATL % BdL | 6.9–9.2 (8.3) | 7.4–9.6 (8.6) | 5.4–8.5 (7.3) |
AT-VS* | 355–560 (439) | 312–508 (391) | 412–771 (558) |
AT-VS % BdL | 33–37 (35) | 31–36 (33) | 36–44 (41) |
PTL* | 86–132 (105) | 87–152 (113) | 77–141 (102) |
PTB* | 77–110 (92) | 70–100 (83) | 67–101 (81) |
PTL % BdL | 6.9–9.4 (8.5) | 8.1–13.1 (9.7) | 5.9–8.9 (7.7) |
Ptes* | 140–263 (177) | 142–200 (166) | 158–315 (216) |
Ptes % BdL | 12–17 (14) | 12–16 (14) | 13–18 (16) |
OVL* | 58–88 (72) | 45–81 (60) | 50–80 (64) |
OVB* | 50–72 (59) | 38–58 (47) | 39–59 (48) |
OVL % BdL | 5.1–6.5 (5.8) | 4.0–6.3 (5.2) | 3.5–6.4 (4.7) |
OV-VS* | 253–424 (318) | 179–398 (285) | 303–591 (421) |
OV-VS % BdL | 23–30 (25) | 19–27 (24) | 27–33 (30) |
OV-AT* | 29–97 (56) | 34–64 (45) | 45–146 (91) |
OV-AT % BdL | 2.1–7.8 (4.7) | 3.1–4.4 (3.9) | 4.0–8.6 (6.5) |
OS-VS* | 176–262 (217) | 171–234 (202) | 93–201 (145) |
OS-VS % BdL | 15–21 (17) | 15–19 (17) | 8–14 (11) |
OS-OV* | 499–737 (582) | 415–692 (553) | 496–851 (627) |
OS-OV % BdL | 43–54 (47) | 45–51 (47) | 40–49 (45) |
OS-Vit* | 318–445 (378) | 291–424 (351) | 359–611 (455) |
OS-Vit % BdL | 27–35 (30) | 27–32 (29) | 29–38 (33) |
Previt | 501–624 (552) | 437–577 (504) | 506–784 (615) |
Previt % Bdl | 40–48 (45) | 39–48 (43) | 40–49 (45) |
Postvit | 26–55 (36) | 34–60 (44) | 21–49 (34) |
Postvit % BdL | 2.2–3.6 (2.9) | 3.0–5.5 (3.8) | 1.8–3.5 (2.6) |
VitOcc % BdL | 48–56 (53) | 47–57 (53) | 48–59 (52) |
EggL | 56–77 (64) | 56–68 (62) | 53–72 (63) |
EggB | 29–42 (34) | 26–39 (31) | 28–41 (34) |
Species . | Gorgocephalus euryaleae . | Gorgocephalus euryaleae . | Gorgocephalus graboides . |
---|---|---|---|
Locality | Point Peron and Rottnest Island, Western Australia | Sodwana Bay, KZN, South Africa | Lizard Island, QLD, Australia |
Host(s) | Kyphosus gladius; Kyphosus sydneyanus | Kyphosus cinerascens | Kyphosus cinerascens |
No. Measured | 15 (10 + 5) | 10 (8 + 2) | 15 (12 + 3) |
BdL* | 1071–1555 (1242) | 920–1463 (1168) | 1109–1768 (1326) |
BdB* | 129–210 (155) | 134–213 (180) | 100–201 (136) |
BdL / BdB | 7.17–9.62 (8.19) | 5.97–7.91 (6.83) | 9.02–12.59 (10.65) |
BdB % BdL | 10–14 (12) | 13–17 (15) | 8–11 (9) |
FBdL* | 352–463 (399) | 300–400 (359) | 271–387 (311) |
FBdL % BdL | 27–34 (32) | 27–33 (31) | 21–26 (24) |
OSL* | 141–184 (167) | 134–161 (147) | 140–194 (159) |
OSB ant* | 108–143 (128) | 91–106 (100) | 97–130 (111) |
OSB post* | 66–83 (75) | 49–65 (58) | 48–69 (58) |
OSL % BdL | 11–16 (13) | 11–15 (13) | 10–13 (12) |
OSL / OSB ant | 1.10–1.44 (1.31) | 1.40–1.61 (1.48) | 1.24–1.66 (1.44) |
OSL / OSB post | 1.98–2.46 (2.23) | 2.25–2.98 (2.56) | 2.25–3.12 (2.76) |
VSL* | 54–72 (61) | 53–68 (60) | 50–78 (61) |
VSB* | 52–70 (62) | 51–66 (59) | 49–83 (62) |
VSL % BdL | 4.3–5.5 (4.9) | 4.3–5.8 (5.1) | 4.1–4.9 (4.5) |
VSL / VSB | 0.88–1.1 (0.99) | 0.95–1.11 (1.02) | 0.88–1.14 (0.99) |
VSB / OSB ant | 0.42–0.53 (0.48) | 0.54–0.64 (0.59) | 0.46–0.68 (0.56) |
VSB / OSB post | 0.72–0.89 (0.82) | 0.96–1.24 (1.03) | 0.83–1.32 (1.07) |
VSL / OSL | 0.30–0.42 (0.37) | 0.37–0.44 (0.41) | 0.32–0.47 (0.38) |
PPhL | up to 74 (54) | up to 64 (39) | up to 72 (44) |
PhL* | 44–71 (56) | 48–64 (55) | 38–67 (50) |
PhB* | 58–83 (67) | 44–59 (51) | 49–78 (56) |
PhL % BdL | 3.8–5.8 (4.6) | 3.7–5.5 (4.6) | 3.0–4.3 (3.6) |
PhL / PhB | 0.63–1.22 (0.84) | 0.81–1.24 (1.09) | 0.73–1.17 (0.89) |
PhL / OSL | 0.26–0.46 (0.34) | 0.34–0.40 (0.37) | 0.27–0.35 (0.31) |
PhB / OSB ant | 0.41–0.61 (0.53) | 0.46–0.58 (0.51) | 0.42–0.67 (0.50) |
PhB / OSB post | 0.74–1.04 (0.90) | 0.78–1.06 (0.88) | 0.83–1.20 (0.96) |
OesL | 15–87 (61) | 37–66 (52) | 19–93 (51) |
CaeL | 652–1131 (854) | 595–1069 (809) | 749–1323 (1006) |
CaeB | 38–72 (52) | 37–62 (47) | 34–63 (42) |
CaeL % BdL | 60–73 (68) | 65–73 (69) | 66–76 (73) |
CaeL / CaeB | 11.2–20.9 (15.8) | 15.3–23.8 (18.8) | 19.7–30.9 (24.2) |
PCae | 83–165 (118) | 74–161 (109) | 79–147 (103) |
PCae % BdL | 7.1–12.7 (9.2) | 6.4–12.5 (9.2) | 6.2–9.2 (7.6) |
CsacL* | 261–412 (333) | 221–325 (287) | 279–467 (382) |
CsacB* | 51–95 (65) | 35–58 (48) | 41–82 (57) |
CsacL % BdL | 22–30 (26) | 19–28 (24) | 24–31 (28) |
CsacL / CsacB | 4.05–6.22 (5.32) | 4.85–6.72 (5.97) | 5.24–8.44 (6.84) |
ATL* | 84–125 (102) | 77–129 (101) | 66–127 (98) |
ATB* | 74–111 (89) | 70–95 (83) | 64–105 (81) |
ATL % BdL | 6.9–9.2 (8.3) | 7.4–9.6 (8.6) | 5.4–8.5 (7.3) |
AT-VS* | 355–560 (439) | 312–508 (391) | 412–771 (558) |
AT-VS % BdL | 33–37 (35) | 31–36 (33) | 36–44 (41) |
PTL* | 86–132 (105) | 87–152 (113) | 77–141 (102) |
PTB* | 77–110 (92) | 70–100 (83) | 67–101 (81) |
PTL % BdL | 6.9–9.4 (8.5) | 8.1–13.1 (9.7) | 5.9–8.9 (7.7) |
Ptes* | 140–263 (177) | 142–200 (166) | 158–315 (216) |
Ptes % BdL | 12–17 (14) | 12–16 (14) | 13–18 (16) |
OVL* | 58–88 (72) | 45–81 (60) | 50–80 (64) |
OVB* | 50–72 (59) | 38–58 (47) | 39–59 (48) |
OVL % BdL | 5.1–6.5 (5.8) | 4.0–6.3 (5.2) | 3.5–6.4 (4.7) |
OV-VS* | 253–424 (318) | 179–398 (285) | 303–591 (421) |
OV-VS % BdL | 23–30 (25) | 19–27 (24) | 27–33 (30) |
OV-AT* | 29–97 (56) | 34–64 (45) | 45–146 (91) |
OV-AT % BdL | 2.1–7.8 (4.7) | 3.1–4.4 (3.9) | 4.0–8.6 (6.5) |
OS-VS* | 176–262 (217) | 171–234 (202) | 93–201 (145) |
OS-VS % BdL | 15–21 (17) | 15–19 (17) | 8–14 (11) |
OS-OV* | 499–737 (582) | 415–692 (553) | 496–851 (627) |
OS-OV % BdL | 43–54 (47) | 45–51 (47) | 40–49 (45) |
OS-Vit* | 318–445 (378) | 291–424 (351) | 359–611 (455) |
OS-Vit % BdL | 27–35 (30) | 27–32 (29) | 29–38 (33) |
Previt | 501–624 (552) | 437–577 (504) | 506–784 (615) |
Previt % Bdl | 40–48 (45) | 39–48 (43) | 40–49 (45) |
Postvit | 26–55 (36) | 34–60 (44) | 21–49 (34) |
Postvit % BdL | 2.2–3.6 (2.9) | 3.0–5.5 (3.8) | 1.8–3.5 (2.6) |
VitOcc % BdL | 48–56 (53) | 47–57 (53) | 48–59 (52) |
EggL | 56–77 (64) | 56–68 (62) | 53–72 (63) |
EggB | 29–42 (34) | 26–39 (31) | 28–41 (34) |
Morphometric data for two new species of Gorgocephalus expressed as a range and mean in micrometres or as percentages. Number of specimens measured presented as total (whole mounts + hologenophores). A dash (-) indicates distance between two features. Abbreviations: Bd, body; L, length; B, breadth; FBd, forebody; OS, oral sucker; ant, measurement taken at anterior; post, measurement taken at posterior; VS, ventral sucker; PPh, prepharynx; Ph, pharynx; Oe, oesophagus; Cae, caecum; PCae, post-caecal space; Csac, cirrus-sac; AT, anterior testis; PT, posterior testis; Ptest, post-testicular space; OV, ovary; Previt, previtteline region; Postvit, postvitteline region; VitOcc, vitellarium occupies. Egg measurements represent the mean obtained from multiple subsamples per specimen. An asterisk (*) next to a feature indicates it was included in the PCA
Species . | Gorgocephalus euryaleae . | Gorgocephalus euryaleae . | Gorgocephalus graboides . |
---|---|---|---|
Locality | Point Peron and Rottnest Island, Western Australia | Sodwana Bay, KZN, South Africa | Lizard Island, QLD, Australia |
Host(s) | Kyphosus gladius; Kyphosus sydneyanus | Kyphosus cinerascens | Kyphosus cinerascens |
No. Measured | 15 (10 + 5) | 10 (8 + 2) | 15 (12 + 3) |
BdL* | 1071–1555 (1242) | 920–1463 (1168) | 1109–1768 (1326) |
BdB* | 129–210 (155) | 134–213 (180) | 100–201 (136) |
BdL / BdB | 7.17–9.62 (8.19) | 5.97–7.91 (6.83) | 9.02–12.59 (10.65) |
BdB % BdL | 10–14 (12) | 13–17 (15) | 8–11 (9) |
FBdL* | 352–463 (399) | 300–400 (359) | 271–387 (311) |
FBdL % BdL | 27–34 (32) | 27–33 (31) | 21–26 (24) |
OSL* | 141–184 (167) | 134–161 (147) | 140–194 (159) |
OSB ant* | 108–143 (128) | 91–106 (100) | 97–130 (111) |
OSB post* | 66–83 (75) | 49–65 (58) | 48–69 (58) |
OSL % BdL | 11–16 (13) | 11–15 (13) | 10–13 (12) |
OSL / OSB ant | 1.10–1.44 (1.31) | 1.40–1.61 (1.48) | 1.24–1.66 (1.44) |
OSL / OSB post | 1.98–2.46 (2.23) | 2.25–2.98 (2.56) | 2.25–3.12 (2.76) |
VSL* | 54–72 (61) | 53–68 (60) | 50–78 (61) |
VSB* | 52–70 (62) | 51–66 (59) | 49–83 (62) |
VSL % BdL | 4.3–5.5 (4.9) | 4.3–5.8 (5.1) | 4.1–4.9 (4.5) |
VSL / VSB | 0.88–1.1 (0.99) | 0.95–1.11 (1.02) | 0.88–1.14 (0.99) |
VSB / OSB ant | 0.42–0.53 (0.48) | 0.54–0.64 (0.59) | 0.46–0.68 (0.56) |
VSB / OSB post | 0.72–0.89 (0.82) | 0.96–1.24 (1.03) | 0.83–1.32 (1.07) |
VSL / OSL | 0.30–0.42 (0.37) | 0.37–0.44 (0.41) | 0.32–0.47 (0.38) |
PPhL | up to 74 (54) | up to 64 (39) | up to 72 (44) |
PhL* | 44–71 (56) | 48–64 (55) | 38–67 (50) |
PhB* | 58–83 (67) | 44–59 (51) | 49–78 (56) |
PhL % BdL | 3.8–5.8 (4.6) | 3.7–5.5 (4.6) | 3.0–4.3 (3.6) |
PhL / PhB | 0.63–1.22 (0.84) | 0.81–1.24 (1.09) | 0.73–1.17 (0.89) |
PhL / OSL | 0.26–0.46 (0.34) | 0.34–0.40 (0.37) | 0.27–0.35 (0.31) |
PhB / OSB ant | 0.41–0.61 (0.53) | 0.46–0.58 (0.51) | 0.42–0.67 (0.50) |
PhB / OSB post | 0.74–1.04 (0.90) | 0.78–1.06 (0.88) | 0.83–1.20 (0.96) |
OesL | 15–87 (61) | 37–66 (52) | 19–93 (51) |
CaeL | 652–1131 (854) | 595–1069 (809) | 749–1323 (1006) |
CaeB | 38–72 (52) | 37–62 (47) | 34–63 (42) |
CaeL % BdL | 60–73 (68) | 65–73 (69) | 66–76 (73) |
CaeL / CaeB | 11.2–20.9 (15.8) | 15.3–23.8 (18.8) | 19.7–30.9 (24.2) |
PCae | 83–165 (118) | 74–161 (109) | 79–147 (103) |
PCae % BdL | 7.1–12.7 (9.2) | 6.4–12.5 (9.2) | 6.2–9.2 (7.6) |
CsacL* | 261–412 (333) | 221–325 (287) | 279–467 (382) |
CsacB* | 51–95 (65) | 35–58 (48) | 41–82 (57) |
CsacL % BdL | 22–30 (26) | 19–28 (24) | 24–31 (28) |
CsacL / CsacB | 4.05–6.22 (5.32) | 4.85–6.72 (5.97) | 5.24–8.44 (6.84) |
ATL* | 84–125 (102) | 77–129 (101) | 66–127 (98) |
ATB* | 74–111 (89) | 70–95 (83) | 64–105 (81) |
ATL % BdL | 6.9–9.2 (8.3) | 7.4–9.6 (8.6) | 5.4–8.5 (7.3) |
AT-VS* | 355–560 (439) | 312–508 (391) | 412–771 (558) |
AT-VS % BdL | 33–37 (35) | 31–36 (33) | 36–44 (41) |
PTL* | 86–132 (105) | 87–152 (113) | 77–141 (102) |
PTB* | 77–110 (92) | 70–100 (83) | 67–101 (81) |
PTL % BdL | 6.9–9.4 (8.5) | 8.1–13.1 (9.7) | 5.9–8.9 (7.7) |
Ptes* | 140–263 (177) | 142–200 (166) | 158–315 (216) |
Ptes % BdL | 12–17 (14) | 12–16 (14) | 13–18 (16) |
OVL* | 58–88 (72) | 45–81 (60) | 50–80 (64) |
OVB* | 50–72 (59) | 38–58 (47) | 39–59 (48) |
OVL % BdL | 5.1–6.5 (5.8) | 4.0–6.3 (5.2) | 3.5–6.4 (4.7) |
OV-VS* | 253–424 (318) | 179–398 (285) | 303–591 (421) |
OV-VS % BdL | 23–30 (25) | 19–27 (24) | 27–33 (30) |
OV-AT* | 29–97 (56) | 34–64 (45) | 45–146 (91) |
OV-AT % BdL | 2.1–7.8 (4.7) | 3.1–4.4 (3.9) | 4.0–8.6 (6.5) |
OS-VS* | 176–262 (217) | 171–234 (202) | 93–201 (145) |
OS-VS % BdL | 15–21 (17) | 15–19 (17) | 8–14 (11) |
OS-OV* | 499–737 (582) | 415–692 (553) | 496–851 (627) |
OS-OV % BdL | 43–54 (47) | 45–51 (47) | 40–49 (45) |
OS-Vit* | 318–445 (378) | 291–424 (351) | 359–611 (455) |
OS-Vit % BdL | 27–35 (30) | 27–32 (29) | 29–38 (33) |
Previt | 501–624 (552) | 437–577 (504) | 506–784 (615) |
Previt % Bdl | 40–48 (45) | 39–48 (43) | 40–49 (45) |
Postvit | 26–55 (36) | 34–60 (44) | 21–49 (34) |
Postvit % BdL | 2.2–3.6 (2.9) | 3.0–5.5 (3.8) | 1.8–3.5 (2.6) |
VitOcc % BdL | 48–56 (53) | 47–57 (53) | 48–59 (52) |
EggL | 56–77 (64) | 56–68 (62) | 53–72 (63) |
EggB | 29–42 (34) | 26–39 (31) | 28–41 (34) |
Species . | Gorgocephalus euryaleae . | Gorgocephalus euryaleae . | Gorgocephalus graboides . |
---|---|---|---|
Locality | Point Peron and Rottnest Island, Western Australia | Sodwana Bay, KZN, South Africa | Lizard Island, QLD, Australia |
Host(s) | Kyphosus gladius; Kyphosus sydneyanus | Kyphosus cinerascens | Kyphosus cinerascens |
No. Measured | 15 (10 + 5) | 10 (8 + 2) | 15 (12 + 3) |
BdL* | 1071–1555 (1242) | 920–1463 (1168) | 1109–1768 (1326) |
BdB* | 129–210 (155) | 134–213 (180) | 100–201 (136) |
BdL / BdB | 7.17–9.62 (8.19) | 5.97–7.91 (6.83) | 9.02–12.59 (10.65) |
BdB % BdL | 10–14 (12) | 13–17 (15) | 8–11 (9) |
FBdL* | 352–463 (399) | 300–400 (359) | 271–387 (311) |
FBdL % BdL | 27–34 (32) | 27–33 (31) | 21–26 (24) |
OSL* | 141–184 (167) | 134–161 (147) | 140–194 (159) |
OSB ant* | 108–143 (128) | 91–106 (100) | 97–130 (111) |
OSB post* | 66–83 (75) | 49–65 (58) | 48–69 (58) |
OSL % BdL | 11–16 (13) | 11–15 (13) | 10–13 (12) |
OSL / OSB ant | 1.10–1.44 (1.31) | 1.40–1.61 (1.48) | 1.24–1.66 (1.44) |
OSL / OSB post | 1.98–2.46 (2.23) | 2.25–2.98 (2.56) | 2.25–3.12 (2.76) |
VSL* | 54–72 (61) | 53–68 (60) | 50–78 (61) |
VSB* | 52–70 (62) | 51–66 (59) | 49–83 (62) |
VSL % BdL | 4.3–5.5 (4.9) | 4.3–5.8 (5.1) | 4.1–4.9 (4.5) |
VSL / VSB | 0.88–1.1 (0.99) | 0.95–1.11 (1.02) | 0.88–1.14 (0.99) |
VSB / OSB ant | 0.42–0.53 (0.48) | 0.54–0.64 (0.59) | 0.46–0.68 (0.56) |
VSB / OSB post | 0.72–0.89 (0.82) | 0.96–1.24 (1.03) | 0.83–1.32 (1.07) |
VSL / OSL | 0.30–0.42 (0.37) | 0.37–0.44 (0.41) | 0.32–0.47 (0.38) |
PPhL | up to 74 (54) | up to 64 (39) | up to 72 (44) |
PhL* | 44–71 (56) | 48–64 (55) | 38–67 (50) |
PhB* | 58–83 (67) | 44–59 (51) | 49–78 (56) |
PhL % BdL | 3.8–5.8 (4.6) | 3.7–5.5 (4.6) | 3.0–4.3 (3.6) |
PhL / PhB | 0.63–1.22 (0.84) | 0.81–1.24 (1.09) | 0.73–1.17 (0.89) |
PhL / OSL | 0.26–0.46 (0.34) | 0.34–0.40 (0.37) | 0.27–0.35 (0.31) |
PhB / OSB ant | 0.41–0.61 (0.53) | 0.46–0.58 (0.51) | 0.42–0.67 (0.50) |
PhB / OSB post | 0.74–1.04 (0.90) | 0.78–1.06 (0.88) | 0.83–1.20 (0.96) |
OesL | 15–87 (61) | 37–66 (52) | 19–93 (51) |
CaeL | 652–1131 (854) | 595–1069 (809) | 749–1323 (1006) |
CaeB | 38–72 (52) | 37–62 (47) | 34–63 (42) |
CaeL % BdL | 60–73 (68) | 65–73 (69) | 66–76 (73) |
CaeL / CaeB | 11.2–20.9 (15.8) | 15.3–23.8 (18.8) | 19.7–30.9 (24.2) |
PCae | 83–165 (118) | 74–161 (109) | 79–147 (103) |
PCae % BdL | 7.1–12.7 (9.2) | 6.4–12.5 (9.2) | 6.2–9.2 (7.6) |
CsacL* | 261–412 (333) | 221–325 (287) | 279–467 (382) |
CsacB* | 51–95 (65) | 35–58 (48) | 41–82 (57) |
CsacL % BdL | 22–30 (26) | 19–28 (24) | 24–31 (28) |
CsacL / CsacB | 4.05–6.22 (5.32) | 4.85–6.72 (5.97) | 5.24–8.44 (6.84) |
ATL* | 84–125 (102) | 77–129 (101) | 66–127 (98) |
ATB* | 74–111 (89) | 70–95 (83) | 64–105 (81) |
ATL % BdL | 6.9–9.2 (8.3) | 7.4–9.6 (8.6) | 5.4–8.5 (7.3) |
AT-VS* | 355–560 (439) | 312–508 (391) | 412–771 (558) |
AT-VS % BdL | 33–37 (35) | 31–36 (33) | 36–44 (41) |
PTL* | 86–132 (105) | 87–152 (113) | 77–141 (102) |
PTB* | 77–110 (92) | 70–100 (83) | 67–101 (81) |
PTL % BdL | 6.9–9.4 (8.5) | 8.1–13.1 (9.7) | 5.9–8.9 (7.7) |
Ptes* | 140–263 (177) | 142–200 (166) | 158–315 (216) |
Ptes % BdL | 12–17 (14) | 12–16 (14) | 13–18 (16) |
OVL* | 58–88 (72) | 45–81 (60) | 50–80 (64) |
OVB* | 50–72 (59) | 38–58 (47) | 39–59 (48) |
OVL % BdL | 5.1–6.5 (5.8) | 4.0–6.3 (5.2) | 3.5–6.4 (4.7) |
OV-VS* | 253–424 (318) | 179–398 (285) | 303–591 (421) |
OV-VS % BdL | 23–30 (25) | 19–27 (24) | 27–33 (30) |
OV-AT* | 29–97 (56) | 34–64 (45) | 45–146 (91) |
OV-AT % BdL | 2.1–7.8 (4.7) | 3.1–4.4 (3.9) | 4.0–8.6 (6.5) |
OS-VS* | 176–262 (217) | 171–234 (202) | 93–201 (145) |
OS-VS % BdL | 15–21 (17) | 15–19 (17) | 8–14 (11) |
OS-OV* | 499–737 (582) | 415–692 (553) | 496–851 (627) |
OS-OV % BdL | 43–54 (47) | 45–51 (47) | 40–49 (45) |
OS-Vit* | 318–445 (378) | 291–424 (351) | 359–611 (455) |
OS-Vit % BdL | 27–35 (30) | 27–32 (29) | 29–38 (33) |
Previt | 501–624 (552) | 437–577 (504) | 506–784 (615) |
Previt % Bdl | 40–48 (45) | 39–48 (43) | 40–49 (45) |
Postvit | 26–55 (36) | 34–60 (44) | 21–49 (34) |
Postvit % BdL | 2.2–3.6 (2.9) | 3.0–5.5 (3.8) | 1.8–3.5 (2.6) |
VitOcc % BdL | 48–56 (53) | 47–57 (53) | 48–59 (52) |
EggL | 56–77 (64) | 56–68 (62) | 53–72 (63) |
EggB | 29–42 (34) | 26–39 (31) | 28–41 (34) |
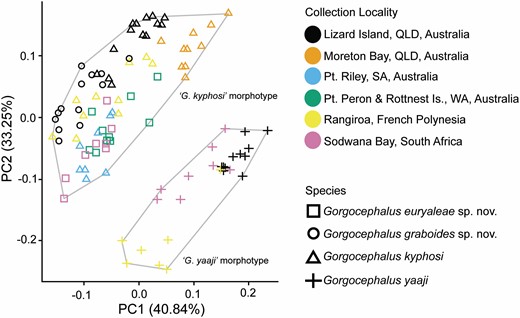
Principal component analysis (PCA) on morphometric data obtained from adult gorgocephalids from multiple localities in the Indo-West Pacific. Note the clustering pattern indicating only two morphotypes, consistent with the two previously described species from the region.
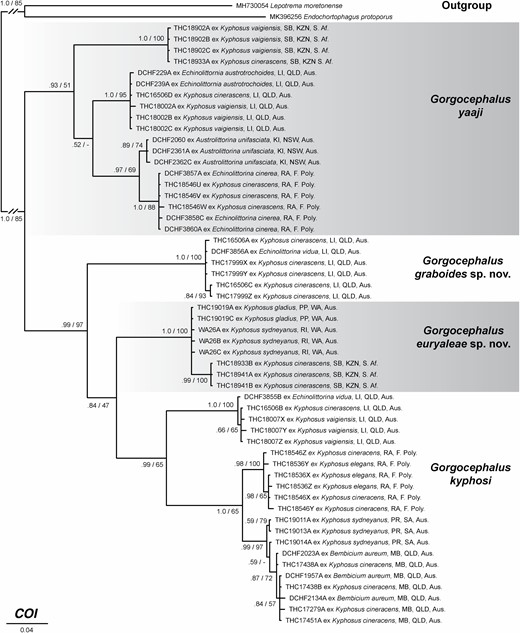
Bayesian majority-rule consensus tree of the COI mtDNA single-gene alignment. Bayesian inference (BI) posterior probabilities and maximum likelihood (ML) bootstrap support shown at nodes. A ‘-‘ symbol indicates the node was not recovered in ML analysis. The scale-bar indicates the number of substitutions per site.

Bayesian majority-rule consensus tree of the ITS2 single-gene alignment. Bayesian inference (BI) posterior probabilities and maximum likelihood (ML) bootstrap support shown at nodes. An asterisk indicates different topology recovered in ML analysis. The scale-bar indicates the number of substitutions per site.
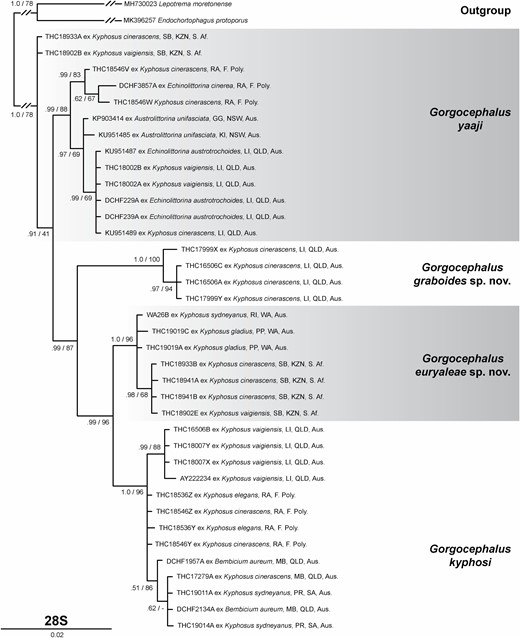
Bayesian majority-rule consensus tree of the 28S rDNA single-gene alignment. Bayesian inference (BI) posterior probabilities and maximum likelihood (ML) bootstrap support shown at nodes. A ‘-‘ symbol indicates the node was not recovered in ML analysis. The scale-bar indicates the number of substitutions per site.
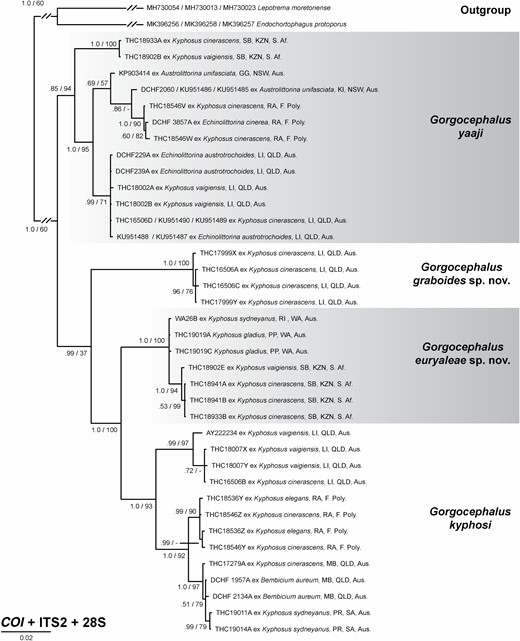
Bayesian majority-rule consensus tree of the concatenated COI + ITS2 + 28S alignment. Bayesian inference (BI) posterior probabilities and maximum likelihood (ML) bootstrap support shown at nodes. A ‘-‘ symbol indicates the node was not recovered in ML analysis. The scale-bar indicates the number of substitutions per site.
Gorgocephalus kyphosi
The type species for the family Gorgocephalidae, Gorgocephalus kyphosi, was described based on five specimens from Kyphosus sydneyanus collected from near Port Noarlunga, South Australia (Manter, 1966). The species has also been reported from K. vaigiensis from Lizard Island, GBR, Australia and from Moorea, Society Islands, French Polynesia (Bray, 2005b; Bray & Cribb, 2005). Molecular sequences have been provided previously for a specimen identified as G. kyphosi (Olson et al., 2003), although this specimen was collected from K. vaigiensis from off Lizard Island, GBR, rather than from the type host and locality. Port Noarlunga is now a protected marine reserve and the closest locality from which we were able to capture specimens of K. sydneyanus was Point Riley, Yorke Peninsula, South Australia. This locality is approximately 160 km from Port Noarlunga. However, we examined the holotype (borrowed from the Smithsonian National Museum of Natural History, USA; USNM 1356481) and found that the morphology of this specimen was consistent with those from Point Riley; thus, we consider our specimens from South Australia to be conspecific with G. kyphosi.
We obtained adults corresponding to the ‘Gorgocephalus kyphosi’ morphotype from all collection localities where gorgocephalids were found, except for Kioloa, NSW, Australia where only gastropods were collected. Based on the measurements and morphological description of G. kyphosi provided by Manter (1966), all of these adult specimens could be diagnosed as that species. However, molecular data indicated that up to six species could be distinguished under the umbrella of this morphological concept. Reassessment of morphology in light of molecular data, on a clade-by-clade basis, allowed separation of G. euryaleae and G. graboides from G. kyphosi, largely on the proportion of the cirrus-sac occupied by the internal seminal vesicle, pars prostatica and ejaculatory duct (see Taxonomy section). Both new species correspond to well-supported clades in all analyses (Figs 3–6). Recognition of these two species allows refinement of our morphological and molecular profile for G. kyphosi.
After exclusion of the two new species, sequences representative of the refined concept of Gorgocephalus kyphosi formed a large clade in all analyses (Figs 3–6), although there was relatively poor bootstrap support (65) for the clade in ML analysis of the COI dataset. This major clade was subdivided into several smaller clades, largely corresponding to collection locality. Sequences of the ITS2 and 28S rDNA gene regions for G. kyphosi were fairly conserved, differing by no more than 3 bp (< 1%) within or between localities (Table 5). Phylogenetic analyses of the ITS2 and 28S datasets demonstrated that sequences generated from specimens from two Australian localities (Moreton Bay and South Australia) were more similar to those from French Polynesia than to those from the third Australian locality (Lizard Island, GBR). Although sequences of the COI mtDNA gene region differed by no more than 6 bp (~1.3%) within each locality, large differences up to 62 bp (~13%) occurred between localities (Table 5). As with the ITS2 and 28S data, COI sequences generated from specimens from Moreton Bay and South Australia were more similar to each other and to those from French Polynesia (~1–6% difference) than to those from Lizard Island, GBR (~12–13% difference; Supporting Information, Table S5). These molecular differences, coupled with this geographic pattern, possibly indicate a species-level distinction. However, no significant morphological differences were observed between any specimens from the above named localities, and there are no host differences. Furthermore, in all phylogenetic analyses the Lizard Island clade was sister to the remaining putative sequences of G. kyphosi from other IWP localities. Thus, we conservatively consider all of these specimens as representative of G. kyphosi. Sequences of G. kyphosi differ from the other species of the Gorgocephalidae investigated here by 65–88 bp (14–15%), 4–18 bp (0.9–4.0%) and 5–20 bp (0.5–2.0%) in the COI, ITS2 and 28S gene regions, respectively (Table 5).
Summary of inter- and intraspecific pairwise comparisons of COI mtDNA, ITS2 and 28S rDNA gene-sequences for four species of Gorgocephalus
. | bp difference (% difference) . | ||
---|---|---|---|
. | COI . | ITS2 . | 28S . |
G. kyphosi within site | 0–6 (0.0–1.3) | 0 (0.0) | 0–1 (0.0–0.1) |
G. kyphosi between site | 3–62 (0.6–13) | 0–3 (0.0–0.7) | 0–3 (0.0–0.3) |
G. kyphosi—G. yaaji | 66–88 (14–19) | 5–10 (1.1–2.2) | 12–15 (1.2–1.5) |
G. kyphosi—G. euryaleae | 66–73 (14–15) | 4–7 (0.9–1.5) | 5–9 (0.5–0.9) |
G. kyphosi—G. graboides | 65–74 (14–16) | 16–18 (3.5–3.9) | 18–20 (1.8–2.0) |
G. yaaji within site | 0–3 (0.0–0.6) | 0 (0.0) | 0–1 (0.0–0.1) |
G. yaaji between site | 12–62 (2.5–13) | 0–5 (0.0–1.1) | 0–6 (0.0–0.6) |
G. yaaji—G. euryaleae | 69–87 (15–18) | 7–11 (1.5–2.4) | 13–16 (1.3–1.6) |
G. yaaji—G. graboides | 69–81 (15–17) | 14–16 (3.1–3.5) | 18–21 (1.8–2.1) |
G. euryaleae within site | 0–2 (0.0–0.4) | 0 (0.0) | 0–1 (0.0–0.1) |
G. euryaleae between site | 10–11 (2.1–2.3) | 1 (0.2) | 1–2 (0.1–0.2) |
G. euryaleae—G. graboides | 68–72 (14–15) | 18–19 (3.9–4.2) | 21–23 (2.1–2.3) |
G. graboides within site | 0–3 (0.0–0.6) | 0 (0.0) | 0–1 (0.0–0.1) |
. | bp difference (% difference) . | ||
---|---|---|---|
. | COI . | ITS2 . | 28S . |
G. kyphosi within site | 0–6 (0.0–1.3) | 0 (0.0) | 0–1 (0.0–0.1) |
G. kyphosi between site | 3–62 (0.6–13) | 0–3 (0.0–0.7) | 0–3 (0.0–0.3) |
G. kyphosi—G. yaaji | 66–88 (14–19) | 5–10 (1.1–2.2) | 12–15 (1.2–1.5) |
G. kyphosi—G. euryaleae | 66–73 (14–15) | 4–7 (0.9–1.5) | 5–9 (0.5–0.9) |
G. kyphosi—G. graboides | 65–74 (14–16) | 16–18 (3.5–3.9) | 18–20 (1.8–2.0) |
G. yaaji within site | 0–3 (0.0–0.6) | 0 (0.0) | 0–1 (0.0–0.1) |
G. yaaji between site | 12–62 (2.5–13) | 0–5 (0.0–1.1) | 0–6 (0.0–0.6) |
G. yaaji—G. euryaleae | 69–87 (15–18) | 7–11 (1.5–2.4) | 13–16 (1.3–1.6) |
G. yaaji—G. graboides | 69–81 (15–17) | 14–16 (3.1–3.5) | 18–21 (1.8–2.1) |
G. euryaleae within site | 0–2 (0.0–0.4) | 0 (0.0) | 0–1 (0.0–0.1) |
G. euryaleae between site | 10–11 (2.1–2.3) | 1 (0.2) | 1–2 (0.1–0.2) |
G. euryaleae—G. graboides | 68–72 (14–15) | 18–19 (3.9–4.2) | 21–23 (2.1–2.3) |
G. graboides within site | 0–3 (0.0–0.6) | 0 (0.0) | 0–1 (0.0–0.1) |
Summary of inter- and intraspecific pairwise comparisons of COI mtDNA, ITS2 and 28S rDNA gene-sequences for four species of Gorgocephalus
. | bp difference (% difference) . | ||
---|---|---|---|
. | COI . | ITS2 . | 28S . |
G. kyphosi within site | 0–6 (0.0–1.3) | 0 (0.0) | 0–1 (0.0–0.1) |
G. kyphosi between site | 3–62 (0.6–13) | 0–3 (0.0–0.7) | 0–3 (0.0–0.3) |
G. kyphosi—G. yaaji | 66–88 (14–19) | 5–10 (1.1–2.2) | 12–15 (1.2–1.5) |
G. kyphosi—G. euryaleae | 66–73 (14–15) | 4–7 (0.9–1.5) | 5–9 (0.5–0.9) |
G. kyphosi—G. graboides | 65–74 (14–16) | 16–18 (3.5–3.9) | 18–20 (1.8–2.0) |
G. yaaji within site | 0–3 (0.0–0.6) | 0 (0.0) | 0–1 (0.0–0.1) |
G. yaaji between site | 12–62 (2.5–13) | 0–5 (0.0–1.1) | 0–6 (0.0–0.6) |
G. yaaji—G. euryaleae | 69–87 (15–18) | 7–11 (1.5–2.4) | 13–16 (1.3–1.6) |
G. yaaji—G. graboides | 69–81 (15–17) | 14–16 (3.1–3.5) | 18–21 (1.8–2.1) |
G. euryaleae within site | 0–2 (0.0–0.4) | 0 (0.0) | 0–1 (0.0–0.1) |
G. euryaleae between site | 10–11 (2.1–2.3) | 1 (0.2) | 1–2 (0.1–0.2) |
G. euryaleae—G. graboides | 68–72 (14–15) | 18–19 (3.9–4.2) | 21–23 (2.1–2.3) |
G. graboides within site | 0–3 (0.0–0.6) | 0 (0.0) | 0–1 (0.0–0.1) |
. | bp difference (% difference) . | ||
---|---|---|---|
. | COI . | ITS2 . | 28S . |
G. kyphosi within site | 0–6 (0.0–1.3) | 0 (0.0) | 0–1 (0.0–0.1) |
G. kyphosi between site | 3–62 (0.6–13) | 0–3 (0.0–0.7) | 0–3 (0.0–0.3) |
G. kyphosi—G. yaaji | 66–88 (14–19) | 5–10 (1.1–2.2) | 12–15 (1.2–1.5) |
G. kyphosi—G. euryaleae | 66–73 (14–15) | 4–7 (0.9–1.5) | 5–9 (0.5–0.9) |
G. kyphosi—G. graboides | 65–74 (14–16) | 16–18 (3.5–3.9) | 18–20 (1.8–2.0) |
G. yaaji within site | 0–3 (0.0–0.6) | 0 (0.0) | 0–1 (0.0–0.1) |
G. yaaji between site | 12–62 (2.5–13) | 0–5 (0.0–1.1) | 0–6 (0.0–0.6) |
G. yaaji—G. euryaleae | 69–87 (15–18) | 7–11 (1.5–2.4) | 13–16 (1.3–1.6) |
G. yaaji—G. graboides | 69–81 (15–17) | 14–16 (3.1–3.5) | 18–21 (1.8–2.1) |
G. euryaleae within site | 0–2 (0.0–0.4) | 0 (0.0) | 0–1 (0.0–0.1) |
G. euryaleae between site | 10–11 (2.1–2.3) | 1 (0.2) | 1–2 (0.1–0.2) |
G. euryaleae—G. graboides | 68–72 (14–15) | 18–19 (3.9–4.2) | 21–23 (2.1–2.3) |
G. graboides within site | 0–3 (0.0–0.6) | 0 (0.0) | 0–1 (0.0–0.1) |
Two intermediate hosts were identified for this species using COI, ITS2 and 28S sequences (Figs 3–6). Six Bembicium auratum, collected from near Dunwich, North Stradbroke Island, Moreton Bay, Australia, were found infected with gorgocephalids. Three infections were matched to adult worms obtained from Kyphosus cinerascens collected nearby, from off Amity Point, North Stradbroke Island. In addition, a single infected Echinolittorina vidua, collected from Lizard Island, GBR, was matched to adults obtained from K. vaigiensis collected from off the same locality using ITS2 gene sequences.
Gorgocephalus euryaleae and gorgocephalus graboides
These two new species are morphologically differentiated from Gorgocephalus kyphosi, and each other, largely on features within the cirrus-sac (see Taxonomy section). Sequences for these species form well-supported clades in all analyses (Figs 3–6), with presumed conspecifics collected from the same locality differing by no more than 3 bp in any of the three sequenced gene regions (Table 5). Sequences of G. euryaleae differ by 10–11 bp (~2%), 1 bp (~0.2%) and 1–2 bp (0.1–0.2%) in the COI, ITS2 and 28S gene regions, respectively, between Western Australia and South Africa. Sequences of G. euryaleae differ from the others species of the Gorgocephalidae recognized here by 66–87 bp (14–18%), 4–19 bp (0.9–4.2%) and 5–23 bp (0.5–2.3%) in the COI, ITS2 and 28S gene regions, respectively. Sequences of G. graboides, found at only one locality, differ from other gorgocephalid species by 65–81 bp (14–17%), 14–19 bp (3.1–4.2%) and 18–23 bp (1.8–2.3%) in the COI, ITS2 and 28S gene regions, respectively (Table 5).
Gorgocephalus graboides was recovered only from Kyphosus cinerascens collected from off Lizard Island, GBR, whereas G. euryaleae was recovered from K. cinerascens in South Africa and from K. gladius and K. sydneyanus from Western Australia. Although the two new species are apparently geographically isolated from one another, they share at least one definitive host. Both species also have an overlapping definitive host range with G. kyphosi (K. sydneyanus and K. cinerascens for G. euryaleae and K. cinerascens for G. graboides). Furthermore, intramolluscan gorgocephalids from a single infected Echinolittorina vidua from Lizard Island were successfully matched to G. graboides. Thus, G. graboides also shares an intermediate host with G. kyphosi.
Gorgocephalus yaaji
This species was described by Bray & Cribb (2005) based on adult specimens obtained from Kyphosus vaigiensis, collected from off Lizard Island, GBR, Australia. Gorgocephalus yaaji is easily distinguished from all other species of the family, largely in having a more robust, dorsoventrally flattened body shape and in having the vitellarium extend into the forebody. Previously, Huston et al. (2016) generated ITS2 and 28S rDNA gene sequences from adult and intramolluscan specimens of G. yaaji from the type locality. In the present study, additional adult gorgocephalids morphologically consistent with G. yaaji were obtained from the type locality and from Rangiroa Atoll in French Polynesia and Sodwana Bay in South Africa (Fig. 1). Despite extensive investigation, including traditional morphometrics, PCA analyses and SEM imaging, we were unable to find any significant morphological differences between specimens from these three localities. Intramolluscan gorgocephalids from three Echinolittorina cinerea collected from Rangiroa Atoll, French Polynesia were molecularly matched to adults consistent with G. yaaji collected from the same locality. Furthermore, intramolluscan gorgocephalids have previously been collected from Austrolittorina unifasciata, from multiple locations along the coast of New South Wales, Australia (O’Dwyer et al., 2015; Huston et al., 2016), but they had not previously been connected to an adult form. BLAST analyses showed that the sequences generated from intramolluscan gorgocephalids collected from Kioloa, NSW, matched sequences of ‘Gorgocephalus sp. Aus’ from the study of O’Dwyer et al. (2015) with identity scores of 98–100%, demonstrating that gorgocephalids collected from A. unifasciata in the studies of O’Dwyer et al. (2015) and Huston et al. (2016) are conspecific. The present phylogenetic analyses (Figs 3–6) demonstrate that these infections are representative of G. yaaji.
Despite adult gorgocephalids consistent with Gorgocephalus yaaji collected from across the IWP being morphologically indistinguishable, phylogenetic analyses of the ITS2 and 28S rDNA single-gene datasets did not recover a monophyletic G. yaaji clade (Figs 4, 5). In the ITS2 single-gene tree (Fig. 4), specimens morphologically consistent with G. yaaji were paraphyletic and formed three well-supported clades: specimens from Lizard Island (adults and intramolluscan stages), specimens from French Polynesia (adults and intramolluscan stages) + those from Kioloa, NSW, Australia (intramolluscan stages only), and those from South Africa (adults only). The first two of these clades were recovered in polytomy with the third + G. euryaleae + G. kyphosi, with G. graboides sister to all of these clades together. All ITS2 sequences for G. yaaji were identical for specimens within each locality and differed by 0–5 bp (0.0–1.1%) between localities (Table 5). In the 28S rDNA single-gene tree (Fig. 5), G. yaaji was also recovered as paraphyletic, but with the South African representatives resolving as basal to all clades. The 28S rDNA sequences of G. yaaji differed by 0–6 bp (0.0–0.6%) between localities.
In contrast to analyses of ITS2 and 28S rDNA, those for the COI mtDNA single-gene dataset (Fig. 3) resolved all specimens morphologically consistent with Gorgocephalus yaaji as a monophyletic group, sister to the remaining lineages of the Gorgocephalidae, albeit without support in ML analysis. Sequences differed by only 0–3 bp (0.0–0.6%) within individual localities but differed by 12–62 bp (~3.0–13%) between localities. The largest difference (62 bp) was between specimens from French Polynesia and South Africa, representing opposite sides of the IWP marine region, although the South African specimens were also relatively divergent from those collected in Australia (50–58 bp or ~11–12%; Supporting Information, Table S5). The concatenated COI + ITS2 + 28S analyses (Fig. 6) also resolved representatives of G. yaaji in a monophyletic clade, with lower BI posterior probability support but higher ML bootstrap support.
Similar to the pattern observed for Gorgocephalus kyphosi, COI and ITS2 sequences of G. yaaji, generated from one Australian locality (Kioloa, NSW) were more similar to those from French Polynesia than to those from another Australian locality, Lizard Island, GBR (Supporting Information, Table S5). Such molecular variation coupled with the lack of monophyly in the ITS2 and 28S rDNA trees suggests the possibility of multiple species. However, in the ITS2 and 28S trees, sequences associated with specimens morphologically indistinguishable from G. yaaji, result in a paraphyletic, rather than a polyphyletic, G. yaaji (i.e. sequences are more, or at least, as related to one another as they are to other species represented). There are also no host-level distinctions to delineate additional species within the G. yaaji concept; the species appears to have a broad definitive and intermediate host range (within the confines of the Kyphosidae and Littorinidae). The issues with paraphyly in the ITS2 and 28S trees might be alleviated with sequences from additional specimens collected from localities between Australia and South Africa. However, without further evidence there appears no justification for splitting the G. yaaji clade into multiple morphologically cryptic species. Sequences of G. yaaji differ from the others species of the Gorgocephalidae recognized here by 66–88 bp (14–19%), 5–16 bp (1.1–3.5%) and 12–21 bp (1.2–2.1%) in the COI, ITS2 and 28S gene regions, respectively.
Taxonomy
Phylum Platyhelminthes Minot, 1876
Class Trematoda Rudolphi, 1808
Subclass Digenea Carus, 1863
Order Plagiorchiida la Rue, 1957
Superfamily Lepocreadioidea Odhner, 1905
Family Gorgocephalidae Manter, 1966,
Genus GorgocephalusManter, 1966,
Gorgocephalus kyphosi Manter, 1966
(Figs 7–9; Tables 2, 6)
Type host and locality
Kyphosus sydneyanus (Günther, 1886) (Perciformes: Kyphosidae), silver drummer, from near Port Noarlunga, South Australia (35°09’10’’S, 138°27’49’’E).
Records
1, Manter (1966); 2, Olson et al. (2003); 3, Bray (2005b). 4, Bray & Cribb (2005); 5, present study.
Definitive hosts
Perciformes, Kyphosidae. Kyphosus cinerascens (Forsskål, 1775), highfin chub (5); Kyphosus elegans (W. K. H. Peters, 1869), Cortez sea chub (5); Kyphosus sydneyanus (Günther, 1886), silver drummer (1, 5). Kyphosus vaigiensis (Quoy & Gaimard, 1825), brassy chub (2, 3, 4, 5). The number in brackets refer to the ‘records’ section directly preceding this - essentially a way to avoid having to list citations repeatedly.
Intermediate hosts
Gastropoda, Littorinimorpha, Littorinidae. Bembicium auratum (Quoy & Gaimard, 1834) (5); Echinolittorina vidua (Gould, 1859) (5).
Other localities
Off Point Riley, Yorke Peninsula, South Australia (33°52’49’’S, 137°35’52’’E) (PR) (5); off Amity Point, North Stradbroke Island, Moreton Bay, Queensland, Australia (27°23’53’’S, 153°26’15’’E) (AP) (5); off Dunwich, North Stradbroke Island, Moreton Bay, Queensland, Australia (27°29’46’’S, 153°23’52’’E) (DW) (5); off Lizard Island, Great Barrier Reef, Queensland, Australia (14°41’10’’S, 145°28’15’’E) (LI) (2, 3, 4, 5); off Moorea, Society Islands, French Polynesia (17°32’46’’S, 149°49’47’’E) (4); off Rangiroa, Tuamotu Islands, French Polynesia (15°10’40’’S, 147°39’04’’W) (RA) (5). The letters in brackets are an abbreviation for the locality.
Voucher material (adult)
Ten whole-mount and three hologenophore specimens, ex K. sydneyanus from PR (SAM AHC36801–36806); ten whole-mount and three hologenophore specimens ex K. cinerascens from AP (QM G238552–G238564); two whole-mount specimens ex K. cinerascens from LI (QM G238571–G238572); 13 whole-mount and three hologenophore specimens ex K. vaigiensis from LI (QM G238573–G238588); nine whole-mount and three hologenophore specimens ex K. cinerascens from RA (MNHN HEL1431–1442); one whole-mount and three hologenophore specimens ex K. elegans from RA (MNHN HEL1433–1446).
Voucher material (intramolluscan)
Six slides of rediae and cercariae ex B. auratum from DW (QM G238565–G238570); three slides of rediae and cercariae ex E. vidua from LI (QM G238589–G238591).
Site in host
Pyloric caeca (definitive); gonad/digestive gland (intermediate).
Representative DNA sequences
Twenty-one sequences deposited for COI mtDNA (MW353629–MW353649); 21 sequences deposited for 5.8S-ITS2-partial 28S rDNA (MW353910–MW353930); 12 sequences deposited for partial 28S rDNA (MW353877–MW353888); see Supporting Information, Table S2.
Description of adult (Figs 7A, B, E, 8, 9A–C)
Measurements in Table 2. Description based on all adult voucher material plus SEM images of eight adult specimens. Body elongate, cylindrical, broadest in region anterior to ventral sucker, tapering slightly posteriorly. Tegument armed with alternating rows of partially overlapping comb-like scales; distal portion of scales forming up to 15 distinct tendrils. Eyespot pigment sparsely scattered in forebody. Oral sucker terminal, partially retractable, infundibuliform, broadest in anterior region with distinct reduction in diameter about mid-length continuing through to posterior margin; margin of anterior portion bearing crown of 14 bifid tentacles; outer branch of tentacles broad, conoid; inner branch of tentacles longer than outer branch, tendril-like, tapering distally. Ventral sucker in anterior third of body, round, far smaller than oral sucker. Prepharynx short, distinct, sigmoid or looped. Pharynx ellipsoidal to dolioform, in line with oral sucker or rotated up to 90°. Oesophagus short, bifurcates just posterior to pharynx with proximal section reaching to ventral surface and opening as ‘ventral anus’, and distal portion expanding to form caecum. Caecum single, broadest in anterior region, passes from mid-forebody to close to posterior extremity, terminates blindly; gastrodermis well developed.
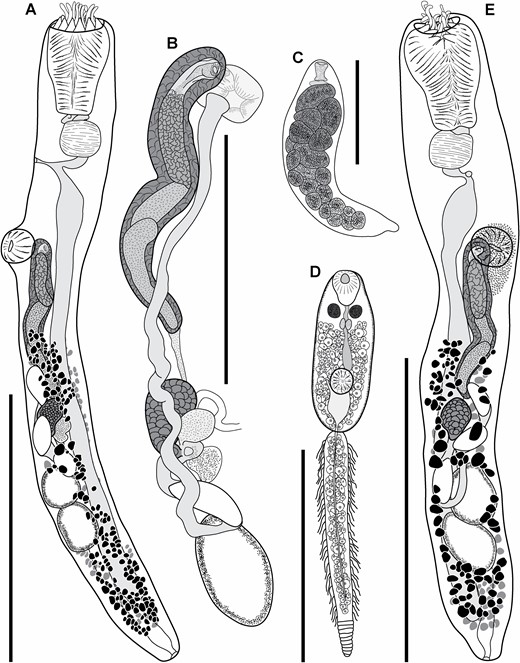
Gorgocephalus kyphosi. A, adult voucher ex Kyphosus sydneyanus, Point Riley, Yorke Peninsula, South Australia; lateral perspective. B, genital atrium, cirrus-sac and ovarian complex of A; lateral perspective. C, redia ex Echinolittorina vidua, Lizard Island, Queensland, Australia; ventral perspective. D, emerged cercaria ex Echinolittorina vidua, Lizard Island; ventral perspective. E, adult voucher ex Kyphosus sydneyanus, Point Riley; ventral perspective. Scale bars: A, E, 500 μm; B, C, D, 250 μm.
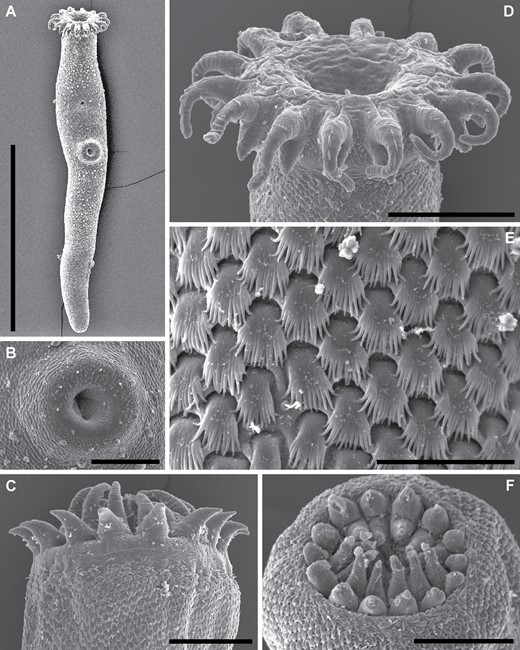
Gorgocephalus kyphosi, scanning electron micrographs. A, whole adult worm ex Kyphosus sydneyanus, Point Riley, Yorke Peninsula, South Australia. B, D, E, ventral sucker, oral sucker and tegument of A, respectively. C, oral sucker of adult worm ex Kyphosus cinerascens, Moreton Bay, Queensland, Australia. F, oral sucker of adult worm ex Kyphosus cinerascens, Rangiroa, Tuamotu Islands, French Polynesia. Scale bars: A, 400 μm; B, 30 μm; C, D, 50 μm; E, 10 μm; F, 40 μm.
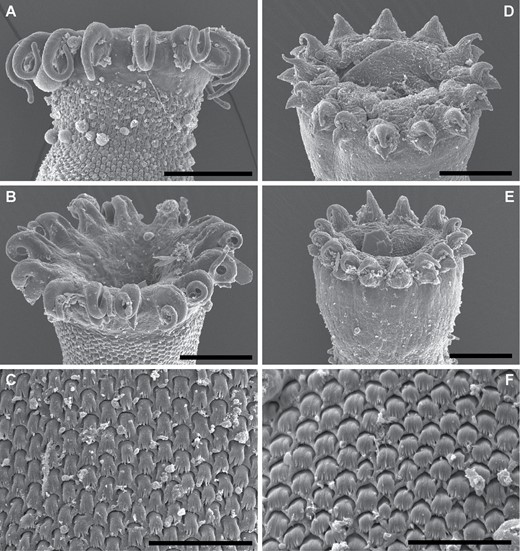
Gorgocephalus kyphosi and Gorgocephalus yaaji, ex Kyphosus vaigiensis, Lizard Island, Queensland, Australia, scanning electron micrographs. A, B, oral suckers of adult Gorgocephalus kyphosi. C, tegument of adult Gorgocephalus kyphosi. D, E, oral suckers of adult Gorgocephalus yaaji. F, tegument of adult Gorgocephalus yaaji. Scale bars: A, B, D, E, 50 μm; C, F, 20 μm.
Testes two, ellipsoidal, tandem, contiguous, in mid-hindbody. Vasa deferentia narrow, passing relatively direct from testes to cirrus-sac. Cirrus-sac elongate, cylindrical, winding, reaching from just anterior to ovary to level of ventral sucker. Internal seminal vesicle tubular, loops once about mid-length, occupies about half length of cirrus-sac. Pars prostatica distinct, vesicular, about half length of internal seminal vesicle, lined with anuclear, cell-like bodies. Ejaculatory duct short, curves back dorsally from pars prostatica to open into genital atrium. Genital atrium broad, dorsal to and larger than ventral sucker. Genital pore large, round to irregular, opening dorsally at level of ventral sucker.
Ovary pre-testicular, pyriform, narrowing posteriorly toward union with oötype. Mehlis’ gland indistinct. Laurer’s canal opens dorsally at level of ovary. Canalicular seminal vesicle saccular, contiguous with and dorsal to ovary. Uterus narrow, passes posteriorly from oötype to anterior testis, loops back, gently winding anteriorly, forming muscular metraterm about mid-level of pars prostatica, opening into genital atrium adjacent to ejaculatory duct. Eggs few, oval, operculate, large; length often exceeding that of ovary. Vitellarium follicular, restricted to hindbody; fields reaching from about mid-cirrus-sac to near posterior extremity; dorsal, lateral and ventral fields confluent, wrap around body from dorsal midline to ventrosinistral and ventrodextral regions anterior to testes, wrap entire body posterior to testes. Vitelline reservoir between ovary and anterior testis; collecting ducts indistinct. Excretory pore terminal; excretory vesicle Y-shaped, passes anteriorly, bifurcating in testicular region, ducts passing anteriorly sinistrally and dextrally, terminating as enlarged pyriform sacs on either side of cirrus-sac.
Description of redia (Fig. 7C)
Measurements in Table 6. Description based on all voucher material. Body elongate, broadest anteriorly, tapering slightly posteriorly. Cercarial embryos numerous, poorly developed. Mouth subterminal. Pharynx cylindrical to hourglass-shaped. Intestine short, saclike, immediately posterior to pharynx.
Morphometric data for Gorgocephalus spp. cercariae and rediae expressed as a range and mean in micrometres or as percentages. Number of specimens measured presented as cercariae/rediae. Abbreviations: Bd, body; L, length; B, breadth; FBd, forebody; Esp, eyespot; OS, oral sucker; VS, ventral sucker; PPh, prepharynx; Ph, pharynx; Ta, tail; Int, Intestine. Egg measurements represent the mean obtained from multiple subsamples per specimen. Measurements of both eyespots combined for each cercaria
Species . | Gorgocephalus kyphosi . | Gorgocephalus kyphosi . | Gorgocephalus yaaji . | Gorgocephalus graboides . |
---|---|---|---|---|
Locality | Moreton Bay, QLD, Australia | Lizard Island, QLD, Australia | Rangiroa, French Polynesia | Lizard Island, QLD, Australia |
Host | Bembicium auratum | Echinolittorina vidua | Echinolittorina cinerea | Echinolittorina vidua |
No. Measured | 10 / 10 | 10 /10 | 10 /10 | 10 / 10 |
Cercariae | ||||
BdL | 197–234 (215) | 167–199 (189) | 202–247 (223) | 178–197 (190) |
BdB | 65–79 (71) | 61–73 (66) | 61–87 (78) | 64–84 (73) |
Body L / W | 2.86–3.21 (3.02) | 2.61–3.21 (2.87) | 2.59–3.46 (2.89) | 2.19–2.91 (2.61) |
FBdL | 115–148 (131) | 95–166 (116) | 117–147 (132) | 102–113 (108) |
FBdL % BL | 0.57–0.64 (0.61) | 0.54–0.86 (0.61) | 0.55–0.62 (0.59) | 0.54–0.62 (0.57) |
EspL | 14–22 (17) | 12–16 (14) | 11–14 (12) | 12–15 (13) |
EspB | 13–22 (17) | 10–17 (13) | 10–14 (12) | 10–14 (12) |
OSL | 40–49 (44) | 32–43 (39) | 34–43 (38) | 36–44 (40) |
OSB | 33–39 (37) | 31–36 (33) | 31–43 (36) | 25–36 (31) |
VSL | 32–41 (36) | 28–38 (33) | 32–38 (35) | 26–40 (34) |
VSB | 32–38 (36) | 28–35 (31) | 30–39 (34) | 23–47 (36) |
OSL / VSL | 1.05–1.38 (1.23) | 0.94–1.35 (1.19) | 1.00–1.31 (1.09) | 0.93–1.52 (1.19) |
OSB / VSB | 0.89–1.18 (1.05) | 0.91–1.29 (1.06) | 0.84–1.23 (1.06) | 0.65–1.09 (0.89) |
PPhL | 12–34 (21) | 12–20 (16) | 17–32 (23) | 9–20 (15) |
PhL | 16–38 (21) | 12–20 (16) | 18–20 (19) | 14–18 (16) |
PhB | 18–38 (22) | 14–18 (17) | 17–22 (20) | 15–20 (17) |
TaL | 326–389 (366) | 159–276 (233) | 134–216 (175) | 157–260 (214) |
TaB | 31–40 (34) | 25–35 (29) | 25–31 (27) | 20–27 (25) |
Rediae | ||||
BdL | 437–958 (726) | 252–627 (465) | 235–387 (292) | 247–702 (478) |
BdB | 104–143 (121) | 63–133 (111) | 82–163 (125) | 55–124 (101) |
PhL | 46–72 (61) | 41–90 (55) | 23–40 (33) | 32–59 (37) |
PhB | 32–48 (41) | 29–88 (42) | 33–41 (36) | 29–67 (38) |
PhL / PhB | 1.25–1.79 (1.49) | 0.97–1.51 (1.34) | 0.70–1.06 (0.91) | 0.88–1.10 (0.97) |
IntL | 25–58 (42) | 18–58 (37) | 26–40 (32) | 22–53 (29) |
IntB | 31–54 (42) | 27–80 (48) | 24–49 (33) | 22–39 (29) |
IntL % BL | 0.04–0.10 (0.06) | 0.03–0.12 (0.08) | 0.08–0.15 (0.11) | 0.05–0.12 (0.07) |
Species . | Gorgocephalus kyphosi . | Gorgocephalus kyphosi . | Gorgocephalus yaaji . | Gorgocephalus graboides . |
---|---|---|---|---|
Locality | Moreton Bay, QLD, Australia | Lizard Island, QLD, Australia | Rangiroa, French Polynesia | Lizard Island, QLD, Australia |
Host | Bembicium auratum | Echinolittorina vidua | Echinolittorina cinerea | Echinolittorina vidua |
No. Measured | 10 / 10 | 10 /10 | 10 /10 | 10 / 10 |
Cercariae | ||||
BdL | 197–234 (215) | 167–199 (189) | 202–247 (223) | 178–197 (190) |
BdB | 65–79 (71) | 61–73 (66) | 61–87 (78) | 64–84 (73) |
Body L / W | 2.86–3.21 (3.02) | 2.61–3.21 (2.87) | 2.59–3.46 (2.89) | 2.19–2.91 (2.61) |
FBdL | 115–148 (131) | 95–166 (116) | 117–147 (132) | 102–113 (108) |
FBdL % BL | 0.57–0.64 (0.61) | 0.54–0.86 (0.61) | 0.55–0.62 (0.59) | 0.54–0.62 (0.57) |
EspL | 14–22 (17) | 12–16 (14) | 11–14 (12) | 12–15 (13) |
EspB | 13–22 (17) | 10–17 (13) | 10–14 (12) | 10–14 (12) |
OSL | 40–49 (44) | 32–43 (39) | 34–43 (38) | 36–44 (40) |
OSB | 33–39 (37) | 31–36 (33) | 31–43 (36) | 25–36 (31) |
VSL | 32–41 (36) | 28–38 (33) | 32–38 (35) | 26–40 (34) |
VSB | 32–38 (36) | 28–35 (31) | 30–39 (34) | 23–47 (36) |
OSL / VSL | 1.05–1.38 (1.23) | 0.94–1.35 (1.19) | 1.00–1.31 (1.09) | 0.93–1.52 (1.19) |
OSB / VSB | 0.89–1.18 (1.05) | 0.91–1.29 (1.06) | 0.84–1.23 (1.06) | 0.65–1.09 (0.89) |
PPhL | 12–34 (21) | 12–20 (16) | 17–32 (23) | 9–20 (15) |
PhL | 16–38 (21) | 12–20 (16) | 18–20 (19) | 14–18 (16) |
PhB | 18–38 (22) | 14–18 (17) | 17–22 (20) | 15–20 (17) |
TaL | 326–389 (366) | 159–276 (233) | 134–216 (175) | 157–260 (214) |
TaB | 31–40 (34) | 25–35 (29) | 25–31 (27) | 20–27 (25) |
Rediae | ||||
BdL | 437–958 (726) | 252–627 (465) | 235–387 (292) | 247–702 (478) |
BdB | 104–143 (121) | 63–133 (111) | 82–163 (125) | 55–124 (101) |
PhL | 46–72 (61) | 41–90 (55) | 23–40 (33) | 32–59 (37) |
PhB | 32–48 (41) | 29–88 (42) | 33–41 (36) | 29–67 (38) |
PhL / PhB | 1.25–1.79 (1.49) | 0.97–1.51 (1.34) | 0.70–1.06 (0.91) | 0.88–1.10 (0.97) |
IntL | 25–58 (42) | 18–58 (37) | 26–40 (32) | 22–53 (29) |
IntB | 31–54 (42) | 27–80 (48) | 24–49 (33) | 22–39 (29) |
IntL % BL | 0.04–0.10 (0.06) | 0.03–0.12 (0.08) | 0.08–0.15 (0.11) | 0.05–0.12 (0.07) |
Morphometric data for Gorgocephalus spp. cercariae and rediae expressed as a range and mean in micrometres or as percentages. Number of specimens measured presented as cercariae/rediae. Abbreviations: Bd, body; L, length; B, breadth; FBd, forebody; Esp, eyespot; OS, oral sucker; VS, ventral sucker; PPh, prepharynx; Ph, pharynx; Ta, tail; Int, Intestine. Egg measurements represent the mean obtained from multiple subsamples per specimen. Measurements of both eyespots combined for each cercaria
Species . | Gorgocephalus kyphosi . | Gorgocephalus kyphosi . | Gorgocephalus yaaji . | Gorgocephalus graboides . |
---|---|---|---|---|
Locality | Moreton Bay, QLD, Australia | Lizard Island, QLD, Australia | Rangiroa, French Polynesia | Lizard Island, QLD, Australia |
Host | Bembicium auratum | Echinolittorina vidua | Echinolittorina cinerea | Echinolittorina vidua |
No. Measured | 10 / 10 | 10 /10 | 10 /10 | 10 / 10 |
Cercariae | ||||
BdL | 197–234 (215) | 167–199 (189) | 202–247 (223) | 178–197 (190) |
BdB | 65–79 (71) | 61–73 (66) | 61–87 (78) | 64–84 (73) |
Body L / W | 2.86–3.21 (3.02) | 2.61–3.21 (2.87) | 2.59–3.46 (2.89) | 2.19–2.91 (2.61) |
FBdL | 115–148 (131) | 95–166 (116) | 117–147 (132) | 102–113 (108) |
FBdL % BL | 0.57–0.64 (0.61) | 0.54–0.86 (0.61) | 0.55–0.62 (0.59) | 0.54–0.62 (0.57) |
EspL | 14–22 (17) | 12–16 (14) | 11–14 (12) | 12–15 (13) |
EspB | 13–22 (17) | 10–17 (13) | 10–14 (12) | 10–14 (12) |
OSL | 40–49 (44) | 32–43 (39) | 34–43 (38) | 36–44 (40) |
OSB | 33–39 (37) | 31–36 (33) | 31–43 (36) | 25–36 (31) |
VSL | 32–41 (36) | 28–38 (33) | 32–38 (35) | 26–40 (34) |
VSB | 32–38 (36) | 28–35 (31) | 30–39 (34) | 23–47 (36) |
OSL / VSL | 1.05–1.38 (1.23) | 0.94–1.35 (1.19) | 1.00–1.31 (1.09) | 0.93–1.52 (1.19) |
OSB / VSB | 0.89–1.18 (1.05) | 0.91–1.29 (1.06) | 0.84–1.23 (1.06) | 0.65–1.09 (0.89) |
PPhL | 12–34 (21) | 12–20 (16) | 17–32 (23) | 9–20 (15) |
PhL | 16–38 (21) | 12–20 (16) | 18–20 (19) | 14–18 (16) |
PhB | 18–38 (22) | 14–18 (17) | 17–22 (20) | 15–20 (17) |
TaL | 326–389 (366) | 159–276 (233) | 134–216 (175) | 157–260 (214) |
TaB | 31–40 (34) | 25–35 (29) | 25–31 (27) | 20–27 (25) |
Rediae | ||||
BdL | 437–958 (726) | 252–627 (465) | 235–387 (292) | 247–702 (478) |
BdB | 104–143 (121) | 63–133 (111) | 82–163 (125) | 55–124 (101) |
PhL | 46–72 (61) | 41–90 (55) | 23–40 (33) | 32–59 (37) |
PhB | 32–48 (41) | 29–88 (42) | 33–41 (36) | 29–67 (38) |
PhL / PhB | 1.25–1.79 (1.49) | 0.97–1.51 (1.34) | 0.70–1.06 (0.91) | 0.88–1.10 (0.97) |
IntL | 25–58 (42) | 18–58 (37) | 26–40 (32) | 22–53 (29) |
IntB | 31–54 (42) | 27–80 (48) | 24–49 (33) | 22–39 (29) |
IntL % BL | 0.04–0.10 (0.06) | 0.03–0.12 (0.08) | 0.08–0.15 (0.11) | 0.05–0.12 (0.07) |
Species . | Gorgocephalus kyphosi . | Gorgocephalus kyphosi . | Gorgocephalus yaaji . | Gorgocephalus graboides . |
---|---|---|---|---|
Locality | Moreton Bay, QLD, Australia | Lizard Island, QLD, Australia | Rangiroa, French Polynesia | Lizard Island, QLD, Australia |
Host | Bembicium auratum | Echinolittorina vidua | Echinolittorina cinerea | Echinolittorina vidua |
No. Measured | 10 / 10 | 10 /10 | 10 /10 | 10 / 10 |
Cercariae | ||||
BdL | 197–234 (215) | 167–199 (189) | 202–247 (223) | 178–197 (190) |
BdB | 65–79 (71) | 61–73 (66) | 61–87 (78) | 64–84 (73) |
Body L / W | 2.86–3.21 (3.02) | 2.61–3.21 (2.87) | 2.59–3.46 (2.89) | 2.19–2.91 (2.61) |
FBdL | 115–148 (131) | 95–166 (116) | 117–147 (132) | 102–113 (108) |
FBdL % BL | 0.57–0.64 (0.61) | 0.54–0.86 (0.61) | 0.55–0.62 (0.59) | 0.54–0.62 (0.57) |
EspL | 14–22 (17) | 12–16 (14) | 11–14 (12) | 12–15 (13) |
EspB | 13–22 (17) | 10–17 (13) | 10–14 (12) | 10–14 (12) |
OSL | 40–49 (44) | 32–43 (39) | 34–43 (38) | 36–44 (40) |
OSB | 33–39 (37) | 31–36 (33) | 31–43 (36) | 25–36 (31) |
VSL | 32–41 (36) | 28–38 (33) | 32–38 (35) | 26–40 (34) |
VSB | 32–38 (36) | 28–35 (31) | 30–39 (34) | 23–47 (36) |
OSL / VSL | 1.05–1.38 (1.23) | 0.94–1.35 (1.19) | 1.00–1.31 (1.09) | 0.93–1.52 (1.19) |
OSB / VSB | 0.89–1.18 (1.05) | 0.91–1.29 (1.06) | 0.84–1.23 (1.06) | 0.65–1.09 (0.89) |
PPhL | 12–34 (21) | 12–20 (16) | 17–32 (23) | 9–20 (15) |
PhL | 16–38 (21) | 12–20 (16) | 18–20 (19) | 14–18 (16) |
PhB | 18–38 (22) | 14–18 (17) | 17–22 (20) | 15–20 (17) |
TaL | 326–389 (366) | 159–276 (233) | 134–216 (175) | 157–260 (214) |
TaB | 31–40 (34) | 25–35 (29) | 25–31 (27) | 20–27 (25) |
Rediae | ||||
BdL | 437–958 (726) | 252–627 (465) | 235–387 (292) | 247–702 (478) |
BdB | 104–143 (121) | 63–133 (111) | 82–163 (125) | 55–124 (101) |
PhL | 46–72 (61) | 41–90 (55) | 23–40 (33) | 32–59 (37) |
PhB | 32–48 (41) | 29–88 (42) | 33–41 (36) | 29–67 (38) |
PhL / PhB | 1.25–1.79 (1.49) | 0.97–1.51 (1.34) | 0.70–1.06 (0.91) | 0.88–1.10 (0.97) |
IntL | 25–58 (42) | 18–58 (37) | 26–40 (32) | 22–53 (29) |
IntB | 31–54 (42) | 27–80 (48) | 24–49 (33) | 22–39 (29) |
IntL % BL | 0.04–0.10 (0.06) | 0.03–0.12 (0.08) | 0.08–0.15 (0.11) | 0.05–0.12 (0.07) |
Description of cercaria (Fig 7D)
Measurements in Table 6. Description based on all voucher material. Oculate gymnocephalous cercariae. Body elongate, ellipsoidal. Eyespots two, in anterior forebody; additional pigment dispersed in forebody. Oral sucker terminal, infundibuliform. Ventral sucker post-equatorial, round. Prepharynx short, passes between eyespots. Pharynx ellipsoidal. Caecum single, terminating in region dorsal to ventral sucker. Tail longer than body, bipartite; proximal portion bearing series of lateral projections; distal portion scaled, lacking lateral projections. Excretory vesicle Y-shaped, arms extending to anterior margin of ventral sucker, stem extending posterior to ventral sucker; posterior collecting duct visible to first few scales of distal potion of tail; anterior collecting ducts not visible beyond ventral sucker. Genital primordia dorsal to ventral sucker.
Remarks
Gorgocephalus kyphosi has now been demonstrated to occur in four species of Kyphosus, the broadest definitive host range of any known member of the family. This species also occurs across a broad geographic range, from eastern and southern Australia to French Polynesia. Despite the discovery of two new species of gorgocephalid, which are morphologically similar to G. kyphosi, all previous records of this species subsequent to the type description have proven accurate. The previously available 28S rDNA sequence for G. kyphosi of Olson et al. (2003), generated from specimens from K. vaigiensis collected off Lizard Island, fell into a well-supported clade of specimens of this species from Lizard Island. Bray (2005b) and Bray & Cribb (2005) also reported G. kyphosi from Lizard Island, but again these specimens were all from K. vaigiensis; the morphologically similar G. graboides has only been recovered from K. cinerascens. No molecular data are available to confirm the identity of specimens reported from Moorea, French Polynesia (Bray & Cribb, 2005), but that report is again based on specimens recovered from K. vaigiensis. Off Rangiroa, Tuamotu Islands, French Polynesia, we obtained only G. kyphosi and G. yaaji, despite examination of specimens comprising three species of Kyphosus. Thus, we have little doubt that the specimens from Moorea represent G. kyphosi.
Both Manter (1966), in the original type description, and Yamaguti (1971), in study of the same material, reported the number of oral sucker tentacles of Gorgocephalus kyphosi at 14–15. We find that, because of the bifid nature of these tentacles, the fact that they are often partially retracted and that they generally overlay one-another in slide-mounted specimens, it is difficult to be confident in the accuracy of counts of these tentacles obtained during light-microscopy. This difficulty was also noted by Bray & Cribb (2005), who reported the number of oral sucker tentacles in G. yaaji as 14–17. We obtained SEM images of the oral sucker of eight individual adult G. kyphosi, including specimens from three Australian localities and French Polynesia. Although not all tentacles were visible in some of these specimens, in all of those in which an accurate count was possible, the number of tentacles was determined to be 14. Furthermore, the number of oral sucker tentacles was determined to be 14 for all species studied here, and Zhukov (1983) reported the number of tentacles in G. manteri at 14. It appears that all presently recognized species of Gorgocephalus possess 14 oral sucker tentacles.
Manter (1966) described the anterior region of the oesophagus as a ‘dorsal sac’ from which the duct opening as the ventral anus arises. Yamaguti (1971) referred to these structures collectively as the ‘post-pharyngeal vesicle’. Both of these authors also described these structures as separated from the oesophagus proper by a muscular sphincter. Bray & Cribb (2005) did not observe these features in specimens of G. yaaji or in serially sectioned specimens of G. kyphosi. We did not observe these features either, although we did not study any sectioned specimens. If such features are present, they are subtle and difficult to observe in whole-mounted specimens. Thus, we agree with Bray & Cribb (2005) that these features are unlikely to be useful for the differentiation of species.
We have discovered two intermediate hosts for Gorgocephalus kyphosi, Bembicium auratum and Echinolittorina vidua, both gastropods of the family Littorinidae. The distribution of these two gastropods suggests that G. kyphosi utilizes additional intermediate host species throughout its range. Extant species of the genus Bembicium occur only on the coasts of mainland Australia, Tasmania, Lord Howe Island and Norfolk Island (Reid, 1988). Bembicium auratum ranges from South Australia to Lizard Island on the GBR (Reid, 1988), covering the Australian range of G. kyphosi. Echinolittorina vidua ranges throughout the central IWP, but not to French Polynesia (Reid, 2007). Thus, Polynesian populations of G. kyphosi must utilize an intermediate host different from those used by Australian populations, although this host will undoubtedly be a species of the Littorinidae.
No morphological differences were found between the intramolluscan stages of Gorgocephalus kyphosi obtained from Bembicium auratum and Echinolittorina vidua. The cercariae of G. kyphosi are similar to those of the family described previously (O’Dwyer et al., 2015; Huston et al., 2016) and those of Gorgocephalus graboides described here. Although there are some subtle differences between intramolluscan gorgocephalids (see Remarks sections for G. yaaji and G. graboides), matching of these stages to adult forms using molecular markers is likely the best approach for future work elucidating life cycles in this family.
Gorgocephalus yaajiBray & Cribb, 2005
(Figs 9D–F, 10; Tables 3, 6)
Synonym
‘Gorgocephalus sp. Aus’ of O’Dwyer et al. (2015).
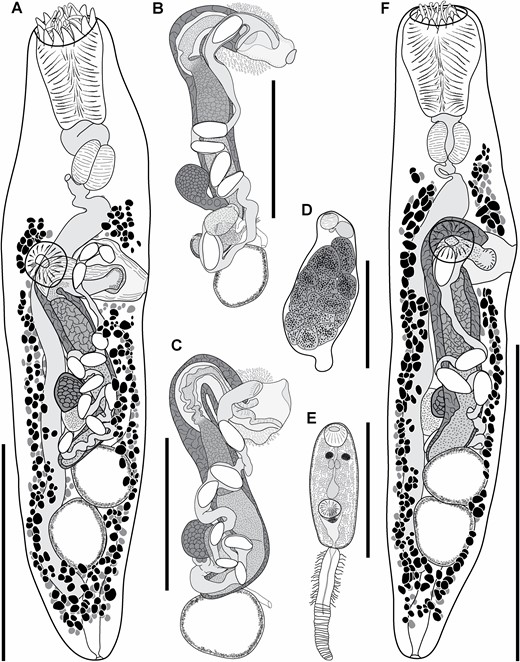
Gorgocephalus yaaji. A, adult voucher ex Kyphosus cinerascens, Sodwana Bay, KwaZulu-Natal, South Africa; ventral perspective. B, genital atrium, cirrus-sac and ovarian complex of adult voucher ex Kyphosus vaigiensis, Sodwana Bay; ventral perspective. C, genital atrium, cirrus-sac and ovarian complex of adult voucher ex Kyphosus cinerascens, Rangiroa, Tuamotu Islands, French Polynesia; ventral perspective. D, redia ex Echinolittorina cinerea, Rangiroa; lateral perspective. E, excised cercaria ex Echinolittorina cinerea, Rangiroa; ventral perspective. F, adult voucher ex Kyphosus cinerascens, Rangiroa; ventral perspective. Scale bars: A, F, 500 μm; B, C, D, E, 250 μm.
Type host and locality
Kyphosus vaigiensis (Quoy & Gaimard, 1825) (Perciformes: Kyphosidae), brassy chub from off Lizard Island, Great Barrier Reef, Queensland, Australia (14°41’10’’S, 145°28’15’’E).
Records
1, Bray & Cribb (2005); 2, O’Dwyer et al. (2015); 3, Huston et al. (2016); 4, present study.
Definitive hosts
Perciformes, Kyphosidae. Kyphosus cinerascens (Forsskål, 1775), highfin chub (3, 4); Kyphosus elegans (W. K. H. Peters, 1869), Cortez sea chub (4); Kyphosus vaigiensis (Quoy & Gaimard, 1825) (1, 4).
Intermediate hosts
Gastropoda, Littorinimorpha, Littorinidae. Austrolittorina unifasciata (Gray) (2, 3); Echinolittorina austrotrochoidesReid, 2007 (3); Echinolittorina cinerea (Pease, 1869) (4).
Other localities
Gerringong, New South Wales, Australia (34°44’18’’S, 150°49’29’’E) (2); Kioloa, New South Wales, Australia (35°33’38’’S, 150°22’27’’E) (KI) (3); Shellharbour, New South Wales, Australia (34°34’59’’S, 150°52’E) (2); Sydney, New South Wales, Australia (33°52’42’’S, 151°12’34’’E) (2); Ulladulla, New South Wales, Australia (35°21’17’’S, 150°27’43’’E) (2); off Rangiroa, Tuamotu Islands, French Polynesia (15°10’40’’S, 147°39’04’’W) (4); Sodwana Bay, KwaZulu-Natal, South Africa (27°32’24’’S, 32°40’41’’E) (SB) (4).
Voucher material (adult)
Ten whole-mount and three hologenophore specimens, ex K. vaigiensis from LI (QM G238592–G238604); six whole-mount and three hologenophore specimens ex K. cinerascens from RA (MNHN HEL1447–1455); one whole-mount specimen ex K. elegans from RA (MNHN HEL1456); three whole-mount and one hologenophore specimen ex K. cinerascens from SB (NMB 730); six whole-mount specimens ex K. vaigiensis from SB (NMB 731).
Voucher material (intramolluscan)
Eight slides of rediae and cercariae ex E. cinerea from RA (MNHN HEL1457–1464).
Site in host
Upper intestine (definitive); gonad/digestive gland (intermediate).
Representative DNA sequences
Nineteen sequences (MW353650–MW353668), using primers of Wee et al. (2017), and three sequences (MW350141–MW350143), following O’Dwyer et al. (2016) (see methods) deposited for COI mtDNA; 17 sequences deposited for 5.8S-ITS2-partial 28S rDNA (MW353931–MW353947); nine sequences deposited for partial 28S rDNA (MW353889–MW353897); see Supporting Information, Table S2.
Description of adult
(Figs 9D–F, 10A–C, F: Measurements in Table 3). Description based upon all adult voucher material and SEM images of three adults. Body elongate-oval, somewhat dorsoventrally flattened, broadest in region of ventral sucker, tapering slightly posteriorly. Tegument armed with alternating rows of partially overlapping comb-like scales; distal portion of scales forming up to 18 distinct tendrils. Eyespot pigment sparsely scattered in forebody. Oral sucker terminal, partially retractable, infundibuliform, broadest in anterior region with distinct reduction in diameter about mid-length continuing through to posterior margin; margin of anterior portion bearing crown of 14 bifid tentacles; outer branch of tentacles broad, conoid; inner branch of tentacles approximately equal in length to outer, tendril-like, tapering distally. Ventral sucker in anterior third of body, round, smaller than oral sucker. Prepharynx short, distinct, sigmoid or looped. Pharynx ellipsoidal to dolioform, in line with oral sucker or rotated up to 45°. Oesophagus short, bifurcates just posterior to pharynx with proximal section reaching to ventral surface and opening as ‘ventral anus’, and distal portion expanding to form caecum. Caecum single, broadest in anterior region, passes from mid-forebody to close to posterior extremity, terminates blindly; gastrodermis well developed.
Testes two, ellipsoidal, tandem, contiguous, in mid-hindbody. Vasa deferentia narrow, passing relatively direct from testes to cirrus-sac. Cirrus-sac elongate, cylindrical, winding, reaching from anterior testis to level of ventral sucker; anterior portion curves back posteriorly from ventral sucker to genital atrium. Internal seminal vesicle tubular, bends slightly about mid-length, occupies about half length of cirrus-sac. Pars prostatica distinct, vesicular, approximately equal in length to internal seminal vesicle, lined with anuclear cell-like bodies. Ejaculatory duct long, occupies recurved portion of cirrus-sac. Genital atrium broad, dorsal and approximately equal in size to ventral sucker. Genital pore large, round to irregular, opening dorsodextrally at level of ventral sucker.
Ovary pre-testicular, ellipsoidal to subglobular. Mehlis’ gland between ovary and anterior testis. Laurer’s canal opens dorsally posterior to ovary. Canalicular seminal vesicle saccular, contiguous with and dorsal to ovary. Uterus narrow, passes posteriorly from oötype to anterior testis, loops back, gently winding anteriorly, forming muscular metraterm about mid-level of pars prostatica, opening into genital atrium adjacent to ejaculatory duct. Eggs few, oval, operculate, large; length of eggs often exceeding that of ovary. Vitellarium follicular, in fore- and hindbody, fields reaching from pharyngoesophageal region to near posterior extremity; dorsal, lateral and ventral fields confluent, wrap around body from dorsal midline to ventrosinistral and ventrodextral regions anterior to testes, wrap entire body posterior to testes. Vitelline reservoir between ovary and anterior testis; collecting ducts indistinct. Excretory pore terminal; excretory vesicle Y-shaped, passes anteriorly, bifurcating in testicular region, ducts passing anteriorly sinistrally and dextrally, terminating as enlarged pyriform sacs on either side of cirrus-sac.
Description of redia (Fig. 10D)
Measurements in Table 6. Description based on voucher material. Body ellipsoidal, broadest posteriorly; distinctive protuberance arising from posterior extremity in most specimens. Cercarial embryos numerous, poorly developed. Mouth subterminal. Pharynx ellipsoidal. Intestine short, globular, immediately posterior and subequal in size to pharynx.
Description of cercaria (Fig. 10E)
Measurements in Table 6. Description based on voucher material. Oculate gymnocephalous cercariae. Body elongate, ellipsoidal. Eyespots two, in anterior forebody; eyespot lenses present in live naturally emerged specimens, not present in immature specimens. Oral sucker subterminal, infundibuliform. Ventral sucker post-equatorial, round. Prepharynx short, passes between eyespots. Pharynx ellipsoidal. Caecum single, terminating near anterior margin of ventral sucker. Tail bipartite; proximal portion bearing series of lateral projections; distal portion scaled, lacking lateral projections. Excretory vesicle Y-shaped; arms extending to ventral sucker, stem extending to near posterior extremity; posterior collecting duct visible to first few scales of distal portion of tail; anterior collecting ducts not visible beyond ventral sucker. Genital primordia darkly stained, dorsal to ventral sucker.
Remarks
Gorgocephalus yaaji occurs across a wider geographic range than any other species of the family. Indeed, to our knowledge the finding of G. yaaji in French Polynesia, South Africa, and in between, is the first report, supported by molecular data, of naturally occurring populations of a single marine digenean species occurring across the whole breadth of the IWP. Although it has fewer definitive host records than G. kyphosi, three gastropod intermediate hosts are known for G. yaaji, the most for any species of the family. O’Dwyer et al. (2015) described the first gorgocephalid cercariae from the littorinid A. unifasciata, but did not connect these infections to an adult gorgocephalid, because their sequences did not match those of G. kyphosi from the study of Olson et al. (2003), the only sequences available for the family at that time. Huston et al. (2016) described the cercariae of G. yaaji from Echinolittorina austrotrochoides from Lizard Island, GBR, and determined that, based on the difference in host, molecular differences in the ITS2 and 28S rDNA gene-regions, and morphological differences, the infections characterized by O’Dwyer et al. (2015) were likely those of an undescribed species. However, by adding an additional molecular marker (COI) and performing expanded phylogenetic study of the family, we have demonstrated that the intramolluscan infections described by O’Dwyer et al. (2015) are best interpreted as representative of G. yaaji. The host, molecular and morphological differences reported by Huston et al. (2016) between their material and that of O’Dwyer et al. (2015) are clearly within the bounds of intraspecific variation for G. yaaji.
Huston et al. (2016) noted that the morphological differences between their cercarial specimens of Gorgocephalus yaaji and those of O’Dwyer et al. (2015) may have been a result of differences between ‘naturally emerged’ cercariae and cercariae excised from gastropods. O’Dwyer et al. (2015) studied live and fixed cercariae dissected from gastropods, whereas Huston et al. (2016) studied live and fixed naturally emerged cercariae. Naturally emerged cercariae are generally considered as ‘mature’, whereas cercariae obtained from dissection of a gastropod may include mature and immature individuals. As stated above, the morphometric differences observed between G. yaaji cercariae appear due to normal intraspecific variation. However, one of the key morphological differences between the material of O’Dwyer et al. (2015) and Huston et al. (2016) was the observation of distinct ‘lenses’ in the eyespots of the live cercariae from the latter study. O’Dwyer et al. (2015) studied live material from dissected gastropods and is unlikely to have missed such a distinctive feature if it was present. Huston et al. (2016) interpreted this lack of eyespot lenses as indicative of a species-level difference. However, we did not obtain naturally emerged cercariae of G. yaaji from Echinolittorina cinerea in French Polynesia, although we did study live material from dissected gastropods. We did not observe eyespot lenses in the French Polynesian cercariae. Thus, as all of these cercariae are conspecific, we interpret the presence of eyespot lenses in the cercariae from the study of Huston et al. (2016) as a feature that is likely present only in mature, naturally emerged cercariae.
Five of the six known intermediate host records for gorgocephalids are attributable to Gorgocephalus kyphosi and G. yaaji. These two species have yet to be confirmed as having overlapping intermediate host ranges, but this remains possible. We obtained naturally emerged cercariae of G. kyphosi from an infected Echinolittorina vidua from Lizard Island, GBR, the same locality from which Huston et al. (2016) obtained naturally emerged cercariae of G. yaaji from E. austrotrochoides. Although there are no clear morphometric differences between the cercariae of G. kyphosi and G. yaaji, we did not observe eyespot lenses in the cercariae of G. kyphosi. However, as naturally emerged cercariae were studied from only a few infected gastropods, at present it is difficult to have confidence in the validity of this character for species differentiation. Additionally, Huston et al. (2016) described the rediae of G. yaaji from infected E. austrochoides from Lizard Island, GBR, with a strange posterior ‘protuberance’. We observed the same feature in the rediae of G. yaaji from infected E. cinerea from Rangiroa, French Polynesia. This feature was not present in any of the rediae of G. kyphosi that we observed. We do note that this ‘protuberance’ was not reported by O’Dwyer et al. (2015), so this would seem a somewhat tenuous feature for the differentiation of species. Despite repeated attempts, we were never able to obtain naturally emerged cercariae of G. kyphosi from Bembicium auratum. Considering the cryptic morphology of gorgocephalid cercariae, and the difficulty of obtaining naturally emerged specimens, use of molecular data for the identification of intramolluscan gorgocephalid infections will be required in most cases.
As noted above, Bray & Cribb (2005) reported the number of oral sucker tentacles in G. yaaji as 14–17; based on our SEM images we believe that this species, and all known gorgocephalids, have only 14 bifid tentacles. Although Manter (1966) reported G. kyphosi from both the pyloric caeca and intestine of K. sydneyanus, Bray & Cribb (2005) found that in K. vaigiensis from Lizard Island, G. kyphosi was restricted to the pyloric caeca whereas G. yaaji was found in the intestine. Our observations agree. With the exception of G. yaaji, all species of Gorgocephalus we collected were found only in the pyloric caeca. We found G. yaaji in the upper intestine, just posterior to the pyloric caeca.
Gorgocephalus euryaleae sp. nov. (Figs 11, 12A–C; Tables 4, 6)
Zoobank registration
urn:lsid:zoobank.org:act:7C5053D1-DE14-400E-A8FC-36F6E1A305B6.
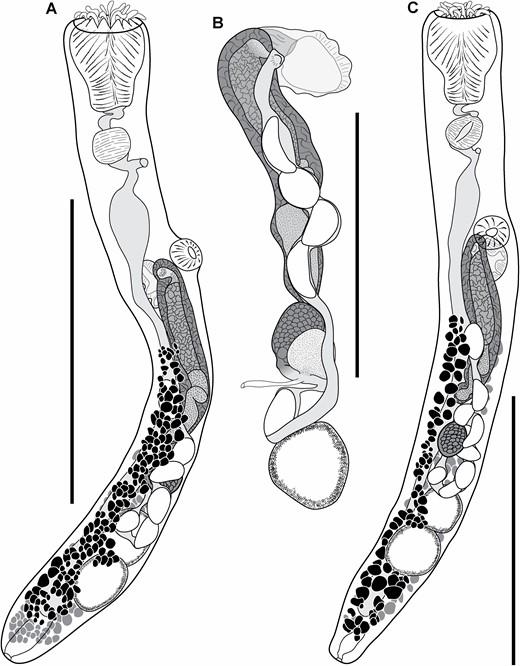
Gorgocephalus euryaleae. A, paratype ex Kyphosus gladius, Point Peron, Rockingham, Western Australia; lateral perspective. B, genital atrium, cirrus-sac and ovarian complex of separate paratype ex Kyphosus gladius, Point Peron; lateral perspective. C, holotype ex Kyphosus gladius, Point Peron; ventral perspective. Scale bars: A, C, 500 μm; B, 250 μm.
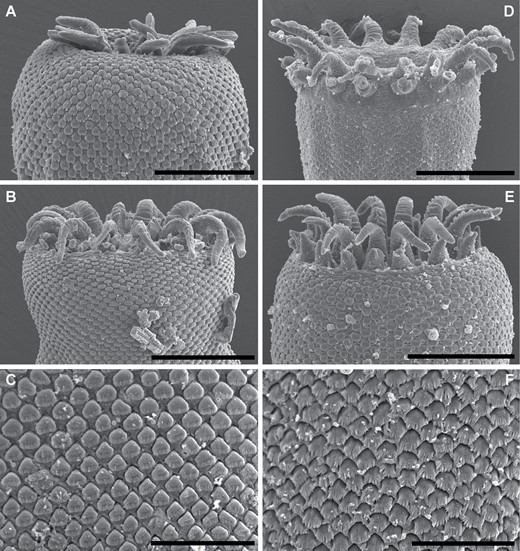
Gorgocephalus euryaleae and Gorgocephalus graboides, scanning electron micrographs. A, B, oral suckers of adult Gorgocephalus euryaleae ex Kyphosus gladius, Point Peron, Rockingham, Western Australia. C, tegument of adult Gorgocephalus euryaleae, ex Kyphosus gladius, Point Peron. D, E, oral suckers of adult Gorgocephalus graboides ex Kyphosus cinerascens, Lizard Island, Queensland, Australia. F, tegument of adult Gorgocephalus graboides ex Kyphosus cinerascens, Lizard Island. Scale bars: A, E, 40 μm; B, D, 50 μm; C, F, 20 μm.
Type host and locality
Kyphosus gladius Knudsen & Clements (Perciformes: Kyphosidae), gladius sea chub, from off Point Peron, Rockingham, Western Australia (32°15’59’’S, 115°41’03’’E) (PP).
Other hosts (definitive)
Kyphosus sydneyanus (Günther, 1886) (Perciformes: Kyphosidae), silver drummer; Kyphosus cinerascens (Forsskål, 1775), highfin chub (Perciformes: Kyphosidae).
Other localities
Off Rottnest Island, Western Australia (32°59’04’’S, 115°31’06’’E) (RI); Sodwana Bay, KwaZulu-Natal, South Africa (27°32’24’’S, 32°40’41’’E).
Type material
Holotype (WAM V9670) ex K. gladius from off PP. Paratypes: eight whole-mount specimens and two hologenophores ex K. gladius from PP (WAM V9671–9680); one whole-mount and three hologenophores ex K. sydneyanus from RI (WAM V9681–9684).
Additional voucher material (adult)
Eight whole-mount and two hologenophore specimens, ex K. cinerascens from SB (NMB 732).
Site in host
Pyloric caeca.
Representative DNA sequences
Eight sequences deposited for COI mtDNA (MW353669–MW353676); 11 sequences deposited for 5.8S-ITS2-partial 28S rDNA (MW353948–MW353958); seven sequences deposited for partial 28S rDNA (MW353898–MW353904); see Supporting Information, Table S2.
Etymology
In Greek mythology, Euryale was one of the Gorgons, three sisters with living snakes for hair. The name Euryale has various translations, including ‘far-roaming’, ‘wide-leaping’, ‘the far-springer’ and ‘of the wide sea’. Because this new species of Gorgocephalus was collected from both sides of the Indian Ocean, naming it for Euryale seems especially appropriate.
Description of adult (Figs 11, 12A–C )
Measurements in Table 4. Description based upon type series and SEM images of three adult specimens. Body elongate, cylindrical, broadest in region just posterior to ventral sucker, tapering slightly posteriorly. Tegument armed with alternating rows of partially overlapping comb-like scales; distal portion of scales forming up to 15 distinct tendrils. Eyespot pigment sparsely scattered in forebody and anterior hindbody. Oral sucker terminal, partially retractable, infundibuliform, broadest in anterior region with distinct reduction in diameter about mid-length continuing through to posterior margin; margin of anterior portion bearing crown of 14 bifid tentacles; outer branch of tentacles broad, conoid; inner branch of tentacles longer than outer, tendril-like, tapering distally. Ventral sucker in anterior of middle-third of body, round, far smaller than oral sucker. Prepharynx short, distinct, sigmoid or looped. Pharynx ellipsoidal to dolioform, in line with oral sucker or rotated up to 90°. Oesophagus short, bifurcates just posterior to pharynx with proximal section reaching to ventral surface and opening as ‘ventral anus’, and distal portion expanding to form caecum. Caecum single, broadest in anterior region, passes from mid-forebody to close to posterior extremity, terminates blindly; gastrodermis well developed.
Testes two, ellipsoidal, tandem, contiguous, in mid-hindbody. Vasa deferentia narrow, passing relatively direct from testes to cirrus-sac. Cirrus-sac elongate, cylindrical, winding, reaching from just anterior to ovary to level of ventral sucker. Internal seminal vesicle tubular, loops once about mid-length, occupies less than half the length of the cirrus-sac. Pars prostatica distinct, vesicular, occupies more than half of the cirrus-sac, lined with anuclear cell-like bodies. Ejaculatory duct short. Genital atrium broad, dorsal and approximately equal in size to ventral sucker. Genital pore large, round to irregular, opening dorsally at level of ventral sucker.
Ovary pre-testicular, pyriform, narrowing posteriorly toward union with oötype. Mehlis’ gland indistinct. Laurer’s canal opens dorsally at level of ovary. Canalicular seminal vesicle saccular, contiguous with and dorsal to ovary. Uterus narrow, passes posteriorly from oötype to anterior testis, loops back, gently winding anteriorly as far as genital atrium, forming muscular metraterm about mid-level of pars prostatica, opening into genital atrium adjacent to ejaculatory duct. Eggs few, oval, operculate, large; length often exceeding that of ovary. Vitellarium follicular, restricted to hindbody; fields reaching from about mid-cirrus-sac to near posterior extremity; dorsal, lateral and ventral fields confluent, wrap around body from dorsal midline to ventrosinistral and ventrodextral regions anterior to testes, wrap entire body posterior to testes. Vitelline reservoir between ovary and anterior testis; collecting ducts indistinct. Excretory pore terminal; excretory vesicle Y-shaped, passes anteriorly, bifurcating in testicular region, ducts passing anteriorly sinistrally and dextrally, terminating as enlarged pyriform sacs on either side of cirrus-sac.
Remarks
There are no clear morphometric differences between Gorgocephalus euryaleae and G. kyphosi; all measured values fall on a continuum (Fig. 2; Tables 2, 4). Although these two species share two definitive hosts, Kyphosus cinerascens and K. sydneyanus, we have not found them in sympatry, as we did not find any specimens of G. kyphosi in Western Australia or South Africa. There are also some subtle morphological differences between G. euryaleae and G. kyphosi. The tegumental scales of G. kyphosi appear somewhat more elongate, with more elongate posterior tendrils than those of G. euryaleae, which are more compact, and have shorter posterior tendrils. However, such differences are potential artefacts of the SEM process. Parts of the tegument may be sloughed off during specimen preparation and detail may be obscured due to the thickness of coating. A more reliable difference between these two species involves the cirrus-sac. In G. kyphosi the internal seminal vesicle and pars prostatica each occupy about half of the cirrus-sac whereas in G. euryaleae the internal seminal vesicle occupies less than half the length of the cirrus-sac and the pars prostatic occupies more than half. The genital atrium is also larger than the ventral sucker in specimens of G. kyphosi, whereas in G. euryaleae the two features are similar in size.
From Gorgocephalus manteri, G. euryaleae differs primarily in having a much larger body and in having vitelline follicles restricted to the hindbody, rather than having them extend into the forebody as in G. manteri. The illustration of G. manteri also shows the cirrus-sac as strongly sigmoid in shape (Zhukov, 1983), versus the slighter bend apparent in specimens of G. euryaleae. Bray & Cribb (2005) calculated several morphometric ratios for G. manteri, and the species has a much greater body width as a percentage of body length (28–34% vs. 10–17%), lesser ovary to ventral sucker distance as a percentage of body length (~5% vs. 19–30%) and a larger ovary to testis distance as a percentage of body length (~17% vs. ~2–8%) than G. euryaleae.
Gorgocephalus euryaleae is readily distinguished from G. yaaji. The latter species has a much broader and more dorsoventrally flattened body shape than that of G. euryaleae, and has vitelline follicles that extend into the forebody, rather than having them entirely restricted to the hindbody. Although G. yaaji has, as a percentage of body length, a longer pharynx (6.0–10.2% vs. 3.7–5.8%), and measures of many features are different on average, most measures and ratios still fall into a continuum. However, there are further morphological differences: the inner and outer portions of the oral sucker tentacles of G. yaaji are approximately equal in length, rather than having the inner portion longer as in G. euryaleae. The tegument scales of G. yaaji also have more posterior tendrils than those of G. euryaleae (up to 18 vs. up to 15), though again, the reliability of the tegument as a diagnostic feature is presently uncertain. Lastly, the cirrus-sac of G. yaaji is much more robust than that of G. euryaleae and has a distinctly recurved anterior portion, a feature not present in the latter species.
Gorgocephalus graboides sp. nov. (Figs 12D–F, 13; Tables 4, 6)
Zoobank registration
urn:lsid:zoobank.org:act:D684AF8B-5CF6-40A0-91AD-7B26A304D47B.
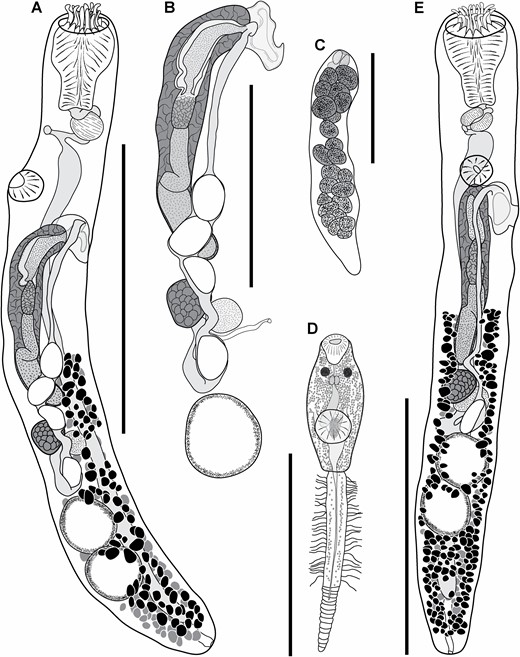
Gorgocephalus graboides A, paratype ex Kyphosus cinerascens, Lizard Island, Queensland, Australia; lateral perspective. B, genital atrium, cirrus-sac and ovarian complex of paratype; lateral perspective. C, redia ex Echinolittorina vidua, Lizard Island; ventral perspective. D, emerged cercaria ex Echinolittorina vidua, Lizard Island; ventral perspective. E, holotype ex Kyphosus cinerascens, Lizard Island; ventral perspective. Scale bars: A, E, 500 μm; B, C, D, 250 μm.
Type host and locality
Kyphosus cinerascens (Forsskål, 1775), highfin chub (Perciformes: Kyphosidae) from off Lizard Island, Great Barrier Reef, Queensland, Australia (14°41’10’’S, 145°28’15’’E).
Other hosts (intermediate)
Echinolittorina vidua (Gould, 1859) (Gastropoda: Littorinimorpha: Littorinidae).
Type material
Holotype (QM G238605) ex K. cinerascens from off LI. Paratypes: 14 whole mount and three hologenophore specimens ex K. cinerascens from LI (QM G238606–G238622).
Additional voucher material (intramolluscan)
Five slides of cercariae and rediae ex E. vidua from LI (QM G238623–G238627).
Site in host
Pyloric caeca (definitive); gonad/digestive gland (intermediate).
Representative DNA sequences
Six sequences deposited for COI mtDNA (MW353677–MW353682); six sequences deposited for 5.8S-ITS2-partial 28S rDNA (MW353959–MW353964); four sequences deposited for partial 28S rDNA (MW353905–MW353908); see Supporting Information, Table S2.
Etymology
This species is named for its resemblance to the monsters from the Tremors films, ‘graboids’. Graboids are blind, subterranean, worm-like predators with grasping tentacles that emerge from their beak-like maws. Graboids even have complex life-cycles, transitioning through several radically different phenotypes. The name is formed by combination of ‘graboid’ and a Latinized Greek suffix indicating a resemblance or likeness, ‘oides’.
Description of adult (Figs 12D–F, 13A, B, E)
Measurements in Table 4. Description based on type material and SEM images of three adult specimens. Body elongate, cylindrical, broadest in region of ventral sucker, tapering slightly posteriorly. Tegument armed with alternating rows of partially overlapping comb-like scales; distal portion of scales forming up to 15 distinct tendrils. Eyespot pigment sparsely scattered in forebody and anterior third of hindbody. Oral sucker terminal, partially retractable, infundibuliform, broadest in anterior region with distinct reduction in diameter about mid-length continuing through to posterior margin; margin of anterior portion bearing crown of 14 bifid tentacles; outer branch of tentacles broad, conoid; inner branch of tentacles longer than outer, tendril-like, tapering distally. Ventral sucker in anterior third of body, round, smaller than oral sucker. Prepharynx short, distinct, sigmoid or looped. Pharynx ellipsoidal to dolioform, in line with oral sucker or rotated up to 90°. Oesophagus short, bifurcates just posterior to pharynx with proximal section reaching to ventral surface and opening as ‘ventral anus’, and distal portion expanding to form caecum. Caecum single, broadest in anterior region, passes from mid-forebody to close to posterior extremity, terminates blindly; gastrodermis well developed.
Testes two, ellipsoidal, tandem, contiguous or separated, in mid hindbody. Vasa deferentia narrow, passing relatively direct from testes to cirrus-sac. Cirrus-sac elongate, cylindrical, gently winding, reaching from just anterior to ovary to posterior margin of ventral sucker. Internal seminal vesicle tubular, loops once about mid-length, occupies less than half length of cirrus-sac. Pars prostatica distinct, vesicular, less than half length of internal seminal vesicle, lined with anuclear cell-like bodies. Ejaculatory duct distinct, approximately equal in length to, or longer than, pars prostatica; opens into genital atrium. Genital atrium narrow, posterior-dorsal and subequal to ventral sucker. Genital pore small, round to irregular, opening dorsally at level of ventral sucker.
Ovary pre-testicular, pyriform, narrowing posteriorly toward union with oötype. Mehlis’ gland indistinct. Laurer’s canal opens dorsally at level of ovary. Canalicular seminal vesicle saccular, contiguous with and dorsal to ovary. Uterus narrow, passes posteriorly from oötype to anterior testis, loops back, gently winding anteriorly, forming muscular metraterm about mid-level of pars prostatica, opening into genital atrium adjacent to ejaculatory duct. Eggs few, oval, operculate, large; length often exceeding that of ovary. Vitellarium follicular, restricted to hindbody; fields reaching from about mid-cirrus-sac to near posterior extremity; dorsal, lateral and ventral fields confluent, wrap around body from dorsal midline to ventrosinistral and ventrodextral regions anterior to testes, wrap entire body posterior to testes. Vitelline reservoir between ovary and anterior testis; collecting ducts indistinct. Excretory pore terminal; excretory vesicle Y-shaped, passes anteriorly, bifurcating in testicular region, ducts passing anteriorly sinistrally and dextrally, terminating as enlarged pyriform sacs on either side of cirrus-sac.
Description of redia (Fig 13C)
Measurements in Table 6. Description based on voucher material. Body elongate, broadest anteriorly, tapering posteriorly. Cercarial embryos numerous, poorly developed. Mouth terminal. Pharynx dolioform. Intestine short, globular, immediately posterior and subequal in size to pharynx.
Description of cercaria (Fig 13E)
Measurements in Table 6. Description based on voucher material. Oculate gymnocephalous cercariae. Body elongate, fusiform. Eyespots two, in anterior forebody; additional pigment conspicuous, dispersed in forebody. Oral sucker terminal, infundibuliform. Ventral sucker post-equatorial, round. Prepharynx short, passes between eyespots. Pharynx ellipsoidal. Caecum single, terminating in region dorsal to ventral sucker. Tail longer than body, bipartite; proximal portion bearing series of lateral projections; distal portion scaled, lacking lateral projections. Excretory vesicle Y-shaped, arms extending to ventral sucker, stem extending to near posterior extremity; posterior collecting duct visible to first few scales of distal potion of tail; anterior collecting ducts not visible beyond ventral sucker. Genital primordia darkly stained, dorsal to ventral sucker.
Remarks
There are no clear morphometric features that differentiate this species from Gorgocephalus kyphosi, and these two species have overlapping host ranges at both the definitive and intermediate host level. However, the cirrus-sac can again be used for differentiation. In G. kyphosi, the ejaculatory duct is short and the internal seminal vesicle and pars prostatica each occupy about half of the cirrus-sac, whereas in G. graboides the internal seminal vesicle occupies less than half of the cirrus-sac and the pars prostatica is less than half the length of the internal seminal vesicle; the remaining space is occupied by a long, distinct ejaculatory duct, which is about as long as, or longer than, the pars prostatica. Furthermore, the genital atrium is larger than the ventral sucker in specimens of G. kyphosi, whereas in G. graboides the two features are similar in size.
From Gorgocephalus euryaleae, G. graboides differs in having a shorter pars prostatica (occupying less than half, rather than more than half, of the cirrus-sac) and in having a longer ejaculatory duct (equal or greater in length than the pars prostatica vs. shorter than the pars prostatica). Although both species share K. cinerascens as a host, they appear to be geographically isolated from one another.
From Gorgocephalus manteri, G. graboides differs primarily in having a larger body and in having vitelline follicles restricted to the hindbody, rather than having them extend into the forebody. The cirrus-sac of G. graboides also lacks the strong sigmoidal shape of that of G. manteri and based on the calculations of Bray & Cribb (2005), G. manteri has, as a percentage of body length, a much greater body width (28–34% vs. 8–11%), lesser ovary to ventral sucker distance (~5% vs. 27–33%) and greater ovary to testis distance (~17% vs. 4–9%) than G. graboides.
Gorgocephalus graboides is easily distinguished from G. yaaji in having a far less dorsoventrally flattened body, and in having vitelline follicles restricted to the hindbody, rather than having them extend into the forebody. Gorgocephalus graboides also differs from G. yaaji morphometrically, namely in having, as a percentage of body length, a shorter forebody (21–26% vs. 31–42%), a shorter pharynx (3.0–4.3% vs. 6.0–10.2%) and a longer testis to ventral sucker distance (36–44% vs. 22–32%). Gorgocephalus graboides also has a lesser pharynx length to oral sucker length ratio than G. yaaji (0.27–0.35 vs. 0.37–0.55). The inner and outer portions of the oral sucker tentacles of G. yaaji are approximately equal in length, rather than having the inner portion longer like in G. graboides and the tegument scales of G. yaaji have more posterior tendrils than those of G. graboides (up to 18 vs. up to 15). Lastly, the cirrus-sac of G. graboides is less robust than that of G. yaaji and does not have the anterior portion recurved.
Gorgocephalus kyphosi and G. graboides share an intermediate host, Echinolittorina vidua, at Lizard Island, GBR, and other than the cercariae of G. graboides having a somewhat more fusiform body shape, we were unable to find any clear morphometric or qualitative differences between the intramolluscan stages of these two species. Thus, intramolluscan stages of G. kyphosi and G. graboides can only be distinguished on a molecular basis. The same subtle differences found between intramolluscan stages of G. kyphosi and G. yaaji apply when comparing G. graboides to G. yaaji. We obtained naturally emerged cercariae of G. graboides and did not observe the eyespot ‘lenses’ described for naturally emerged cercariae of G. yaaji (Huston et al., 2016). We also did not observe the posterior ‘protuberance’ in the rediae of G. graboides. However, as discussed above, these seem weak characters for species delineation.
DISCUSSION
We chose to use an integrated approach, requiring more than one line of evidence, for recognition of species in the Gorgocephalidae. Because there is significant overlap in host, at both the definitive and intermediate levels, and relatively little evidence of geographic isolation between species of Gorgocephalus, we could rely only on morphology and molecular sequence data for developing species hypotheses. Thus, although our molecular phylogenetic analyses suggest multiple lineages of Gorgocephalus among our IWP collections, only four species were supported upon synthesis of morphological and molecular data and a conservative interpretation. Ultimately, we find our species concepts derived from this approach to be the most satisfactory, largely because it allows us to avoid the invocation of cryptic species. The existence of cryptic species has gained wide acceptance in parasite systematics (Pérez-Ponce de León & Nadler, 2010; Nadler & Pérez-Ponce de León, 2011; Poulin, 2011; Pérez-Ponce de León & Poulin, 2018), but recognition and delineation of cryptic species still presents significant practical and philosophical challenges for cataloguing and understanding biodiversity as a whole (Schlick-Steiner et al., 2007; Pérez-Ponce de León & Nadler, 2010; Jörger & Schrödl, 2013; Delić et al., 2017; Huston et al., 2019a). Although our phylogenetic results might be considered to include evidence for one or more truly cryptic species within our concepts of G. kyphosi and G. yaaji, we think that these molecular phenomena can be rationalized such that they fit within the umbrella of intraspecific species variation.
Enigmatic population connectivity in Indo-West Pacific gorgocephalids
Among the most notable phenomena in the present study is the apparent genetic isolation of gorgocephalids collected from off Lizard Island (northern GBR) from other Pacific populations. Sequences of both Gorgocephalus kyphosi and G. yaaji from Lizard Island are less similar to sequences from other Australian localities than the other Australian sequences are to those from French Polynesia. This is an unusual phenomenon, but not one without precedent. Using COI and ITS2 gene sequences, McNamara et al. (2014) characterized species of the monorchiid genus Hurleytrematoides Yamaguti, 1954 from multiple sites across the IWP, including specimens from two sites on the GBR (Lizard Island, northern GBR, and Heron Island, southern GBR) and from off Moorea, French Polynesia. McNamara et al. (2014) found that sequences of one morphologically distinctive species, Hurleytrematoides morandi McNamara & Cribb, 2011, generated from specimens from Lizard Island, were more similar to sequences of the same species from French Polynesia, a straight distance of about 6900 km, than they were to sequences from Heron Island, only some 1200 km to the south. This is similar to what we have observed for G. kyphosi and G. yaaji, although in the present study it is the Lizard Island populations that appear more isolated. This pattern of genetic distinctiveness exhibited by G. kyphosi and G. yaaji at Lizard Island suggests that there is a barrier to gene flow between Lizard Island gorgocephalids and those from other Pacific localities sampled in the present study. However, the specific processes resulting in such a barrier are unclear.
The nature of the gorgocephalid life cycle suggests that cercarial dispersal is local in scale (Huston et al., 2016) and, therefore, these trematodes are reliant on the vagility of their kyphosid hosts for the dispersal of eggs, and thus genetic mixing, across range. It would follow then, that genetic isolation for a population of gorgocephalids would correspond to a break in the connectedness of their hosts. However, there are no obvious breaks in the distributions of susceptible littorinid or kyphosid hosts between Lizard Island and other Australian localities, as there are suitable habitat patches for both groups along essentially all of the coastline of Australia (Reid, 1988; Reid et al., 2006; Reid, 2007; Knudsen & Clements, 2013b, 2016). Although ocean currents and winds along the coastline of eastern Australia may inhibit genetic mixing for some marine species (Gopurenko & Hughes, 2002), the phylogeographic structure of Australian littorinid gastropods shows no clear correspondence with prevailing currents (Reid et al., 2006). Furthermore, kyphosids are known to regularly travel much greater distances than other marine herbivorous fishes, including across breaks in habitat continuity and between reefs and islands (Welsh & Bellwood, 2014; Sakihara et al., 2015). Some species of kyphosids are colloquially referred to as ‘rudderfish’ because of their habit of following ships. Present-day host distribution and ocean currents appear to provide little explanation for isolation of Lizard Island gorgocephalids. Thus, other ecological, geological or historic explanations require exploration, but further speculation is beyond the scope of this study.
Species delineation in the face of genetic variation over geographic range
Choosing an integrated model for species recognition mitigates the potential pitfalls of using molecular ‘yardsticks’ to determine digenean species boundaries over geographic range; i.e. misinterpreting intraspecific variation as interspecific variation. The impact of this model on gorgocephalid species richness in the IWP is perhaps most pronounced in our treatment of Gorgocephalus yaaji. Although we did not detect any morphological differences between adult specimens consistent with G. yaaji from any of our collection sites, this species was not recovered as monophyletic in all phylogenetic analyses. In addition, in our concept of G. yaaji we observe maximum intraspecific genetic variation nearing, or in some cases even exceeding, interspecific variation for the family. Interspecific variation for the four species of Gorgocephalus sequenced here, incorporating all geographic combinations, ranges from 14–18%, 0.9–4.2% to 0.5–2.3% in the COI mtDNA, ITS2 and 28S rDNA gene regions, respectively. For G. yaaji, the maximum intraspecific variation for COI sequences is 13%, close to the interspecific minima, and intraspecific differences up to 1.1% in the ITS2 and up to 0.6% in the 28S rDNA gene regions were observed, exceeding minimum interspecific differences. However, these intraspecific maxima are observed when comparing sequences from South Africa with those from the two most geographically distant collection localities (New South Wales, Australia and Rangiroa, French Polynesia), which, for parasites with a wide range but low dispersal, should be expected. The paraphyletic relationships resolved for G. yaaji in some of our phylogenetic analyses are likely the result of a lack of sequences for this species from intermediate localities, i.e. northern Australia, the Asian-Pacific and along the northern borders of the Indian Ocean. Considering the paraphyletic, rather than polyphyletic, relationships resolved for G. yaaji, coupled with the lack of any host or morphological differences over a vast geographic range, we think it most satisfactory to view G. yaaji as a single wide-ranging species rather than assigning divergent clades to morphologically cryptic species.
While our integrated approach permits ‘lumping’ all our specimens corresponding to the Gorgocephalus yaaji morphotype into a single species, it also allows for the splitting of the G. kyphosi morphotype into three species, despite only subtle morphological differences. Similar to G. yaaji, maximum intraspecific variation for sequences of G. kyphosi, at 13%, 0.7% and 0.3% in the COI, ITS2 and 28S regions, respectively, nearly reach interspecific minima for the family. However, these intraspecific maxima are associated with the Lizard Island clade, which, as discussed above, we consider as divergent but still representative of that species. The minimum number of bp differences between a COI mtDNA gene sequence of G. kyphosi and that of another species is 65 (13.7%). This difference is found when comparing sequences of G. kyphosi and G. graboides from the same locality, Lizard Island. Within each locality, intraspecific variation for these clades did not exceed 3 bp for any gene region sequenced, and the clades representing these two species were never resolved as sister in phylogenetic analyses. Curiously, G. kyphosi and G. graboides are the most divergent species pair when comparing sequences of the ITS2 and 28S rDNA gene regions, with minimum differences of 16 bp (3.5%) and 18 bp (1.8%), respectively. Because G. kyphosi and G. graboides occur in sympatry at Lizard Island and share Kyphosus cinerascens and Echinolittorina vidua as hosts, the genetic distances between these clades imply a real barrier to gene flow, fitting both the biological and phylogenetic species concepts (Knowlton & Weigt, 1997; Knowlton, 2000). This suggests that these levels of sequence divergence are representative of a species-level difference for populations occurring in sympatry and that, despite only subtle morphological differences, G. graboides can be confidently considered distinct from G. kyphosi.
Gorgocephalus euryaleae is also similar morphologically to G. kyphosi, but specimens of these two species were not collected in sympatry, complicating our ability to differentiate them on a molecular basis. All phylogenetic analyses resolved the G. euryaleae clade as sister to G. kyphosi, and minimum genetic differences between these species, at 66 bp (14%), 4 bp (0.9%) and 5 bp (0.5%) in the COI, ITS2 and 28S gene regions, respectively, only just exceed the maximum intraspecific variation observed for G. kyphosi. This morphological and molecular similarity across range might be enough justification to consider G. euryaleae as conspecific with G. kyphosi. However, there seems no clear geographic or ecological barrier to gene flow that might be used to rationalize these clades as divergent lineages of the same species. The two closest collection localities for these species are Point Peron, Western Australia (for G. euryaleae) and Point Riley, South Australia (for G. kyphosi). Although these two localities are separated by the Great Australian Bight, a large open bay with high levels of endemism (Edyvane, 2000), Kyphosus sydneyanus, which hosts both G. euryaleae and G. kyphosi, ranges across the entire region (Knudsen & Clements, 2013b). A number of littorinid species also range across the Great Australian Bight, including Austrolittorina unifasciata and Bembicium auratum, and although there are gaps in the distribution maps of some littorinid species across the region, these gaps have been attributed to a lack of collections due to the remoteness of much of the coastline, rather than lack of suitable habitat and absence of the gastropods (Reid, 1988; Reid & Williams, 2004). Furthermore, the absolute geographic distance (in terms of kilometres of coastline) between Point Peron and Point Riley is around the same as the distance between Point Riley and Moreton Bay, where the next closest population of G. kyphosi was sampled. Sequences of G. kyphosi from Point Riley and Moreton Bay differ by a maximum of 7 bp (1.5%) and 1 bp (0.2%) in the COI and ITS2 gene regions, respectively, and 28S sequences were identical between these localities. Thus, if G. euryaleae is conspecific with G. kyphosi, it is not clear why the Western Australian population is genetically distinct from the Point Riley population. We predict further collections are likely to show that these species co-occur.
Despite a linear separation of approximately 7000 km, COI, ITS2 and 28S sequences generated for specimens of Gorgocephalus euryaleae differed by a maximum of 11 bp (2.3%) 1 bp (0.2%) and 2 bp (0.2%) between South Africa and Western Australia. If we were to consider G. euryaleae as conspecific with G. kyphosi, this would suggest greater connectivity across the Indian Ocean than across the Great Australian Bight. In addition, sequences from the two most geographically disparate populations of G. kyphosi (from Point Riley, South Australia and Rangiroa, French Polynesia) are more similar to each other than sequences from Point Riley are to those of G. euryaleae from Western Australia. It is possible that the genetic distinctiveness that we have detected in Western Australia is the result of an isolation phenomenon similar to that which we have observed at Lizard Island. However, sequences from putative specimens of G. kyphosi from Lizard Island are still more similar to sequences of G. kyphosi from Point Riley than sequences from Point Riley are to sequences from Western Australia. Future sampling from additional localities, especially northern Australia, may resolve G. kyphosi and G. euryaleae as conspecific, but at present it seems best to consider them as distinct species.
This study is the first to provide molecular sequence data for members of a marine digenean lineage from localities on the eastern (Sodwana Bay, South Africa) and western (Rangiroa, French Polynesia) extremes of the IWP. As such, the patterns of genetic divergence observed for species of the Gorgocephalidae may inform future interpretations of species boundaries in other IWP digenean lineages. In short, it is apparently possible for the same parasite species to occur in the entire region, just as are many fishes. However, extrapolation of patterns observed in the present study to other systems requires caution, as many variables (e.g. larval dispersal, host vagility, etc.) are likely to affect the distribution and genetic connectivity of parasites (e.g. Barrett et al., 2008; Blasco-Costa et al., 2012; Grégoir et al., 2015).
The present study also highlights the value of a multilocus molecular approach, especially the inclusion of independent markers with different rates of evolution. Most molecular taxonomic studies of digeneans to date have employed only one or two markers, primarily ITS2 and/or 28S rDNA (Blasco-Costa et al., 2016; Pérez-Ponce de León & Hernández-Mena, 2019). However, inclusion of COI sequences is becoming more common in studies of IWP digeneans (e.g. McNamara et al., 2014; O’Dwyer et al., 2015; Wee et al., 2017; Bray et al., 2018b; Bennett & Presswell, 2019; Corner et al., 2020; Presswell & Bennett, 2020; Wee et al., 2020). In the present study, phylogenetic analyses of the three molecular markers utilized provided similar results, but the two nuclear genes (ITS2 and 28S rDNA) were highly conserved and failed to resolve all specimens consistent with G. yaaji in a monophyletic clade. Furthermore, a number of studies of other IWP trematode lineages (e.g. Trieu et al., 2015; Bray et al., 2018b; Huston et al., 2018a) have reported fewer bp differences in sequences between well-defined species than we have observed within Gorgocephalus kyphosi and G. yaaji. Inclusion of COI sequences in the present study augmented patterns revealed by ITS2 and 28S rDNA gene sequences and improved our ability to recognize intraspecific variation, which we might previously have interpreted as interspecific variation. Lastly, the molecular results of this study emphasize that, at present, sequence data alone are not enough to understand digenean biodiversity in the IWP; detailed morphological study is still vital. All three molecular markers employed for the study of the Gorgocephalidae had the potential to inflate species richness if interpreted uncritically.
Drivers of speciation in the Gorgocephalidae
The forces driving speciation in the Gorgocephalidae are not clear. Key to exploring these processes in this family is the stenoxenic host-specificity (sensuMiller et al., 2011) exhibited by its members. No gorgocephalid has yet been reported from a non-kyphosid fish, but at least three species are capable of parasitizing multiple kyphosids. Intermediate hosts are now known for three of five gorgocephalids, and in all cases they are littorinid gastropods. Furthermore, Gorgocephalus kyphosi and G. yaaji are capable of parasitizing at least three species of littorinid each. The overall pattern suggests a ubiquitous two-host life-cycle with broad host-specificity at both the definitive and intermediate host level, but with restriction to fishes of the Kyphosidae and gastropods of the Littorinidae, respectively. Thus, although host-switching is thought to be one of the major drivers of speciation in the Digenea (Cribb et al., 2001a, b), the modern-day stenoxenic host-specificity exhibited by gorgocephalids suggests that host-switching has not been the defining force in diversification of the lineage. There does appear to be some patterns of divergence based on geographic isolation (i.e. Lizard Island populations), but the specifics regarding the origin of such isolation remains unclear. A few additional insights can be gained from a more ecological perspective. Gorgocephalus yaaji is resolved as sister to the other three gorgocephalids represented in all phylogenetic analyses, with the exception of analyses of the ITS2 single-gene dataset. This sister relationship makes ecological sense in that G. yaaji is found primarily in the upper intestine, whereas all other gorgocephalids are restricted to the pyloric caeca. The divergence of these two clades seems likely to have been driven by niche-partitioning. However, the drivers of speciation in the pyloric-caeca inhabiting gorgocephalids remain unexplained.
SUPPORTING INFORMATION
Additional Supporting Information may be found in the online version of this article at the publisher’s web-site.
Table S1. Summary of littorinid collections performed between 2016 and 2018 and associated gorgocephalid infections.
Table S2. Collection information for gorgocephalid specimens used for the generation of molecular sequence data, with associated GenBank accession numbers. A single asterisk (*) next to a GenBank sequence indicates that the sequence was obtained from genomic DNA extracted for the study of Huston et al. (2016); associated GenBank accessions previously uploaded are included for reference. Two asterisks (**) indicates COI sequences obtained using the primers and cycling conditions from O’Dwyer et al. (2015) (see methods’ section).
Table S3. Nucleotide substitution models for phylogenetic analyses selected using PartitionFinder v.2.1.1, employing the Akaike’s information criterion (AICc), the greedy algorithm and PhyML (Guindon et al., 2010; Lanfear et al., 2012; Lanfear et al., 2017).
Table S4. Summary of kyphosid collections performed between 2015 and 2018 and respective gorgocephalid infections detected.
Table S5. Inter- and intraspecific pairwise comparisons of COI mtDNA, ITS2 and 28S rDNA gene-sequences for four species of Gorgocephalus. Collection site abbreviations: PR, Point Riley, Yorke Peninsula, South Australia; MB, North Stradbroke Island, Moreton Bay, Queensland, Australia (specimens collected from Amity Point and Dunwich, North Stradbroke Island); LI, Lizard Island, Great Barrier Reef, Queensland, Australia; KI, Kioloa, New South Wales, Australia; WA, off Rockingham, Western Australia (specimens collected from off Point Peron and from off Rottnest Island); RA, Rangiroa atoll, Tuamotu Islands, French Polynesia; SB, Sodwana Bay, KwaZulu-Natal, South Africa.
[Version of record, published online 10 March 2021; http://zoobank.org/ urn:lsid:zoobank.org:pub:AAA956A8- 14F7-49E4-888F-072FAC7D3826]
ACKNOWLEDGEMENTS
We thank the staff of the Lizard Island (Australian Museum), Heron Island and Moreton Bay (University of Queensland) research stations, Australia, and the staff of the Centre for Insular Research and Observatory of the Environment (CRIOBE), in Moorea, French Polynesia for their support of our collecting efforts. We thank Drs Rod Bray, Storm Martin, Russell Yong, Nick Wee and all students of the Marine Parasitology Laboratory (University of Queensland) for their assistance in collecting fish and digeneans over the many years of this project. We thank Ms Adri Joubert and Dr Olena Kudlai, both from the Water Research Group (North-West University), for logistics and assistance during the fieldwork in South Africa. DCH thanks Dr Nick Kalfas for allowing him to dissect fish in his garage and to couch crash while collecting in South Australia in 2017, and Dr Marty Deveney for providing laboratory space and advice during collections in South Australia in 2016. We thank the Direction of Marine Resources from French Polynesia for its help during the field trip in Rangiroa. The Western Australian Department of Fisheries–Fish Health Laboratory team kindly offered laboratory space and facilities during the fieldwork conducted in Western Australia. The authors acknowledge the facilities, and the scientific and technical assistance, of the Australian Microscopy & Microanalysis Research Facility at the Centre for Microscopy and Microanalysis, The University of Queensland. Lastly, we thank our reviewers for their insightful comments, which improved the content of this manuscript. This is contribution number 478 from the North-West University Water Research Group.
Funding
This study was funded in part by grants to DCH from the PADI Foundation (USA), the University of Queensland (Australia), Holsworth Wildlife Research Endowment (Australia), Linnean Society of New South Wales (Australia) and the Systematics Research Fund (Systematics Association in partnership with the Linnean Society of London) (UK). THC and SCC are supported by an Australian Biological Resources Study National Taxonomy Research Grant (RF19-37). Fieldwork in South Africa was funded in part by the National Research Foundation (NRF) of South Africa (NRF project CPRR160429163437 grant 105979, NJ Smit, PI). Opinions expressed, and conclusions arrived at, are those of the authors and are not necessarily those of the NRF.
Ethics
This study was conducted in compliance with all institutional, national and international guidelines on the care and use of animals.
REFERENCES