-
PDF
- Split View
-
Views
-
Cite
Cite
Mingjun Sun, Weitao Shen, Xinxin Guo, Yinghao Liao, Yang Huang, Mohan Hu, Ping Ye, Ran Liu, A critical review of advances in tumor metabolism abnormalities induced by nitrosamine disinfection by-products in drinking water, Toxicological Sciences, Volume 199, Issue 1, May 2024, Pages 12–28, https://doi.org/10.1093/toxsci/kfae012
- Share Icon Share
Abstract
Intensified sanitation practices amid the recent severe acute respiratory syndrome coronavirus 2 (SARS-CoV-2) outbreak might result in the increased release of chloramine disinfectants into surface water, significantly promoting the formation of nitrosamine disinfection by-products (DBPs) in drinking water. Unfortunately, these nitrosamine DBPs exhibit significant genotoxic, carcinogenic, and mutagenic properties, whereas chlorinating disinfectants remain in global practice. The current review provides valuable insights into the occurrence, identification, contamination status, exposure limits, and toxicity of the new unregulated disinfection by-products (nitrosamine DBPs) in drinking water. As a result, concentrations of nitrosamine DBPs far exceed allowable limits in drinking water, and prolonged exposure has the potential to cause metabolic disorders, a critical step in tumor initiation and progression. Importantly, based on recent research, we have concluded the role of nitrosamines DBPs in different metabolic pathways. Remarkably, nitrosamine DBPs can induce chronic inflammation and initiate tumors by activating sphingolipid and polyunsaturated fatty acid metabolism. Regarding amino acid and nucleotide metabolism, nitrosamine DBPs can inhibit tryptophan metabolism and de novo nucleotide synthesis. Moreover, inhibition of de novo nucleotide synthesis fails to repair DNA damage induced by nitrosamines. Additionally, the accumulation of lactate induced by nitrosamine DBPs may act as a pivotal signaling molecule in communication within the tumor microenvironment. However, with the advancement of tumor metabolomics, understanding the role of nitrosamine DBPs in causing cancer by inducing metabolic abnormalities significantly lags behind, and specific mechanisms of toxic effects are not clearly defined. Urgently, further studies exploring this promising area are needed.
Drinking water disinfectants can deactivate pathogens, thus preventing waterborne diseases like typhoid and cholera. However, these disinfectants can also interact with specific precursors (eg, dissolved carbon and organic nitrogen) in water (Hashemi et al., 2022; Hrudey 2009; Zeng et al., 2016), leading to the formation of disinfection by-products (DBPs) that pose a potential threat to human health (Jurado-Sánchez et al., 2012; Richardson and Kimura 2020). Currently, approximately 600–700 DBPs have been identified, with only a small portion quantified in drinking water (Krasner et al., 2006). Regulators have set specific limits for the levels of DBPs allowed in drinking water. The WHO Guidelines for Drinking Water Quality has set regulations for the concentration of 2 haloacetonitriles, and dimethylnitrosamine (NDMA), 3 haloacetic acids (HAAs) (monochloro-, dichloro-, trichloro-, monobromo-, dibromoacetic acid) and inorganic DBPs, and 4 trihalomethanes (THMs) (chloroform, bromoform, bromodichloromethane, chlorodibromomethane) (WHO, 2011). Similarly, in China, a total of 15 DBPs were regulated. Nevertheless, certain new unregulated disinfection by-products (UDBPs) exhibit higher toxicity and health risks even at very low concentrations compared with regulated disinfection by-products (RDBPs), like nitrosamines (NAs), iodinated THMs, and halonitromethanes (HNMs) (Krasner 2009).
NAs, as UDBPs containing nitrogen, in drinking water have gained significant attention recently because of their high carcinogenicity, frequent presence, and potential regulation (Bei et al., 2016). Presently, 9 types of nitrosamines DBPs have been identified in drinking water (Figure 1), including N-nitrosodiphenylamine (NDPhA), N-nitrosopyrrolidine (NPYR), N-Nitrosopiperidine (NPIP), N-nitrosodiethylamine (NDEA), N-nitrosodipropylamine (NDPA), N-nitrosomethylethylamine (NMEA), N-nitrosomorpholine (NMOR), N-nitrosodibutylamine (NDBA), and NDMA (Shah and Mitch 2012; Wang et al., 2022; Zhao et al., 2020). Over the past few decades, numerous epidemiological studies have uncovered a link between prolonged consumption of water containing nitrosamine DBPs and an elevated risk of gastrointestinal cancers (eg, esophageal cancer [Wang et al., 2022; Zhao et al., 2020], liver cancer [Cai et al., 2022], gastric cancer [Chen 1989], colorectal cancer [Luo et al., 2022], and bladder cancer [SeyyedSalehi et al., 2021]). However, cancer development is influenced by a blend of environmental risk factors and genetic variations. As a result, the potential carcinogenic mechanisms of nitrosamine DBPs remain undeciphered.
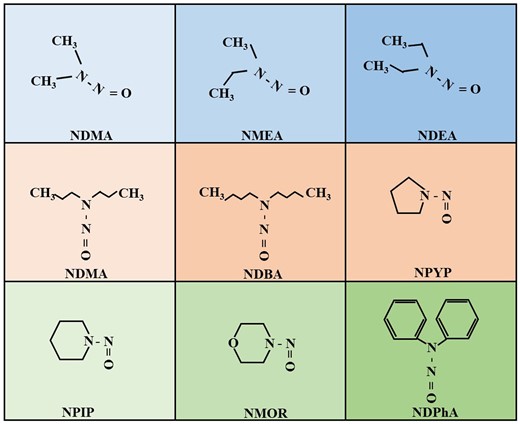
Chemical structure of 9 nitrosamines DBPs (created in BioRender.com).
Transcriptional reprogramming of cellular metabolism is a distinctive trait observed in both cancer cells and their neighboring cells within the tumor microenvironment (TME). Tumor cells alter their flux through various metabolic pathways to meet the increased bioenergetic and biosynthetic demands and to mitigate the oxidative stress required for tumor cell proliferation and survival (Martínez-Reyes and Chandel 2021). Recently, the untargeted metabolomics using liquid chromatography-tandem mass spectrometry (LC-MS/MS) technology has identified the dynamic metabolic response in crucial intermediate biochemical pathways of tumors at various stages, from initiation to invasion, providing a new direction for comprehensively interpreting the molecular mechanism of tumor occurrence (Luo et al., 2022; Yang et al., 2022; Yu et al., 2021a). Simultaneously, the gas chromatography-mass spectrometer (GC-MS) and LC-MS/MS also offer a novel platform for detecting trace substances (DBPs and neonicotinoid insecticides) in human urine and serum samples (Luo et al., 2022; Ueyama et al., 2020; Zhao et al., 2020). Yet, the relationship between nitrosamine DBPs and metabolic disorders during tumor initiation and progression and mechanism of action have not been clearly described so far. Certain nitrosamine carcinogens can cause disruptions in TRP metabolism, nicotinate and nicotinamide (NAM) metabolism, and sphingolipid metabolism during ESCC initiation (Zhao et al., 2022a). In colorectal cancer, hypoxanthine and xanthine metabolic pathways were linked to alterations in volatile nitrosamines (VNAs) (Luo et al., 2022). In addition, the study of oxidative stress and metabolism-related proteins (eg, CPT1, ACBP, CD36, ACAA2, and PPARα) expression mediated by nitrosamines DBPs has also been published (Zhang et al., 2022b). This contributes to a better understanding of the role of nitrosamines DBPs in tumor evolution.
Therefore, this article briefly analyzes the generation, identification, exposure limits, and distribution status of nitrosamine DBPs. Primarily, we summarize the carcinogenic effects and mechanisms of nitrosamine DBPs. it outlines the link between nitrosamine DBPs and the metabolic diversity within the TME that has been a subject of recent research, encompassing lipid metabolism, amino acid and derivative metabolism, carbohydrate metabolism, nucleotide metabolism, and more. Lastly, we identify the need for further research in this area, which might offer new insights into the investigation of chemical-induced metabolic heterogeneity in carcinogenesis.
Drinking water nitrosamines DBPs contamination status
Formation of nitrosamines DBPs
According to the WHO report, approximately 30% of underdeveloped and developing countries lack access to high-quality drinking water sources, leading to over 80% of diseases in the region (Adelodun et al., 2021; Kali et al., 2021). In Pakistan, 20% of the population has access to safe drinking water, but 30% of all diseases and 40% of all deaths are linked to unsafe drinking water (Daud et al., 2017). Therefore, disinfection is a crucial measure in treating drinking water for public supply. Presently, common methods for disinfecting drinking water encompass ozone, peracetic acid chlorine, sodium hypochlorite, ultraviolet radiations, chloramine, and chlorine dioxide (Du et al., 2021; Lanrewaju et al., 2022; Srivastav et al., 2020). However, some disinfectants can generate DBPs by interacting with natural organic matter in water (eg, humic acid, fulvic acid, carboxylic acid, sugars, amino acids, etc.), as well as organic matter (containing carboxyl, aromatic, amino and hydroxyl groups) and inorganic substances (bromides, iodides) and other precursors, and bacteria and viruses will also react with disinfectants to form DBPs (Liu and Li 2010).
Chlorination is a traditional and widely favored method, although it can produce a significant amount of THMs and HAAs (Krasner et al., 2006). However, to comply with for THMs and HAAs in drinking water, more water treatment plants in the United States, Canada, and the United Kingdom are shifting from chlorine to chloramine as a secondary disinfectant (Goslan et al., 2009). It has been shown that the transition from chlorine to chloramines reduces the formation of THMs and trihalogenated HAAs more effectively than dihalogenated HAAs (Diehl et al., 2000). Surprisingly, the concentration of specific nitrogenous DBPs increased, particularly NDMA (Mitch et al., 2003). Therefore, chloramine disinfection is the primary pathway for the generation of nitrosamine DBPs. Presently, research on the formation mechanisms of nitrosamine DBPs predominantly centers on NDMA, including chloramine disinfection generation pathway (Shah and Mitch 2012), O3 oxidation generation pathway, and free-chlorine-enhanced nitrosation reaction pathway (Sgroi et al., 2018) (Figure 2).
The chloramine disinfection generation pathway (Figure 2A): Chloramine disinfection is one of the primary pathways for the formation of nitrosamines DBPs. In cases where the water source contains high concentrations of organic amine precursors (eg, dimethylamine [DMA]), NH2Cl reacts with them, forming the intermediate product unsymmetrical dimethylhydrazine (UDMH). Subsequently, NH2Cl oxidizes UDMH to produce NH4+, NDMA, and Cl− (Choi and Valentine 2002; Mitch and Sedlak 2002). However, NDMA generated through the reaction of NHCl2 with organic amines is significantly higher than that from NH2Cl, leading to the majority of NDMA in drinking water being created by NHCl2 (Schreiber and Mitch 2006b). Briefly, NHCl2 with DMA by nucleophilic substitution to create chlorinated UDMH, which occurs at a rate 2–3 orders of magnitude higher than the rate of UDMH formation by NH2Cl and DMA. Further, UDMH can interact with dissolved oxygen (O2) to produce NDMA, and also with NHCl2 to generate other products, yet the reaction with dissolved oxygen is marginally faster (Schreiber and Mitch 2006a). Some studies have found that the pH of untreated water and the types of organic amine precursors significantly influence NDMA formation during chloramine disinfection. As McCurry et al. discovered that low pH (approximately 5.5) promoted the conversion of NH2Cl to NHCl2 and increased the formation of NDMA with increasing pH 8 (McCurry et al., 2017). Meanwhile, Tom et al. revealed that the NDMA productivity from DMA in chloramine disinfection ranged from 0.5% to 2.7%, whereas the NDMA productivity from ranitidine, containing a tertiary amine group, reached levels between 62.9% and 89.9% (Seid et al., 2018). Generally speaking, tertiary amines and quaternary amines can convert into primary amines, producing nitrosamines DBPs during chlorine or chloramine disinfection. but some substances containing tertiary amine functional groups (such as ranitidine) can directly generate nitrosamines without generating intermediate products of primary amines (Sgroi et al., 2018). Furthermore, bromine ions can facilitate NDMA formation by reacting with NH2Cl to generate brominated oxidants (eg, NHBrCl) and the intermediary product brominated UDMH, primarily occurring in weakly alkaline conditions at pH 8–9 (Le Roux et al., 2012; Luh and Mariñas 2012).
Nitrosation reaction pathway (Figure 2B): The previous studies primarily concentrated on discovering effective approaches for removing NDMA and investigating NDMA degradation pathways, yet little attention has been given to the destinies of the degradation products. DMA and NO2− represent the primary byproducts of NDMA degradation while also serving as precursors for NDMA formation (Xu et al., 2009). In chlorination disinfection, free chlorine interacted with NO2− to create ClNO2, leading to the subsequent formation of N2O4. N2O4 directly interacted with DMA as a nitrosating agent, generating NDMA. However, the NDMA produced through this path (approximately 0.0007%) was notably lower than that via the chloramine route (approximately 0.05%) (Shah and Mitch 2012).
Ozonation (O3) oxidation generation pathway: The O3, known for its potent oxidizing capability, was initially indicated to decrease the potential for NDMA formation during subsequent chloramination (Zou et al., 2018). Nevertheless, recent observations have shown NDMA can be directly generated during O treatment of water containing typical precursors (with hydrazine groups) (Gao et al., 2021; Gerrity et al., 2015; Kosaka et al., 2014; Pisarenko et al., 2015). For instance, the NDMA molar conversion percentages of UDMH in ozone oxidation was as high as 80%, and the NDMA conversion rate of N,N-dimethylsulfamide was 52%, indicating that the role of ozone oxidation in the generation of NDMA should not be neglected (Schmidt and Brauch 2008). Under neutral and alkaline conditions, 2 pathways contribute to NA generation during ozone oxidation. (1) Through the nitrosation reaction pathway, utilizing the oxidation product N2O4 of NO2− as a nitrosating agent (Figure 2B). (2) The reaction of secondary amine precursors with hydroxylamine (NH2OH) to form UDMH, subsequently oxidized to produce the corresponding nitrosamines (Figure 2C) (Yang et al., 2009).
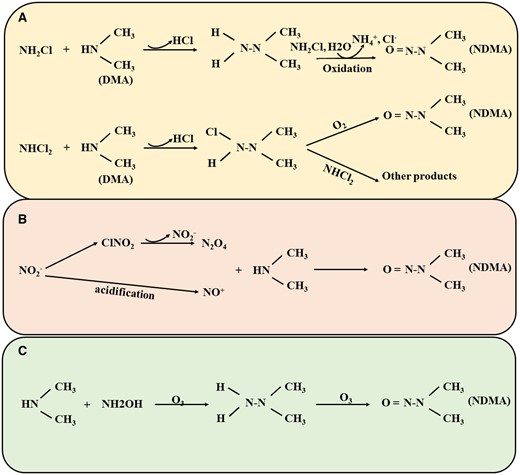
Identification of nitrosamines DBPs
Nitrosamines, a set of emerging nitrogenous DBPs, were present in drinking water at ng/l levels. Hence, achieving accurate measurements of nitrosamines DBPs at such low trace levels in water samples presents a challenging task. Current techniques such as solid-phase extraction and liquid-liquid extraction employed in sample pretreatment do partially fulfill the requirements for precise determination of nitrosamines DBPs, utilizing materials like activated carbon, coconut charcoal, and hydrophilic-lipophilic balance-type sorbents (Zhang et al., 2020b). However, most of these methods necessitate significant amounts of organic solvents, are time-intensive, and have revealed that activated carbon can catalyze the transformation of secondary amines into nitrosamines in the presence of oxygen (Padhye et al., 2010, 2011). Therefore, a major bottleneck is how to efficiently and simultaneously extract a trace level of nitrosamines from drinking water.
In general, nitrosamines DBPs exhibit high solubility in water and a relatively low octanol-water partition coefficient (KOW) (Table 1). Owing to these special physicochemical properties, specific challenges arise during their preconcentration and extraction prior to analysis. Currently, their extraction and preconcentration methods rely solely on sorbent-based techniques. A study by Taguchi et al. (1994) suggested that that Ambersorb 572 was effective for nitrosamines; however, Rohm & Haas discontinued the production of Ambersorb 572 in 2007 due to the necessity for more than 2.5 g of adsorbent and a long extraction time (>60 min) (Padhye et al., 2010). In 2012, Fu et al. demonstrated the use of dispersive micro-solid-phase extraction with carboxen sorbents for extracting 5 NAs in swimming pool water samples, achieving quantitation limits of <0.9 ng/l and an average extraction recovery ranging from 62% to 109% (Fu et al., 2012). Recently, Zhang et al. (2020b) reported that the use of dispersive solid-phase extraction employing clover-shaped nano-titania functionalized covalent organic framework sorbents to extract 8 N-nitrosamines in drinking water for subsequent determination via LC-MS/MS. This method achieved relatively low detection limits of 0.13–2.45 ng/l and satisfactory recoveries of 88.6%–105.5%. The introduction of these new materials offers a means for accurately detecting trace nitrosamines in drinking water. Additionally, nitrosamines, as volatile DBPs, are identified using gas chromatography-nitrogen phosphorus detector (GC-NPD) (Hu et al., 2008) and chemiluminescent nitrogen detector (Tomkins and Griest 1996) after pretreatment, with detection limits at the μg/l level, which are insufficient for trace detection. The recent advancements in gas chromatography coupled to tandem mass spectrometry (GC-MS/MS) and gas chromatography coupled with high-resolution mass spectrometry (GC-HRMS) have addressed the limitations of traditional analysis, providing wide linear range, high sensitivity and specificity, and low detection limits down to ng/l, making them ideal methods for detecting nitrosamines in water.
Compound names . | Molecular weight (g/mol) . | Solubility (mg/l) . | KOW . | Unit carcinogenic risk (10−6 ng/l) . |
---|---|---|---|---|
NDBA | 158.24 | 1.27 × 103 | 2.54 | 6.0 |
NDEA | 102.14 | 10.6 × 104 | 0.52 | 0.2 |
NDMA | 74.08 | 1.0 × 105 | −0.57 | 0.7 |
NDPA | 130.19 | 1.3 × 104 | 1.54 | 5.0 |
NDPhA | 198.22 | 35 | 3.16 | 7000 |
NMEA | 88.11 | 3 × 105 | 0.01 | 2.0 |
NMOR | 116.12 | 8.62 × 105 | −0.59 | — |
NPYP | 100.10 | 1.0 × 106 | −0.09 | 20 |
NPIP | 114.15 | 7.65 × 104 | 0.44 | — |
Compound names . | Molecular weight (g/mol) . | Solubility (mg/l) . | KOW . | Unit carcinogenic risk (10−6 ng/l) . |
---|---|---|---|---|
NDBA | 158.24 | 1.27 × 103 | 2.54 | 6.0 |
NDEA | 102.14 | 10.6 × 104 | 0.52 | 0.2 |
NDMA | 74.08 | 1.0 × 105 | −0.57 | 0.7 |
NDPA | 130.19 | 1.3 × 104 | 1.54 | 5.0 |
NDPhA | 198.22 | 35 | 3.16 | 7000 |
NMEA | 88.11 | 3 × 105 | 0.01 | 2.0 |
NMOR | 116.12 | 8.62 × 105 | −0.59 | — |
NPYP | 100.10 | 1.0 × 106 | −0.09 | 20 |
NPIP | 114.15 | 7.65 × 104 | 0.44 | — |
Compound names . | Molecular weight (g/mol) . | Solubility (mg/l) . | KOW . | Unit carcinogenic risk (10−6 ng/l) . |
---|---|---|---|---|
NDBA | 158.24 | 1.27 × 103 | 2.54 | 6.0 |
NDEA | 102.14 | 10.6 × 104 | 0.52 | 0.2 |
NDMA | 74.08 | 1.0 × 105 | −0.57 | 0.7 |
NDPA | 130.19 | 1.3 × 104 | 1.54 | 5.0 |
NDPhA | 198.22 | 35 | 3.16 | 7000 |
NMEA | 88.11 | 3 × 105 | 0.01 | 2.0 |
NMOR | 116.12 | 8.62 × 105 | −0.59 | — |
NPYP | 100.10 | 1.0 × 106 | −0.09 | 20 |
NPIP | 114.15 | 7.65 × 104 | 0.44 | — |
Compound names . | Molecular weight (g/mol) . | Solubility (mg/l) . | KOW . | Unit carcinogenic risk (10−6 ng/l) . |
---|---|---|---|---|
NDBA | 158.24 | 1.27 × 103 | 2.54 | 6.0 |
NDEA | 102.14 | 10.6 × 104 | 0.52 | 0.2 |
NDMA | 74.08 | 1.0 × 105 | −0.57 | 0.7 |
NDPA | 130.19 | 1.3 × 104 | 1.54 | 5.0 |
NDPhA | 198.22 | 35 | 3.16 | 7000 |
NMEA | 88.11 | 3 × 105 | 0.01 | 2.0 |
NMOR | 116.12 | 8.62 × 105 | −0.59 | — |
NPYP | 100.10 | 1.0 × 106 | −0.09 | 20 |
NPIP | 114.15 | 7.65 × 104 | 0.44 | — |
Exposure levels and limit standards of nitrosamines DBPs
Enhanced sanitation measures during the recent severe acute respiratory syndrome coronavirus 2 (SARS-CoV-2) pandemic may result in the release of chlorine-based disinfectants into surface water, potentially triggering the formation of nitrosamines DBPs in domestic wastewater and drinking water (Li et al., 2021c; Wang et al., 2021). Data from previous research conducted in Wuhan revealed that total nitrosamines concentrations in rivers ranged from 1.6 to 62.4 ng/l before the SARS-CoV-2 pandemic, whereas in the 2022 study, nitrosamines DBPs concentrations varied from 23.1 to 97.4 ng/l (Wang et al., 2021). Elevated nitrosamines DBPs levels may have 2 primary sources: (1) Nitrosamines DBPs could form when residual chlorine is present in surface water; (2) wastewater treatment plant effluents and industrial/domestic wastewaters containing nitrosamines DBPs may be directly discharged into surface water. Surprisingly, Spearman’s rank correlation analysis revealed a significant positive correlation between NDEA and dissolved organic nitrogen (r = 0.8, p < .05) (Wang et al., 2021), indicating that higher dissolved organic nitrogen levels lead to increased NDEA concentrations, underscoring potential health risks in surface water.
The potential carcinogenic of nitrosamines was reported by Magee in 1956 (Magee 1956), and further in 1989, when NDMA was first detected in drinking water in Ontario, Canada (Taguchi et al., 1994), extensive research on nitrosamines DBPs began in the United States, Spain, Japan, the United Kingdom, China, and Australia. In 1998, around 400 μg/l of NDMA was identified in California’s drinking water, leading to the establishment of concentration limits for NDMA, NDEA, and NDPA in drinking water at 10 ng/l (Ding et al., 2019). However, the occurrence of nitrosamines in Chinese drinking water was relatively high due to prevalent chloramination, wastewater recycling, and effective chloramination from high ammonia concentrations in the source water. A recent survey in central China detected NDMA, NDEA, NPIP, and NDBA in influent water, treated water, and tap water, with the highest detection rate for NDMA (93.1% in tap water and 100% in influent water and treated water), followed by NDEA (66.7%–83.3%) and NPIP (58.3%–66.7%) (Luo et al., 2020). In Jiangsu, eastern China, the total nitrosamines contamination in drinking water was 135.14 ng/l, including NDPA (34.91 ng/l), NDBA (16.47 ng/l), NMor (32.64 ng/l), and NDEA (36.53 ng/l). However, to date, only Shanghai and Shenzhen in China have established local NDMA criteria (100 ng/l) (Li et al., 2021a). Despite matching the WHO’s standard, this level may not effectively reduce the health risks related to NDMA in drinking water, as drinking water accounts for 45%–52% of the total exogenous NDMA intake. Outside China, NDMA has been more frequently identified in Australia, where 75% of chloraminated waters contained NDMA, with 37% exceeding concentrations of >10 ng/l (Krasner et al., 2013). In Japan, NDMA was found in raw waters up to 4.3 ng/l, but concentrations in finished drinking water were generally lower due to the exclusive use of chlorination. However, NDMA reached 10 ng/l in an ozone plant’s effluent due to anti-yellowing agents, which were identified as NDMA precursors during ozonation. NDMA was detected in distribution systems in Singapore but at relatively low concentrations (1.1–6.4 ng/l). Thus, only a small portion of the country requires investment to mitigate the threat posed by nitrosamine DBPs in drinking water. Overall, safeguarding water sources, updating treatment processes, and optimizing disinfection are crucial to reduce the formation of nitrosamines DBPs. In China, we recommend setting the limit for nitrosamine DBPs in drinking water at 40 ng/l based on water quality safety and feasibility.
Toxic effects of nitrosamines DBPs
Genotoxicity of nitrosamines DBPs
NAs are well-recognized environmental toxins that, through P450-catalyzed α-hydroxylation in liver microsomes, can be metabolized into unstable genotoxic agents. The available data indicated that NDMA and NDEA as the most thoroughly studied genotoxic substances primarily metabolized and activated by CYP2E1 (Johnson et al., 2021). With the increase of the carbon numbers of the alkyl chains, however, NDEA, NDPA, NMOR, NPIP, NPYR, and NDBA are metabolized by CYP2A6 and CYP1A1, respectively (Liu et al., 2012). But, aside from NDMA, there is less extensive research on the genotoxicity of other compounds. Notably, NPYR and NDMA were found to be mutagenic in Salmonella in the presence of S9, indicating that nitrosamines DBPs act as indirect mutagenic agents. Additionally, it’s essential to note that CYP2E1 gene polymorphism can affect the genotoxicity of nitrosamines DBPs, for instance, 4-(methylnitrosamino)-1-(3-pyridyl)-1-butanone induced significantly higher chromosome aberration levels in cells with the CYP2E1 WT/*5B genotype compared with those with the CYP2E1 WT/WT (Abdel-Rahman et al., 2000). These findings underscore the crucial role of polymorphic metabolizing genes in influencing the genotoxic responses to nitrosamines DBPs.
Early data regarding the genotoxicity of nitrosamines DBPs revealed that NAs metabolites can cause chromosomal aberrations, micronuclei, gene mutations, and DNA damage in both in vitro and in vivo. For instance, the methyl radical produced during NAs metabolic activation could cause alkylation at the O6 position of guanine (Arimoto-Kobayashi et al., 1997) and the O4 position of thymidine (Verna et al., 1996). Comet assay results in cultured human cells demonstrated significant oxidative DNA damage caused by NPYR and NDMA at effective concentrations of 5 and 27 mM, respectively. Notably, NPYR showed greater genotoxic effects than NDMA (Arranz et al., 2007). Liviac et al. found that NDMA did not significantly increase the frequency of micronuclei in binucleated cells, contrary to the earlier report by Valentin-Severin et al. (2003) in HepG2 cells. The discrepancy might be attributed to the different cell lines used in these studies. In Serovar Typhimurium, the rank order of the nitrosamines DBPs in concentration response curves and mutagenic potency values was NDMA > NPIP > NMOR > NPYR, with NDPhA being nonmutagenic (Wagner et al., 2012). Most significantly, NAs mixtures demonstrated greater genotoxic potential than individual compounds, for instance, compared with single exposure, the NAs-mixture containing NDMA, NDEA, and NMEA doubled the revertant in the TA98 and TA100 S. typhimurium strains, leading to increased DNA double-strand breaks and micronuclear frequency in the embryonic mouse fibroblast cell line NIH3T3 (Wang et al., 2017); exposure to the mixture of 3 NAs (NDMA, NDPA, and NMEA) at 1000-fold actual drinking-water concentration resulted in a doubling of revertant in the TA100 S. typhimurium strains, DNA, and chromosome damage in HepG2 cells (Dong et al., 2022). Surprisingly, subjects consuming well water containing 25 mg of nitrate/l had higher HPRT mutant frequencies in their peripheral lymphocytes than those consuming tap water containing 0.2 or 17.5 mg of nitrate/l. Additionally, NPYP was found in the urine of 18 out of 22 subjects (van Maanen et al., 1996). This study suggests that drinking water with high nitrate levels might pose a mutagenic risk to humans due to the endogenous formation of carcinogenic N-nitroso compounds from nitrate-derived nitrite. Hence, controlling NDEA and other nitrosamines simultaneously is vital to reduce the risk of genetic toxicity.
Carcinogenicity of nitrosamines DBPs
Nitrosamines DBPs, particularly NDMA and NDEA, are frequently found in various foods and drinking water. In China, the average NDMA intake is approximately 251 ng/capita/day, significantly higher than that of France (188 ng/day) (Li et al., 2021a), Canada (87.6 ng/day) (Hrudey et al., 2013), and the United States (136 ng/day) (Fristachi and Rice 2007). Estimates suggest that drinking water could contribute 1.3%, 10.8%, and 13.1% of the exogenous intake of NDEA, total volatile NAs, and NDMA, respectively (Li et al., 2021a). Currently, the International Agency for Research on Cancer has listed NDEA and NDMA as probable human carcinogens (2A), NDPhA as a Group 3 agent, and 6 other NAs (NDPA, NDBA, NMEA, NMOR, NPIP, and NPYR) as probable carcinogens (2B) (Wang et al., 2022), prompting concerns about drinking water safety and public health. However, the Group 2B carcinogen has limited evidence of causing cancer in humans. Thus, further prospective epidemiological studies and animal experiments are essential to provide reliable evidence.
Nitrosamines DBPs have the potential to induce different types of tumors based on their metabolic rate and structure (Edler et al., 2014; Swenberg et al., 1991). For instance, symmetrical nitrosamines DBPs primarily contribute to liver cancer, whereas asymmetrical nitrosamines are linked to esophageal squamous cell carcinoma (ESCC) (Wang et al., 2023a). Additionally, metabolic activation systems and repair mechanisms might operate at different rates in various organs and species. As mentioned previously, nitrosamines DBPs are predominantly metabolized in the liver—CYP2E1 is highly expressed in this organ. Other tissues with lower expression levels of CYP2E1 are kidney cortex, gastrointestinal tract, lungs, ovary, nasal mucosa, brain, and testis (Blum et al., 2023). Epidemiological studies have shown that high levels of nitrosamine DBPs increase the risk of bladder cancer (Walse and Mitch 2008), ESCC (Zhao et al., 2019), liver cancer (Mitacek et al., 1999), and prostate cancer (Morales-Suarez-Varela et al., 1995), such as nitrosamines DBPs attributable risk reached at 54.3% in ESCC (Wang et al., 2023a). Research on the mechanism of carcinogenesis suggested that nitrosamines were initially metabolically activated by P450 enzymes, generating reactive electrophilic intermediates causing DNA alkylation damage, whereas intermediate metabolites such as free radicals and inducible nitric oxide synthase can also trigger a series of genetic mutations, chromosomal damage, and impaired DNA repair, ultimately leading to cancer (Stoner et al., 2007). Carcinogenesis is a progressive process involving changes in genes, proteins, circulating metabolites, cellular functions, and immune response in normal cells, accumulating and progressing to an aggressive tumor. However, understanding the role of nitrosamines DBPs in tumor development requires a thorough comprehension of the dynamic molecular changes during nitrosamine-induced carcinogenesis. However, to elucidate the role of nitrosamines DBPs in tumor development requires a thorough understanding of the dynamic molecular changes in the process of nitrosamine-induced carcinogenesis.
Recent studies reveal that nitrosamines DBPs could trigger tumor growth by a series of molecular events, including gene mutation, abnormal coding RNA expression, and competing endogenous RNA regulatory networks (Figure 3). It is generally accepted that the carcinogenic effects of nitrosamines DBPs mainly associated with their active electrophilic intermediates, leading to DNA alkylation damage and gene mutation (Stoner et al., 2007). For instance, connexin32 (Cx32, G—> A transition mutation at codon 220) (Omori et al., 1996), β-catenin (exon 3) (Yamada et al., 2005), and Ras (G—> A mutation at codon 12) mutation induced by nitrosamines DBPs, leading to cell cycle arrest (Figure 3A). Further, an increasing number of toxicological experiments verified nitrosamines DBPs can induce the precancerous lesions in the different tissues of rats and trigger coding RNA (Figure 3B). Khan et al. (2023) and Li et al. (2019a) revealed that DEN-medicated SOCS1 and reveal GSTZ1 deficiency can promotes HCC proliferation via activation of the KEAP1/NRF2 pathway. Additionally, nitrosamines DBPs can also regulate tumor immunity and inflammatory response, such IL-1β (Lee et al., 2014) and TNF-α (Badawy et al., 2022). The NAs standard mixture, with equal amounts of NDMA, NMEA, NDEA, NDPA, NDBA, NMor, NPyr, NPip, and NDPhA, triggered pyroptosis in Het-1A cells through the NLRP3/caspase-1/GSDMD pathway (Liu et al., 2022). Although gene mutations play an important role in tumorigenesis, epigenetic modifications are more common, and they can regulate the expression of proto-oncogenes and anti-oncogenes. As our preliminary research revealed that nitrosamines-induced downregulation of LncRNA-UCA1 caused esophageal carcinogenesis by altering FGFR2 alternative splicing (Wang et al., 2023a). Further, prolonged exposure to nitrosamines can also promote epithelial-mesenchymal transition (EMT) process and cell proliferation by circ_Foxo3/miR-191-5p/caspase3 (Wang et al., 2019), miR-411/MLLT11 (Jin et al., 2018), and miR-17-92/NTN4 axis (Figure 3C) (Zhu et al., 2015). However, these studies often focus on limited targets or pathways, making it challenging to explain the complete molecular network changes during carcinogenesis. Hence, a comprehensive understanding of systemic body regulation is crucial to explore the mechanism behind cancer development due to nitrosamines DBPs.
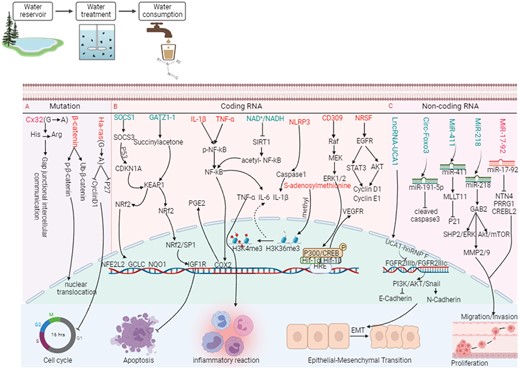
The mechanism pathway of nitrosamines DBPs involvement in tumor progression (created in BioRender.com).
Tumor metabolism abnormalities induced by nitrosamines DBPs
In 1927, Otto Warburg, the Nobel Prize winner in Medicine or Physiology, discovered a distinct metabolic pattern in tumor tissues compared with normal tissues, revealing cancer cells’ preference for robust aerobic glycolysis to generate lactate, even in the presence of adequate oxygen (Warburg et al., 1927). Regrettably, tumor metabolism received limited attention until recently when it was recognized as a critical aspect of cancer, playing a vital role in tumor initiation and progression (Zhang et al., 2013). Ongoing research has shown that tumor cells can reprogram glucose metabolism, amino acid, lipid, and nucleic acid metabolism (Levine and Puzio-Kuter 2010; Oermann et al., 2012; Zhang et al., 2012b), which fulfills the energetic, biosynthetic, and redox demands of rapidly dividing tumors (Reina-Campos et al., 2017). Notably, the tumor initiation is driven in part by metabolism-related genes alterations and genomic DNA mutations. For example, overexpressing GLDC enhances pyrimidine synthesis, promoting cell proliferation, colony formation, and initiating nonsmall-cell lung cancer (Zhang et al., 2012b); Recent studies also highlight MYC mutation as a pivotal oncogenic metabolic driver for metabolic reprogramming and tumorigenesis (Zhang et al., 2012b). Furthermore, certain metabolites are identified as contributors to tumor initiation and progression by acting as signaling molecules. Excessive fumarate, for instance, can induce the EMT phenotype by regulating epigenetics (Gomes et al., 2020). Notably, research reveals that an accumulation or deficiency of metabolic intermediates is linked to environmental exposure, such as bisphenol S (Huang et al., 2020), PM2.5 (Cui et al., 2019), Heavy metal (Li et al., 2019c), Nanosilver (Chen et al., 2014), and dioxin (Chen et al., 2014). However, for nitrosamines DBPs, although some studies have reported that its can mediate metabolism of lipids (Cheng et al., 2017; Zhao et al., 2022a), metabolism of carbohydrates (Bliemsrieder et al., 2021), and metabolism of amino acids and derivatives (Luo et al., 2022) homeostasis imbalance (Table 2), the tumor metabolic turbulence caused by nitrosamines DBPs has not been fully explained.
Tumor type . | Study type . | Subjects . | The levels of nitrosamines DBPs . | Metabolites . | Metabolic pathways . | |
---|---|---|---|---|---|---|
Colorectal cancer (Luo et al., 2022) | Case-control study | Human (plasma samples) |
| Downregulation |
|
|
Upregulation |
| |||||
Esophageal squamous cell carcinoma (Zhao, 2021) | Case-control study | Human (plasma samples) |
| Downregulation |
|
|
Upregulation | Dihydrosphingosine; sphingosine-1-phosphate; dihydrosphingosine-1-phosphate; quinolinic acid | |||||
Esophageal squamous cell carcinoma (Zhang et al., 2022a) | Experimental Study | Malignant transformation Het-1a cells | MNNG (2 μmol/l) | Downregulation | Cosapentaenoic acid |
|
Upregulation |
| |||||
Esophageal squamous cell carcinoma (Zhang et al., 2022c) | Experimental Study | Mice (serum and urine samples) |
| Downregulation |
|
|
Upregulation | Palmitoylcarntine | |||||
Esophageal squamous cell carcinoma (Zhao et al., 2022a) | Experimental Study | Mice (serum samples) | NMBA (0.4 mg/kg) | Downregulation |
|
|
Upregulation |
| |||||
Hepatocellular carcinoma (Ding et al., 2020) | Experimental Study | Mice (liver tissues samples) | DEN (100 mg/l DEN in drinking water) | Upregulation | Proline | Proline metabolic pathway |
Bladder cancer (Afify et al., 2021) | Experimental Study | Mice (bladders tissues samples) | BBN (0.05% in drinking water) | Upregulation |
|
|
Hepatocellular carcinoma (Yang et al., 2018) | Experimental Study | Mice (serum and urine samples) | DEN (50 mg/kg DEN intraperitoneal injection) | Downregulation |
| — |
Upregulation | Acetate; glutamate; tyrosine | |||||
Hepatocellular carcinoma (Wang et al., 2022) | Experimental Study | Mice (urine samples) | DEN (60 mg/l DEN intraperitoneal injection) | Upregulation |
|
|
Hepatocellular carcinoma (Peng et al., 2015) | Experimental Study | Mice (liver tissues samples) | DEN (80 mg/l DEN intraperitoneal injection) | Downregulation |
|
|
Upregulation | l-glutamine; l-aspartate; malate | |||||
Hepatocellular carcinoma (Haberl et al., 2021) | Experimental Study | Mice (liver tissues samples) | DEN (25 µg/g DEN intraperitoneal injection) | Downregulation |
| — |
Upregulation |
| — | ||||
Hepatocellular carcinoma (Chen et al., 2019) | Experimental Study | Mice (liver tissues samples) | DEN (5 μg/g DEN intraperitoneal injection) | Downregulation | α-Glucose; β-glucose |
|
Upregulation |
|
Tumor type . | Study type . | Subjects . | The levels of nitrosamines DBPs . | Metabolites . | Metabolic pathways . | |
---|---|---|---|---|---|---|
Colorectal cancer (Luo et al., 2022) | Case-control study | Human (plasma samples) |
| Downregulation |
|
|
Upregulation |
| |||||
Esophageal squamous cell carcinoma (Zhao, 2021) | Case-control study | Human (plasma samples) |
| Downregulation |
|
|
Upregulation | Dihydrosphingosine; sphingosine-1-phosphate; dihydrosphingosine-1-phosphate; quinolinic acid | |||||
Esophageal squamous cell carcinoma (Zhang et al., 2022a) | Experimental Study | Malignant transformation Het-1a cells | MNNG (2 μmol/l) | Downregulation | Cosapentaenoic acid |
|
Upregulation |
| |||||
Esophageal squamous cell carcinoma (Zhang et al., 2022c) | Experimental Study | Mice (serum and urine samples) |
| Downregulation |
|
|
Upregulation | Palmitoylcarntine | |||||
Esophageal squamous cell carcinoma (Zhao et al., 2022a) | Experimental Study | Mice (serum samples) | NMBA (0.4 mg/kg) | Downregulation |
|
|
Upregulation |
| |||||
Hepatocellular carcinoma (Ding et al., 2020) | Experimental Study | Mice (liver tissues samples) | DEN (100 mg/l DEN in drinking water) | Upregulation | Proline | Proline metabolic pathway |
Bladder cancer (Afify et al., 2021) | Experimental Study | Mice (bladders tissues samples) | BBN (0.05% in drinking water) | Upregulation |
|
|
Hepatocellular carcinoma (Yang et al., 2018) | Experimental Study | Mice (serum and urine samples) | DEN (50 mg/kg DEN intraperitoneal injection) | Downregulation |
| — |
Upregulation | Acetate; glutamate; tyrosine | |||||
Hepatocellular carcinoma (Wang et al., 2022) | Experimental Study | Mice (urine samples) | DEN (60 mg/l DEN intraperitoneal injection) | Upregulation |
|
|
Hepatocellular carcinoma (Peng et al., 2015) | Experimental Study | Mice (liver tissues samples) | DEN (80 mg/l DEN intraperitoneal injection) | Downregulation |
|
|
Upregulation | l-glutamine; l-aspartate; malate | |||||
Hepatocellular carcinoma (Haberl et al., 2021) | Experimental Study | Mice (liver tissues samples) | DEN (25 µg/g DEN intraperitoneal injection) | Downregulation |
| — |
Upregulation |
| — | ||||
Hepatocellular carcinoma (Chen et al., 2019) | Experimental Study | Mice (liver tissues samples) | DEN (5 μg/g DEN intraperitoneal injection) | Downregulation | α-Glucose; β-glucose |
|
Upregulation |
|
Tumor type . | Study type . | Subjects . | The levels of nitrosamines DBPs . | Metabolites . | Metabolic pathways . | |
---|---|---|---|---|---|---|
Colorectal cancer (Luo et al., 2022) | Case-control study | Human (plasma samples) |
| Downregulation |
|
|
Upregulation |
| |||||
Esophageal squamous cell carcinoma (Zhao, 2021) | Case-control study | Human (plasma samples) |
| Downregulation |
|
|
Upregulation | Dihydrosphingosine; sphingosine-1-phosphate; dihydrosphingosine-1-phosphate; quinolinic acid | |||||
Esophageal squamous cell carcinoma (Zhang et al., 2022a) | Experimental Study | Malignant transformation Het-1a cells | MNNG (2 μmol/l) | Downregulation | Cosapentaenoic acid |
|
Upregulation |
| |||||
Esophageal squamous cell carcinoma (Zhang et al., 2022c) | Experimental Study | Mice (serum and urine samples) |
| Downregulation |
|
|
Upregulation | Palmitoylcarntine | |||||
Esophageal squamous cell carcinoma (Zhao et al., 2022a) | Experimental Study | Mice (serum samples) | NMBA (0.4 mg/kg) | Downregulation |
|
|
Upregulation |
| |||||
Hepatocellular carcinoma (Ding et al., 2020) | Experimental Study | Mice (liver tissues samples) | DEN (100 mg/l DEN in drinking water) | Upregulation | Proline | Proline metabolic pathway |
Bladder cancer (Afify et al., 2021) | Experimental Study | Mice (bladders tissues samples) | BBN (0.05% in drinking water) | Upregulation |
|
|
Hepatocellular carcinoma (Yang et al., 2018) | Experimental Study | Mice (serum and urine samples) | DEN (50 mg/kg DEN intraperitoneal injection) | Downregulation |
| — |
Upregulation | Acetate; glutamate; tyrosine | |||||
Hepatocellular carcinoma (Wang et al., 2022) | Experimental Study | Mice (urine samples) | DEN (60 mg/l DEN intraperitoneal injection) | Upregulation |
|
|
Hepatocellular carcinoma (Peng et al., 2015) | Experimental Study | Mice (liver tissues samples) | DEN (80 mg/l DEN intraperitoneal injection) | Downregulation |
|
|
Upregulation | l-glutamine; l-aspartate; malate | |||||
Hepatocellular carcinoma (Haberl et al., 2021) | Experimental Study | Mice (liver tissues samples) | DEN (25 µg/g DEN intraperitoneal injection) | Downregulation |
| — |
Upregulation |
| — | ||||
Hepatocellular carcinoma (Chen et al., 2019) | Experimental Study | Mice (liver tissues samples) | DEN (5 μg/g DEN intraperitoneal injection) | Downregulation | α-Glucose; β-glucose |
|
Upregulation |
|
Tumor type . | Study type . | Subjects . | The levels of nitrosamines DBPs . | Metabolites . | Metabolic pathways . | |
---|---|---|---|---|---|---|
Colorectal cancer (Luo et al., 2022) | Case-control study | Human (plasma samples) |
| Downregulation |
|
|
Upregulation |
| |||||
Esophageal squamous cell carcinoma (Zhao, 2021) | Case-control study | Human (plasma samples) |
| Downregulation |
|
|
Upregulation | Dihydrosphingosine; sphingosine-1-phosphate; dihydrosphingosine-1-phosphate; quinolinic acid | |||||
Esophageal squamous cell carcinoma (Zhang et al., 2022a) | Experimental Study | Malignant transformation Het-1a cells | MNNG (2 μmol/l) | Downregulation | Cosapentaenoic acid |
|
Upregulation |
| |||||
Esophageal squamous cell carcinoma (Zhang et al., 2022c) | Experimental Study | Mice (serum and urine samples) |
| Downregulation |
|
|
Upregulation | Palmitoylcarntine | |||||
Esophageal squamous cell carcinoma (Zhao et al., 2022a) | Experimental Study | Mice (serum samples) | NMBA (0.4 mg/kg) | Downregulation |
|
|
Upregulation |
| |||||
Hepatocellular carcinoma (Ding et al., 2020) | Experimental Study | Mice (liver tissues samples) | DEN (100 mg/l DEN in drinking water) | Upregulation | Proline | Proline metabolic pathway |
Bladder cancer (Afify et al., 2021) | Experimental Study | Mice (bladders tissues samples) | BBN (0.05% in drinking water) | Upregulation |
|
|
Hepatocellular carcinoma (Yang et al., 2018) | Experimental Study | Mice (serum and urine samples) | DEN (50 mg/kg DEN intraperitoneal injection) | Downregulation |
| — |
Upregulation | Acetate; glutamate; tyrosine | |||||
Hepatocellular carcinoma (Wang et al., 2022) | Experimental Study | Mice (urine samples) | DEN (60 mg/l DEN intraperitoneal injection) | Upregulation |
|
|
Hepatocellular carcinoma (Peng et al., 2015) | Experimental Study | Mice (liver tissues samples) | DEN (80 mg/l DEN intraperitoneal injection) | Downregulation |
|
|
Upregulation | l-glutamine; l-aspartate; malate | |||||
Hepatocellular carcinoma (Haberl et al., 2021) | Experimental Study | Mice (liver tissues samples) | DEN (25 µg/g DEN intraperitoneal injection) | Downregulation |
| — |
Upregulation |
| — | ||||
Hepatocellular carcinoma (Chen et al., 2019) | Experimental Study | Mice (liver tissues samples) | DEN (5 μg/g DEN intraperitoneal injection) | Downregulation | α-Glucose; β-glucose |
|
Upregulation |
|
Metabolism of lipids
Abnormal lipid metabolism is a relatively new and increasingly explored area that reveals the pleiotropic effects of various lipid molecules (eg, sphingolipids, saturated and unsaturated fatty acids, and steroids) on tumor initiation and progression (Corbet and Feron 2017). Importantly, our previous epidemiological investigation in the high incidence area of ESCC have revealed an increasing dose-response relationship between nitrosamines DBPs (NMEA and NPyr) and serum sphingosine-1-phosphate (S1P) and sphingolipids (SPH) (Zhao 2019, 2021). Furthermore, based on a rat ESCC model, it was demonstrated that nitrosamines DBPs can disrupt sphingolipid metabolic homeostasis through the regulation of SPHK1-S1P-S1PR1 axis to create a pro-inflammatory immune microenvironment and further initiate tumorigenesis (Zhao et al., 2022a). Notably, S1P is produced from ceramide to sphingosine by ceramidase and is then phosphorylated to S1P by SphK1/2 (Schneider 2020). And intracellular S1P can upregulates Ca2+ concentrations (Himmel et al., 1998), regulates transcription of p21 by binding to histone deacetylases (Hait et al., 2009), or the formation of endoplasmic reticulum stress response protein complex (IRE1α, TRAF2, and RIP1) (Park et al., 2016), which is involved in tumor progression. Meanwhile, S1P/S1PRs also activate STAT3, NF-κB, and Erg-1, stimulating the expression of pro-inflammatory cytokines (Gurgui et al., 2010; Liang et al., 2013). In EMT, S1P/S1PRs also promote MAPK-dependent cell migration and invasion (Sukocheva 2018). These researches shown that sphingolipid metabolism may contribute to tumor development by mediating slow inflammation, immune escape, and EMT. Importantly, sphingolipid metabolism is a key regulatory mechanism in nitrosamines-induced cancer, yet further studies are required to confirm the relevant molecular mechanisms.
External environmental chemicals cause lipid metabolism disruption, leading to imbalances in fatty acid metabolism, potentially identifying fatty acid molecules as biomarkers post-exposure to toxic substances (Goncalves et al., 2021). Simultaneously, research shown a close relationship between the inflammatory process and lipid oxidation, where polyunsaturated fatty acids act as precursors for potent pro-inflammatory and pro-cancer substances (Cai et al., 2012; Rossner et al., 2021). Surprisingly, the nitrosamines DBPs mixture reduced the levels of docosahexaenoic acid and eicosapentaenoic acid, arachidonic acid, palmitic acid, y-linoleic acid, and linoleic acid in rat serum, likely due to the upregulation of CPT1 and downregulation of ACAA2 mediated by nitrosamines DBPs (Zhang et al., 2022d). As essential fatty acids that the human body cannot synthesize, linoleic acid interacts with PPAR to regulate fatty acid metabolism (Li et al., 2021b), reducing the risk of breast (Zhou et al., 2016), colorectal (Deng et al., 2022), and prostate cancers (Yousefi et al., 2023). Notably, γ-linolenic acid demonstrated a protective effect against diethyl-nitrosamine-induced hepatocellular carcinoma (HCC) by altering mitochondrial-mediated apoptosis and an anti-inflammatory pathway (Cui et al., 2018). In addition, palmitic acid, a precursor for the synthesis of numerous unsaturated fatty acids, effectively maintains levels of linoleic and oleic acids, increasing cytotoxicity, inflammatory response, and ROS generation (Ku et al., 2021; Yu et al., 2021b). A Pterospermum lanceifolium fraction enriched with palmitic acid lowered the biochemicals markers (ALT, AST, ALP, and GGT), total bilirubin, TNF-α, carcinoembryonic antigen, interleukins, and reinstated the level of antioxidant enzyme (GSH, GST, catalase, SOD, and GPX), the expression of pro-apoptotic (p53, caspase3/9, and BAX), and anti-apoptotic (Bcl-2) genes in NDEA-induced rats in a dose-dependent manner (Pal et al., 2022). In clinic application, Fuzheng Xiaozheng prescription promoted lipid-related metabolisms and enhanced anti-inflammatory capacity by activating arachidonic acid metabolism in HCC rats induced by diethylnitrosamine (DEN) (Li et al., 2022). In summary, these findings suggest that nitrosamines DBPs might contribute to tumor initiation through the regulation of fatty acid metabolism, offering potential for new chemopreventive agents.
Afify et al. (2021) recently highlighted that early preneoplastic changes involve a significant enrichment of lipid metabolism, specifically phospholipids crucial for plasma membrane structure, in the N-butyl-N-(4-hydroxybutyl)-nitrosamine (BBN) mouse model of chemical carcinogenesis. At different stages of the ESCC, lyso-phosphatidylcholine (LPC) were shown with varying degrees of upregulation at basal cell hyperplasia, light/mild dysplasia, severe dysplasia, carcinoma in situ, and invasive carcinoma (Zhao 2021). It is generally accepted that phospholipids promote immune evasion, angiogenesis, tumor growth, and invasion by mediating the TME (Vecchi et al., 2021). In addition, the dynamic changes in the phospholipid content of cell membranes also provide substrates and secondary messengers for the PI3K axis that links oncogenic signaling to downstream survival, invasive and metabolic programing, and is hyperactivated bladder cancer (Koundouros and Poulogiannis 2020). Conversely, in hepatotoxicity of ICR mice, the nitrosamines DBPs mixture decreased levels of palmitic acid, phosphatidyl cholines, phosphatidylethanolamine, LPC, and lysophosphatidylethanolamine (Zhang et al., 2022b); In animal model of urothelial carcinoma, a lipidomic analysis of isolated mitochondria indicated a significant reduction in phosphatidic acid, phosphatidylglycerol, and cardiolipin induced by BBN (Antunes et al., 2014). This paradoxical result can be interpreted as that nitrosamines DBPs have different effects on different target organs. Even so, these results strongly suggest the potential participation of nitrosamines DBPs in drinking water in tumor carcinogenesis through the regulation of lipid metabolism.
Metabolism of amino acids and derivatives
Amino acid metabolism is part of the altered processes in cancer cells. Currently, an increasing number of studies have substantiated the link between altered amino acid metabolism and the initiation and progression of various cancers like ovarian cancer (Wang et al., 2015), endometrial cancer (Zhai et al., 2023), breast carcinoma (Wang et al., 2023b), and pancreatic cancer (Fu et al., 2023). Surprisingly, differential amino acid metabolites accounted for 11% of the total metabolites induced in rats with nitrosamine-induced ESCC, especially TRP metabolism (TRP, kynurenine, 5-hydroxyindoleacetic acid and 5-hydroxytryptophan [5-HTP] decreased; Indole-3-acetic acid increased) and genes IDO1/TDO2 upregulation. Zhang et al. (2012a) observed considerably lower serum TRP and 5-HTP levels in ESCC patients compared with healthy controls, corroborated by other reports (Naini et al., 1988; Wang et al., 2016). Importantly, the trends in dose-response correlations between urinary NPYR and serum TRP are statistically significant (Zhao 2021). Additionally, IDO knockout, an intracellular enzyme of the L-kynurenine pathway significantly reduces HCC incidence induced by DEN (Avila et al., 2016). In bladder cancer patients, 93% exhibit elevated N-nitrosamine levels, displaying a negative correlation between specific TRP metabolites and nitrosamine content (Chung and Gadupudi 2011). However, the TRP metabolites, such as hydroxyindole acetic acid, indoleacetic acid, and tryptamine, can activate the aryl hydrocarbon receptor, leading to an inflammatory response and facilitating tumor immune escape (Gao et al., 2018). As Zhao et al. reported N-methyl-N-benzylnitrosamine triggers esophageal carcinogenesis by regulating the IDO1/TDO2-AHR and TRP-KYN-NAD axis (Zhao 2021). These results confirm that TRP metabolism is an important metabolic regulatory mechanism in nitrosamines DBPs-mediated tumor development.
Zhao et al. recently demonstrated that methionine restriction prevented HCC tumorigenesis induced by DEN. Furthermore, they identified the aberrant regulation of the mTORC1-c-Myc-SIRT4 axis, dependent on methionine, influencing the progression of HCC (Zhao et al., 2022b). Notably, the highest proline levels were detected in the rapidly growing orthotopic Morris Hepatoma model, whereas the lowest proline levels were found in the slow-growing DEN model, further the proline biosynthetic enzyme PYCR1 knockout also significantly reduces the incidence of HCC induced by DEN (Ding et al., 2020). Additionally, DEN exposure reduced serum concentrations of leucine, isoleucine, valine, glutamine, and glycine, while elevating tyrosine levels (Yang et al., 2018). However, the level of serine in primary hepatocytes can’t be influenced by DEN treatment (Li et al., 2019b). Particularly in cancer treatment, activating Gαi-coupled GABAB-receptor signaling by GABA administration can prevent the development and progression of nitrosamines-induced pancreatic cancer (Schuller 2018). Thus, dietary restriction or supplementation with specific amino acids may be protective against chemical carcinogenesis in the presence of high levels nitrosamine DPBs exposure in drinking water. However, at present, the carcinogenic effect of nitrosamine DPBs through abnormal amino acid metabolism is mainly focused on HCC or ESCC, whereas other malignant tumors will be a new direction for future research.
Metabolism of nucleotides
Genome instability is a common feature in nearly all cancers and often emerges early in tumorigenesis (Hanahan and Weinberg 2011). The lack of nucleotides has emerged as a new explanation for early-stage genomic instability in tumor initiation and progression. However, the insufficiency in nucleotide biosynthetic pathways leads to a low nucleotide pool that cannot support normal DNA replication (Bester et al., 2011). As Li et al. (2019b) reported DEN treatment suppressed de novo nucleotide synthesis, and supplementing dNTPs alleviated DEN-induced DNA damage, cell death, inflammatory response, and cell proliferation in vitro. Furthermore, aberrations in adenine, guanine, and hypoxanthine-xanthine metabolic pathways were closely linked to changes in VNAs, notably NDMA and NPYR, in the plasma of colorectal cancer patients (Luo et al., 2022). Numerous studies have reported purine metabolism produces essential components like adenine, guanine, and nucleotides necessary for cell survival and proliferation, linked to cancer progression (Yin et al., 2018). Xanthine oxidoreductase catalyzes xanthine production from hypoxanthine and generates reactive oxygen species that can trigger cancer cell apoptosis (Ding et al., 2016; Liu et al., 2021a). However, exposure to nitrosamine DPBs resulted in significant downregulation of hypoxanthine and xanthine (Luo et al., 2022). Currently, it is now well accepted that after metabolic activation in the body, nitrosamine DPBs can form active electrophilic reagents that alkylate with DNA bases, leading to DNA damage and ultimately inducing cancer. However, few studies have focused on the nucleotide metabolism pathway during early nitrosamine-induced carcinogenesis. Thus, focusing on the role of nucleotide biosynthesis in DNA repair could provide new insights into the early prevention of chemical carcinogenesis.
Metabolism of carbohydrates
Cancer cells have extensive connections to other cells within the TME, allowing metabolite exchange that supports cell survival, proliferation, metastasis, EMT, and angiogenesis. Carbohydrate metabolism, functioning as a central axis for metabolic and biosynthetic pathways such as amino acid and lipid metabolism and the pentose phosphate pathway, ensures a consistent energy supply in this context (Adhikari et al., 2022). Presently, researchers have turned their focus toward understanding the effects and mechanisms of exogenous environmental chemical exposure on carbohydrates metabolism. Although the relationship between nitrosamine DPBs and carbohydrate metabolism has been confirmed (Table 2), the specific mechanisms remain unclear. As Qi et al. research showed that DEN treatment significantly reduced the levels of tricarboxylic acid (TCA) cycle and promoted the conversion of DL-2-glycerophosphate and phosphoenolpyruvic acid to lactic acid in the rat model of HCC (Qi et al., 2022), suggesting DEN induces the conversion of gluconeogenesis from oxidative phosphorylation to aerobic glycolysis. Furthermore, the nitrosamine-mediated reprogramming of glucose metabolism satisfies the need for rapid supply of adenosine triphosphate during abnormal cell proliferation (Burk and Schade 1956), and also permits the involvement of metabolic intermediates in various biosynthetic pathways, ultimately favoring the synthesis of macromolecules and new organelles, thereby meeting the demand for uncontrolled proliferation and increasing biomass to sustain daughter cell production (Lunt and Vander Heiden 2011; Schulze and Harris 2012). For instance, lactic acid produced via glycolysis promotes glioma cell proliferation by upregulating PLEKHA4 expression (Ye et al., 2023). Similarly, lactic acid stimulates carcinogenesis in susceptible cancer cells by boosting MCT1 expression (Zhang et al., 2023). Nitrosamines can also cause lactic acid accumulation in ESCC (Zhao 2021). In addition, DEN also significantly increased lactate levels in plasma, kidney tissue, liver, and spleen tissue of HCC, whereas the levels of lactate were reduced in brain, heart tissue, and urine (Yalikun, 2019), suggesting nitrosamine DPBs-induced carbohydrates metabolism disorder has tissue specificity. Recently, Liu et al. (2021b) reported that a negative correlation between glycogen levels and tumor volume in DEN-induced rat liver tumors. Further research found that NDEA can downregulate the glucose-6-phosphatase level (Birk et al., 1985; Liu et al., 2021b; Sivalokanathan et al., 2005). Notably, glycogen storage plays a significant role as an oncogenic driver. Accumulated glycogen leads to liquid-liquid phase separation, interfering with Hippo signaling, thus facilitating tumor initiation (Liu et al., 2021b). Consequently, accumulated glycogen might serve as a potential biomarker for early diagnosis and prognosis of cancers induced by nitrosamine DBPs. Moreover, eliminating glycogen could present a potential strategy for chemically induced cancer prevention.
Conclusion
Since the late 20th century, global water environments have deteriorated, leading to increased chlorine disinfectant usage, elevating the presence of nitrosamine DBPs in drinking water, posing significant health risks. Currently, nitrosamine DBPs, categorized as a new UDBP, are confirmed to have genotoxic and carcinogenic properties. However, due to limited monitoring data on nitrosamine DBPs in drinking water and a lack of information on human exposure and effects, there is currently no globally established health standard for nitrosamine DBPs in drinking water, or the existing standards are much higher than their toxic effects dose, which means that even low doses of continuous exposure to nitrosamine DBPs may initiate the development of various solid tumors. Therefore, a more comprehensive survey to better assess nitrosamine DBPs risk in the water supply in worldwide is recommended.
During tumorigenesis, the metabolites are the end products of signal transduction pathways, and their information is closer to the final phenotype, making them more conducive to cancer classification and the discovery of biomarkers. However, recent discoveries have highlighted that the accumulation of abnormal metabolic intermediates is a significant signaling event that drives tumor development. Significantly, low doses of continuous exposure to nitrosamine DBPs can trigger tumor initiation by regulating abnormalities in glucose metabolism, lipid metabolism, and amino acid metabolism, whereas the potential mechanism of carcinogenesis remains unclear. Herein, there is a need for future studies to decipher the role and mechanism of nitrosamines DBPs in triggering tumor initiation and progression through the regulation of metabolic disorders. It is worth mentioning that researchers have increasingly focused on the role of nitrosamines in conjunction with novel pollutants in the initiation of tumorigenesis, such as heavy metal (Dai et al., 2017), pathogenic microorganism (Zhang et al., 2020a; Zhou et al., 2022), and microcystins (Bond et al., 2011). However, the synergistic carcinogenesis of nitrosamines and novel pollutants through metabolic reprogramming needs to be further researched.
Meanwhile, policy adaptations are crucial to establish guidelines for limiting nitrosamine DBP contamination in water sources. For instance, economically viable technologies should be developed for DBP reduction. The stepwise chlorination approach is a viable method as it reduces DBP formation, increases disinfection efficiency, and lowers operational costs. In the field of cancer prevention in chemistry, collaboration between public health experts and clinical doctors is vital to propose practical dietary supplements to compensate for nutrient deficiencies caused by chemical carcinogens’ toxic effects on the body.
Declaration of conflicting interests
The authors declared no potential conflicts of interest with respect to the research, authorship, and/or publication of this article.
Acknowledgment
The authors acknowledge the use of Biorender that is used to create schematic all figures.
Funding
National Natural Science Foundation of China (82173479, 81872579, 81573108); New Century Excellent Talents in University from Ministry of Education (NCET-13-0124).
Author contributions
Ran Liu (Conceptualization, Supervision, Project administration, Funding acquisition, Writing—review & editing), Mingjun Sun (Conceptualization, Data curation, Writing—original draft), Weitao Shen (Conceptualization, Data curation, Writing—original draft), Xinxin Guo (Conceptualization, Data curation, Writing—original draft), Yinghao Liao (Data curation, Writing—original draft), Yang Huang (Data curation, Writing—original draft), Mohan Hu (Data curation), and Ping Ye (Data curation)
Comments